the Creative Commons Attribution 4.0 License.
the Creative Commons Attribution 4.0 License.
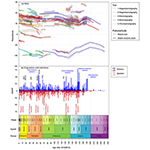
DINOSTRAT: a global database of the stratigraphic and paleolatitudinal distribution of Mesozoic–Cenozoic organic-walled dinoflagellate cysts
Mesozoic–Cenozoic organic-walled dinoflagellate cyst (dinocyst) biostratigraphy is a crucial tool for relative and numerical age control in complex ancient sedimentary systems. However, stratigraphic ranges of dinocysts are found to be strongly diachronous geographically. A global compilation of state-of-the-art calibrated regional stratigraphic ranges could assist in quantifying regional differences and evaluating underlying causes. For this reason, DINOSTRAT is here introduced – an open-source, iterative, community-fed database intended to house all regional chronostratigraphic calibrations of dinocyst events (https://github.com/bijlpeter83/DINOSTRAT.git, last access: 1 February 2022) (DOI – https://doi.org/10.5281/zenodo.5772616, Bijl, 2021). DINOSTRAT version 1.0 includes >8500 entries of the first and last occurrences (collectively called “events”) of >1900 dinocyst taxa and their absolute ties to the chronostratigraphic timescale of Gradstein et al. (2012). Entries are derived from 199 publications and 188 sedimentary sections. DINOSTRAT interpolates paleolatitudes of regional dinocyst events, allowing evaluation of the paleolatitudinal variability in dinocyst event ages. DINOSTRAT allows for open accessibility and searchability, based on region, age and taxon. This paper presents a selection of the data in DINOSTRAT: (1) the (paleo)latitudinal spread and evolutionary history of modern dinocyst species, (2) the evolutionary patterns and paleolatitudinal spread of dinocyst (sub)families, and (3) a selection of key dinocyst events which are particularly synchronous. Although several dinocysts show – at the resolution of their calibration – quasi-synchronous event ages, in fact many species have remarkable diachroneity. DINOSTRAT provides the data storage approach by which the community can now start to relate diachroneity to (1) inadequate ties to chronostratigraphic timescales, (2) complications in taxonomic concepts, and (3) ocean connectivity and/or the affinities of taxa to environmental conditions.
- Article
(13138 KB) - Full-text XML
- Companion paper
-
Supplement
(33200 KB) - BibTeX
- EndNote
Over 50 years of research efforts has established a framework to use organic-walled dinoflagellate cysts (dinocysts) as biostratigraphic and chronostratigraphic tools. Dinocyst biostratigraphy is particularly applied to sediments which are difficult to date otherwise, such as in restricted nearshore marine settings (e.g., Poulsen, 1994; Brinkhuis et al., 1998; Iakovleva et al., 2001; Śliwińska et al., 2012; Clyde et al., 2014) and polar regions (e.g., Sluijs et al., 2006; Bijl et al., 2013a; Houben et al., 2013; Radmacher et al., 2015; Śliwińska et al., 2020; Nøhr-Hansen et al., 2020). As with all biostratigraphy, the reliability of dinocyst biostratigraphy heavily depends on the accuracy, precision and regional consistency of the numerical ages of the first and last stratigraphic occurrences (FOs and LOs, hereafter jointly referred to as “events”) of easily recognized taxa. Through the past decades, numerical ages of dinocyst events have become increasingly better chronostratigraphically constrained, using independent age control from magnetostratigraphy (e.g., Brinkhuis et al., 1992; Powell et al., 1996), other biostratigraphic tools (e.g., Davey, 1979; Leereveld, 1997a, b; Oosting et al., 2006; Awad and Oboh-Ikuenobe, 2019) and astrochronology (Versteegh, 1997). However, efforts to compile a global chronostratigraphic calibration of dinocyst events have revealed strong diachroneity for many species between broad latitudinal bands and endemism of many species within latitudinal bands (e.g., Williams et al., 2004). Because this impacts the development of quasi-global dinocyst zonation schemes, as have been proposed for other microfossil groups (e.g., Martini, 1971; Gradstein et al., 2020), the question is, how should the research field of dinocyst biostratigraphy progress?
Two questions arise from the notion of the geographic diachroneity of dinocyst events.
-
What kind of error or uncertainty should be applied to the numerical ages of events? Now that diachroneity has been demonstrated, the next step is to quantify the uncertainty in numerical ages of dinocyst events for each species and to assess regional consistency. This is particularly important when calibrated species ranges are geographically extrapolated over large distances. And a related question is, what is the impact of regional variability in the numerical ages of events on the regional consistency of the stratigraphic order of events?
-
What are the underlying causes for the observed diachroneity? Broadly, three reasons could apply: (1) inaccurate or inadequate tie-in of dinocyst events to the chronostratigraphic timescale can lead to apparent (but perhaps false) diachroneity of species events between sites. (2) Complexities in taxonomic concepts could obscure comparison of species ranges between sites. This aspect relates to the ease by which subtle morphological differences between species can be recognized (e.g., Hoyle et al., 2019). It also relates to the question of whether the last occurrence of a fossil dinocyst taxon reflects extinction of its producer, adjustment of cyst morphology by its producer (e.g., Rochon et al., 2009), or a change in its life cycle strategy (e.g., towards less preservable pellicle cysts; Bravo and Figueroa, 2014). (3) Finally, paleoenvironmental/paleoceanographic conditions can impact species occurrence: ocean connectivity (Van Simaeys et al., 2005; Bijl et al., 2013b; Van Helmond et al., 2016), leads and lags in the biotic response to climate change (e.g., Sluijs et al., 2007), or the temperature affinity of dinocyst taxa (Van Simaeys et al., 2005; Van Helmond et al., 2016). For instance, in geologic time intervals of global climate cooling, warm-loving plankton species have diachronous last occurrences which are progressively later at lower latitudes. A good example is the modern occurrence in the western Pacific warm pool of Dapsilidinium pastielsii, a species that was long thought to be extinct in the Pliocene (Head et al., 1989). This example serves as an indicator that asynchronous biostratigraphic events could actually be the result of paleoceanographic or paleoecologic influences, rather than just biostratigraphic error.
A process that takes us towards answering these questions and improving the accuracy of dinocyst biostratigraphy requires a data compilation approach that incorporates data from as many sites as possible, with detailed metadata on the paleogeographic evolution of sites and the means of chronostratigraphic calibration. It further requires that such data compilations are constantly updated with new insights: updates to the geologic time scale and bio-magnetostratigraphic zonation schemes, altered taxonomic concepts, age models of sections, and stratigraphic sections. A complication on a logistical front is that dinocyst ranges are typically published in the closed-access peer-reviewed literature, which is not easily accessible to all, is inconsistent in its approach and is easily updated with new insights.
This paper introduces DINOSTRAT, an open-source, online platform intended to house, disseminate and iteratively update all published chronostratigraphic calibrations of dinocyst ranges: the way in which they are tied to the chronostratigraphic timescale and the (paleo)geographic position of the site from which they were calibrated. DINOSTRAT version 1.0 currently contains over 8500 entries of the first and last occurrences of over 1900 dinocyst taxa tied to the international timescale. These entries originate from 199 peer-reviewed papers presenting data from 188 sites. Including as many reports/sites as possible, with verifiable independent age control and their latitudinal evolution through time, allows for proper evaluation of error and uncertainty. DINOSTRAT will allow assessment and quantification of regional variability/consistency in event ages and provides the basic information to evaluate the paleoceanographic signal that diachroneity may hold. Open accessibility of the basic dinocyst stratigraphic data will further allow a proper evaluation and update of evolutionary patterns in dinocyst families (MacRae et al., 1996) with full disclosure of the underlying data. The approach to the selection of appropriate data and entry and calculations of ages and paleolatitudes is explained in Sect. 2. Section 3 presents examples of calibrated dinocyst events: the stratigraphic and paleolatitudinal distribution of selected modern dinocysts and that of extant and extinct dinocyst families, with selected taxa highlighted. Section 4 discusses the implications of the DINOSTRAT approach and future directions. This paper represents the start of a community-fed data assembly approach to iteratively improve regional constraints on dinocyst biostratigraphy.
DINOSTRAT version 1.0 represents a compilation of dinocyst events from peer-reviewed literature, with a publication date predating 1 January 2021 (see Table 1). The taxonomic nomenclature, supra-generic classification and synonymy cited in Williams et al. (2022) are followed. One inherent assumption in the initial setup of DINOSTRAT is that the authors of the reviewed literature have applied a consistent taxonomic framework. DINOSTRAT reports events of dinocyst species as they were presented in the papers but applying the synonymy index of Williams et al. (2022). Most dinocyst species are easily recognized, have a stable morphology (both regionally and through time) and clearly defined species concepts. However, some species (and subspecies) diagnoses are more subtle or represent endmembers in a continuum (e.g., Hoyle et al., 2019), in part imposed by environmental conditions (e.g., Ellegaard, 2000). Some authors tend to lump species in complexes, while others split them into subspecies. The international recognition of these lumps and splits may have evolved through time and may have restricted regional significance only. Therefore, subtle differences in species concept interpretation may exist between authors and regions, which the current approach was unable to account for, and identifying them is considered a next step for when individual studies or sites are revisited.
For the subfamily Wetzelielloideae, DINOSTRAT deviates from the taxonomic index of Williams et al. (2022). The fundamental redefinition of species concepts in the taxonomic revisions for the Wetzelielloideae (Williams et al., 2015) eliminates many stratigraphically useful Eocene dinocyst taxa (Bijl et al., 2016). Therefore, for this subfamily, the calibration of dinocyst species is presented in the taxonomic classification of Wetzelielloideae prior to Williams et al. (2015).
Table 1Papers used in this review. Reference, geography, age base and age top (in Ma), tier (see Fig. 1), and means of calibration to the geologic time scale (GTS). The abbreviations of the microplankton zones indicated in the column “Calibrated to” (NJ, NC, NP, NN, CC, CP, CN, UC, N, P, E) are those commonly used in the literature and relate to the zones presented in the GTS2012 (Gradstein et al., 2012).

A decision tree has been used to determine which papers to include in DINOSTRAT (Fig. 1). This tree first discards studies in which dinocysts were the only stratigraphic tool to date the sequence. Although these papers do provide valuable information on the stratigraphic order of events, discarding them from this review eliminates the risk of circular reasoning and inherited chronostratigraphic ties. Only those dinocyst events are included that could be calibrated against a stratigraphic tool that can be traced back to the bio-, magneto- or chronozones in the geologic time scale 2012 (GTS2012; Gradstein et al., 2012). This decision tree can be used to define five tiers of reliability in these papers (Fig. 1):
-
Tier 1 studies present dinocyst events along with magnetostratigraphic constraints obtained from the same sedimentary section. The interpretation of magnetochrons from the paleomagnetic signal was performed without the use of dinocyst biostratigraphy. Since magnetic reversals are globally synchronous, evaluating the synchroneity of dinocyst events with the use of paleomagnetostratigraphy is most robust.
-
Tier 2 studies present dinocyst events calibrated along with compromised or problematic magnetostratigraphic constraints on the same sedimentary section, for instance when the inclination signal suffers from a strong overprint or when the magnetochron assignment is not clear. Studies in which dinocyst events served as biostratigraphic tools for magnetochron assignment are included in this tier as well.
-
Tier 3 studies report dinocyst events together with biostratigraphic zones (from nannoplankton, foraminifer or ammonite zones) identified in the same sequence. These studies provide clear reports on the identification of these zones in the sequence.
-
Tier 4 studies report dinocyst events with biostratigraphy, of which either the derivation is unclear or the tie to the geologic time scale (GTS; e.g., for outdated ammonite zonations) or biostratigraphic data does not come from the same sequence but, for example, has been interpreted from nearby outcrops.
-
Tier 5 studies report dinocyst events with independent chronostratigraphy, of which the derivation is unverifiable or represents a regional synthesis.
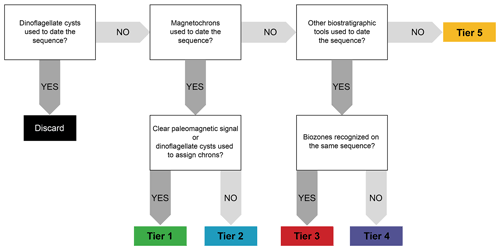
Figure 1Decision tree for including studies in this review and categorization criteria for the five tiers.
The numerical age of each dinocyst event is not explicitly entered into DINOSTRAT. Rather, its position within the zone it was calibrated to is entered. Ages are subsequently calculated via linear interpolation between these tie points, as follows:
in which [##]% is linearly interpolated between the base (0 %) and top (100 %) of tie points; [stratigraphic tool]$[zone] is the name of the zone in the bio-magneto- or chronozonation in GTS2012 in which the dinocyst event falls. The rationale behind this approach instead of simple entry of the age is that while the numerical ages of dinocyst events are dependent on the evolving knowledge of the chronostratigraphic timescale, the stratigraphic position of the event relative to the tie points in the record is fixed. This approach makes it easier to update the ages of the dinocyst events when the ages of the chrono-, magneto- and biozones are updated in the future. If dinocyst events fall between two different stratigraphic ties, the event is noted as follows:
Outdated Jurassic and Cretaceous ammonite zonation schemes have been converted to those presented in GTS2012 (see Supplement File 1; following Ogg and Hinnov, 2012a, b, and citations therein). FOs in the bottom of sections and LOs at the top of sections are systematically omitted, unless they were specifically indicated to represent an FO or LO. More recent publications presenting calibrations of dinocyst species from the same section overwrite older publications. Extant dinocyst species and their latitudes (from Marret et al., 2020, and Mertens et al., 2014, for Dapsilidinium pastielsii) are entered with an LO of 0 Ma (modernst.csv in Bijl, 2021, for surface sediment station locations, modernsp.csv in Bijl, 2021, for dinocyst species at those stations).
Each event entry in DINOSTRAT (Dinoevents_Jan2021.csv in Bijl, 2021) includes the (paleo)latitude of that event. This is interpolated using the age of the event and its location, which has a paleolatitude evolution through time (Paleolatitude.csv in Bijl, 2021; with use of http://paleolatitude.org, last access: 1 February 2022; Van Hinsbergen et al., 2015). Paleolatitudes of sites in mobile orogenic belts are interpolated using regional tectonic reconstructions and as such are prone to additional latitudinal uncertainty.
3.1 Sites
DINOSTRAT currently contains dinocyst events from 199 publications and 188 sites. The wider North Atlantic–European area is strongly overrepresented (Fig. 2). Few sites are from the Pacific Ocean, the southern Atlantic and Indian Ocean, and the equatorial region. This probably reflects a genuine bias in the available information because of focus of the community towards economically interesting regions (e.g., for hydrocarbon industry). It may also in part reflect a bias towards research from developed nations and poor accessibility of publications from non-western societies.
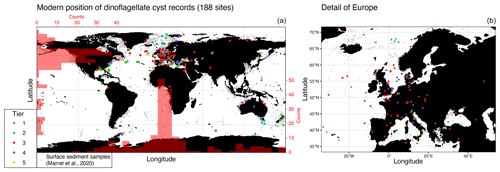
Figure 2Present-day geographic distribution of sedimentary sequences used in DINOSTRAT (colors of the dots correspond to the to the tier status to which the site has been allocated) and surface sediment stations (in grey dots; Marret et al., 2020; Mertens et al., 2014). (a) Global. Red scale bars on x and y axes represent the number of sites per longitudinal and latitudinal bin, respectively. (b) Detailed map of sites in Europe.
