the Creative Commons Attribution 4.0 License.
the Creative Commons Attribution 4.0 License.
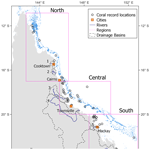
Coral skeletal proxy records database for the Great Barrier Reef, Australia
Helen V. McGregor
Tara R. Clark
Jody M. Webster
Stephen E. Lewis
Jennie Mallela
Nicholas P. McKay
Hugo W. Fahey
Supriyo Chakraborty
Tries B. Razak
Matt J. Fischer
The Great Barrier Reef (GBR), Australia, has a long history of palaeoenvironmental coral research. However, it can be logistically difficult to find the relevant research and records, which are often unpublished or exist as “grey literature”. This hinders researchers' abilities to efficiently assess the current state of coral core studies on the GBR and thus identify any key knowledge gaps. This study presents the Great Barrier Reef Coral Skeletal Records Database (GBRCD), which compiles 208 records from coral skeletal research conducted since the early 1990s. The database includes records from the Holocene, from ∼8000 years ago, to the present day; records are from the northern, central, and southern GBR from inshore and offshore locations. Massive Porites spp. coral records comprise the majority (92.5 %) of the database, and the remaining records are from Acropora, Isopora, or Cyphastrea spp. The database includes 78 variables, with , , and being the most frequently measured. Most records measure data over 10 or more years and are at monthly or lower resolution. The GBRCD is machine readable and easily searchable so that users can find records relevant to their research, e.g. by filtering for site name, time period, or coral type. It is publicly available as comma-separated values (CSV) data and metadata files with entries linked by the unique record ID and as Linked Paleo Data (LiPD) files. The GBRCD is publicly available from the NOAA National Center for Environmental Information World Data Service for Paleoclimatology at https://doi.org/10.25921/hqxk-8h74 (Arzey et al., 2024). The intention is to update the GBRCD annually, depending on the availability of relevant new GBR records or submission of legacy records to the GBRCD for archiving. The collection and curation of existing GBR coral research data provide researchers with the ability to analyse common proxies such as across multiple locations and/or examine regional to reef-scale trends. The database is also suitable for multi-proxy comparisons and combination or composite analyses to determine overarching changes recorded by the proxies. This database represents the first comprehensive compilation of coral records from the GBR. It enables the investigation of multiple environmental factors via various proxy systems for the GBR, northeastern Australia, and potentially the broader Indo-Pacific region.
- Article
(15770 KB) - Full-text XML
- BibTeX
- EndNote
Scleractinian coral skeletons have long been used to reconstruct past changes of reef environments and climates, as well as to understand reef responses to those changes (e.g. Druffel and Griffin, 1993; Webster et al., 2018; Thompson, 2022; Clark et al., 2017; Felis et al., 2014). Foundational to the field are studies on corals from the World Heritage-listed Great Barrier Reef (GBR). Studies on GBR corals have established, for example, the relationships between coral skeletal and δ18O with sea surface temperature (SST) (Weber and Woodhead, 1969, 1970, 1972; Weber, 1973; McCulloch et al., 1994), coral luminescence with riverine inputs (Isdale, 1984; Boto and Isdale, 1985; Isdale et al., 1998), and coral radiocarbon with oceanographic processes (Druffel and Griffin, 1993), as well as the environmental signature recorded in coral density bands (Lough and Barnes, 1990, 1997), evidence of sediment and nutrient exposure (McCulloch et al., 2003; Wyndham et al., 2004; Sammarco et al., 1999), and a host of new analytical methods and approaches (Gagan et al., 1998; Sinclair et al., 1998; Barnes et al., 2003). In addition, palaeoclimate and palaeoenvironmental research based on GBR corals (typically massive Porites spp.) has advanced our knowledge on the most pressing threats to the GBR and coral reef ecosystems more broadly (McCulloch et al., 2003; De'ath et al., 2009; Lough et al., 2015; Wei et al., 2009; Koop et al., 2001), tropical climate processes (Hendy et al., 2002; Lough, 2007; Druffel and Griffin, 1993), reef responses to climate and sea-level change (Webster et al., 2018; Sanborn et al., 2020; Yokoyama et al., 2011; Leonard et al., 2020), and coral bleaching (Suzuki et al., 2003; D'Olivo and McCulloch, 2017; De'ath et al., 2012).
Despite the long history of coral palaeoclimate and environmental research on the GBR, the GBR coral research data like other research fields are being lost to time (Vines et al., 2014). While there is a recent push to ensure that coral geochemical records are publicly available upon publication (Kaufman and PAGES 2k special-issue editorial team, 2018; Khider et al., 2019; Dassié et al., 2017), many GBR coral core records are not yet publicly archived; thus, we risk losing these valuable datasets. The need to publicly archive GBR coral geochemical data is even more pressing as corals are under threat from human-induced stresses (Hughes et al., 2017; Maynard et al., 2015; Guan et al., 2020; Ortiz et al., 2018). Long-term monitoring of the GBR indicates that reef-wide hard coral cover was down to 18 % in 2017 (Australian Institute of Marine Science, 2017). Since then, hard coral cover has increased, but recovery has primarily been driven by fast-growing Acropora species (Australian Institute of Marine Science, 2017, 2022), which are less suitable for producing continuous multi-century records. The future of GBR corals and therefore the valuable geochemical records they contain faces an uncertain future, which makes existing GBR records a precious and potentially finite resource.
This study presents a comprehensive database of GBR coral geochemical and luminescence records, as well as associated variables such as growth characteristics (where available), compiling both published records (201) and records published here for the first time (7 records). The GBR Coral Skeletal Records Database (GBRCD) transfers data into a standardised machine-readable format (structured using a consistent logic readable by and between machines) and makes the records available to researchers in a searchable database. The database consists of two main parts: (1) individual comma-separated values (CSV) files consisting of a single coral's geochemical and luminescence measurements with the associated age estimate and (2) a metadata file for the entire suite of corals, which includes details of location, genus or species, analytical techniques, publications, cross-references, and other details. Alternatively, the GBRCD is available as Linked Paleo Data (LiPD) files with serialisations in Python, MATLAB, and R, which include the same fields standardised to the LiPD format and terminology. Basic analysis of the scope of the database is presented, as is a discussion of database caveats and future use. The GBRCD addresses the potential loss of existing GBR coral data and provides the ability to easily explore what records exist. The GBRCD is a valuable resource for exploratory analysis to understand the GBR (and the wider Pacific) environment (e.g. Henley et al., 2024) and preserves the records for future use.
1.1 GBR setting
The GBR is made up of more than 3000 reefs that span 14° of latitude, and the World Heritage area comprises an area of 348 000 km2. The width of the GBR across the continental shelf changes with latitude, ranging from 50 to 250 km wide (Steinberg, 2007; Hopley et al., 2007). Thus, coral reefs in the GBR can be classified based on their proximity to the Queensland coast and sea floor depth as either inner-shelf, mid-shelf, or outer-shelf reefs and; depending on their classification, these reefs will experience various gradients of terrigenous or open ocean influences. There is a seasonal atmospheric circulation that is characterised by an annual summer monsoon (December to March), which brings rainfall and increased river discharge to neighbouring GBR marine waters.
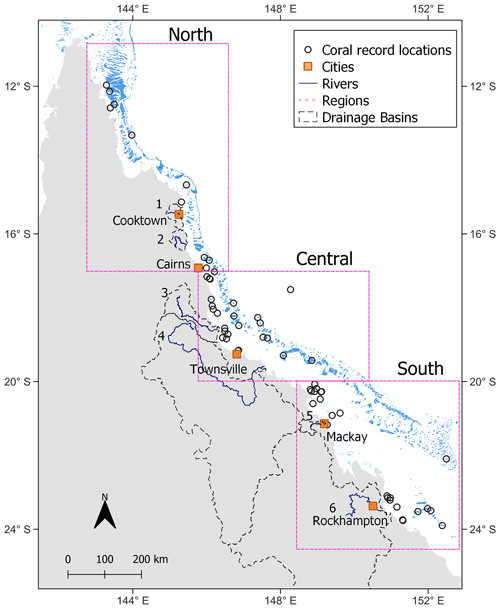
Figure 1Map of locations with coral records in the Great Barrier Reef Coral Skeletal Records Database. Record locations are indicated by circles; select cities are indicated by orange squares; select rivers are indicated by dark blue lines; reefs are in light blue; and the nominal regions of the north, central, and south GBR are indicated by the dashed pink lines. Numbers identify rivers – 1: Endeavour River, 2: Daintree River, 3: Herbert River, 4: Burdekin River, 5: Pioneer River, and 6: Fitzroy River. The drainage basin boundary associated with the included rivers is indicated by the dashed black lines. GIS layers © State of Queensland (Department of Resources) 2023. This material is licensed under a Creative Commons – Attribution 4.0 International licence (https://creativecommons.org/licenses/by/4.0/, last access: 9 February 2024). See Appendix E for a full list of included GIS layers.
Average SST varies latitudinally, with a 1.65 °C difference between the northern region (11–17° S) and the southern region (20–24° S) (Fig. 1) for the 1981–2010 period (HadISST; Rayner et al., 2003). The timing of the annual minimum and maximum also varies slightly; mean maximum SST in the 1981–2010 period (HadISST1.1) most frequently occurs in February in the central and southern GBR and in January in the northern GBR, while minimum SSTs most frequently occur in August in the northern and southern GBR and in July in the central GBR.