The paleolatitudinal position of the sites through time confirms the strong overrepresentation of Northern Hemisphere mid-latitude sections (Fig. 3) and underrepresentation of the tropical regions, Pacific Ocean and southern mid-latitudes. The Paleogene has the largest latitudinal spread of records. The Mesozoic in particular has few entries from the Southern Hemisphere or equatorial regions. The Mesozoic records are predominantly calibrated to ammonite stratigraphy (tiers 3 and 4) and on some occasions to magnetostratigraphy (tiers 1 and 2; Fig. 3). Ammonite zones presented in the papers often had to be converted to those in GTS2012, which is not always straightforward, as the zone definitions have changed through time (Ogg and Hinnov, 2012a, b). The ammonite zonations are prone to regional diachroneity, which was demonstrated particularly for the Late Jurassic (Ogg and Hinnov, 2012b). This may create a level of circular reasoning when dinocyst events are calibrated against these zones because diachronous dinocyst events in DINOSTRAT may be the result of diachronous ammonite zones rather than actually being diachronous dinocyst events.
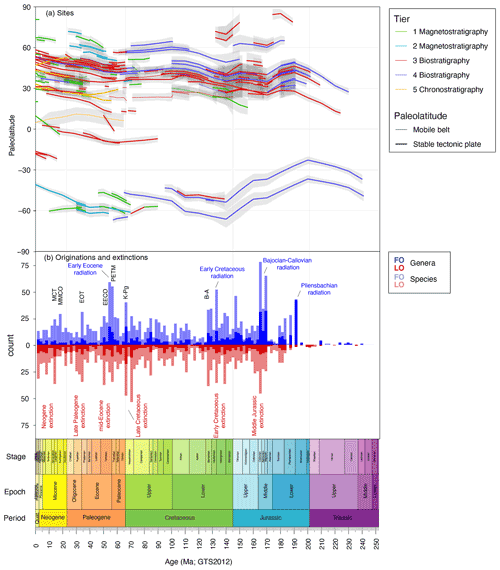
Figure 3Data in DINOSTRAT. (a) Paleolatitude and age span of sites used in DINOSTRAT. Colors correspond to tier; line thickness separates sites on stable oceanic or continental plates from those in mobile orogenic belts. Grey envelopes represent the error of the paleolatitude reconstruction inherited from the paleomagnetic reference frame (see Van Hinsbergen et al., 2015). (b) Dinocyst events in DINOSTRAT, filtered for oldest FOs (blue) and youngest LOs (red) of dinocyst species (lighter shade) and genera (darker shade), in 2 Myr bins. Several phases of climatic environmental change are highlighted in black: Barremian–Aptian boundary (B–A), Cretaceous–Paleogene boundary (K–Pg), Paleocene–Eocene boundary (PETM), Early Eocene Climatic Optimum (EECO), Eocene–Oligocene transition (EOT), Mid-Miocene Climatic Optimum (MMCO) and Miocene climatic transition (MCT). Extinction and radiation phases in dinocysts are highlighted in red and blue text, respectively.
3.2 Calibrated dinocyst events
DINOSTRAT version 1.0 includes over 8500 entries of calibrated dinocyst events (excluding the modern dinocyst database). On a species level, originations in DINOSTRAT peak in the Middle Jurassic (Bajocian–Callovian), the Early Cretaceous (late Valanginian–Barremian) and the Eocene (Ypresian; Fig. 3b). Extinctions peak in the Early Cretaceous (Berriasian–Barremian), Upper Cretaceous (Maastrichtian), Oligocene (Rupelian) and Miocene (Serravallian; Fig. 3b). This pattern is generally followed on a generic level, which likely has a stronger relation to the biologic diversity than dinocyst species diversity (Fensome et al., 1993).
The interpolated paleolatitudes for dinocyst events in DINOSTRAT allow detailed evaluation of the latitudinal synchroneity of dinocyst events. This paper presents a selection of the data in DINOSTRAT, focusing on the stratigraphic and geographic range of modern dinocyst species, of dinocyst families/subfamilies and of a selection of quasi-synchronous dinocyst events. Users can filter DINOSTRAT by locality (to present the stratigraphic order of events per site) and/or by taxon (to see the geographic variability in the range of any taxon), to serve their purposes.
3.2.1 The stratigraphic range of modern dinocyst species
Modern dinocysts from surface sediment samples (Marret et al., 2020, n=3600, and Mertens et al., 2014, n=5) have a species-specific latitudinal spread. Sea surface temperature and nutrient conditions are the main controlling factors on modern assemblage compositions (Zonneveld et al., 2013). The database presented here allows comparison of the modern latitudinal spread of these species to that of the past and of their age and the latitude of their oldest first occurrence (Supplement File 2 and a selection in Fig. 4). Most modern species that have entries in DINOSTRAT have originations in the mid-Cenozoic: Impagidinium species, Operculodinium centrocarpum, Tectatodinium pellitum and Tuberculodinium vancampoae (Fig. 4). Lingulodinium machaerophorum has a first occurrence at around 60 Ma. The exception is Spiniferites ramosus, a generalist species with a robust morphology through time that has a remarkably consistent FO in the Berriasian (∼ 145 Ma; Fig. 4). The dinocyst species that have geographic distributions restricted to one hemisphere today were also latitudinally restricted in the geologic past (e.g., Spiniferites elongatus, Trinovantedinium variabile; Fig. 4). Achomosphaera andalousiensis, Dapsilidinium pastielsii, Impagidinium velorum, Melitasphaeridium choanophorum, Tectatodinium pellitum and Tuberculodinium vancampoae had wider latitudinal distributions until the recent past, across both hemispheres. Melitasphaeridium choanophorum had progressively older LOs north and south of its restricted modern latitudinal distribution in northern mid-latitudes. Lingulodinium machaerophorum and Polysphaeridium zoharyi had a higher paleolatitudinal occurrence in only one hemisphere. Several modern taxa (e.g., Bitectatodinium spongium, Polykrikos spp., Protoperidinium spp., Echinidinium spp., most Islandinium species, most Stelladinium species, Polarella glacialis) have no entry yet in DINOSTRAT. This could be because some species concepts are relatively novel or have poor preservation potential in the fossil record (e.g., because of selective degradation; Zonneveld et al., 2010).
3.2.2 Dinocyst (sub)families
Range charts of the sites in DINOSTRAT are provided in the Supplement (see “Sites” folder in Supplement File 2). The age-over-paleolatitude entry in DINOSTRAT allows evaluation of the latitudinal difference in event ages for each individual species in DINOSTRAT (n=1914), as well as for groupings per genus (n=460) and family (n=28) (Supplement File 2). Users can produce/adapt these plots themselves with the help of the R markdown script “plot creator.Rmd” in Bijl (2021). The most robust dinocyst events will have synchronous ages of FOs and LOs per paleolatitude (i.e., vertical blue and red lines in the plots of Supplement File 2). The FOs and LOs connected per species and grouped in (sub)families are plotted and described below, with particularly synchronous taxa highlighted. The purpose of these plots is threefold: first, they show the total stratigraphic range and latitudinal spread of these dinoflagellate (sub)families and time intervals of when and where phases of strong diversification and extinction occurred in that (sub)family. Second, as with the plots of modern species, they show not only in which paleolatitudes these supra-generic groups first appear but also where they last became extinct. Although earlier compilations of the evolution of dinocyst families do exist (e.g., MacRae et al., 1996), DINOSTRAT presents the fundamental spatio-temporal observations that underpin these compilations. Thirdly, the plots allow the presentation of the database in such a way that the validity of extrapolating dinocyst events on a supra-regional scale can be critically evaluated in the discussion.
-
Order Gonyaulacales
-
Family Areoligeraceae (Fig. 5)
Range. The Areoligeraceae range from the Bathonian (∼ 168 Ma, FOs of Adnatosphaeridium spp. and Senoniasphaera spp.) to the mid-Miocene (∼ 18 Ma, LO of Chiropteridium galea). Areoligeraceae seem to range for longer in Northern Hemisphere (NH) mid-latitudes (FO ∼ 169 Ma, LO ∼ 18 Ma) than in the rest of the world (FO ∼ 145 Ma, LO ∼ 36 Ma), although this can be in part related to a sampling bias. The oldest FOs in NH mid-latitudes are species with a stratigraphic occurrence restricted to that area.
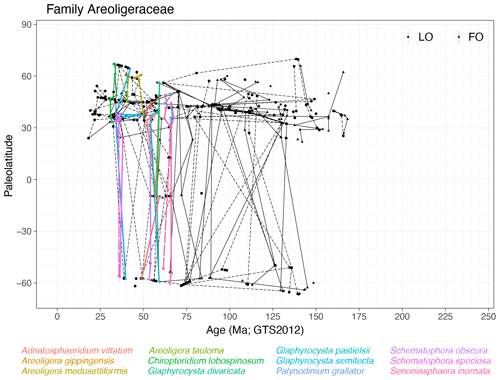
Figure 5Ages and paleolatitudes of first (solid line and triangles) and last (dashed line and circles) occurrences of dinocyst species of the family Areoligeraceae. Solid and dashed lines connect first and last occurrences, respectively, for each species, between sites. Colored lines represent quasi-synchronous species events.
Quasi-synchronous events. There are quasi-synchronous events of species of Areoligera, Chiropteridium, Glaphyrocysta, Palynodinium, Schematophora and Senoniasphaera,, particularly in the Late Cretaceous and Paleogene (Fig. 5). Many taxa in this subfamily however show strongly diachronous events between hemispheres.
-
Family Ceratiaceae (Fig. 6)
Range. The Ceratiaceae first appear in the Tithonian (∼ 152 Ma, FO of Muderongia simplex) in NH mid-latitudes, represent a diverse group in the Early Cretaceous and have an LO in the latest Cretaceous (∼ 66 Ma, LO of Odontochitina operculata).
Quasi-synchronous events. The LO Odontochitina costata, LO Phoberocysta neocomica and range of Pseudoceratium pelliferum are quasi-synchronous (Fig. 6).
-
Family Cladopyxiaceae (Fig. 7)
Range. This family first appears in the Pliensbachian (∼ 188 Ma, FO of Freboldinium spp.) and ranges until the late Oligocene (∼ 25 Ma, Licracysta semicirculata).
Quasi-synchronous events. Several species of Enneadocysta are synchronous, as are LO of Fibradinium annetorpense around 60 Ma and the LO of Licracysta semicirculata around 26 Ma. Most entries in the Late Cretaceous and Paleogene are highly diachronous.
-
Family Goniodomaceae (Fig. 8)
Range. Goniodomaceae first appear in the mid-Tithonian (∼ 150 Ma, FO of Hystrichosphaeridium petilum) in the NH mid-latitudes; most entries are from the Paleogene and continue with the modern species Polysphaeridium zoharyi and Tuberculodinium vancampoae.
Quasi-synchronous events. Species of Alisocysta, Eisenackia, Heteraulacacysta and Homotryblium have quasi-synchronous ranges. Many species ranges in this family are notably diachronous. Although some species do seem to show similar event ages between Southern Hemisphere (SH) high latitudes and NH mid-latitudes (Fig. 8), those with multiple entries in the northern mid-latitudes, where site density is highest, show strong diachroneity over short latitudinal distances. Modern species have a restricted latitudinal spread to subtropical and tropical regions, but not too long ago in the geologic past, species of this family exhibited much wider latitudinal ranges (65∘ S–70∘ N).
-
Family Gonyaulacaceae
-
Subfamily Cribroperidinioideae (Fig. 9)
Range. This subfamily includes the extant species Operculodinium centrocarpum and Lingulodinium machaerophorum. The subfamily first appears in NH mid-latitudes in the Aalenian (∼ 172 Ma) with Kallosphaeridium spp. and in the Bajocian (∼ 169 Ma) with Cribroperidinium spp. and shortly thereafter Aldorfia and Korystocysta. Cribroperidinium is a long-ranging genus. Many entries are from the Early Cretaceous (∼ 125 Ma) and early Paleogene (66–34 Ma).
Quasi-synchronous events. Several species of Cordosphaeridium and Danea and species of Aldorfia, Apteodinium, Carpatella, Cooksonidinium, Diphyes, Hystrichokolpoma and Operculodinium have quasi-synchronous ranges. The subfamily has many entries in the Paleogene, but many of these events are not synchronous latitudinally.
-
Subfamily Gonyaulacoideae (Fig. 10)
Range. The subfamily of Gonyaulacoideae includes common modern cyst genera such as Spiniferites spp., Achomosphaera spp., Impagidinium spp., Nematosphaeropsis spp. and Tectatodinium spp. The subfamily first occurs in the Bajocian (∼ 170 Ma), with the FO of Gonyaulacysta spp. and Tubotuberella spp.
Quasi-synchronous events. Species of Achomosphaera, Ataxiodinium, Callaiosphaeridium, Corrudinium, Ectosphaeropsis Hystrichodinium, Impagidinium, Spiniferites and Unipontidinium have quasi-synchronous events (Fig. 10). Events of species of Escharisphaeridia spp., Gonyaulacysta spp. and Tubotuberella spp. range for slightly longer in Northern Hemisphere high latitudes than in mid-latitudes. Many species in this subfamily are strongly diachronous.
-
Subfamily Leptodinioideae (Fig. 11)
Range. Leptodinioideae first appear in the Aalenian (∼ 172 Ma, FO of Meiourogonyaulax valensii) and include many species events in the Bajocian and Bathonian. Although most entries are in the Jurassic and Early Cretaceous, the subfamily ranges into the late Miocene (∼ 8 Ma, LO of Acanthaulax miocenica).
Quasi-synchronous events. Events are in quasi-synchronous species of Ambonosphaera, Areosphaeridium (NH), Cooksonidium, Ctenidodinium, Dichadogonyaulax, Endoscrinium, Herendeenia, Kleithriasphaeridium, Leptodinium, Limbodinium, Litosphaeridium, Rigaudella aemula, Sirmiodiniopsis, Stiphrosphaeridium and Wanaea.
-
Family Gonyaulacaceae, other (Fig. 12)
Remarks. Other species in the family Gonyaulacaceae could not be assigned to a subfamily. Species of Barbatacysta, Chytroeisphaeridia, Glossodinium, Hemiplacophora, Nelchinopsis, Saturnodinium, Scriniodinium, Sepispinula, Stephodinium and Trichodinium spp. have remarkably consistent events.
-
Family Mancodiniaceae (Fig. 13)
Range. Species of Mancodiniaceae occur in sediments from the late Sinemurian (∼ 190 Ma) to the mid-Bathonian (∼ 167 Ma) and seem quasi-synchronous latitudinally.
-
Family Pareodiniaceae (Fig. 14)
Range. Pareodiniaceae first appear in the late Toarcian (∼ 176 Ma, FO of Pareodinia halosa) and range in NH mid-latitudes into the Cenomanian (∼ 95 Ma, LO of Batioladinium jaegeri). Events of species in Carpathodinium, Pareodinia (both NH only), Aprobolocysta and Batioladinium appear to be quasi-synchronous.
-
Family Scriniocassiaceae (Fig. 15)
Range. Scriniocassiaceae range from the Pliensbachian (∼ 187 Ma, FO of Scriniocassis weberi) to the Bajocian (∼ 169 Ma, LO of Scriniocassis weberi) and comprise only three species. Events from this family are only reported from the Northern Hemisphere.
-
Family Shublikodiniaceae (Fig. 16)
Range. Cysts from the family Shublikodiniaceae occur in the Late Triassic (FO of Rhaetogonyaulax wigginsii in the Carnian, ∼ 230 Ma) to Early Jurassic (LOs of Dapcodinium sacculus and Dapcodinium ovale in the mid-Pliensbachian, 187 Ma).
Quasi-synchronous events. The LO of Rhaetogonyaulax rhaetica close to the Triassic–Jurassic boundary and LO of Dapcodinium priscum are quasi-synchronous.
-
Family uncertain (Fig. 17)
Remarks. This group of which the family is uncertain contains several stratigraphically synchronous species (Fig. 17). Ranges of species of Amiculosphaera, Atopodinium, Batiacasphaera, Cleistosphaeridium, Dingodinium, Distatodinium, Heslertonia, Labyrinthodinium, Membranilarnacia, Mendicodinium, Oligokolpoma and Valensiella are quasi-synchronous.
-
Order uncertain
-
Family Comparodiniaceae (Fig. 18)
Range. Cysts from this family range from the late Sinemurian (191 Ma, FO of Valvaeodinium spp.) to the mid-Valanginian (134 Ma, LO of Biorbifera johnwingii). All species except Valvaeodinium spinosum and Biorbifera ferox have ranges restricted to the Northern Hemisphere.
Quasi-synchronous events. The range of Biorbifera johnwingii, FO of Valvaeodinium spinosum and LO of Valvaeodinium koessenium are quasi-synchronous.
-
Family Stephanelytraceae (Fig. 19)
Range. Stephanelytraceae cysts comprise one genus, which ranges from the Callovian (∼ 166 Ma) to the late Aptian (∼ 117 Ma) and seems quasi-synchronous.
-
Order Peridiniales
-
Family Heterocapsaceae (Fig. 20)
Range. Heterocapsaceae range from the mid-Sinemurian (195 Ma, FO of Liasidium variabile) to the mid-Albian (105 Ma, LO of Angustidinium acribes).
Quasi-synchronous events. Range of Liasidium variabile and Parvocysta bullula, restricted to NH mid-latitudes.
-
Family Peridiniaceae
-
Subfamily Deflandroideae (Fig. 21)
Range. Deflandroideae first occur in the Southern Hemisphere in the Oxfordian (∼ 161 Ma) with Pyxidiella spp. Isabelidinium and Eurydinium first appear in the Albian (∼ 109 Ma), and many species first appear in the Late Cretaceous (∼ 95–66 Ma). The subfamily became extinct with the LO of Sumatradinium spp. around 5 Ma and appears to range for the longest in low and middle latitudes. Deflandeoideae have many FO and LO entries in both hemispheres, particularly in the Late Cretaceous and early Paleogene.
Quasi-synchronous events. Several species of Cerodinium, Manumiella, Trithyrodinium and Isabelidinium have synchronous events in the Maastrichtian–Paleocene (70–60 Ma).
-
Subfamily Palaeoperidinioideae (Fig. 22)
Range. The Palaeoperidinioideae range from the mid-Valanginian (∼ 135 Ma, FO of Subtilisphaera perlucida) to the late Oligocene (∼ 26 Ma, LO of Phthanoperidinium comatum).
Quasi-synchronous events. The range of Palaeoperidinium pyrophorum and the LO of Phthanoperidinium comatum are quasi-synchronous.
-
Subfamily Wetzelielloideae (Fig. 23)
Range. Wetzelielloideae range from the mid-Paleocene (∼ 62 Ma, FO of Apectodinium homomorphum) to the late Oligocene (∼ 23 Ma, LO of Wetzeliella symmetrica). Diversification particularly in the Ypresian leads to many species with short stratigraphic ranges, many of which are relatively synchronous latitudinally. Several species appear to range for longer in the NH than on equal paleolatitudes in the SH. Many species lack chronostratigraphic ties in equatorial records.
-
Family Peridiniaceae, other (Fig. 24)
Remarks. There is one quasi-synchronous event in this remaining group: the FO of Ovoidinium cinctum at around 129 Ma.
-
Family Protoperidiniaceae (Fig. 25)
Range. Protoperidiniaceae first appear in the Santonian (FO of Phelodinium magnificum) and range into the modern era with 30 species in 13 genera, which is exceptionally diverse for modern cyst families. Species have the oldest first occurrences in low latitudes rather than in high latitudes. Events are extremely diachronous.
-
Order Nannoceratopsiales
-
Family Nannoceratopsiaceae (Fig. 26)
Range. Cysts from the family Nannoceratopsiaceae occur from the late Sinemurian (191 Ma, FO of Nannoceratopsis deflandrei subsp. senex) to the mid-Kimmeridgian (∼ 155 Ma, LO of Nannoceratopsis pellucida).
-
Order Ptychodiscales
-
Family Ptychodiscaceae (Fig. 27)
Range. This family only has entries in the Late Cretaceous (91–66 Ma), where species represent fairly synchronous stratigraphic markers. Although cysts are only found in a relatively short geologic time interval, motile cells of Ptychodiscaceae are known from modern plankton.
-
Order Suessiales
-
Family Suessiaceae (Fig. 28)
Range. Suessiaceae occur in the Triassic–Early Jurassic (229–182 Ma).
Quasi-synchronous events. The LO of Suessia swabiana. Other events are highly diachronous is quasi-synchronous.
4.1 Geographic extrapolation of dinocyst events
A suite of dinocyst events throughout the entire stratigraphic record have quasi-synchronous ages across all latitudes (Figs. 5–28). The uneven geographic spread of data, with voids in the equatorial region and the Pacific Ocean, makes global synchroneity of these events highly uncertain. Still, the synchronous events confirm the potential and value of dinocyst biostratigraphy to date complex sedimentary systems. They also imply that ocean connectivity did allow dinocyst species to migrate globally, as far as their environmental tolerances permitted.
Yet, the majority of dinocyst species have very diachronous ranges in DINOSTRAT, as well as latitudinally restricted geographic spreads, which confirms previous interpretations (Williams et al., 2004). By using DINOSTRAT the underlying causes of this diachroneity can now be further explored. The shortness of some of the records used in this review may lead to “false” events, i.e., those that represent re-appearance or temporal disappearance rather than “true” first or last occurrences (FOs and LOs, respectively). The obviously false FOs and LOs have been removed from DINOSTRAT by omitting events that occur at the base or the top of the sections. Particularly rare species or those occurring at the end of their preferred environmental niche come and go in stratigraphic sections, and these lead to false events in DINOSTRAT. Although such false FOs and LOs may obscure a uniform age of events over latitudes, they may still have important regional stratigraphic significance, which is why their entries are retained in DINOSTRAT. As a result, the age and region of the oldest FOs and youngest LOs have the most significance for the reconstruction of evolutionary patterns. Although caving of material typically falsely increases the age of the oldest FOs, this is unlikely to have a large influence on the entries in DINOSTRAT, as most studies come from core or outcrop material, and not from ditch cuttings, for which caving is much more likely. Reworking could falsely extend the age of the youngest LOs of species. Although species that were reported as reworked in the papers have been omitted from DINOSTRAT, some reworked dinocysts could have been falsely identified as in situ in the original papers. It cannot be excluded that this causes some level of diachroneity in LOs, although this is unlikely a large factor.
The complexity of taxonomic concepts in some dinocyst genera (species definitions or morphological continua) hinders proper evaluation of latitudinal synchroneity of events. The reviewed literature covers 50 years, during which taxonomic concepts of dinocysts species have iteratively evolved. The extensive synonymy database of Williams et al. (2022) does deliver crucial organization of the taxonomic framework. Still, some of the subtle morphological differences in species are limited to the expert eye of individual researchers, and these may not have been recognized by others (which occasionally has led to the presentation of taxa on a generic level, instead of further specification to species level). Making the taxonomic framework consistent for all studies now included in DINOSTRAT would be a cardinal effort and will be part of the iterative setup of DINOSTRAT. For example, reviews of dinocyst taxonomic frameworks on a per-family basis, such as has been initiated for the Spiniferites complex (e.g., Mertens and Carbonell-Moore, 2018), could help in adjusting inconsistencies in species concepts and their stratigraphic occurrence. In any case, it must be stressed that the quality of any biostratigraphic marker is defined not only by the accuracy of the tie to the chronostratigraphic timescale or global consistency of the age of FO or LOs but also by their morphological distinctiveness.
Events may also appear diachronous in DINOSTRAT because of inadequate or inaccurate ties to the chronostratigraphic timescale. In such cases, minor diachroniety (∼ 104–5 years) may be related to the inherent assumption of linear sedimentation rates between age tie points. Larger diachroneity (∼ 105–6 years) may be because the zonation through which dinocyst events were calibrated to the chronostratigraphic timescale is diachronous. For calibrations against magnetostratigraphy (tiers 1 and 2) this is unlikely and could occur only when magnetochrons were wrongly interpreted in the sites used. For events calibrated against Cenozoic nannoplankton and foraminifer zonations (in tiers 3 and 4) this is also unlikely, as these events are relatively robustly calibrated to chronostratigraphy (Watkins and Raffi, 2020; Petrizzo et al., 2012). Less robust are the Mesozoic ammonite zonation schemes, which have been shown to be quite latitudinally diachronous themselves (e.g., Ogg and Hinnov, 2012a, b, and references therein). The geographic variability in the ages of zone boundaries and also numerous adjustments of zone definitions throughout the past 50 years further complicate accurate tying of dinocyst events with ammonite data to GTS2012. So far, the majority of Mesozoic dinocyst events have been calibrated against these ammonite zonations, which makes their absolute tie to the chronostratigraphic timescale most uncertain. A major challenge for future versions of DINOSTRAT is to improve the independent age control of calibrated Mesozoic dinocyst events.
Also, ecological reasons could cause geographically diachronous events. When local environmental or depositional conditions change, assemblages adjust, which leads to local and temporal (dis)appearances of species that may be falsely interpreted as extinction or origination events. If so, dinocyst taxa associated with the most dynamic environmental niches on the continental shelf are expected to have the most diachronous events. Indeed, there are particularly diachronous events in Goniodomaceae and Protoperidinioideae – both families are associated with nearshore depositional settings (Zonneveld et al., 2013; Sluijs et al., 2005; Frieling and Sluijs, 2018) that are the most environmentally dynamic. Settings in which these species occur offshore, such as in upwelling regions (Sangiorgi et al., 2018) or hyperstratified waters (Reichart et al., 2004; Cramwinckel et al., 2019), are environmentally equally dynamic. In contrast, families typically associated with offshore conditions, such as the Wetzeliellioideae (Frieling and Sluijs, 2018), reveal much more synchronous events. For regional stratigraphy, the diachroneity is of less concern because these events can still be used for regional stratigraphic correlation (e.g., as in Vieira and Jolley, 2020). It does mean that for such species, dinocyst biostratigraphy applies regionally, and caution should be taken to extrapolate event ages far outside of these regions. There are also species that clearly show regional inconsistency in origination or extinction ages because of climate change – e.g., Melitasphaeridium choanophorum had a much wider geographic distribution during warmer past climates and a progressively younger LO in lower latitudes as climate cooled (Fig. 4).
Diachroneity is usually larger between latitudinal bands than within latitudinal bands. The sparsity of records from the SH high latitudes complicates robust assessment of interhemispheric differences in dinocyst event ages. In the Mesozoic, the diachroneity is likely related to the inadequate calibration of events to the international timescale. DINOSTRAT is short of Mesozoic records that are tied to stratigraphic tools other than ammonites. For the Cenozoic, the diachroneity between hemispheres cannot be explained by inadequate calibration since many events are calibrated against magnetostratigraphy. For those, environmental reasons must be at play. While in the early Paleogene many dinocyst events are quasi-synchronous (events within the Wetzeliellioideae, of Cerodinium and Palaeoperidinium), in the late Paleogene and Neogene diachroneity seems to become stronger. This may be in part because of stronger latitudinal temperature gradients as the global average climate cools (Cramwinckel et al., 2018; Westerhold et al., 2020), which creates more diverse ecological niches and complicates latitudinal migration.
Many dinocyst species and higher generic ranks have their oldest first occurrence and youngest last occurrence in NH mid-latitudes (for example Areoligeraceae, Cladopyxiaceae, Comparodiniaceae, Goniodomaceae, Nannoceratopsiaceae, Palaeoperidinioideae, Wetzeliellioideae; Figs. 5, 7, 18, 8, 26, 22, 23). This may be because of a much higher density of records at those latitudes. However, the vast continental shelf area in Europe throughout the Mesozoic and much of the Cenozoic did likely serve as the perfect habitat for taxa to find a new niche and to linger on. A higher record density in SH and equatorial regions should shed light on this idea.
4.2 Evolutionary patterns in dinocyst (sub)families
DINOSTRAT presents for the first time a quantitative overview of the stratigraphic and paleolatitudinal distribution of fossil and modern dinocyst taxa. Through that, it refines with coherent, independent, open-access data the evolutionary patterns presented previously (e.g., Fensome et al., 1993; MacRae et al., 1996) and adds their latitudinal distribution through time. Following up on 60 million years of experimentation in cyst formation among a wide group of dinoflagellates (Figs. 13, 15, 16, 18–20, 26, 28), gonyaulacoid dinocysts developed their most fundamental taxonomic features in a rapid diversity phase in the Bajocian (∼ 169 Ma), likely on vast continental shelf areas on the European continent (Figs. 5, 9–12, 17). The extremely high diversity in gonyaulacoid dinocysts in the Late Jurassic and Cretaceous is reflected in the density of the events in DINOSTRAT. Peridinioid dinocyst taxa strongly diversified in the Late Cretaceous and Paleogene (Figs. 21–25). The decline in dinocyst diversity in the Neogene is visible in the scarcity of FOs from 25 Ma onwards (except in Protoperidinioideae). DINOSTRAT allows the further exploration of spatial patterns in dinocyst evolution in the future.
4.3 Functionality of DINOSTRAT
Once downloaded, DINOSTRAT can be filtered by location, allowing users to compare newly generated dinocyst chronologies to calibrated regional dinocyst events nearby. DINOSTRAT can also be filtered by species, genus or higher taxonomic rank for further evaluation of the latitudinal spread of any species of interest. The data in DINOSTRAT are readily visualized in Supplement File 2, and these plots can be adjusted and reproduced using the R markdown file plot creator.Rmd in Bijl (2021). The community is invited to contact the author either via email or through GitHub, with suggestions, error reports, and/or additional papers or data to be entered so that the data content of DINOSTRAT is iteratively improved.
4.4 Future directions
DINOSTRAT will be regularly updated. Annual minor updates include the addition of sites, adjustments in the current entries (e.g., through the feedback process) or minor revisions in taxonomy/stratigraphy. Major updates will occur in a 3-year cycle and will be the result of updates to the geologic time scale or profound revisions in dinocyst taxonomic concepts. Major updates will be accompanied by a short communication in this journal; minor updates will be communicated through the GitHub repository. Updates of the geologic time scale (e.g., to GTS2020; Gradstein et al., 2020) will be implemented once the metadata of that geologic time scale have become available. All versions of DINOSTRAT will remain archived on GitHub.