The modern GBR coral reef ecosystem initiated ∼8000–9000 years ago (ka) following the Holocene marine transgression (Davies and Hopley, 1983; Davies et al., 1985; Dechnik et al., 2015; Hopley et al., 2007), although the timing of individual coral reef initiation and vertical accretion rates varied considerably (range of 1–16 m per 1000 years) (Dechnik et al., 2017; Sanborn et al., 2020; Davies and Hopley, 1983; Hopley et al., 2007; Leonard et al., 2020; Ryan et al., 2018). Decreases in reef growth rate occur where the reef surface is within ∼ 2–3 m of sea level due to reduced growth and/or erosional loss (Davies and Hopley, 1983). Evidence from reef drilling suggests that there was little latitudinal variation in reef growth rate throughout the GBR, although the depth to the Pleistocene reef foundations in the northern and southern GBR regions is shallower than the central region (e.g. Davies et al., 1985; Hopley et al., 2007). Additionally, there are reef-flat growth hiatuses in both the northern and southern GBR, but not in the central GBR, due to a relative fall in sea level of ∼0.5 m in the other regions that was not evident in the central GBR due to subsidence in the central region (Halifax Basin) from hydro-isostatic adjustment (Dechnik et al., 2017).
1.2 Palaeoclimate and palaeoenvironment reconstructed from GBR coral skeletons
Research on the GBR has a long history of discovery and scientific development, with ventures as far back as the 1928–1929 GBR expedition lauded as having promoted the development of empirical and analytical approaches important to the foundation of modern coral reef science (Spencer et al., 2021). Coral skeletons provide in situ records of the reef environment as they incorporate trace elements, a variety of element isotopes, and organic materials in proportion to climate and environmental variations in the marine environment. These palaeoclimate and palaeoenvironment proxies are a long-established method to assess the reef environment in the past and present, and they provide a means to quantify relative changes over space and time. Proxy records from coral skeletons offer the ability to extract high-resolution (weekly–monthly) information to assess a range of climate and environmental variables, most commonly SST and rainfall, and at a variety of scales, e.g. near-weekly records that extend from the present day back several centuries prior to the instrumental record (e.g. Lewis et al., 2012; D'Olivo and McCulloch, 2022).
Beginning in the late 1960s, studies using GBR corals provided empirical evidence of a relationship between skeletal oxygen isotopes, luminescence, trace elements, radiocarbon, density, and the coral's marine environment (Weber and Woodhead, 1969, 1970, 1972; Isdale, 1984; Druffel and Griffin, 1993; Lough and Barnes, 1990; McCulloch et al., 2003), and these studies were at the forefront of the development of new technologies and methods to measure these coral properties (Isdale et al., 1998; Gagan et al., 1998; Sinclair et al., 1998; Barnes et al., 2003; D'Olivo et al., 2018).
After the description of the utility of as an SST proxy by Beck et al. (1992), coral skeleton proxy research in the GBR focused on development and calibration of proxy–SST relationships in the 1990s. GBR coral records from this period are generally short (< 10 years) and were analysed using a variety of spectrometry methods (Alibert and McCulloch, 1997; Druffel and Griffin, 1993, 1999; McCulloch et al., 1994; Gagan et al., 1998; Sinclair et al., 1998). Research in the 1990s also included the first application of paired and δ18O proxies to reconstruct the GBR palaeoenvironment (McCulloch et al., 1994; Gagan et al., 1998) and the further exploration of coral luminescence as a proxy for river discharge (Isdale et al., 1998). These approaches were applied to extend our knowledge of GBR SST, riverine input, and oceanographic variability prior to the 1800s (Lough, 2007, 2011b; Hendy et al., 2002, 2003a; Calvo et al., 2007) and during the Holocene (Gagan et al., 1998; Leonard et al., 2016; Sadler et al., 2016b; Lough et al., 2014; Roche et al., 2014) .
Since the 2000s, partially facilitated by the development of the Australian Institute of Marine Science luminometer and The Australian National University laser ablation methods, research on coral proxies in the GBR has focused on proxies for terrigenous inputs. These include indicators of terrestrial runoff and sediment exposure (e.g. and rare earth elements (REEs) (Jupiter et al., 2008; Jupiter, 2008; Saha et al., 2021; McCulloch et al., 2003; Lewis et al., 2012; Leonard et al., 2019)), land-use changes (e.g. δ15N and Mn (Lewis et al., 2007, 2012; Erler et al., 2016, 2020; Marion et al., 2021)), and river flow patterns (e.g. and luminescence (Lough et al., 2002; Lough, 2007; Lough et al., 2014; D'Olivo and McCulloch, 2022)). Additionally, there is continuing research that aims to explore new proxies (e.g. (Saha et al., 2019a) and δ98Mo (Wang et al., 2019)), as well as to develop proxies relevant to global issues such as ocean acidification (e.g. δ11B (Pelejero et al., 2005; Wei et al., 2009; D'Olivo et al., 2015; McCulloch et al., 2017)).
2.1 Selection criteria for inclusion in GBRCD
The GBRCD was not assembled around a specific research goal, unlike other coral palaeoclimate databases such as the CoralHydro2k database (Walter et al., 2023), which was designed to support “the project's goal of reconstructing tropical hydroclimatic variability at seasonal and longer timescales”. The GBRCD compiles and makes available coral geochemical and luminescence data from the GBR in a user-friendly format to facilitate their use in research. The GBRCD is similar to the Coral Trait Database (Madin et al., 2016) but focuses on the GBR and coral proxy measurements (i.e. geochemical and luminescence measurements). Therefore, only three broad selection criteria are considered for including a record in the GBRCD:
-
Location. The GBRCD compiles coral records from the GBR and, due to its regional context and latitudinal relevance, Flinders Reef.
-
Length. The records are continuous (multi-year) measurements from coral skeletons.
-
Age model. Each record includes a relevant chronology (i.e. an estimate of age as the independent variable) or has a chronology that could be recreated relatively easily based on available data.
All available geochemical or luminescence data were included in the GBRCD if they met these three criteria. No screening was applied on the quality of the data, and no records were excluded based on analytical uncertainties, diagenesis screening, resolution (e.g. weekly, monthly, annual), significance of the correlation between proxy and target parameter, or record length. It is at the discretion of researchers using data from the GBRCD to determine the suitability for their aims.
Where available, additional variables, such as coral growth data (density, extension, etc.), are included alongside the geochemical and luminescence data for records in the GBRCD where the additional variable data are at the same resolution as the geochemical or luminescence measurements.
2.2 Data sources
The majority of GBR coral records are from peer-reviewed published studies (see Appendix Table A1). In many cases, the published records were publicly archived in repositories such as the NOAA National Centers for Environmental Information World Data Service for Paleoclimatology (NOAA/WDS Paleoclimatology) and World Data Center PANGAEA. The GBRCD includes digital object identifier (DOI) information linking back to these original sources and publications. In some cases, published studies included additional records that were not archived in public repositories. Instead, these data were obtained from the publication's supplemental material or by reaching out to the corresponding authors. As part of the GBRCD compilation process, 114 previously unarchived records, as well as 7 previously unpublished records, were submitted to NOAA/WDS Paleoclimatology for archiving. These files are in the standard NOAA/WDS Paleoclimatology (tab-delimited) file format.
Seven records have not been published previously and are published in the GBRCD for the first time (Table 1 and Appendix A and C). These records are accompanied by their associated metadata record and appropriate background information (see Appendix A, C, D). If further information about the record is required, users are encouraged to reach out to the first author/contact listed in the GBRCD publication metadata.
Records included in the database are multi-year measurements of coral geochemistry and luminescence signals. In total, there are 78 variables available to investigate the GBR environment or to determine new proxy–environment relationships. The records include commonly measured isotopes and trace elements (TEs) such as δ18O, , , and , as well as less explored isotopes (such as δ11B and δ15N) and emerging TE proxies such as and rare earth elements (REEs). Luminescence records are included in the database as coral luminescence is a proven hydrological proxy in the GBR (Lough, 2011a; Lough et al., 2014).
Care is advised for the use of all records in the GBRCD as the relevance of the measured variables may vary by location, or the sampling resolution of the records may not be suitable. Therefore, further processing needs to be considered before integrating or comparing the records.
2.3 Database structure
The database is provided in a split form and includes a table of metadata describing each record, as well as individual CSV files that include the coral variables such as age, distance down core, measured trace elements, and so on. It is also provided as LiPD files and serialisations with the metadata fields structured as per the LiPD format (see Table B1). The database is available in both formats to facilitate ease of use. The CSV files are easily accessible and do not need programming languages (e.g. R or Python) to explore the datasets, whereas the LiPD files require programming languages to fully access that data and are designed as structured data to enable interoperability and quick analysis of palaeoclimate data (McKay and Emile-Geay, 2016).
The metadata supplied alongside the coral record data provide a thorough background on each record, with assumptions and limitations made clear for researchers. While every best attempt has been made to provide information for all metadata fields that are relevant, there are some fields where the information was not available (i.e. flagged with “NA”). Metadata fields allow for filtering by site, coral genus or species, analysis method, proxy type, etc.
Individual CSV and LiPD files are provided for each coral record. In general, an individual record is defined as being from a single coral, whether a coral head was drilled directly or the coral was retrieved from a core that was drilled into a coral reef matrix (coral reef matrices can intersect individual coral heads as well as the reef framework in which they are preserved).
In some instances, multiple geochemical and/or luminescence records have been measured from the same coral head. In this situation, the multiple records have their own separate entries as they are usually either part of an included composite record, measured using different techniques, or have sufficient differences in the metadata or dataset to provide a good reason not to attempt to join the datasets. However, they are identified and cross-referenced in the database as being from the same coral or core.