The database is available under a CC BY 4.0 license on GitHub (Bijl, 2021; https://github.com/bijlpeter83/DINOSTRAT.git, last access: 1 February 2022; DOI https://doi.org/10.5281/zenodo.5772616). The database consists of four .csv files: (1) Paleolatitude.csv – paleolatitude and present-day position of sites in DINOSTRAT, (2) modernst.csv – the site locations of core top sediments, (3) modernsp.csv – a modified modern dinocyst dataset, and (4) Dinoevents_Jan2021.csv – the calibrated dinocyst events. plot creator.Rmd is an R markdown file to reproduce the figures presented in this paper.
This paper presents the database DINOSTRAT version 1.0 (Bijl, 2021), a database containing >8500 entries of regional dinocyst first and last occurrences (events) from over 1900 species, in 188 sites. The geographic distribution of sites used in DINOSTRAT is strongly concentrated in the Northern Hemisphere mid-latitudes, notably in Europe and the North Atlantic, and few sites are in the Pacific or Southern Hemisphere. Ages of events were calibrated using their ties to the geologic time scale. The paper presents the location and age of the origin of modern dinocyst species, genera, subfamilies and families. It reviews the age range and geographic spread of modern and extinct dinocyst taxa and highlights the most latitudinally synchronous dinocyst events.
Many dinocyst taxa show quasi-synchronous events latitudinally, which can be widely used to stratigraphically date complex sedimentary sequences. Latitudinal diachroneity in events can be the result of inadequate calibration to the chronostratigraphic timescale, false interpretations of true events, complicated species concepts or paleoceanographic reasons. In any case, this dictates caution when extrapolating ages of dinocyst events to far distances and demonstrates the need for regionally calibrated dinocyst zonations, which DINOSTRAT here provides. It further provides a solid foundation to review spatio-temporal patterns in dinocyst evolution, dispersal and extinction. DINOSTRAT is freely available under a CC BY 4.0 license. It allows the user to filter by region or by species, genus or higher taxonomic rank.
Supplement File 1 is a table of conversions of published zones to those in GTS2012. Supplement File 2 is a zip file containing ages and latitudes of events in individual dinocyst species (1914 plots), grouped by genus (459 plots) and by family (27 plots), including modern cyst species (92 plots) and the range charts for all sites (188 plots). The supplement related to this article is available online at: https://doi.org/10.5194/essd-14-579-2022-supplement.
The contact author has declared that there are no competing interests.
Publisher's note: Copernicus Publications remains neutral with regard to jurisdictional claims in published maps and institutional affiliations.
I thank Henk Brinkhuis, Bas vd Schootbrugge, Francesca Sangiorgi and Appy Sluijs for useful discussions. The “Advanced course in organic-walled dinoflagellate cyst taxonomy, stratigraphy and paleoecology” has been a great “playground” to discuss progress in the field, and for that I have Martin Head, Martin Pearce, Jörg Pross, Jim Riding and Poul Schiøler to thank. I acknowledge the then research assistants who helped in building predecessors of DINOSTRAT: Tjerk Veenstra, Keechy Akkerman and Caroline van der Weijst. Thanks to Martin Schobben and Ilja Kocken for help with the data analysis and visualization in R and to Douwe van Hinsbergen for help reconstructing the paleolatitudes of the sites. James Ogg is thanked for providing the data from GTS2012. The constructive and detailed comments from Henrik Nøhr-Hansen and Ian Harding greatly improved the final paper.
The LPP Foundation has financially supported the development of DINOSTRAT.
This paper was edited by Thomas Blunier and reviewed by Henrik Nøhr-Hansen and Ian Harding.
Açıkalın, S., Vellekoop, J., Ocakoğlu, F., Yılmaz, İ. Ö., Smit, J., Altıner, S. Ö., Goderis, S., Vonhof, H., Speijer, R. P., Woelders, L., Fornaciari, E., and Brinkhuis, H.: Geochemical and palaeontological characterization of a new K-Pg Boundary locality from the Northern branch of the Neo-Tethys: Mudurnu – Göynük Basin, NW Turkey, Cretaceous Res., 52, 251–267, 2015.
Århus, N., Birkelund, T., and Smelror, M.: Biostratigraphy of some Callovian and Oxfordian cores off Vega, Helgeland, Norsk Geol. Tidsskr., 69, 39–56, 1989.
Aubry, A. M. R., De Schepper, S., and de Vernal, A.: Dinocyst and acritarch biostratigraphy of the Late Pliocene to Early Pleistocene at Integrated Ocean Drilling Program Site U1307 in the Labrador Sea, J. Micropalaeontol., 39, 41–60, https://doi.org/10.5194/jm-39-41-2020, 2020.
Awad, W. K. and Oboh-Ikuenobe, F. E.: Early Paleogene dinoflagellate cysts from ODP Hole 959D, Côte d'Ivoire-Ghana Transform Margin, West Africa: New species, biostratigraphy and paleoenvironmental implications, J. Afr. Earth Sci., 123, 123–144, https://doi.org/10.1016/j.jafrearsci.2016.07.014, 2016.
Awad, W. K. and Oboh-Ikuenobe, F. E.: Paleogene-early Neogene paleoenvironmental reconstruction based on palynological analysis of ODP Hole 959A, West Africa, Mar. Micropaleontol., 148, 29–45, 2019.
Bailey, D. A., Milner, P., and Varney, T.: Some dinoflagellate cysts from the Kimmeridge Clay Formation in North Yorkshire and Dorset, U.K., P. Yorks. Geol. Soc., 51, 235–243, 1997.
Baruffini, L., Lottaroli, F., and Torricelli, S.: Integrated high-resolution stratigraphy of the lower oligocene tusa tuffite formation in the Calabro-Lucano area and sicily (southern Italy), Riv. Ital. Paleontol. S., 108, 457–478, 2002.
Besems, R.: Dinoflagellate cyst biostratigraphy of Tertiary and Quaternary deposits of offshore NW Borneo, Geological Society of Malaysia, Bulletin of the Royal Society, 33, 65–93, 1993.
Biffi, U. and Manum, S. B.: Late Eocene-Early Miocene dinoflagellate cyst stratigraphy from the Marche region (Central Italy), Bulletino della Società Paleontologica Italiana, 27, 163–212, 1988.
Bijl, P. K.: DINOSTRAT V1.1, Zenodo [data set], https://doi.org/10.5281/zenodo.5772616, 2021.
Bijl, P. K. and Brinkhuis, H.: A new genus and two new species of dinoflagellate cysts from lower Eocene marine sediments of the Wilkes Land Margin, Antarctica, Rev. Palaeobot. Palyno., 220, 88–97, https://doi.org/10.1016/j.revpalbo.2015.05.004, 2015.
Bijl, P. K., Sluijs, A., and Brinkhuis, H.: A magneto- chemo- stratigraphically calibrated dinoflagellate cyst zonation of the early Paleogene South Pacific Ocean, Earth-Sci. Rev., 124, 1–31, https://doi.org/10.1016/j.earscirev.2013.04.010, 2013a.
Bijl, P. K., Bendle, A. P. J., Bohaty, S. M., Pross, J., Schouten, S., Tauxe, L., Stickley, C. E., McKay, R. M., Röhl, U., Olney, M., Sluijs, A., Escutia, C., Brinkhuis, H., and Expedition 318 scientists: Eocene cooling linked to early flow across the Tasmanian Gateway, P. Natl. Acad. Sci. USA, 110, 9645–9650, https://doi.org/10.1073/pnas.1220872110, 2013b.
Bijl, P. K., Sluijs, A., and Brinkhuis, H.: Erratum to “A magneto- and chemostratigraphically calibrated dinoflagellate cyst zonation of the early Paleogene South Pacific Ocean” [Earth Sci. Rev. 124 (2013) 1–31], Earth-Sci. Rev., 134, 160–163, https://doi.org/10.1016/j.earscirev.2014.03.010, 2014.
Bijl, P. K., Brinkhuis, H., Egger, L. M., Eldrett, J. S., Frieling, J., Grothe, A., Houben, A. J. P., Pross, J., Sliwinska, K. K., and Sluijs, A.: Comment on “Wetzeliella and its allies – the “hole” story: a taxonomic revision of the Paleogene dinoflagellate subfamily Wetzelielloideae” by Williams et al. (2015), Palynology, 41, 423–429, https://doi.org/10.1080/01916122.2016.1235056, 2016.
Bijl, P. K., Houben, A. J. P., Bruls, A., Pross, J., and Sangiorgi, F.: Stratigraphic calibration of Oligocene–Miocene organic-walled dinoflagellate cysts from offshore Wilkes Land, East Antarctica, and a zonation proposal, J. Micropalaeontol., 37, 105–138, https://doi.org/10.5194/jm-37-105-2018, 2018.
Bowman, V. C., Francis, J. E., Riding, J. B., Hunter, S. J., and Haywood, A. M.: A latest Cretaceous to earliest Paleogene dinoflagellate cyst zonation from Antarctica, and implications for phytoprovincialism in the high southern latitudes, Rev. Palaeobot. Palyno., 171, 40–56, https://doi.org/10.1016/j.revpalbo.2011.11.004, 2012.
Bowman, V., Ineson, J., Riding, J., Crame, J., Francis, J., Condon, D., Whittle, R., and Ferraccioli, F.: The Paleocene of Antarctica: Dinoflagellate cyst biostratigraphy, chronostratigraphy and implications for the palaeo-Pacific margin of Gondwana, Gondwana Res., 38, 132–148, https://doi.org/10.1016/j.gr.2015.10.018, 2016.
Bravo, I. and Figueroa, R. I.: Towards an Ecological Understanding of Dinoflagellate Cyst Functions, Microorganisms, 2, 11–32, https://doi.org/10.3390/microorganisms2010011, 2014.
Brinkhuis, H.: Late Eocene to Early Oligocene dinoflagellate cysts from the Priabonian type-area (northeast Italy); biostratigraphy and palaeoenvironmental interpretation, Palaeogeogr. Palaeocl., 107, 121–163, 1994.
Brinkhuis, H. and Biffi, U.: Dinoflagellate cyst stratigraphy of the Eocene/Oligocene transition in Central Italy, Mar. Micropaleontol., 22, 131–183, 1993.
Brinkhuis, H., Powell, A. J., and Zevenboom, D.: High-resolution dinoflagellate cyst stratigraphy of the Oligocene/Miocene transition interval in northwest and central Italy, in: Neogene and Quaternary Dinoflagellate Cysts and Acritarchs, edited by: Head, M. J., and Wrenn, J. H., American Association of Stratigraphic Palynologists Foundation, Dallas, 1992.
Brinkhuis, H., Bujak, J. P., Smit, J., Versteegh, G. J. M., and Visscher, H.: Dinoflagellate-based sea surface temperature reconstructions across the Cretaceous-Tertiary boundary, Palaeogeogr. Palaeocl., 141, 67–83, 1998.
Brinkhuis, H., Munsterman, D. M., Sengers, S., Sluijs, A., Warnaar, J., and Williams, G. L.: Late Eocene to Quaternary dinoflagellate cysts from ODP Site 1168, off western Tasmania, in: Proceedings of the ODP, Scientific results, Leg 189, edited by: Exon, N., and Kennett, J. P., U.S. Government Printing Office, College Station, Texas, https://doi.org/10.2973/odp.proc.sr.189.105.2003, 2003a.
Brinkhuis, H., Sengers, S., Sluijs, A., Warnaar, J., and Williams, G. L.: Latest Cretaceous to earliest Oligocene, and Quaternary dinoflagellates from ODP Site 1172, East Tasman Plateau, in: Proceedings of the ODP, Scientific results, Leg 189, edited by: Exon, N., and Kennett, J. P., U.S. Government Printing Office, College Station, Texas, https://doi.org/10.2973/odp.proc.sr.189.106.2003, 2003b.
Brown, S. and Downie, C.: Dinoflagellate cyst biostratigraphy of late Paleocene and early Eocene sediments from Holes 552, 553A, and 555, Leg 81, Deep Sea Drilling Project (Rockall Plateau), in: Proceedings of the deep sea drilling project, Initial reports, vol. 81, Washington, U.S.A., 565–579, https://doi.org/10.2973/dsdp.proc.81.113.1984, 1984.
Brown, S. and Downie, C.: Dinoflagellate cyst stratigraphy of Paleocene to Miocene sediments from the Goban Spur (Sites 548–550, Leg 80), in: Proceedings of the Deep Sea Drilling Project, Initial reports, vol. 80, Washington, U.S.A., 643–651, https://doi.org/10.2973/dsdp.proc.80.120.1985, 1985.
Bucefalo Palliani, R. and Riding, J. B.: Lower Toarcian palynostratigraphy of Pozzale, central Italy, Palynology, 21, 91–103, 1997a.
Bucefalo Palliani, R. and Riding, J. B.: The influence of palaeoenvironmental change on dinoflagellate cyst distribution. An example from the Lower and Middle Jurassic of Quercy, southwest France, Bull. Cent. Rech. Elf E., 21, 107–123, 1997b.
Bucefalo Palliani, R. and Riding, J. B.: A palynological investigation of the Lower and lowermost Middle Jurassic strata (Sinemurian to Aalenian) from North Yorkshire, UK, Proceedings of the Yorkshire Geological Society, 53, 1–16, 2000.
Bucefalo Palliani, R. and Riding, J. B.: Biostratigraphy, Provincialism and evolution of European Early Jurassic (Pliensbachian to early Toarcian) dinoflagellate cysts, Palynology, 27, 179–214, 2003.
Bujak, J. P. and Matsuoka, K.: Late Cenozoic dinoflagellate cyst zonation in the western and Northern Pacific, Palynology, 17, 7–25, 1986.
Bujak, J. P. and Mudge, D. C.: A high-resolution North Sea Eocene dinocyst zonation, Journal of the Geological Society London, 151, 449–462, 1994.
Clyde, W. C., Wilf, P., Iglesias, A., Slingerland, R. L., Barnum, T., Bijl, P. K., Bralower, T. J., Brinkhuis, H., Comer, E. E., Huber, B. T., Ibañez-Mejia, M., Jicha, B. R., Krause, J. M., Schueth, J. D., Singer, B. S., Raigemborn, M. S., Schmitz, M. D., Sluijs, A., and Zamaloa, M. C.: New age constraints for the Salamanca Formation and lower Río Chico Group in the western San Jorge Basin, Patagonia, Argentina: Implications for cretaceous-paleogene extinction recovery and land mammal age correlations, GSA Bulletin, 126, 289–306, https://doi.org/10.1130/B30915.1, 2014.
Correia, V. F., Riding, J. B., Henriques, M. H., Fernandes, P., Pereira, Z., and Wiggan, N. J.: The middle Jurassic palynostratigraphy of the northern Lusitanian Basin, Portugal, Newsl. Stratigr., 52, 73–79, https://doi.org/10.1127/nos/2018/0471, 2019.
Costa, L. I. and Davey, R. J.: Dinoflagellate cysts of the Cretaceous system, in: A stratigraphix index of dinoflagellate cysts, in: A stratigraphix index of dinoflagellate cysts, edited by: Powell, A. J., British Micropaleontological Society Publications Series, London, UK, 99–154, ISBN-13 978-9401050524, 1992.
Costa, L. I. and Downie, C.: The Wetzeliellaceae; Palaeogene dinoflagellates, in: Proceedings of the 4th International Palynological Conference, Lucknow, 1976, 34–46, 1979.
Cramwinckel, M. J., Huber, M., Kocken, I. J., Agnini, C., Bijl, P. K., Bohaty, S. M., Frieling, J., Goldner, A., Hilgen, F. J., Kip, E. L., Peterse, F., van der Ploeg, R., Röhl, U., Schouten, S., and Sluijs, A.: Synchronous tropical and deep ocean temperature evolution in the Eocene, Nature, 559, 382–386, 2018.
Cramwinckel, M. J., van der Ploeg, R., Bijl, P. K., Peterse, F., Bohaty, S. M., Röhl, U., Schouten, S., Middelburg, J. J., and Sluijs, A.: Harmful algae and export production collapse in the equatorial Atlantic during the zenith of Middle Eocene Climatic Optimum warmth, Geology, 47, 247–250, https://doi.org/10.1130/G45614.1, 2019.
Crouch, E. M., Willumsen, P. S., Kulhanek, D. K., and Gibbs, S.: A revised Paleocene (Teurian) dinoflagellate cyst zonation from eastern New Zealand, Palaeogeogr. Palaeocl., 202, 47–79, 2014.
Crouch, E. M., Shepherd, C. L., Morgans, H. E. G., Naafs, B. D. A., Dallanave, E., Phillips, A., Hollis, C. J., and Pancost, R. D.: Climatic and environmental changes across the early Eocene climatic optimum at mid-Waipara River, Canterbury Basin, New Zealand, Earth-Sci. Rev., 200, 102961, https://doi.org/10.1016/j.earscirev.2019.102961, 2020.
Dallanave, E., Bachtadse, V., Crouch, E. M., Tauxe, L., Shepherd, C. L., Morgans, H. E. G., Hollis, C. J., Hines, B. R., and Sugisaki, S.: Constraining early to middle Eocene climate evolution of the southwest Pacific and Southern Ocean, Earth Planet. Sc. Lett., 433, 380–392, https://doi.org/10.1016/j.epsl.2015.11.010, 2016.
Davey, R. J.: Marine Apto-Albian palynomorphs from Holes 400A and 402A, IPOD Leg 48, northern Bay of Biscay, in: Init. Rep. DSDP, vol. 48, edited by: Montardert, L., Roberts, D. G., and Thompson, R. W., DSDP, Washington, USA, https://doi.org/10.2973/dsdp.proc.48.123.1979, 1979.
Davey, R. J.: Dinocyst stratigraphy of the latest Jurassic to Early Cretaceous of the Haldager No. 1 borehole, Denmark, Danmarks Geologiske Undersögelse, Series B, 6, 1–57, 1982.
Davey, R. J.: A summary of the palynology of the lower Hauterivian (Lower Cretaceous) from Speeton, east England, Neues Jahrbuch für Paläontologische Abhandlungen, 122, 83–93, 2001.
Davey, R. J. and Verdier, J.-P.: An investigation of microplankton assemblages from the Albian of the Paris Basin, Verh., Ned. Akad. Wet., Afd. Natuurkd., Eerste Reeks, 26, 1–58, 1971.
De Lira Mota, M. A., Harrington, G., and Dunkley Jones, T.: Organic-walled dinoflagellate cyst biostratigraphy of the upper Eocene to lower Oligocene Yazoo Formation, US Gulf Coast, J. Micropalaeontol., 39, 1–26, https://doi.org/10.5194/jm-39-1-2020, 2020.
De Schepper, S. and Head, M. J.: Age calibration of dinoflagellate cyst and acritarch events in the Pliocene-Pleistocene of the eastern North Atlantic (DSDP Hole 610A), Stratigraphy, 5, 137–161, 2008.
De Schepper, S. and Head, M. J.: Pliocene and pleistocene dinoflagellate cyst and acritarch zonation of DSDP Hole 610A, Eastern North Atlantic, Palynology, 33, 179–218, 2009.
De Schepper, S., Beck, K. M., and Mangerud, G.: Late Neogene dinoflagellate cyst and acritarch biostratigraphy for Ocean Drilling Program Hole 642B, Norwegian Sea, Rev. Palaeobot. Palyno., 236, 12–32, https://doi.org/10.1016/j.revpalbo.2016.08.005, 2017.
De Vernal, A. and Mudie, P. J.: Late Pliocene to Holocene palynostratigraphy at ODP Site 645, Baffin Bay, in: Proceedings of the ODP, Scientific Results, Leg 105, edited by: Srivastava, S. P., Arthur, M., and Clement, B., College Station, Texas, USA, https://doi.org/10.2973/odp.proc.sr.105.133.1989, 1989.
De Vernal, A., Londeix, L., Mudie, P. J., Harland, R., Morzadec-Kerfourn, M. T., Turon, J.-L., and Wrenn, J. H.: Quaternary organic-walled dinoflagellate cysts of the North Atlantic Ocean and adjacent seas: ecostratigraphy and biostratigraphy, in: Neogene and Quaternary dinoflagellate cysts and acritarchs, edited by: Head, M. J. and Wrenn, J. H., AASP Foundation, 289–329, 1992.
De Verteuil, L. and Norris, G.: Miocene dinoflagellate stratigraphy and systematics of Maryland and Virginia, Micropaleontology, 42, 1–172, https://doi.org/10.2307/1485926, 1996.
Dimter, A. and Smelror, M.: Callovian (Middle Jurassic) marine microplankton from southwestern Germany: Biostratigraphy and paleoenvironmental interpretations, Palaeogeogr. Palaeocl., 80, 173–195, https://doi.org/10.1016/0031-0182(90)90131-P, 1990.
Dodsworth, P.: Trans-Atlantic dinoflagellate cyst stratigraphy across the Cenomanian–Turonian (Cretaceous) Stage boundary, J. Micropalaeontol., 19, 69–84, https://doi.org/10.1144/jm.19.1.69, 2000.
Duffield, S. L. and Stein, J. A.: Peridiniacean-dominated cyst assemblage from the Miocene of the Gulf of Mexico shelf, offshore Louisiana, American Association of Stratigraphic Palynologists Contribution Series, 17, 27–45, 1986.
Duque-Herrera, A.-F., Helenes, J., Pardo-Trujillo, A., Flores-Villarejo, J.-A., and Sierro-Sánchez, F.-J.: Miocene biostratigraphy and paleoecology from dinoflagellates, benthic foraminifera and calcareous nannofossils on the Colombian Pacific coast, Mar. Micropaleontol., 141, 42–54, https://doi.org/10.1016/j.marmicro.2018.05.002, 2018.
Duxbury, S.: A study of dinoflagellate cysts and acritarchs from the Lower Green- sand (Aptian to Lower Albian) of the Isle of Wight, southern England, Palaeontographica, Abt. B, 186, 18–80, 1983.
Duxbury, S.: A palynological zonation scheme for the Lower Cretaceous – United Kingdom Sector, Central North Sea, Neues Jahrb. Geol. P-A., 219, 95–137, 2001.
Dybkjær, K. and Piasecki, S.: A new Neogene biostratigraphy for Denmark, Geol. Surv. Den. Greenl., 15, 1–29, https://doi.org/10.34194/geusb.v15.5036 , 2008.
Dybkjær, K. and Piasecki, S.: Neogene dinocyst zonation for the eastern North Sea Basin, Denmark, Rev. Palaeobot. Palyno., 161, 1–29, https://doi.org/10.1016/j.revpalbo.2010.02.005, 2010.
Egger, L. M., Sliwinska, K. K., van Peer, T. E., Liebrand, D., Lippert, P. C., Friedrich, O., Wilson, P. A., Norris, R. D., and Pross, J.: Magnetostratigraphically-calibrated dinoflagellate cyst bioevents for the uppermost Eocene to lowermost Miocene of the western North Atlantic (IODP Expedition 342, Paleogene Newfoundland sediment drifts), Rev. Palaeobot. Palyno., 234, 159–185, https://doi.org/10.1016/j.revpalbo.2016.08.002, 2016.
Eldrett, J. S. and Harding, I. C.: Palynological analyses of Eocene to Oligocene sediments from DSDP Site 338, Outer Vøring Plateau, Mar. Micropaleontol., 73, 226–240, 2009.
Eldrett, J. S., Harding, I. C., Firth, J. V., and Roberts, A. P.: Magnetostratigraphic calibration of Eocene-Oligocene dinoflagellate cyst biostratigraphy from the Norwegian-Greenland Sea, Mar. Geol., 204, 91–127, 2004.
Eldrett, J. S., Harding, I. C., Wilshaw, R., and Xuan, C.: A new high northern latitude dinocyst-based magneto-biostratigraphic calibration for the Norwegian-Greenland Sea, Newsl. Stratigr., 52, 435–460, 2019.
Ellegaard, M.: Variations in dinoflagellate cyst morphology under conditions of changing salinity during the last 2000 years in the Limfjord, Denmark, Rev. Palaeobot. Palyno., 109, 65–81, 2000.
Eshet, Y., Moshkovitz, S., Habib, D., Benjamini, C., and Magaritz, M.: Calcareous nannofossil and dinoflagellate stratigraphy across the Cretaceous/Tertiary boundary at Hor Hahar, Israel, Mar. Micropaleontol., 18, 199–228, https://doi.org/10.1016/0377-8398(92)90013-A, 1992.
Feist-Burkhardt, S.: Dinoflagellate assemblages of the Hausen coreholes (Aalenian to Early Bajocian), southwest Germany, Bull. Cent. Rech. Elf E., 14, 611–633, 1990.
Feist-Burkhardt, S. and Monteil, E.: Dinoflagellate cysts from the Bajocian stratotype (Calvados, Normandy, western France). Kystes de dinoflagellés du stratotype du Bajocien (Calvados, Normandie, France), Bull. Cent. Rech. Elf E., 21, 31–105, 1997.
Fensome, R. A., Taylor, F. J. R., Norris, G., Sarjeant, W. A. S., Wharton, D. I., and Williams, G. L.: A Classification of Modern and Fossil Dinoflagellates, edited by: Dinkins, G., Micropalaeontology, Special Paper, Salem, No. 7, 1993.
Fensome, R. A., Crux, J. A., Gard, G., MacRae, R. A., Williams, G. L., Thomas, F. C., Fiorini, F., and Wach, G.: The last 100 million years on the Scotian Margin, offshore eastern Canada: an event-stratigraphic scheme emphasizing biostratigraphic data, Atl. Geol., 44, 93–126, 2008.
Firth, J. V.: Upper middle Eocene to Oligocene dinoflagellate biostratigraphy and assemblage variations in Hole 913B, Greenland Sea, in: Proceedings of the Ocean Drilling Program. Scientific Results, vol. 151, edited by: Thiede, J., Myrhe, A. M., Firth, J. V., Johnson, G. L., and Ruddiman, W. F., 203–242, https://doi.org/10.2973/odp.proc.sr.151.105.1996, 1996.
Firth, J. V., Eldrett, J. S., Harding, I. C., Coxall, H. K., and Wade, B. S.: Integrated biomagnetochronology for the palaeogene of ODP Hole 647A: Implications for correlating palaeoceanographic events from high to low latitudes, Geol. Soc. Sp., 373, 29–78, https://doi.org/10.1144/SP373.9, 2013.
Frieling, J. and Sluijs, A.: Towards quantitative environmental reconstructions from ancient non-analogue microfossil assemblages: Ecological preferences of Paleocene – Eocene dinoflagellates, Earth-Sci. Rev., 185, 956–973, https://doi.org/10.1016/j.earscirev.2018.08.014, 2018.
Frieling, J., Iakovleva, A. I., Reichart, G. J., Aleksandrova, G. N., Gnibidenko, Z. N., Schouten, S., and Sluijs, A.: Paleocene–Eocene warming and biotic response in the epicontinental West Siberian Sea, Geology, 42, 767–770, 2014.
Gradstein, F. M., Kristiansen, I. L., Loemo, L., and Kaminski, M. A.: Cenozoic foraminiferal and dinoflagellate cyst biostrtigraphy of the central North Sea, Micropaleontology, 38, 101–137, 1992.
Gradstein, F. M., Ogg, J. G., Schmitz, M. D., and Ogg, G. M.: The Geologic Time Scale 2012, Elsevier, Amsterdam, 1–1144, ISBN 9780444594488, 2012.
Gradstein, F. M., Ogg, J. G., Schmitz, M. D., and Ogg, G. M.: The Geologic Time Scale 2020, Elsevier, Amsterdam, 1–1358, ISBN 9780128243619, 2020.
Grothe, A., Sangiorgi, F., Brinkhuis, H., Stoica, M., and Krijgsman, W.: Migration of the dinoflagellate Galeacysta etrusca and its implications for the Messinian Salinity Crisis, Newsl. Stratigr., 51, 73–91, https://doi.org/10.1127/nos/2016/0340, 2017.
Guasti, E., Speijer, R. P., Brinkhuis, H., Smit, J., and Steurbaut, E.: Paleoenvironmental change at the Danian-Selandian transition in Tunisia: Foraminifera, organic-walled dinoflagellate cyst and calcareous nannofossil records, Mar. Micropaleontol., 59, 210–229, 2005.
Habib, D. and Drugg, W. S.: Dinoflagellate age of Middle Jurassic–Early Cretaceous sediments in the Blake-Bahama Basin, in: Deep Sea Drilling Project, vol. 76, edited by: Gradstein, F. M., Sheridan, R. E. et al., U.S. Govt. Printing Office, Washington, https://doi.org/10.2973/dsdp.proc.76.126.1983, 1983.
Habib, D. and Drugg, W. S.: Palynology of Sites 603 and 605, Leg 93, Deep Sea Drilling Project, in: Deep Sea Drilling Project, edited by: van Hinte, J. E. et al., Washington, Initial Reports, vol. 93, 751–775, https://doi.org/10.2973/dsdp.proc.93.122.1987, 1987.
Harding, I. C., Smith, G. A., Riding, J. B., and Wimbledon, W. A. P.: Inter-regional correlation of Jurassic/Cretaceous boundary strata based on the Tithonian-Valanginian dinoflagellate cyst biostratigraphy of the Volga Basin, western Russia, Rev. Palaeobot. Palyno., 167, 82–116, https://doi.org/10.1016/j.revpalbo.2011.07.002, 2011.
Harland, R.: Dinoflagellate biostratigraphy of Neogene and Quaternary sediments at holes 400/400A in the Bay of Biscay (DSDP Leg 48), Initial Reports of the deep sea drilling project Leg 48, https://doi.org/10.2973/dsdp.proc.48.122.1979, 1979.
Harland, R.: Dinoflagellate cysts of the Quaternary system, in: A stratigraphix index of dinoflagellate cysts, edited by: Powell, A. J., British Micropaleontological Society Publications Series, London, UK, 253–273, ISBN-13 978-9401050524, 1992.
Head, M. J.: Pollen and dinoflagellates from the Red Crag at Walton-on-the-Naze, Essex: Evidence for a mild climatic phase during the early Late Pliocene of eastern England, Geol. Mag., 135, 803–817, 1998.
Head, M. J. and Norris, G.: Palynology and dinocyst stratigraphy of the Eocene and Oligocene in ODP Leg 105, Hole 647A, Labrador Sea, in: Proceedings of the ODP, Scientific Results, Leg 105, edited by: Srivastava, S. P., Arthur, M., and Clement, B., College Station, Texas, https://doi.org/10.2973/odp.proc.sr.105.178.1989, 1989.
Head, M. J. and Norris, G.: New species of dinoflagellate cysts and other palynomorphs from the latest Miocene and Pliocene of DSDP Hole 603C, Western North Atlantic, Journal of Palaeontology, 77, 1–15, https://doi.org/10.1666/0022-3360(2003)077<0001:NSODCA>2.0.CO;2, 2003.