2.4 Data processing
Published data, as was available or supplied, have been archived in the database format described here. Potentially abnormal data (e.g. values <0 for (after correcting against a method and/or lab standard)) were not removed from the datasets as they may be indicative of climate or environmental events, and it is up to the user to determine appropriate screening procedures when using the database.
A basic quality check was conducted on records published for the first time in the GBRCD (see Appendix C). This consisted of creating or adjusting the coral age model based on available information and checking for diagenetic alteration where there was a physical sample available.
Coral records that were archived or supplied with the ages in standard date format (e.g. DD/MM/YYYY) had all ages converted to decimal date in the Common Era (CE) or Before Common Era (BCE) format. This means that there is no year zero, and negative (BCE) numbers start at “−1”. Since there are rounding issues with decimal dates assigned to the first day of the month (at midnight), 12 h was added to each date to ensure the age remained assigned to the correct month of the year.
For archiving purposes, 16 records were assigned a new age model. This was primarily because the coral data were not available with an age model or did not have one appropriate for archiving (e.g. date not assigned to each data point). A note is included in the relevant field about the chronology for records where the chronology was (re)created for archiving in the GBRCD, and further details are provided in Appendix C. Nine fossil records were supplied or previously archived with chronologies relative to the record itself (i.e. 0 = first or youngest year of record). The available dating information was used to transform these chronologies to Common Era ages.
Before Common Era fossil coral data are included in the GBRCD. Absolute age estimates for these corals are based on uranium–thorium (U-Th, or U-series) dating and radiocarbon (14C) measurements, with basic information about the dating supplied in the GBRCD metadata. Five coral records dated by radiocarbon measurements were recalibrated using CALIB rev. 8 (Stuiver and Reimer, 1993) and the Marine20 radiocarbon age calibration curve (Heaton et al., 2020) to standardise the age estimates, and details (including the local delta-R reservoir correction) are available in the “dating_notes” field. Users of the GBRCD should determine if the radiocarbon ages of the fossil records should be revised based on future updates to the calibration curves and/or reservoir ages. For records where U-Th dating information is reported for the first time in this study, information is provided in the GBRCD metadata, and complete relevant U-Th dating information (Dutton et al., 2017) is included in Appendix Table D1.
Relevant metadata were obtained from source publications and, where necessary, subsequent or cited publications and data archives. Coral IDs were also matched across publications using literature searches and the AIMS reef core database, and subsequently cross-checked within the GBRCD.
2.5 Database records and identification
Records are identified here as datasets from a single coral that may include one or more measured variables unified with a single age model. Similar to the Iso2k database protocols (Konecky et al., 2020), each record in the database was given a nine-digit alphanumeric ID. The dataset ID was created from the first two letters of the first author's last name, the last two numbers of the year of publication, the first three letters of the location (or in some cases a commonly accepted shortening or three-letter acronym for the location was used), and two numbers that represent the core number. If data were not previously published, publication year was substituted with the year of this compilation. For reef locations in the GBR that are currently represented by a number rather than a name and for records that are the composite of multiple locations, “GBR” has been used as the three-letter reef location for the GBRCD ID.
However, the Iso2k nine-digit alphanumeric identifier was insufficient to separate records where researchers have published multiple papers from the same GBR location within the same year. To separate these records, a 10th digit was added to the ID, adding an alphabetical end code of “a”, “b”, and so on following the order of publication (Table 2), similar to many journal standards for uniquely identifying references. Additionally, where the core record has been split due to notable differences in the record (e.g. due to data resolution or age model), a split identifier of “_1” or “_2” has been appended to the end of the identifier (Table 2).
2.6 Database variables, units, and standards
Records are all standardly formatted as CSV and LiPD files, with each record containing an age field and variable field(s) as per Table 3. Each GBRCD record includes between 1 and 33 variables.
Table 4Data variables. Describes variables included in the database, units, type, and brief description. The list of variables is alphabetical.
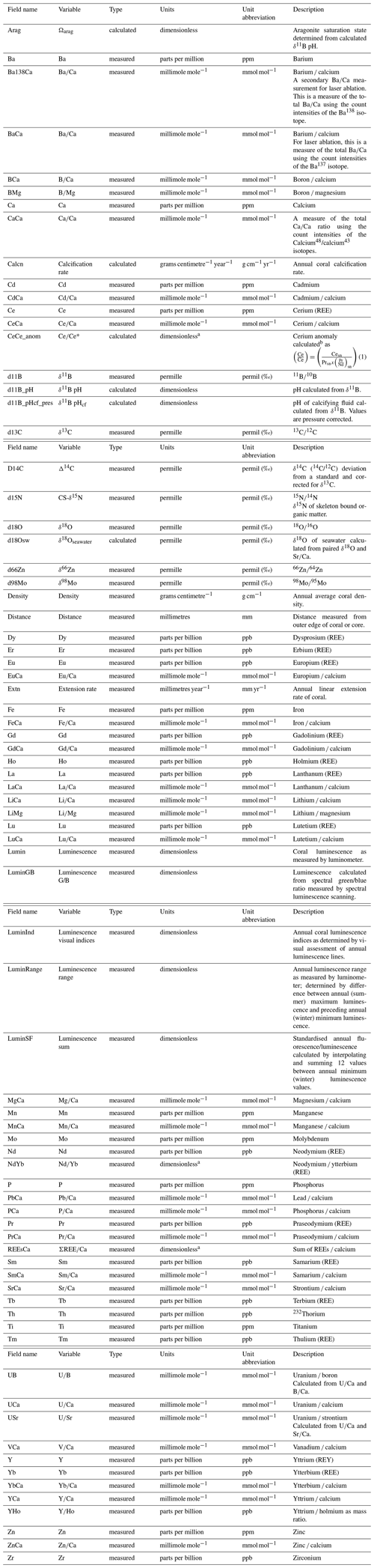
a data considered dimensionless (Narottam Saha, personal communication, 2023)
b * formula (Eq. 1) from Saha et al. (2019b) per Lawrence et al. (2006)
The database collates 78 variables obtained from coral records: 72 measured and 6 calculated variables (Table 4).
Units reported in the database were standardised to enable interoperability among records. All trace element / calcium () ratios were included (as mmol mol−1), and where relevant, the calibrations (proxy–SST) for the ratios included in the record metadata are in the same units. Isotopes were archived as permille (‰), and, where relevant, the calibrations for the ratios are in the same units. The majority of non-ratio elements were archived as parts per million. The exceptions to this were the rare earth elements (REEs) (lanthanum (La), cerium (Ce), praseodymium (Pr), neodymium (Nd), samarium (Sm), europium (Eu), gadolinium (Gd), terbium (Tb), dysprosium (Dy), holmium (Ho), erbium (Er), thulium (Tm), ytterbium (Yb), and lutetium (Lu)), yttrium (Y), and zirconium (Zr), which were archived as parts per billion (i.e. 10−9).
The list of included dataset field names for each record is provided in the CSV version metadata file (i.e. “meths_primaryVariableList” or “meths_additionalVariableList”; Table 4).
All records include an “Age” field that is the age of each sample as determined by the coral age model. For records that are at greater than annual resolution, the age supplied is representative of the grouping of years included in each data point. For example, the Druffel and Griffin (1999) biennial data list the middle year of the sampled coral (i.e. October 1889 to October 1891 has the age of 1890.8), while Hendy et al. (2002) quinquennial resolution data list the middle year of the sampled coral (i.e. 1981 to 1985 has the age of 1983). For sub-annual resolution records, the tie point within each month varies depending on the researchers' choice in the original publications. It is up to researchers to inspect the data to understand the “Age” field for each record they intend to use.
2.7 Coral database metadata
The GBRCD contains 104 metadata fields in the CSV version (reduced or combined into 76 fields in the LiPD version; Table B1) that enable identification and investigation of the coral records. Information for the metadata fields have been sourced from publications, supplementary materials, data archive information, and personal communications with researchers.
Nine fields are notes or free-form text fields that provide useful information for future investigations and understanding the record that cannot be succinctly reduced to controlled vocabulary and/or a numerical value.
The metadata included in the GBR coral record database were divided into six categories (identifier, geographic, publication, analysis, calibration, and dating), based on best practice standards suggested by the Marine Annually Resolved Proxy Archives (MARPA) (Dassié et al., 2017), Paleoclimate Community reporTing Standard (PaCTS) 1.0 (Khider et al., 2019), and Paleoenvironmental Standard Terms (PaST) Thesaurus (Morrill et al., 2021), and standards implemented in other palaeo-archive databases such as Iso2k and CoralHydro2k (Walter et al., 2023; Konecky et al., 2020).
Table 5Identifier metadata for the CSV version of the GBRCD for each coral record including database ID, publication core name, species, and time span.
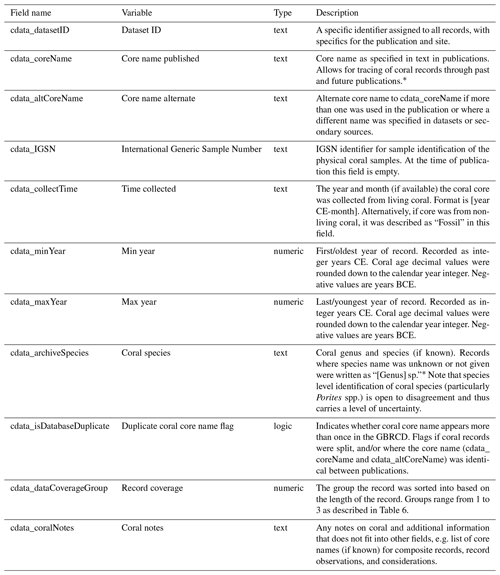
∗ Descriptions based on Walter et al. (2023) CoralHydro2k database fields.
2.7.1 Identifier metadata
Identifier fields were used to distinguish and characterise each coral record and identify potential crossover (or duplication) between records in the database and other research (Table 5). Identifier fields include core names (as specified in the original publication(s)), International Generic Sample Number (IGSN), core collection time, coral archive species, and the record period (maximum (max) and minimum (min) year). The metadata for the record period aim to maximise users' abilities to find relevant records that may only include measurements for part of a year; for example, the max year for records ending in March 2000 or November 2000 would be 2000 for both.
The database includes 67 duplicate core names, which generally indicate different types of analyses on the same coral head, reflecting the development and use of new analysis techniques. For example, may be measured from the same core using different methods (e.g. inductively coupled plasma atomic emission spectrometer (ICP-AES) or laser ablation inductively coupled plasma mass spectrometer (LA-ICP-MS)) or may be measurements of different variables (e.g. and luminescence). However, it also demonstrates the concentration of analyses on particular coral heads. For example, 5 of the 21 records from Magnetic Island in the central GBR are analyses from MAG01D and include an approximately weekly resolution multi-trace-element LA-ICP-MS record, approximately annual resolution stable isotope record, biennial resolution multi-trace-element inductively coupled plasma mass spectrometer (ICP-MS) record, quinquennial resolution stable isotope and trace-element record, and annual resolution luminescence record.
A notes field (“cdata_coralNotes”; Table 5) is also included for additional information on the coral, including issues and observations for consideration if using the record.
2.7.2 Geographic metadata
Geographic fields (Table 7) provide further core identification information and give the physical location of the coral archive: geographic coordinates, site name, and depth of the colony (termed “elevation” below).
Coral records that did not have listed GPS coordinates for the data were assigned GPS coordinates using the “Reefs and shoals – Queensland” dataset (EPSG:4283 – GDA94 coordinate reference system) from the State of Queensland (Department of Natural Resources, Mines and Energy) 2023, available under a Creative Commons – Attribution 4.0 International (https://creativecommons.org/licenses/by/4.0/, last access: 9 February 2024) licence. GPS coordinates were approximately matched to the supplied location if a sufficiently detailed map was included in the publication or the coordinates approximating the reef centre were used. Where coordinates were supplied for the GBRCD or the coordinates have been changed or updated from what was published in the original publication, this is noted in the “geo_notes” field.
Elevation information is supplied for coral records in the “geo_elevation” field. This value includes uncertainties inherent in the published data as elevation may describe depth below sea level to top or bottom of the coral, and this is not always explicitly described. Likewise, the choice of height datum used to describe sea level is also not always explicitly stated. Fossil coral records from drill core matrices have the depth down core range (if known), as well as an estimated depth within that range, noted in the “geo_notes” field. As there is uncertainty around the estimated depth down core, as well as uncertainty due to past changes in GBR sea level compared to present-day sea level (Lewis et al., 2013; Leonard et al., 2020; Hopley et al., 2007), it is left to the user to convert depth down core to an elevation relative to present-day sea level for fossil corals.
2.7.3 Dating metadata
The GBRCD includes 18 BCE records that have chronologies determined in part by U-Th or radiocarbon dating. The dating metadata (Table 8) provide a brief overview of information available in the related record publications and include either the corrected U-Th age or uncalibrated 14C age (± uncertainty) referenced to 1950 (BP; years before present) as well as a summary of related information and/or assumptions.
The complete record (or all available) of essential information for reporting U-Th and radiocarbon dating can be found in the original publications. Dating information published for the first time in this study is supplied in Table D1.
Table 9Analysis metadata for the CSV version of the GBRCD. Description of fields relating to the measurement of coral data, including variables measured, analysis method and the age model used. Note “X” is used to represent a list of variables as stated in the description.
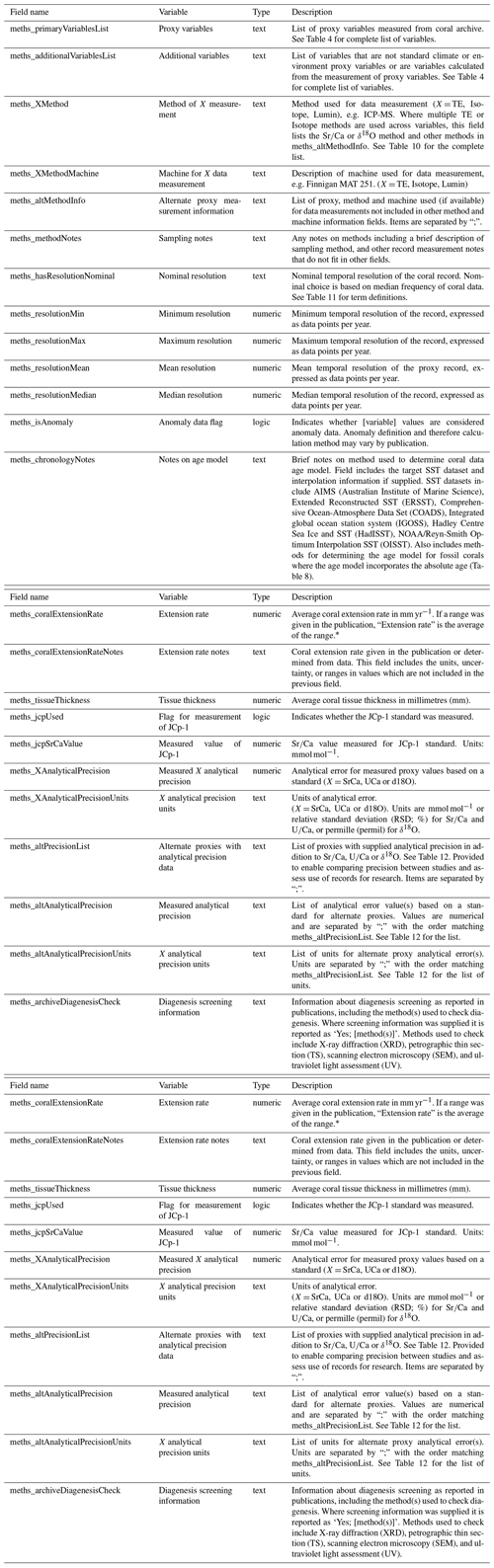
∗ Descriptions based on Walter et al. (2023) CoralHydro2k database fields.
Table 11Description of terms for nominal resolution.
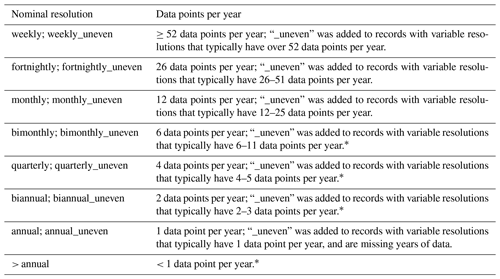
∗ Descriptions based on Walter et al. (2023) CoralHydro2k database fields.
Table 12List of isotopes, trace elements, and trace-element ratios, with analytical precision values supplied in meths_altPrecisionList. The list of variables is formatted as per meths_altPrecisionList. Units are as provided by the original publication(s) or authors but are all 1 standard deviation.

∗ Sr88Ca = as per D'Olivo and McCulloch (2022).
2.7.4 Analysis metadata
Analysis fields provide information about or obtained during measurements of coral variables (Table 9). They include the list of proxies measured, information about sampling, analytical methods (acronyms given in Table 10), data resolution (as contained in the database record; Table 11), and analytical precision of measurements (annotations given in Table 12). They also include information such as whether the international coral reference JCp-1 (Okai et al., 2002; Hathorne et al., 2013) was measured and information published about screening for diagenetic alteration of the corals.
The coral data resolution is described as the minimum, maximum, mean, and median number of data points per year for each record. The measurements per year were calculated for complete calendar years based on all unique age dates for each record, as records were filtered assuming duplicate dates represent replicate samples. A nominal data resolution label was assigned to each record based on the calculated median resolution described in Table 11 and is a key field for filtering records in the database. The term nominal resolution suffix “_uneven” has been adopted from the CoralHydro2k database (Walter et al., 2023) to describe corals with a variable resolution (i.e. where the record's min, max, mean, and median resolutions are not the same). A notes field (“meths_methodNotes”; Table 9) is also included for information on the measurement method(s), including the sampling method and any additional methodology notes, as well as an identifier (“REEs measured”) for every record where any of the REEs (as listed in Sect. 2.6 above) were included in the record.
Table 13Calibration metadata for the CSV version of the GBRCD. Available information on the calibration of coral records to environmental variables, specifically SST. Note “X” is used to represent a list of variables as stated in the description.
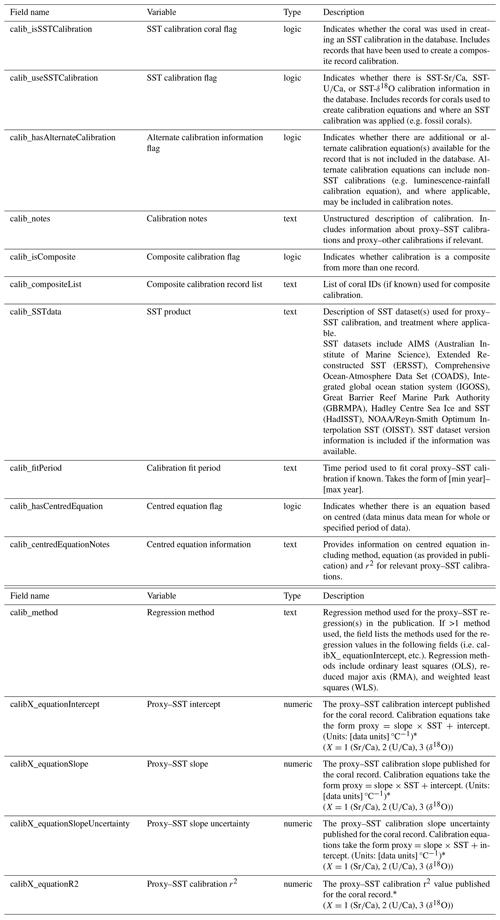
∗ Descriptions based on Walter et al. (2023) CoralHydro2k database fields.