Head, M. J., Norris, G., and Mudie, P. J.: 25. Palynology and dinocyst stratigraphy of the Upper Miocene and lowermost Pliocene, ODP Leg 105, Site 646, Labrador Sea, in: Ocean Drilling Program, Proceedings, Scientific Results, Leg 105, edited by: Srivastava, S. P. et al., College Station, Texas, 423–451, https://doi.org/10.2973/odp.proc.sr.105.135.1989, 1989.
Heilmann-Clausen, C.: Dinoflagellate stratigraphy of the uppermost Danian to Ypresien in the Viborg I borehole, central Jylland, Denmark, Danmarks Geologische Untersogelse A, 7, 1–69, 1985.
Heilmann-Clausen, C.: Lower Cretaceous dinoflagellate biostratigraphy in the Danish Central Trough, Danmarks Geologische Untersogelse A, 17, 1–89, 1987.
Heilmann-Clausen, C. and Van Simaeys, S.: Dinoflagellate cysts from the Middle Eocene to lowermost Oligocene succession in the Kysing research borehole, central Danish basin, Palynology, 29, 143–204, https://doi.org/10.1080/01916122.2005.9989606, 2005.
Helby, R. and McMinn, A.: A preliminary report of Early Cretaceous dinocyst floras from Site 765, Argo Abyssal Plain, northwest Australia, edited by: Gradstein, F. M. et al., Proc. ODP, Sci. Results, 123, 407–420, College Station, TX, https://doi.org/10.2973/odp.proc.sr.123.121.1992, 1992.
Helby, R., Morgan, R., and Partridge, A. D.: A palynological zonation of the Australian Mesozoic, in: Studies in Australian Mesozoic Palynology, edited by: Jell, P. A., Mem. Assoc. Australas. Palaeontol., 1987.
Hoek, R. P., Eshet, Y., and Almogi-Labin, A.: Dinoflagellate cyst zonation of Campanian-Maastrichtian sequences in Israel, Micropaleontology, 42, 125–150, 1996.
Hollis, C. J., Crouch, E. M., Morgans, H. E. G., Handley, L., Baker, J. A., Creech, J., Collins, K. S., Gibbs, S. J., Huber, M., Schouten, S., Zachos, J. C., and Pancost, R. D.: Tropical sea temperatures in the high latitude South Pacific during the Eocene, Geology, 37, 99–102, 2009.
Houben, A. J. P., Bijl, P. K., Guerstein, G. R., Sluijs, A., and Brinkhuis, H.: Malvinia escutiana, a new biostratigraphically important Oligocene dinoflagellate cyst from the Southern Ocean, Rev. Palaeobot. Palyno., 165, 175, https://doi.org/10.1016/j.revpalbo.2011.03.002, 2011.
Houben, A. J. P., Bijl, P. K., Pross, J., Bohaty, S. M., Passchier, S., Stickley, C. E., Röhl, U., Sugisaki, S., Tauxe, L., Van De Flierdt, T., Olney, M., Sangiorgi, F., Sluijs, A., Escutia, C., and Brinkhuis, H.: Reorganization of Southern Ocean plankton ecosystem at the onset of Antarctic glaciation, Science, 340, 341–344, https://doi.org/10.1126/science.1223646, 2013.
Houben, A. J. P., Bijl, P. K., Sluijs, A., Schouten, S., and Brinkhuis, H.: Late Eocene Southern Ocean cooling and invigoration of circulation preconditioned Antarctica for full-scale glaciation, Geochem. Geophy. Geosy., 20, 2214–2234, 2019.
Hoyle, T. M., Sala-Pérez, M., and Sangiorgi, F.: Where should we draw the lines between dinocyst “species”? Morphological continua in Black Sea dinocysts, J. Micropalaeontol., 38, 55–65, https://doi.org/10.5194/jm-38-55-2019, 2019.
Iakovleva, A. I. and Heilmann-Clausen, C.: Eocene dinoflagellate cyst biostratigraphy of research borehole 011-BP, Omsk region, southwestern Siberia, Palynology, 34, 195–232, 2010.
Iakovleva, A. I., Brinkhuis, H., and Cavagnetoo, C.: Late Paleocene-Early Eocene dinoflagellate cysts from the Turgay Strait, KAzachstan; correlations across ancient seaways, Palaeogeogr. Palaeocl., 172, 243–268, 2001.
Ioannides, N. S., Colin, J.-P., and Jan du Chêne, R.: A preliminary investigation of Kimmeridgian dinoflagellates and ostracodes from Quercy, southwest France, Bull. Cent. Rech. Elf E., 12, 471–491, 1988.
King, C., Iakovleva, A., Heilmann-Clausen, C., and Steurbaut, E.: Ypresian (early Eocene) stratigraphy of the Suvlu-Kaya reference section in the Bakhchisaray area (Crimea), Newsl. Stratigr., 51, 167–208, https://doi.org/10.1127/nos/2017/0384, 2018.
Kirsch, K. H.: Dinoflagellaten-Zysten aus der Oberkreide des Helvetikums und Nordultrahelvetikums von Oberbayern, Muenchner Geowiss. Abh. Reihe A, Geol. Palaeontol., 22, 1–306, 1991.
Köthe, A.: A revised cenozoic dinoflagellate cyst and calcareous nannoplankton zonation for the german sector of the southeastern north sea basin, Newsl. Stratigr., 45, 189–220, https://doi.org/10.1127/0078-0421/2012/0021, 2012.
Köthe, A., Khan, A. M., and Ahsraf, M.: Biostratigraphy of the Surghar Range, Salt Range, Sulaiman Range and the Kohat area, Pakistan, according to Jurassic through Paleogene calcareous nannofossils and Paleogene dinoflagellates, Geol. Jb. Reihe B, 71, 3–87, 1988.
Krijgsman, W., Hilgen, F. J., Langereis, C. G., Santarelli, A., and Zachariasse, W. J.: Late Miocene magnetostratigraphy, biostratigraphy and cyclostratigraphy in the Mediterranean, Earth Planet. Sc. Lett., 136, 475–494, https://doi.org/10.1016/0012-821X(95)00206-R, 1995.
Kuhlmann, G., Langereis, C. G., Munsterman, D., Leeuwen, R.-J. van, Verreussel, R., Meulenkamp, J. E., and Wong, T. E.: Integrated chronostratigraphy of the Pliocene-Pleistocene interval and its relation to the regional stratigraphical stages in the southern North Sea region, Geol. Mijnbouw, 85, 20–45, 2006.
Lebedeva, N. K., Aleksandrova, G. N., Shurygin, B. N., Ovechkina, M. N., and Gnibidenkoa, Z. N.: Paleontological and Magnetostratigraphic Data on Upper Cretaceous Deposits from Borehole no. 8 (Russkaya Polyana District, Southwestern Siberia), Stratigr. Geol. Correl., 21, 48–78, 2013.
Leereveld, H.: Dinoflagellate cysts from the Lower Cretaceous Rio Argos succession (southeast Spain), PhD thesis, Utrecht University, Laboratory of Palaeobotany and Palynology, Contributions series no. 2, Utrecht, the Netherlands, 1995.
Leereveld, H.: Upper Tithonian-Valanginian (Upper Jurassic-Lower Cretaceous) dinoflagellate cyst stratigraphy of the western Mediterranean, Cretaceous Res., 18, 385–420, 1997a.
Leereveld, H.: Hauterivian-Barremian (Lower Cretaceous) dinoflagellate cyst stratigraphy of the western Mediterranean, Cretaceous Res., 18, 421–456, 1997b.
Londeix, L. and Jan Du Chene, R.: Burdigalian dinocyst stratigraphy of the stratotypic area (Bordeaux, France), GEOBIOS, 31, 283–294, 1998.
Louwye, S., Head, M. J., and De Schepper, S.: Dinoflagellate cyst stratigraphy and Palaeoecology of the Pliocene in Northern Belgium, southern North Sea Basin, Geol. Mag., 141, 353–378, 2004.
Louwye, S., Mertens, K. N., and Vercauteren, D.: New dinoflagellate cysts from the Miocene of the Porcupine Basin, offshore southwest Ireland, Palynology, 32, 131–142, 2008.
MacRae, R. A., Fensome, R. A., and Williams, G. L.: Fossil dinoflagellate diversity, originations and extinctions and their significance, Can. J. Botany, 74, 1687–1694, 1996.
Mao, S. and Mohr, B. A. R.: Late Cretaceous dinoflagellate cysts (Santonian-Maestrichtian) from the Southern Indian Ocean (Hole 748C), in: Proceedings of the Ocean Drilling Program, Scientific results, volume 120, edited by: Wise, S. W. and Schlich, R., College Station, TX, USA, 1992.
Marret, F., Bradley, L., de Vernal, A., Hardy, W., Kim, S.-Y., Mudie, P., Penaud, A., Pospelova, V., Price, A. M., Radi, T., and Rochon, A.: From bi-polar to regional distribution of modern dinoflagellate cysts, an overview of their biogeography, Mar. Micropaleontol., 159, 101753, https://doi.org/10.1016/j.marmicro.2019.101753, 2020.
Martini, E.: Standard Tertiary and Quaternary calcareous nannoplankton zonation, in: Proceedings 2nd International Conference Planktonic Microfossils Roma, Rome, 1970, ed. Tecnosci., Vol. 2, edited by: Farinacci, A., 739–785, 1971.
Masure, E.: Berriasian to Aptian dinoflagellate cysts from the Galicia margin, offshore Spain, Sites 638 and 639, Leg 103, in: Proceedings of the Ocean Drilling Program, Scientific Results, volume 103, edited by: Boillot, G., Winterer, E. L., and et al., College Station, Texas, https://doi.org/10.2973/odp.proc.sr.103.183.1988, 1988.
Masure, E., Rauscher, R., Dejax, J., Schuler, M., and Ferre, B.: Cretaceous-Paleocene palynology from the Cote D'ivoire-Ghana Transform Margin, sites 959, 960, 961, and 962, Proc. Ocean Drill. Program Sci. Res., 159, 253–276, https://doi.org/10.2973/odp.proc.sr.159.040.1998, 1998.
Matsuoka, K., Bujak, J. P., and Shimazaki, T.: Late Cenozoic dinoflagellate cyst biostratigraphy from the west coast of northern Japan, Micropaleontology, 33, 214–229, 1987.
Matthiessen, J. and Brenner, W.: Dinoflagellate cyst ecostratigraphy of Pliocene-Pleistocene sediments from the Yermak Plateau (Arctic Ocean, Hole 911A), in: Proceedings of the Ocean Drilling Program, Scientific Results, vol. 151, College Station, Texas, USA, https://doi.org/10.2973/odp.proc.sr.151.109.1996, 1996.
McLachlan, S. M. S., Pospelova, V., and Hebda, R. J.: Dinoflagellate cysts from the upper Campanian (Upper Cretaceous) of Hornby Island, British Columbia, Canada, with implications for Nanaimo Group biostratigraphy and paleoenvironmental reconstructions, Mar. Micropaleontol., 145, 1–20, https://doi.org/10.1016/j.marmicro.2018.10.002, 2018.
McMinn, A.: Neogene dinoflagellate distribution in the eastern Indian Ocean from Leg 123, Site 765, in: Proc. ODP, Sci. Results, volume 123, edited by: Gradstein, F. M., Ludden, J. N., et al., College Station, TX, https://doi.org/10.2973/odp.proc.sr.123.120.1992, 1992.
McMinn, A.: Neogene dinoflagellate cyst biostratigraphy from sites 815 and 823, Leg 133, northeastern Australian margin, in: Proceedings of the Ocean Drilling Program, Scientific Results, volume 133, edited by: McKenzie, J. A., Davies, P. J., and Palmer-Julson, A., U.S. Government Printing Office, College Station, Texas, https://doi.org/10.2973/odp.proc.sr.133.219.1993, 1993.
Mertens, K. N. and Carbonell-Moore, C.: Introduction to Spiniferites Mantell 1850 special issue, Palynology, 42, 1–9, https://doi.org/10.1080/01916122.2018.1465741, 2018.
Mertens, K. N., Takano, Y., Head, M. J., and Matsuoka, K.: Living fossils in the Indo-Pacific warm pool: A refuge for thermophilic dinoflagellates during glaciations, Geology, 42, 531–534, https://doi.org/10.1130/G35456.1, 2014.
Mohr, B. A. R. and Mao, S.: Maastrichtian dinocyst floras from Maud Rise and Georgia Basin (Southern Ocean): Their Stratigraphic and Palaeoenvironmental implications, Palynology, 21, 41–65, 1997.
Montanari, A., Bice, D. M., Capo, R., Coccioni, R., Deino, A., DePaolo, D. J., Emmanuel, L., Monechi, S., Renard, M., and Zevenboom, D.: Integrated stratigraphy of the Chattian to mid-Burdigalian pelagic sequence of the Contessa Valley (Gubbio, Italy), in: Miocene Stratigraphy: an Integrated Approach, edited by: Montanari, A., Odin, G. S., and Coccioni, R., Elsevier, Amsterdam, the Netherlands, ISBN 0-444-82498-7, 1997.
Monteil, E.: Kystes de dinoflagellés index (Tithonique-Valanginien) du sud-est de la France: proposition d'une nouvelle zonation palynologique, Rev. Paleobiol., 11, 299–306, 1992.
Monteil, E.: Dinoflagellate cyst biozonation of the Tithonian and Berriasian of south-east France. Correlation with the sequence stratigraphy, Bull. Cent. Rech. Elf E., 17, 249–273, 1993.
Mudge, D. C. and Bujak, J. P.: An integrated stratigraphy for the Paleocene and Eocene of the North Sea, Geol. Soc. Sp., 101, 91–113, https://doi.org/10.1144/GSL.SP.1996.101.01.06, 1996.
Mudge, D. C. and Bujak, J. P.: Biostratigraphic evidence for evolving palaeoenvironments inthe Lower Paleogene of the Faeroe-Shetland Basin, Mar. Petrol. Geol., 18, 577–590, https://doi.org/10.1016/S0264-8172(00)00074-X, 2001.
Mudie, P. J.: Palynology and dinoflagellate biostratigraphy of DSDP Leg 94, Sites 607 and 611, North Atlantic Ocean, Initial Reports of the DSDP, vol. 94, Washington, USA, https://doi.org/10.2973/dsdp.proc.94.118.1987, 1987.
Nikitenko, B., Pestchevitskaya, E. B., Lebedeva, N. K., and Ilyina, V. I.: Micropalaeontological and palynological analyses across the Jurassic-Cretaceous boundary on Nordvik Peninsula, Northeast Siberia, Newsl. Stratigr., 42, 181–222, 2008.
Nøhr-Hansen, H., Sheldon, E., and Dam, G.: A new biostratigraphic scheme for the Paleocene onshore West Greenland and its implications for the timing of the pre-volcanic evolution, Geol. Soc. Sp., 197, 111–156, 2002.
Nøhr-Hansen, H., Piasecki, S., and Alsen, P.: A Cretaceous dinoflagellate cyst zonation for NE Greenland, Geol. Mag., 157, 1658–1692, 2020.
Ogg, J. G. and Hinnov, L. A.: Cretaceous, in: The Geologic Time Scale 2012, edited by: Gradstein, F. M., Ogg, J. G., Schmitz, M. D., and Ogg, G. M., Elsevier, Amsterdam, ISBN 978-0-12-824360-2, 2012a.