2.7.5 Calibration metadata
Calibration metadata (Table 13) provide information about the creation of proxy–environment equations. Due to the ongoing focus and broader regional significance of proxy–SST calibrations, detailed information has been provided for proxy–SST calibrations (intercept, slope, slope uncertainty, and r2) for “raw” (non-centred) data in associated fields for , , and δ18O. Raw data are the data values after measurement processing, while centred (or anomaly) data are the raw data minus the data mean (which can be the mean of the whole record or part thereof). The calibration information for these SST proxies was included due to their historical use and the body of published research on their feasibility and/or application for reconstructing SSTs in the GBR. Where a centred calibration was preferred or the only one published, the calibration information is separate from the more frequently used non-centred equations. As there were additional proxy–environmental variable calibrations published for the database records, other calibration equations are provided in calibration notes where applicable. Availability of additional proxy–environment calibrations that are not provided in the database are also flagged.
2.7.6 Publication metadata
The GBRCD includes metadata for relevant publications relating to the coral records. The bibliographic information is supplied for up to three related publications with relevant bibliographic information separated into separate fields for ease of filtering and includes (where applicable) the DOI for the publication and the archived data (Table 14). Another field for an alternate data URL related to the coral record is also provided to enable referencing the original data location when it has been provided in article supplements or similar. If a record (or part thereof) was featured in multiple publications, the order of the bibliographic information was determined by the primary publication source of the data archived, and then the publications are generally in chronological order (oldest to newest) with preference for the most complete dataset or archive and method description for the data.
The GBRCD is a comprehensive compilation of coral records from the GBR to date that spans −5885 to 2017 CE. The database enables the investigation of multiple environmental factors via various coral proxy systems for the GBR, northeastern Australia, and potentially the broader Indo-Pacific region. Researchers can select from a list of 78 measured or calculated variables to investigate the GBR environment or determine new proxy–environment relationships.
The GBRCD is freely available on the NOAA/WDS Paleoclimatology website at https://doi.org/10.25921/hqxk-8h74 (Arzey et al., 2024).
It is up to the user to determine applicable proxies for their investigation and filter records as needed. Best practice (Fig. 2) should begin with filtering by proxy or proxies of interest. Next the CSV dataset(s) should be joined with the metadata fields to enable further filtering of the database. It is then suggested to subsequently filter the data by other metadata of interest such as record coverage, resolution(s), and archive species. Users should read the field “cdata_coralNotes” for all records of interest as this includes relevant information on caveats and assumptions (if any) that were noted for each record.
Sample code is available for R and Python.
As previously noted, care is advised for the use of all records in the GBRCD as relevance of the measured variables may vary by location or resolution and so forth. Records have been collated for the GBRCD and basic quality checks have been completed on the data to enable archiving for this study.
If researchers use the GBRCD, they should cite this study and the most recent version description of the GBRCD. It is also best practice to cite the original publications of the data (Table A1) alongside the GBRCD database. Citation information and reference links to the original publications and data archive are included in the metadata (i.e. Table 14).
4.1 Record coverage
The GBRCD compiles 208 individual records published since the early 1990s from 58 named reefs and islands (Fig. 1). There are 193 (92.8 %) records from Porites spp., 8 (3.8 %) from Isopora spp., 6 (2.9 %) from Acropora spp., and 1 (0.5 %) from a Cyphastrea sp. coral. The coral records include 78 variables, of which 72 are measured and 6 are calculated. The majority (116) of records are from the central GBR region (17 to 20° S), 74 records are from the southern GBR (20 to 24° S), and 18 records are from the northern GBR (11 to 17° S).
Most records (58.2 %) have a maximum (core-top) age between 2000 and 2017 CE, and 73.1 % have a maximum age between 1990–2017 CE. The peak density of records occurs in the 1980s with ≥105 records in each year between 1980–1984 and nearly as many (≥100) in the early 1990s (1990–1993) (Fig. 3). Only 59 (28.4 %) of the records have a minimum age that occurs before 1900 CE, and 18 of those records are fossil coral records that range from ∼2296 to 5885 BCE. Of these fossil records, 16 (88.9 %) are from the central GBR and 2 are from the southern GBR.
Of the 140 records measuring trace elements in the GBRCD, 49.3 % of records are a result of the development of laser ablation methods. There have been 69 LA-ICP-MS trace-element records published and 71 trace-element records analysed by other mass spectrometry methods. Many (54 records) of the non-LA-ICP-MS records are from studies that assess or use SST proxies, likely due to the expectation that trace-element records from laser ablation methods have a poorer fit with SST than TE records derived from solution methods. For example, the Wu et al. (2021b) LA-ICP-MS study found a notable poor periodicity and –SST relationship in two out of six records, which led them to suggest that the usefulness of LA-ICP-MS for –SST analysis is potentially limited by the relatively larger LA-ICP-MS analytical error than other mass spectrometry methods.
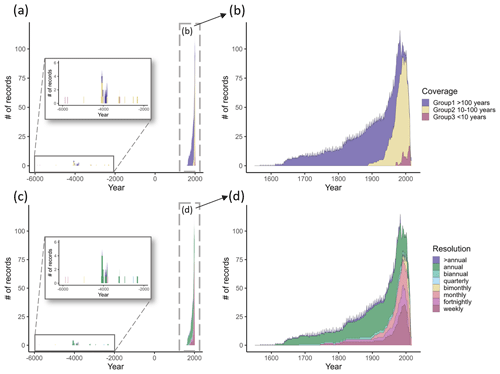
Figure 3Temporal coverage of records in the GBRCD colour coded by (a) record coverage Group 1–3 as per Table 6 (1: records >100 years; 2: records >10 to <100 years; 3: records <10 years), includes an inset that enlarges the period −6000 to −2000 CE, (b) subset of (a) showing only records since 1550 CE, (c) nominal resolution as per Table 11 (see figure legend) (records with “_uneven” nominal resolution were combined with even resolution records), includes an inset that enlarges the period −6000 to −2000 CE, and (d) subset of (c) showing only records since 1550 CE. The “spikiness” visible in (b) Group 1 and (d) > annual resolution records is due to the combination of biennial and quinquennial resolution records.
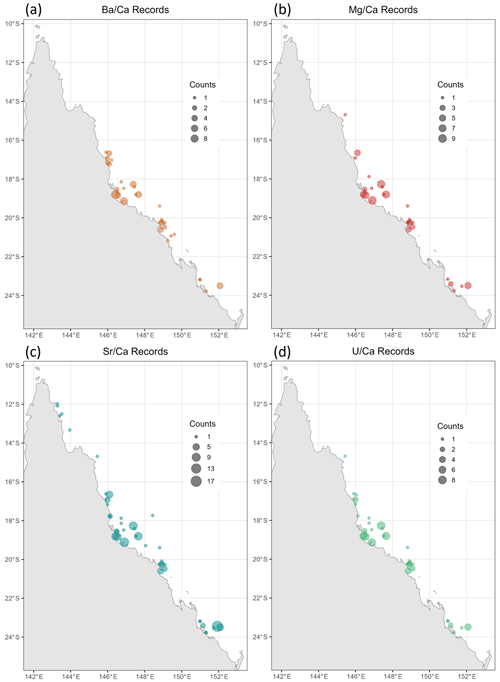
Figure 4Location map of GBRCD records for common major trace-element ratios: (a) , (b) , (c) , and (d) . The size of the point indicates the number of records, and it is specific to each map.
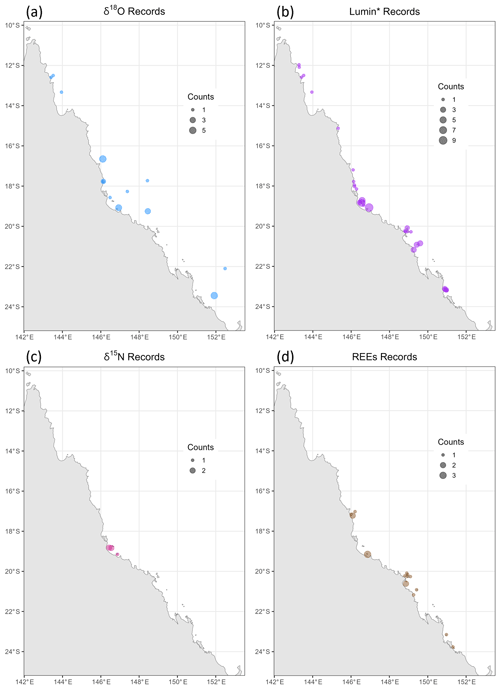
Figure 5Location map of GBRCD records for (a) δ18O, (b) luminescence (Lumin), (c) δ15N, and (d) rare earth elements (REEs). The size of the point indicates the number of records, and it is specific to each map. Note that Lumin* includes all the methods included in the GBRCD for measuring luminescence.
The majority of records in the database include measurements (118 of 208; Fig. 4), and 63 of these records are used in SST calibrations. The other variables measured most frequently include (59 records), (78 records; Fig. 4), (73 records; Fig. 4), (77 records; Fig. 4), and luminescence (57 records; Fig. 5). There are 51 isotope records, of which 27 include δ18O records (Fig. 5), 17 include δ13C, 15 include δ11B, 6 include δ14C, 5 include δ15N (Fig. 5), 1 includes δ66Zn, and 1 includes δ98Mo. Additionally, there are 18 records measuring REEs (Fig. 5). Note that this number excludes records that only measure yttrium and no REEs.
The number and spatial distribution of records (Fig. 4c) reflect the dual utility of the ratio as an SST proxy and as a method to determine the coral age model. Similarly, (Fig. 4d) is also used as an SST proxy and to determine coral age models. When combined with other proxies (e.g. ), may improve SST predictions (Wu et al., 2021b; DeCarlo et al., 2016). The proxy is commonly measured in addition to target proxies rather than being the focus of the study. However, has more recently been paired with δ11B in studies examining pH to determine the dissolved inorganic carbon in the calcifying fluid. has been measured relatively frequently (Fig. 4b), and while known as an SST proxy in other genera, the –SST relationship in Porites spp. is weak or results are highly variable (e.g. Sadler et al., 2014; Wu et al., 2021b), possibly due to the coral actively discriminating against Mg2+ during biomineralisation (Marchitto et al., 2018). (Fig. 4a) is frequently measured in the GBR, primarily to assess terrigenous inputs and river flow, and is mainly measured by LA-ICP-MS (58 of 78 records).
Despite the widespread analysis of coral δ18O across the tropical Pacific (∼100 δ18O-only and paired -δ18O records; Walter et al., 2023), there are few (only 27) δ18O records available for the GBR (Fig. 5a), especially considering the length of time δ18O has been used as an SST or hydrological proxy. This is potentially due to the paired forcing (SST and hydrology) that determines the coral δ18O for nearshore corals, as well as the development and use of coral luminescence for assessing freshwater inputs into the GBR. Records including luminescence measurements make up 27.4 % of the GBRCD (Fig. 5b).
There has been a push for coral records to include a range of details to meet minimum reporting standards for palaeo-archive records (such as MARPA (Dassié et al., 2017) and PaCTS (Khider et al., 2019)). These community-led publications report the ideal metadata that should be supplied with the publication of each record and provide a useful guide for best practice. The call for minimum reporting standards for data and metadata is echoed here. A particular challenge in producing the GBRCD was that metadata were often spread across multiple publications. To improve traceability between publications, relevant metadata could be included in the Supplement and in a publicly available data repository. The addition of IGSNs to capture the metadata associated with the physical samples would also improve the utility of the coral data (Dassié et al., 2017).
Another area for improvement is the reporting of screening corals for diagenesis. Diagenesis is a known source of error in geochemical analysis as it can remove the primary coral proxy signal (Hendy et al., 2007; Sayani et al., 2011; McGregor and Gagan, 2003; Nothdurft et al., 2007; Quinn and Taylor, 2006; Weerabaddana et al., 2024). Only 40 of 208 records in the GBRCD reported on diagenetic alteration. The majority fail to mention the screening method(s) or outcomes (see “meths_archiveDiagenesisCheck”) in the publication text or supplementary materials, or they could not be determined from cross-referencing sample IDs in other publications. There is no established standard of best practice for screening corals for diagenesis at present, though it is suggested that it is ideal to use petrographic analysis with a combination of methods, such as XRD and densitometry (McGregor and Abram, 2008). Other emerging approaches (e.g. Murphy et al., 2017; Takada et al., 2017) may also be useful for diagenesis screening. However, due to the strengths and weaknesses of the various methods as noted by Nothdurft and Webb (2009), examination by petrographic analysis (preferably using scanning electron microscopy) is required to confirm the type and level of diagenetic alteration (if any) present in a sample. Given the consequences of diagenesis for palaeoclimate and palaeoenvironment reconstructions from coral records, we strongly advocate for authors to report their diagenetic screening methods in their publications or supplements.
X-ray radiographs (X-radiographs hereafter) showing sampling paths should also be routinely published with the data, either as a figure in the paper or as supplementary material. Pioneering work on corals from the GBR showed that it is essential for corals to be sampled along the maximum growth axis. Providing this information was previously common (Alibert and McCulloch, 1997; Calvo et al., 2007; Pelejero et al., 2005; Chakraborty et al., 2000; DeLong et al., 2007), but x-radiographs have not been published consistently in recent years. Computed tomography (CT) scans of coral cores are sometimes used instead of, or in addition to, X-radiographs. Unlike X-radiographs, CT scans can be run on uncut coral cores and drill core matrices, and similar to X-radiographs, they can provide information about extension rates, density, and calcification (Bosscher, 1993; Mollica et al., 2018; Crook et al., 2013), as well as growth morphologies and bioerosion (Prouty et al., 2017; DeCarlo et al., 2015; Li et al., 2021). As with X-radiographs, the CT scan images providing relevant information should be included with the paper.
With the continued passage of time, it is likely there will be further loss of existing coral records, physical samples, and associated data. Due to physical misplacement or destruction of data files, inability to read old computer files, and/or retirement of researchers with existing records, the coral data underpinning early coral research may be lost forever. We urge researchers in possession of existing records to reach out to archival teams such as those sustaining existing databases (e.g. the GBRCD or the CoralHydro2k database; Walter et al., 2023) to ensure records are archived for future use. An email address for submissions to the GBRCD is provided at the GBRCD GitHub and NOAA/WDS Paleoclimatology study page to facilitate capture of legacy or new coral data.
The GBRCD lends itself to meeting some of the suggestions put forward by Lough (2004) to improve the use of corals for palaeoclimatology. While not absolute replication, the GBRCD facilitates using multiple records to determine common environmental signals and identify non-climatic artefacts in those records. For example, the database enables combining multiple records to assess trends, similar to methods used by Hendy et al. (2002).
The long and comprehensive history of coral research on the GBR can make it difficult to find relevant research and records to understand the current state of coral palaeoclimate and palaeoenvironment studies on the GBR and thus identify gaps in existing research. The GBRCD can be used to discern where future coral proxy records are needed. Additionally, the database includes 67 duplicate core names, which generally indicates different types of analyses on the same coral head and may provide an opportunity for comparisons between methods.
The GBRCD can be used for analysis and examination of trends at local to regional scales. Records from the GBR database can be selectively used alongside other datasets to assess the past environment of Australia and/or the Pacific and identify trends and periods of note. It enables comparisons of different periods, such as the modern corals and older Holocene coral records, making it possible to identify notable changes between the time periods. Furthermore, records from the GBRCD can be combined with new and existing models of the palaeoenvironment.
6.1 The GBRCD
This is stage 1 of the GBRCD. The database is archived on the NOAA/WDS Paleoclimatology website. To access the database, visit https://doi.org/10.25921/hqxk-8h74 (Arzey et al., 2024) and click on the “NOAA Study Page” link. Example scripts to help users access the database, in either format, are available on the NOAA/WDS Paleoclimatology study page.
Example scripts and database information are also available on the GBRCD GitHub page (https://github.com/arzeyak/GBR-Coral-Skeletal-Records-Database, last access: 7 August 2024; GBRCD, 2024).
6.2 Updates to the GBRCD
Future updates to the database are expected to occur on an annual basis. We welcome submission of historic GBR coral record data for inclusion in the GBRCD, including existing unpublished datasets, supporting FAIR data principles (Wilkinson et al., 2016). Submitted records should meet general criteria outlined above in “Selection criteria for inclusion in GBRCD” (Sect. 2.1). For researchers that want to submit records (published or unpublished) to the GBRCD, please check the GBRCD GitHub for details on how to submit your records via the email address that is available on the GBRCD GitHub and NOAA/WDS Paleoclimatology study page.
The GBRCD promotes efforts to ensure important research data are archived and that existing coral records are not lost to time. In conclusion, we note the following:
-
The GBRCD is a comprehensive database of coral records from the GBR produced and published since the early 1990s, as well as seven new records that are first published here. The database archives 208 coral records that are publicly available at NOAA/WDS Paleoclimatology.
-
Records have been standardised and converted to a machine-readable format that is easy to use to investigate the database and to select records of interest for analysis. With detailed metadata in the GBRCD, there are many avenues for investigating the past and present GBR environment.
-
There is scope in the future for improving the archiving of coral research datasets by building on the GBRCD and including the minimum recommended metadata information for traceability and use.
Table A1Reference data for publications cited in the GBRCD. Primary and secondary variables are the variable name as per Table 4. Citations in the “Record publication(s)” columns are listed in the order presented in the database (pub1; pub2; pub3). Citations in the “Additional core publication(s)” column are listed in alphanumerical order.

Note the additional publications referenced in the GBRCD:
– Chakraborty and Ramesh (1993, 1997) for CH93SLY01 and CH93SLY02;
– Davies and Hopley (1983) for MA00MYR02 and MA00MYR03;
– Kamber et al. (2005) for JU08KES01b and JU08RTI01b;
– Min et al. (1995) for HE02GBR01, LE07MAG01, and LE07NEL01.
Several of the coral records were measured in specific contexts, had additional processing to recreate the original published age model in the GBR database, or are published in the GBR database for the first time. This contextual and methodological information is summarised here and is included in the relevant note field(s) in the GBR database metadata table.