Ogg, J. G. and Hinnov, L. A.: Jurassic, edited by: Gradstein, F. M., Ogg, J. G., Schmitz, M. D., and Ogg, G. M., Elsevier, Amsterdam, ISBN 978-0-12-824360-2, 2012b.
Olde, K., Jarvis, I., Pearce, M., Uličný, D., Tocher, B., Trabucho-Alexandre, J., and Gröcke, D.: A revised Northern European Turonian (upper Cretaceous) dinoflagellate cyst biostratigraphy: Integrating palynology and carbon isotope events, Rev. Palaeobot. Palyno., 213, 1–16, https://doi.org/10.1016/j.revpalbo.2014.10.006, 2015.
Oosting, A. M., Leereveld, H., Dickens, G. R., Henderson, R. A., and Brinkhuis, H.: Correlation of Barremian-Aptian (mid-Cretaceous) dinoflagellate cyst assemblages between the Tethyan and Austral realms, Cretaceous Res., 27, 792–813, https://doi.org/10.1016/j.cretres.2006.03.012, 2006.
Pearce, M. A.: New organic-walled dinoflagellate cysts from the Cenomanian to Maastrichtian of the Trunch borehole, UK, J. Micropalaeontol., 29, 51–72, https://doi.org/10.1144/jm.29.1.51, 2010.
Petrizzo, M. R., Wade, B. S., and Gradstein, F. M.: Planktonic Foraminifera, in: The Geologic Time Scale 2012, edited by: Gradstein, F. M., Ogg, J. G., Schmitz, M. D., and Ogg, G. M., chapter 3, 74–87, Elsevier, Amsterdam, ISBN 978-0-12-824360-2, 2012.
Piasecki, S., Larsen, L. M., Pedersen, A. K., and Pedersen, G. K.: Palynostratigraphy of the Lower Tertiary volcanics and marine clastic sediments in the southern part of West Greenland Basin: implications for the timing and duration of the volcanism, Rapport Grønlands Geologiske Undersøgelse, 154, 13–31, 1992.
Poulsen, N. E.: Jurassic dinoflagellate cyst biostratigraphy of the Danish Subbasin in relation to sequences in England and Poland; a preliminary review, Rev. Palaeobot. Palyno., 75, 1–32, 1992.
Poulsen, N. E.: Dinoflagellate cyst biostratigraphy of the Late Jurassic of Poland, GEOBIOS, 17, 401–407, 1994.
Poulsen, N. E.: Upper Bajocian to Callovian (Jurassic) dinoflagellate cysts from central Poland, Acta Geol. Pol., 48, 237–245, 1998.
Poulsen, N. E. and Riding, J. B.: The Jurassic dinoflagellate cyst zonation of Subboreal Northwest Europe, Geol. Surv. Den. Greenl., 1, 115–144, 2003.
Powell, A. J.: Latest Palaeogene and Earliest Neogene dinoflagellate cysts from the Lemme section, NW Italy, AASP Contributions Series, 17, 83–104, 1986.
Powell, A. J.: A modified dinoflagellate cyst biozonation for latest Palaeocene and earliest Eocene sediments from the central North Sea, Rev. Palaeobot. Palyno., 56, 327–344, https://doi.org/10.1016/0034-6667(88)90064-4, 1988.
Powell, A. J.: Dinoflagellate cysts of the Tertiary System, in: A stratigraphic index of dinoflagellate cysts, edited by: Powell, A. J., British Micropaleontological Society Publications Series, London, UK, ISBN-13 978-9401050524, 1992.
Powell, A. J., Brinkhuis, H., and Bujak, J. P.: Upper Paleocene-lower Eocene dinoflagellate cyst sequence biostratigraphy of southeast England, edited by: R. W. O. Knox, R. M. Corfield, and R. E. Dunay, Geol. Soc. Spec. Publ., 101, 145–183, 1996.
Prince, I. M., Jarvis, I., Pearce, M. A., and Tocher, B. A.: Dinoflagellate cyst biostratigraphy of the Coniacian-Santonian (Upper Cretaceous): New data from the English Chalk, Rev. Palaeobot. Palyno., 150, 59–96, https://doi.org/10.1016/j.revpalbo.2008.01.005, 2008.
Pross, J., Houben, A. J. P., Simaeys, S. van, Williams, G. L., Kotthoff, U., Coccioni, R., Wilpshaar, M., and Brinkhuis, H.: Umbria-Marche revisited: A refined magnetostratigraphic calibration of dinoflagellate cyst events for the Oligocene of the Western Tethys, Rev. Palaeobot. Palyno., 158, 213–235, 2010.
Quaijtaal, W. and Brinkhuis, H.: Pentadinium alabamensis: A new, unusual dinoflagellate from the early Oligocene of the Gulf Coast, Alabama, USA, Rev. Palaeobot. Palyno., 175, 47–54, https://doi.org/10.1016/j.revpalbo.2012.03.002, 2012.
Quaijtaal, W., Donders, T. H., Persico, D., and Louwye, S.: Characterising the middle Miocene Mi-events in the Eastern North Atlantic realm: A first high-resolution marine palynological record from the Porcupine Basin, Palaeogeogr. Palaeocl., 399, 140–159, 2014.
Radmacher, W., Pérez-Rodríguez, I., Arz, J. A., and Pearce, M. A.: Dinoflagellate biostratigraphy at the Campanian-Maastrichtian boundary in Zumaia, northern Spain, Cretaceous Res., 51, 309–320, https://doi.org/10.1016/j.cretres.2014.07.004, 2014a.
Radmacher, W., Tyszka, J., Mangerud, G., and Pearce, M. A.: Dinoflagellate cyst biostratigraphy of the Upper Albian to Lower Maastrichtian in the southwestern Barents Sea, Mar. Petrol. Geol., 57, 109–121, 2014b.
Radmacher, W., Mangerud, G., and Tyszka, J.: Dinoflagellate cyst biostratigraphy of Upper Cretaceous strata from two wells in the Norwegian Sea, Rev. Palaeobot. Palyno., 216, 18–32, 2015.
Reichart, G.-J., Brinkhuis, H., Huiskamp, F., and Zachariasse, W. J.: Hyperstratification following glacial overturning events in the northern Arabian Sea, Paleoceanography, 19, PA2013, https://doi.org/10.1029/2003PA000900, 2004.
Riding, J. B. and Helby, R.: Early Jurassic (Toarcian) dinoflagellate cysts from the Timor Sea, Australia, Studies in Australian Mesozoic palynology II, Memoir of the Association of Australasian Palaeontologists, vol. 24, edited by: Laurie, J. R. and Foster, C. B., 1–32, lSSN 0810 8889, 2001a.
Riding, J. B. and Helby, R.: A selective reappraisal of Wanaea Cookson & Eisenack 1958 (Dinophyceae), Studies in Australian Mesozoic palynology II; Memoir of the Association of Australasian Palaeontologists, vol. 24, edited by: Laurie, J. R. and Foster, C. B., 33–58, lSSN 0810 8889, 2001b.
Riding, J. B. and Helby, R.: Phallocysta granosa sp. nov., a Mid Jurassic (Bathonian) dinoflagellate cyst from the Timor Sea, Australia., Studies in Australian Mesozoic palynology II; Memoir of the Association of Australasian Palaeontologists, vol. 24, edited by: Laurie, J. R. and Foster, C. B., 59–63, 2001c.
Riding, J. B. and Helby, R.: Microplankton from the Mid Jurassic (late Callovian) Rigaudella aemula Zone in the Timor Sea, north-western Australia, Studies in Australian Mesozoic palynology II; Memoir of the Association of Australasian Palaeontologists, vol. 24, edited by: Laurie, J. R. and Foster, C. B., 65–109, lSSN 0810 8889, 2001d.
Riding, J. B. and Helby, R.: Dinoflagellate cysts from the Late Jurassic (Oxfordian) Wanaea spectabilis Zone in the Timor Sea region, Studies in Australian Mesozoic palynology II; Memoir of the Association of Australasian Palaeontologists, vol. 24, edited by: Laurie, J. R. and Foster, C. B., 111–140, lSSN 0810 8889, 2001e.
Riding, J. B. and Helby, R.: Dinoflagellate cysts from the Late Jurassic (Kimmeridgian) Dingodinium swanense Zone in the North-West Shelf and Timor Sea, Australia., Studies in Australian Mesozoic palynology II; Memoir of the Association of Australasian Palaeontologists, vol. 24, edited by: Laurie, J. R. and Foster, C. B., 141–176, lSSN 0810 8889, 2001f.
Riding, J. B. and Helby, R.: Marine microplankton from the Late Jurassic (Tithonian) of the north-west Australian region., Studies in Australian Mesozoic palynology II; Memoir of the Association of Australasian Palaeontologists, vol. 24, edited by: Laurie, J. R. and Foster, C. B., 177–220, lSSN 0810 8889, 2001g.
Riding, J. B. and Thomas, J. E.: Dinoflagellate cyst stratigraphy of the Kimmeridge Clay (Upper Jurassic) from the Dorset Coast, Southern England, Palynology, 12, 65–88, 1988.
Riding, J. B. and Thomas, J. E.: Dinoflagellate cysts of the Jurassic System, in: A stratigraphix index of dinoflagellate cysts, edited by: Powell, A. J., British Micropaleontological Society Publications Series, London, UK, 7–98, ISBN-13 978-9401050524, 1992.
Riding, J. B. and Thomas, J. E.: Marine palynomorphs from the Staffin Bay and Staffin Shale formations (Middle–Upper Jurassic) of the Trotternish Peninsula, NW Skye, Scot. J. Geol., 33, 59–74, 1997.
Riding, J. B., Mantle, D. J., and Backhouse, J.: A review of the chronostratigraphical ages of Middle Triassic to Late Jurassic dinoflagellate cyst biozones of the North West Shelf of Australia, Rev. Palaeobot. Palyno., 162, 543–575, https://doi.org/10.1016/j.revpalbo.2010.07.008, 2010.
Riley, L. A. and Fenton, J. P. G.: A dinocyst zonation for the Callovian to iddle Oxfordian succession (Jurassic) of northwest Europe, Palynology, 6, 193–202, 1982.
Rochon, A., Lewis, J., Ellegaard, M., and Harding, I. C.: The Gonyaulax spinifera (Dinophyceae) “complex”: Perpetuating the paradox?, Rev. Palaeobot. Palyno., 155, 52–60, 2009.
Sangiorgi, F., Bijl, P. K., Passchier, S., Salzmann, U., Schouten, S., McKay, R., Cody, R. D., Pross, J., Van De Flierdt, T., Bohaty, S. M., Levy, R., Williams, T., Escutia, C., and Brinkhuis, H.: Southern Ocean warming and Wilkes Land ice sheet retreat during the mid-Miocene, Nat. Commun., 9, 317, https://doi.org/10.1038/s41467-017-02609-7, 2018.
Schiøler, P.: New species of dinoflagellate cysts from Maastrichtian-Danian chalks of the Danish North Sea, J. Micropalaeontol., 12, 99–112, https://doi.org/10.1144/jm.12.1.99, 1993.
Schreck, M. and Matthiessen, J.: Batiacasphaera bergenensis and Lavradosphaera elongata – new dinoflagellate cyst and acritarch species from the Miocene of the Iceland Sea (ODP Hole 907A), Rev. Palaeobot. Palyno., 211, 97–106, 2014.
Schreck, M., Matthiessen, J., and Head, M. J.: A magnetostratigraphic calibration of middle Miocene through Pliocene dinoflagellate cyst and acritarch events in the Iceland Sea (Ocean Drilling Program Hole 907A), Rev. Palaeobot. Palyno. 187, 66–94, https://doi.org/10.1016/j.revpalbo.2012.08.006, 2012.
Schreck, M., Meheust, M., Stein, R., and Matthiessen, J.: Response of marine palynomorphs to Neogene climate cooling in the Iceland Sea (ODP Hole 907A), Mar. Micropaleontol., 101, 49–67, https://doi.org/10.1016/j.marmicro.2013.03.003, 2013.
Schreck, M., Nam, C., Clotten, S. I., Fahl, K., De Schepper, S., Forwick, M., and Matthiessen, J.: Neogene dinoflagellate cysts and acritarchs from the high northern latitudes and their relation to sea surface temperature, Mar. Micropaleontol., 136, 51–65, https://doi.org/10.1016/j.marmicro.2017.09.003, 2017.
Shulgina, N. I., Burdykina, M. D., Basov, V. A., and Århus, N.: Distribution of ammonites, foraminifera and dinoflagellate cysts in the lower Cretaceous reference sections of the Khatanga Basin, and Boreal Valanginian biogeography, Cretaceous Res., 15, 1–16, 1994.
Skupien, P.: Albian non-calcareous dinoflagellates of the western Carpathians, Slovak Geological Magazine, 10, 203–214, 2004.
Skupien, P. and Vasícek, Z.: Lower Cretaceous ammonite and dinocyst biostratigraphy and paleoenvironment of the Silesian Basin (outher western Carpathians), Geol. Carpath., 53, 179–189, 2002.
Slimani, H. and Louwye, S.: New dinoflagellate cyst species of the Microdinium and Phanerodinium Complexes (Evitt) from the Upper Cretaceous-Lower Paleogene Chalk Group in the Meer borehole, northern Belgium, Rev. Palaeobot. Palyno., 168, 41–50, 2011.
Śliwińska, K. K., Abrahamsen, N., Beyer, C., Brünings-Hansen, T., Thomsen, E., Ulleberg, K., and Heilmann-Clausen, C.: Bio- and magnetostratigraphy of Rupelian-mid Chattian deposits from the Danish land area, Rev. Palaeobot. Palyno., 172, 48–69, 2012.
Śliwińska, K. K., Jelby, M. E., Grundvåg, S.-A., Nohr-Hansen, H., Alsen, P., and Olaussen, S.: Dinocyst stratigraphy of the Valanginian-Aptian Rurikfjellet and Helvetiafjellet formations on Spitsbergen, Arctic Norway, Geol. Mag., 157, 1693–1714, 2020.
Sluijs, A., Brinkhuis, H., Stickley, C. E., Warnaar, J., Williams, G. L., and Fuller, M.: Dinoflagellate cysts from the Eocene–Oligocene transition in the Southern Ocean: Results from ODP Leg 189, in: Proceedings of the ODP, Scientific Results, Leg 189, edited by: Exon, N. and Kennett, J. P., U.S. Government Printing Office, College Station, Texas, https://doi.org/10.2973/odp.proc.sr.189.104.2003, 2003.
Sluijs, A., Pross, J., and Brinkhuis, H.: From greenhouse to icehouse; organic walled dinoflagellate cysts as paleoenvironmental indicators in the Paleogene, Earth-Sci. Rev., 68, 281–315, 2005.
Sluijs, A., Schouten, S., Pagani, M., Woltering, M., Brinkhuis, H., Sinninghe Damsté, J. S., Dickens, G. R., Huber, M., Reichart, G.-J., Stein, R., Matthiessen, J., Lourens, L. J., Pedentchouk, N., Backman, J., Moran, K., and Expedition 302 Scientists: Subtropical Arctic Ocean temperatures during the Palaeocene/Eocene Thermal Maximum, Nature, 441, 610–613, 2006.
Sluijs, A., Brinkhuis, H., Schouten, S., Bohaty, S., John, C. M., Zachos, J. C., Reichart, G.-J., Sinninghe Damsté, J. S., Crouch, E. M., and Dickens, G. R.: Environmental precursors to rapid light carbon injection at the Palaeocene/Eocene boundary, Nature, 450, 1218–1221, 2007.