C1 Split datasets
In the GBRCD, four corals and their related records from three publications were given split IDs due to differences in the data, such as resolution and/or age model. The Alibert et al. (2003) (Davies-2; GBRCD ID: AL03DAV01_1 and AL03DAV01_2. PAN 98-2; AL03PAN01_1 from AL03PAN01_2, AL03PAN01_3, and AL03PAN01_4), Druffel and Griffin (1999) (Abraham-1; DR99ABR01_1 and DR99ABR01_2) and Wei et al. (2009) Arlington Reef (AREO 4; WE09ARL01_1 and WE09ARL01_2) coral records were split due to differences in the resolution of the data. AREO4 (WE09ARL01_1 and WE09ARL01_2) was split where the resolution changed from quinquennial (1800–1939) to annual (1940–2004) for all measured variables. The Abraham-1 (DR99ABR01_1 and DR99ABR01_2) coral record was previously archived separately (δ13C and δ18O is approximately annual, and the Δ14C is approximately biennial), and this was maintained in the database. Davies-2 (AL03DAV01_1 and AL03DAV01_2) and PAN 98-2 (AL03PAN01_1 from AL03PAN01_2, AL03PAN01_3 and AL03PAN01_4) records were split due to differences in resolutions arising from two methods of measuring trace elements (thermal ionisation mass spectrometer (TIMS) vs. laser ablation inductively coupled plasma mass spectrometer (LA-ICP-MS)). Additionally, the LA-ICP-MS measurements from Alibert et al. (2003) were not combined into a single record as there were three distinct age models for the data, and each of these was presented in the original publication.
C2 Additions, modifications, and PhD theses
The GBRCD includes 15 records from five publications where the age model was created for archiving in the GBRCD. As described in the main paper, a base level of information was required to archive each record in the GBRCD; notably, each dataset should have an age model with an age assigned to each data point. A brief description of the method(s) used to create the age model for these records, as well any change(s) in the record compared to the previously published version, is described below. Additionally, as PhD theses can be more difficult to access than published journal articles, a brief description of relevant information is supplied for six records from three PhD theses.
C2.1 Chakraborty (1993) – PhD thesis
SR coral records (track 1 and track 2) (CH93SLY01 and CH93SLY02) were collected from Stanley Reef in December 1986 (Chakraborty et al., 2000). The coral was prepared and analysed for stable isotopes along two tracks: track 1 close to the central growth axis and track 2 ∼20° off the axis, as described in Chakraborty et al. (2000). Methods were as described in Chakraborty and Ramesh (1993, 1997). The original coral age model was based on Comprehensive Ocean-Atmosphere Data Set (COADS) SST data; however, since COADS is missing months of data for the Stanley Reef region, the HadISST1.1 data (grid centred on 19.5° S, 148.5° E; Rayner et al., 2003) were used for archiving in the GBRCD. A new age model was created to obtain ages based on linear interpolation using QAnalySeries to align δ18O minima (maxima) with SST maxima (minima) for quarterly averaged HadISST data. The age model was based on available information; however, the records published in Chakraborty et al. (2000) have additional δ18O measurements not available to be archived in the GBRCD. There was no change in the SR track 1 age model, but for SR track 2, ∼ 2 years of data (summer 1978 to winter 1980) do not have sufficient data points to pair with the SST data, so these years were not included in the database.
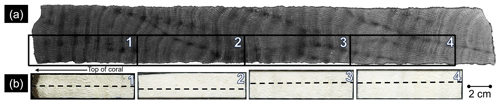
Figure C1PET12 (EL19OTI01) (a) X-radiograph (negative) of PET12 (EL19OTI01) coral. Black boxes indicate the four pieces analysed, and they are labelled with the piece number (1–4 in the top of the box). (b) Photos of PET12 pieces 1–4, and dashed black lines indicate the track analysed by LA-ICP-MS for each section of the record included in EL19OTI01. X-radiograph and photos supplied by Jennie Mallela.
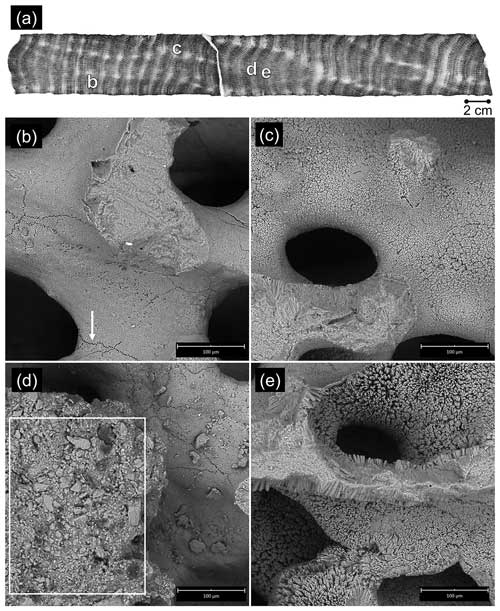
Figure C2PET12 (EL18OTI01) SEM images from alternate slice of PET12 coral. (a) Approximate locations of SEM images labelled (b)–(e) on coral X-radiograph. X-radiograph by PRP Diagnostic Imaging Wollongong. (b) to (e) Select SEM images from PET12 (EL19OTI01); (b) No secondary aragonite. Evidence of micro-borers (example noted by white arrow), (c) Low-level diagenetic alteration with nearly 100 % cover of secondary aragonite needles ≤5 µm and evidence of micro-borers, (d) Bioclastic cement plug (marked by white box) above relatively pristine coral surface with cement chips and evidence of micro-borers, (e) 90 % cover of secondary aragonite needles of 5–20 µm. Scale bar indicates 100 µm (b–e).
C2.2 Ellis et al. (2019)
PET12 (EL19OTI01; Ellis et al., 2019) was harvested by J. Mallela in May 2012 from Pete's Bay in the One Tree Island lagoon. The original PET12 chronology published in Ellis et al. (2019) was based on maxima and minima and was applied to three pieces of PET12 (PET12-1, 2 and 3) after data smoothing with a 10-point running mean and then interpolated to a monthly time series. LA-ICP-MS data from an additional piece of PET12 (PET12-4) was combined with the data from the other pieces (PET12-1, 2 and 3) (Fig. C1). To align the entire PET12 LA-ICP-MS dataset with the standard procedure used by other LA-ICP-MS datasets in the GBRCD, the original data smoothed with a 10-point running mean were further reduced with a 10-point mean. A new chronology was then assigned to the dataset using QAnalySeries to tie maxima (minima) with SST minima (maxima) using a weekly averaged NOAA Reyn_SmithOIv2 weekly SST dataset (Reynolds et al., 2002) centred on 23.5° S, 152.5° E. Scanning electron microscopy (SEM) analysis of an alternate slice of the PET12 coral by Ariella Arzey indicated variable and patchy diagenetic alteration with evidence of micro-borers and secondary aragonite crystals generally 0–10 µm in length but up to 20 µm in length observed in some sections (Fig. C2).
C2.3 Fallon (2000) – PhD thesis
Pan 1-98b (FA00PAN01) is a Porites sp. coral collected from Pandora Reef in October 1998, and it is an additional core from the same coral as Pan 1-98a (related record published in Fallon et al. (2003); FA03PAN01). Pan 1-98b was dead on top when collected and likely stopped recording environmental information in March/April 1998, as described in Fallon (2000). A 46 mm section from Pan 1-98b covering the period 1996–1998 was analysed by LA-ICP-MS as described in Fallon et al. (2003); the method used an ArF excimer laser (193 nm) that was masked to illuminate a 50 µm × 500 µm rectangle on the coral surface and a laser pulsed at 5 Hz using a 50 mJ power setting.
C2.4 Jupiter (2008)
KIA (JU08KES01b) and RTF (JU08RTI01b) are Porites spp. coral cores collected in 2004 from Keswick Island and Round Top Island, respectively, and were previously described in Jupiter (2006) (PhD thesis). Both corals' annual growth bands were bulk sampled, and 3–5 consecutive years per decade were analysed for rare earth elements and yttrium (REYs) for the period 1950–2002. Samples were analysed using a Thermo X-Series inductively coupled plasma mass spectrometer (ICP-MS) at the University of Queensland.
C2.5 Lewis (2005) – PhD thesis
MAG01D (LE05MAG01) is a modern Porites sp. coral collected from Geoffrey Bay, Magnetic Island, by the Australian Institute of Marine Science (AIMS) in 1987, while NEL01D (LE05NEL01) and NEL03D (LE05NEL02) are fossil Porites spp. corals collected from Nelly Bay Harbour, Magnetic Island, in 2001, and they were previously described in Lewis (2005). Top and bottom growth bands for NEL01D and NEL03D were U-Th dated at the ACQUIRE laboratory at the University of Queensland. Fragments (2 cm × 2 cm samples) of the bottom and top of MAG01D, NEL01D, and NEL03D were platinum-coated and examined for diagenesis using scanning electron microscopy, and additional thin sections were also examined with a Leica IM50 microscope. Analysis for diagenetic alteration of the corals noted no distinct differences between the modern and fossil corals. The corals were sampled by homogenising 5-yearly increments and were split into two batches. One batch was pre-treated with H2O2 and was analysed for , , , and Mn at the Queensland Health Scientific Services (QHSS) laboratory. The other batch was not pre-treated and was analysed for δ13C and δ18O with a Micromass Prism III stable isotope mass spectrometer at the University of Wollongong.
C2.6 Marshall (2000) – PhD thesis
Myr-2 (MA00MYR01) is a Porites sp. coral collected from Myrmidon Reef in July 1996 (Marshall and McCulloch, 2002). As the data were archived in the PhD thesis appendix without an age model, a new coral age model was created for archiving in the GBRCD. The new coral age model was based on published information from Marshall and McCulloch (2002) and Marshall (2000). The original data chronology and calibration was based on the Australian Institute of Marine Science (AIMS) weekly instrumental Myrmidon Reef SST data. However, the AIMS Myrmidon loggers' SST data only covered the period from 1988 to 1996, whereas the Myr-2 record extends back to 1973 and is at approximately monthly resolution (<20 measurements per year). A new chronology was assigned to the data using QAnalySeries to align minima (maxima) with SST maxima (minima) for HadISST grid square centred on 18.5° S, 147.5° E.