Smelror, M.: Late Bathonian to Early Oxfordian dinoflagellate cyst stratigraphy of Jameson Land and Milne Land, East Greenand, Rapp. Grønlands Geol. Unders., 137, 135–159, 1988a.
Smelror, M.: Bathonian to early Oxfordian dinoflagellate cysts and acritarchs from Kong Karls Land, Svalbard, Rev. Palaeobot. Palyno., 56, 275–303, https://doi.org/10.1016/0034-6667(88)90061-9, 1988b.
Smelror, M.: Jurassic stratigraphy of the western Barents Sea region: a review, GEOBIOS, 17, 441–451, 1994.
Smelror, M. and Dietl, G.: Dinoflagellates cysts of the Bathonian/Callovian boundary beds in Southern Germany, GEOBIOS, 17, 453–459, 1994.
Smelror, M. and Lominadze, T. A.: Callovian dinoflagellate cysts from the Caucasus, U.S.S.R., N. Jb. Geol. Palõont., Abh., 178, 147–166, 1989.
Smelror, M., Århus, N., Meléndez, G., and Lardies, M. Q.: A reconnaissance study of Bathonian to Oxfordian (Jurassic) dinoflagellates and acritarchs from the Zaragoza region (NE Spain) and Figueira da Foz (Portugal), Rev. Esp. Micropaleont., 23, 47–82, 1991.
Soliman, A., Coric, S., Head, M. J., Piller, W. E., and El Beialy, S. Y.: Lower and Middle Miocene biostratigraphy, Gulf of Suez, Egypt based on dinoflagellate cysts and calcareous nannofossils, Palynology, 36, 38–79, 2012.
Steeman, T., De Weirdt, J., Smith, T., De Putter, T., Mees, F., and Louwye, S.: Dinoflagellate cyst biostratigraphy and palaeoecology of the early Paleogene Landana reference section, Cabinda Province, Angola, Palynology, 44, 280–309, https://doi.org/10.1080/01916122.2019.1575091, 2020.
Stover, L. E. and Hardenbol, J.: Dinoflagellates and depositional sequences in the Lower Oligocene (Rupelian) Boom Clay Formation, Belgium, Bulletin de la Société Belge de Géologie, 102, 5–77, 1994.
Strauss, C. and Lund, J. J.: A Middle Miocene dinoflagellate cyst microflora from Papendorf near Hamburg, Germany, Mitt. Geol.-Palõont. Inst. Univ. Hamburg, 73, 157–189, 1992.
Thorn, V. C., Riding, J. B., and Francis, J. E.: The Late Cretaceous dinoflagellate cyst Manumiella: Biostratigraphy, systematics, and palaeoecological signals in Antarctica, Rev. Palaeobot. Palyno., 156, 436–448, 2009.
Tocher, B. A.: Campanian to Maestrichtian dinoflagellate cysts from the United States Atlantic margin, Deep Sea Drilling Project Site 612, Initial Reports DSDP, Leg 95, 419–428, https://doi.org/10.2973/dsdp.proc.95.114.1987, 1987.
Tocher, B. A. and Jarvis, I.: Dinoflagellate cyst distribution and stratigraphy of the lower–middle Cenomanian (Upper Cretaceous) at Fumichon, Normandy, northern France, Revue de Micropaleontologie, 37, 223–232, 1994.
Tocher, B. A. and Jarvis, I.: Dinocyst distributions and stratigraphy of two Cenomanian–Turonian boundary (Upper Cretaceous) sections from the western Anglo-Paris Basin, J. Micropalaeontol., 14, 97–105, https://doi.org/10.1144/jm.14.2.97, 1995.
Tocher, B. A. and Jarvis, I.: Dinoflagellate cyst distributions and the Albian–Cenomanian boundary (mid-Cretaceous) at Cordebugle, NW France and Lewes, southern England, J. Micropalaeontol., 15, 55–67, https://doi.org/10.1144/jm.15.1.55, 1996.
Torricelli, S.: Lower Cretaceous dinoflagellate cyst and acritarch stratigraphy of the Cismon APTICORE (southern Alps, Italy), Rev. Palaeobot. Palyno., 108, 213–266, https://doi.org/10.1016/S0034-6667(99)00041-X, 2000.
Torricelli, S.: Dinoflagellate cyst stratigraphy of the Scisti a Fucoidi Formation (Early Cretaceous) from Piobbico, Central Italy: calibrated events for the Albian of the Tethyan Realm, Riv. Ital. Paleontol. S., 112, 95–112, 2006.
Torricelli, S. and Rosa Amore, M.: Dinoflagellate cysts and calcareous nannofossils from the upper Cretaceous Saraceno formation (Calabria, Italy): Implications about the history of the Liguride Complex, Rivista Italiana di Paleontologia e Stratigrafia, 109, 499–516, 2003.
Torricelli, S., Knezaurek, G., and Biffi, U.: Sequence biostratigraphy and paleoenvironmental reconstruction in the early Eocene Figols Group of the Tremp–Graus Basin (South-Central Pyrenees, Spain), Palaeogeogr. Palaeocl., 232, 1–35, https://doi.org/10.1016/j.palaeo.2005.08.009, 2006.
Türkecan, A. T., Munsterman, D., Işik, U., Altiner, D., Pinar, M., Çevik, T., and Alay, Z.: Dinoflagellate cyst biostratigraphy of Miocene strata in the Adana Basin, Eastern Mediterranean, Turkey, Palynology, 42, 516–539, https://doi.org/10.1080/01916122.2017.1403389, 2018.
Van De Schootbrugge, B., Houben, A. J. P., Ercan, F. E. Z., Verreussel, R., Kerstholt, S., Janssen, N. M. M., Nikitenko, B., and Suan, G.: Enhanced Arctic-Tethys connectivity ended the Toarcian Oceanic Anoxic Event in NW Europe, Geol. Mag., 157, 1593–1611, https://doi.org/10.1017/S0016756819001262, 2019a.
Van De Schootbrugge, B., Richoz, S., Pross, J., Luppold, F. W., Hunze, S., Wonik, T., Blau, J., Meister, C., van der Weijst, C. M. H., Suan, G., Fraguas, A., Fiebig, J., Herrle, J. O., Guex, J., Little, C. T. S., Wignall, P. B., Püttmann, W., and Oschmann, W.: The Schandelah Scientific Drilling Project: A 25-million year record of Early Jurassic palaeo-environmental change from northern Germany, Newsl. Stratigr., 52, 249–296, 2019b.
van Helmond, N. A. G. M., Sluijs, A., Papadomanolaki, N. M., Plint, A. G., Gröcke, D. R., Pearce, M. A., Eldrett, J. S., Trabucho-Alexandre, J., Walaszczyk, I., van de Schootbrugge, B., and Brinkhuis, H.: Equatorward phytoplankton migration during a cold spell within the Late Cretaceous super-greenhouse, Biogeosciences, 13, 2859–2872, https://doi.org/10.5194/bg-13-2859-2016, 2016.
Van Hinsbergen, D. J. J., De Groot, L. V., Van Schaik, S. J., Spakman, W., Bijl, P. K., Sluijs, A., Langereis, C. G., and Brinkhuis, H.: A paleolatitude calculator for paleoclimate studies, PLOS One, 10, e0126946, https://doi.org/10.1371/journal.pone.0126946, 2015.
Van Mourik, C. A. and Brinkhuis, H.: The Massignano Eocene–Oligocene golden spike section revisited, Stratigraphy, 2, 13–30, 2005.
Van Mourik, C. A., Brinkhuis, H., and Williams, G. L.: Mid-to late Eocene organic-walled dinoflagellate cysts from ODP Leg 171B, offshore Florida, Geol. Soc. Spec. Publ., 183, 225–251, https://doi.org/10.1144/GSL.SP.2001.183.01.11, 2001.
Van Simaeys, S., de Man, E., Vandenberghe, N., Brinkhuis, H., and Steurbaut, E.: Stratigraphic and palaeoenvironmental analysis of the Rupelian-Chattian transition in the type region: evidence from dinoflagellate cysts, foraminifera and calcareous nannofossils, Palaeogeogr. Palaeocl., 208, 31–58, 2004.
Van Simaeys, S., Munsterman, D., and Brinkhuis, H.: Oligocene dinoflagellate cyst biostratigraphy of the southern North Sea Basin, Rev. Palaeobot. Palyno., 134, 105–128, https://doi.org/10.1016/j.revpalbo.2004.12.003, 2005.
Vellekoop, J., Smit, J., van de Schootbrugge, B., Weijers, J. W. H., Galeotti, S., Sinninghe Damsté, J. S., and Brinkhuis, H.: Palynological evidence for prolonged cooling along the Tunisian continental shelf following the K-Pg boundary impact, Palaeogeogr. Palaeocl., 426, 216–228, 2015.
Versteegh, G. J. M.: The onset of major Northern Hemisphere glaciations and their impact on dinoflagellate cysts an acritarchs from the Singa section, Calabria (southern Italy) and DSDP Holes 607/607A (North Atlantic), Mar. Micropaleontol., 30, 319–343, 1997.
Versteegh, G. J. M. and Zevenboom, D.: New genera and species of dinoflagellate cysts from the Mediterranean Neogene, Rev. Palaeobot. Palyno., 85, 213–229, 1995.
Vieira, M. and Jolley, D.: Stratigraphic and spatial distribution of palynomorphs in deep-water turbidites: A metastudy from the UK central North Sea Paleogene, Mar. Petrol. Geol., 12, 104683, https://doi.org/10.1016/j.marpetgeo.2020.104638, 2020.
Vieira, M., Casas-Gallego, M., Mahdi, S., and Fenton, J.: Impagidinium obscurum sp. nov., a marker dinoflagellate cyst for the Thanetian (Paleocene) of the North Sea and the Barents Sea, Palynology, 44, 382–390, https://doi.org/10.1080/01916122.2019.1630494, 2020.
Watkins, D. K. and Raffi, I.: Calcareous nannofossils, in: Geologic Time Scale 2020, Volume 1, edited by: Gradstein, F. M., Ogg, J. G., Schmitz, M. D., and Ogg, G. M., Elsevier, Amsterdam, 69–74, ISBN 0128243619, 2020.
Westerhold, T., Marwan, N., Drury, A. J., Liebrand, D., Agnini, C., Anagnostou, E., Barnet, J. S. K., Bohaty, S. M., De Vleeschouwer, D., Florindo, F., Frederichs, T., Hodell, D. A., Holbourn, A. E., Kroon, D., Lauretano, V., Littler, K., Lourens, L. J., Lyle, M., Pälike, H., Röhl, U., Tian, J., Wilkens, R. H., Wilson, P. A., and Zachos, J. C.: An astronomically dated record of Earth's climate and its predictability over the last 66 million years, Science, 369, 1383–1387, https://doi.org/10.1126/science.aba6853, 2020.
Williams, G. L. and Bujak, J. P.: Distribution patterns of some North Atlantic Cenozoic dinoflagellate cysts, Mar. Micropaleontol., 2, 223–234, 1977.
Williams, G. L., Stover, L. E., and Kidson, E. J.: Morphology and stratigraphic ranges of selected Mesozoic–Cenozoic dinoflagellate taxa in the Northern Hemisphere, Pap. Geol. Surv. Can., 92, 1–140, https://doi.org/10.4095/183916, 1993.
Williams, G. L., Brinkhuis, H., Pearce., M. A., Fensome, R. A., and Weegink, J. W.: Southern Ocean and global dinoflagellate cyst events compared: Index events for the late Cretaceous–Neogene, in: Proceedings of the ODP, scientific Results, volume 189, edited by: Exon, N. F., Kennett, J. P., and Malone, M. J., https://doi.org/10.2973/odp.proc.sr.189.107.2004, 2004.
Williams, G. L., Damassa, S. P., Fensome, R. A., and Guerstein, G. R.: Wetzeliella and its allies – The “hole” story: A taxonomic revision of the Paleogene dinoflagellate subfamily Wetzelielloideae, Palynology, 39, 289–344, https://doi.org/10.1080/01916122.2014.993888, 2015.
Williams, G. L., Fensome, R. A., and MacRae, R. A.: DINOFLAJ3, American Association of Stratigraphic Palynologists, Data Series no. 2, available at: http://dinoflaj.smu.ca/dinoflaj3, last access: 1 February 2022.
Willumsen, P. S.: Three new species of dinoflagellate cyst from Cretaceous-Paleogene (K-Pg) boundary sections at mid-Waipara River and Fairfield Quarry, South Island, New Zealand, Palynology, 36, 48–62, https://doi.org/10.1080/01916122.2011.642260, 2012.
Wilpshaar, M., Santarelli, A., Brinkhuis, H., and Visscher, H.: Dinoflagellate cysts and mid-Oligocene chronostratigraphy in the central Mediterranean region, Journal of the Geological Society of London, 153, 553–561, 1996.
Woollam, R. and Riding, J. B.: Dinoflagellate cyst zonation of the English Jurassic, Report of the Institute of Geological Sciences, Report 83/2, 1–42, 1983.
Wrenn, J. H. and Kokinos, J. P.: Preliminary comments on Miocene through Pleistocene dinoflagellate cysts from De Soto Canyon, Gulf of Mexico, American Association of Stratigraphic Palynologists, Contributions Series, 17, 169–225, 1986.
Zegarra, M. and Helenes, J.: Changes in Miocene through Pleistocene dinoflagellates from the Eastern Equatorial Pacific (ODP Site 1039), in relation to primary productivity, Mar. Micropaleontol., 81, 107–121, https://doi.org/10.1016/j.marmicro.2011.09.005, 2011.
Zevenboom, D.: Dinoflagellate cysts from the Mediterranean late Oligocene and Miocene, PhD thesis, Utrecht University, Utrecht, the Netherlands, 1995.
Zonneveld, K. A. F., Versteegh, G. J. M., Kasten, S., Eglinton, T. I., Emeis, K.-C., Huguet, C., Koch, B. P., de Lange, G. J., de Leeuw, J. W., Middelburg, J. J., Mollenhauer, G., Prahl, F. G., Rethemeyer, J., and Wakeham, S. G.: Selective preservation of organic matter in marine environments; processes and impact on the sedimentary record, Biogeosciences, 7, 483–511, https://doi.org/10.5194/bg-7-483-2010, 2010.
Zonneveld, K. A. F., Marret, F., Versteegh, G. J. M., Bogus, K., Bonnet, S., Bouimetarhan, I., Crouch, E., de Vernal, A., Elshanawany, R., Edwards, L., Esper, O., Forke, S., Grøsfjeld, K., Henry, M., Holzwarth, U., Kielt, J.-F., Kim, S.-Y., Ladouceur, S., Ledu, D., Chen, L., Limoges, A., Londeix, L., Lu, S.-H., Mahmoud, M. S., Marino, G., Matsouka, K., Matthiessen, J., Mildenhal, D. C., Mudie, P., Neil, H. L., Pospelova, V., Qi, Y., Radi, T., Richerol, T., Rochon, A., Sangiorgi, F., Solignac, S., Turon, J.-L., Verleye, T., Wang, Y., Wang, Z., and Young, M.: Atlas of modern dinoflagellate cyst distribution based on 2405 datapoints, Rev. Palaeobot. Palyno., 191, 1–197, https://doi.org/10.1016/j.revpalbo.2012.08.003, 2013.