Myr2-16D (MA00MYR02) and Myr2-17D (MA00MYR03) are Porites sp. coral collected from the Myrmidon Hole 2 drill core matrix (Davies and Hopley, 1983; Marshall, 2000). Myr2-16D was analysed by Marshall (2000) for δ13C and δ18O at The Australian National University using a Finnigan MAT 251 mass spectrometer coupled with a Kiel device to assess the fluorescent banding in the coral and its potential link with precipitation/river flow. The δ18O data presented in Marshall (2000) have a correction of 0.011 ‰ per metre applied for ice volume that assumed sea level was 15–20 m below present-day sea level. The correction is not applied to the δ18O data archived in the GBRCD. Myr2-17D was analysed for B, Mg, Ca, Sr, Ba, and U by LA-ICP-MS using a 193 nm ArF excimer laser connected to a VG Elemental PlasmaQuad PQ2+ instrument with a 50 µm × 500 µm slit as described in Marshall (2000). TIMS U-series dates were available for both coral pieces; however, the data for both corals did not include an age model. An age model for Myr-2-16D and Myr2-17D was created for archiving using QAnalySeries to match δ18O or maxima with generalised monthly SST minima (minimum SST occurring in July).
The Myr2-17D U-Th calibrated age published in Marshall (2000) was 7880±60 years (ago). However, no date for the U-series analysis is supplied. Thus, to determine the age relative to the Common Era for archiving, it is assumed that it was dated in the same year as publication (i.e. 2000), thus transforming the Myr2-17D age to CE (i.e. 5881±60 BCE).
Myr2-16D was in three parts, and each section was previously U-Th dated separately, giving a calibrated age range from 7646±60 years (ago) for the basal section to 7968±100 years (ago) for the top section (with a middle section dated as 7846±30 years (ago)) (Marshall, 2000). Density band counting of an alternate slice suggests a maximum of 20 years between the top and bottom of the coral piece. The U-series dates are not within error and/or suggest an age reversal in the Myrmidon Hole 2 drill core when considering the date of the top (youngest) section of Myr2-16D is older than Myr2-17D. In 2022, a piece of the middle section (∼5–6 cm from the top of Myr2-16D) of the alternate slice was U-Th dated and used as the age of Myr2-16D for the GBRCD. The 2022 U-series date was measured at the Radiogenic Isotope Facility, University of Queensland, and gave a calibrated age of CE (i.e. 5755±13 BCE; Table D1).
St-1 is a Porites sp. coral collected from Stanley Reef in January 1999 (Marshall, 2000; Marshall and McCulloch, 2002). The age model was created based on published information from Marshall and McCulloch (2002) and Marshall (2000). A chronology was assigned to the data for archiving in the GBRCD using QAnalySeries to align minima (maxima) with SST maxima (minima) for fortnightly averaged AIMS in situ logger data from Davies Reef and Hardy Reef. The original publications only used the Davies Reef SST data, which due to missing data can only create an age model for the period of August 1996 to January 1999. The AIMS Hardy Reef SST data closely resembles the Davies Reef SST data, and both are approximately equidistant from Stanley Reef, making them a suitable dataset to estimate the regional SST seasonality. Using both SST datasets enables the St-1 age model to be extended to cover the period from February 1995 to January 1999.
C2.7 Razak et al. (2017)
Samples myra-30 (RA17MYR01) and myra-31 (RA17MYR02) are both Isopora palifera corals harvested from Myrmidon Reef in May 2013 (Razak et al., 2017). The age models were created for archiving in the GBRCD based on published information from Razak et al. (2017). A chronology was assigned using QAnalySeries to align minima (maxima) with Myrmidon SST maxima (minima) for fortnightly averaged SSTs from a composite of 10 AIMS in situ loggers. Outliers were removed from the records to match values reported in Razak et al. (2017). No outliers were removed from other measured trace-element ratios.
C2.8 Sadler et al. (2016b)
All modern and Holocene coral records from Heron Reef published in Sadler et al. (2016b) (SA16HER01b–SA16HER06b; modern: P HS 1, P HS 3, P HS 4, and P HS 7. Holocene: R9C, R15B3) were publicly archived with time series for all data points expressed as calendar year integers (i.e. all data from 2014 had an age listed as 2014). Therefore, to recreate the seasonal cycle (as presented in Sadler et al., 2016b), the chronology was assigned by tying minima (maxima) to bimonthly averaged SST maxima (minima) using QAnalySeries. The original coral chronologies and –SST calibration for the modern records were created using the Commonwealth Scientific and Industrial Research Organisation/Pacific Marine Environmental Laboratory Moored Autonomous pCO2 (MAPCO 2) project in the Wistari Channel (Sadler et al., 2016b), but this was not available for the GBRCD. For the GBRCD, modern SST data were averaged from AIMS in situ temperature loggers. The AIMS data loggers from the region have patchy temporal coverage, so the bimonthly SST dataset is an average of data logger data from Erskine Island (×1), Halftide Rocks (×1), Halfway Island (×2), Heron Reef (×16), Square Rocks (×2), and Tryon Island (×2). For the Holocene corals, the SST data used to reconstruct the age model were a generalised seasonal cycle based on the AIMS bimonthly averaged SST data for the southern GBR region (SST minimum occurs in July–August). Holocene corals were dated at the Radiogenic Isotope Facility, University of Queensland, as described in Sadler et al. (2016b). However, no measurement date for U-Th analysis was published. To determine the coral ages relative to the Common Era, the measurement date was determined using the Radiogenic Isotope Facility records (June 2015 date of chemistry; personal communication Tara Clark, March 2023).
C2.9 Xiao et al. (2020)
10AR2 (XI20ARL01) is a Porites sp. coral harvested from Arlington Reef in April 2010 (Deng et al., 2014). The age model was created for archiving in the GBRCD based on information published in Xiao et al. (2020). A chronology was assigned using QAnalySeries to align minima with the start of each year (i.e. January) to obtain a record with 10–16 data points per year.
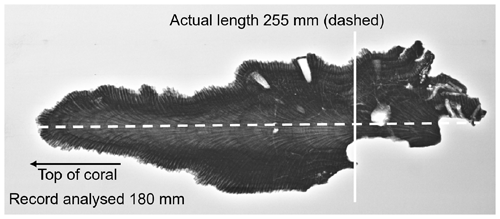
Figure C3X-radiograph (positive) of Dip-04 (AR24DIP01) coral. The dashed white line indicates the track analysed with white solid line the end of record section. X-radiograph supplied by Tries Razak.
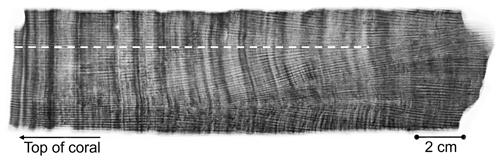
Figure C4X-radiograph (positive) of GUT12 (AR24OTI01) coral. The dashed white line indicates the track analysed by ICP-AES for the record included in AR24OTI01. X-radiographs by PRP Diagnostic Imaging Wollongong.
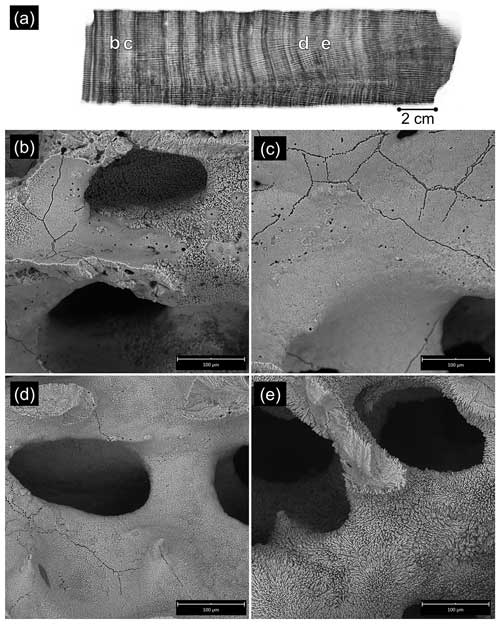
Figure C5GUT12 (AR24OTI01) SEM images (a) Approximate locations of SEM images labelled (b)–(e) on coral X-radiograph. (b–e) Select SEM images from GUT12; (b) Secondary aragonite needles ≤5 µm patchy cover of coral surface (∼50 %) and evidence of micro-borers, (c) No secondary aragonite needles. Evidence of micro-borers, (d) low-level diagenetic alteration with 100 % cover of secondary aragonite needles <5 µm, (e) low-level diagenetic alteration with 100 % cover of secondary aragonite needles of 5–15 µm. Scale bar indicates 100 µm (b–e).
C3 Unpublished records
C3.1 AR24DIP01
Dip-04 (AR24DIP01) is an Isopora palifera coral that was harvested from Dip Reef in January 1988, which was in an existing collection held at the Australian Institute of Marine Science (AIMS). The data were measured by Tries Razak but have not been published previously. As per the methods described in Razak et al. (2017), ∼500 µg of coral powder was milled at 1 mm increments with a 1–2 mm depth using a hand-held drill with a 1 mm drill bit (Fig. C3). Trace elements (, , , , and ) were measured using a Thermo X-Series II quadruple ICP-MS at the University of Queensland Radiogenic Isotope Facility following a modified method described in Nguyen et al. (2013). The age model for this study was created by aligning minima (maxima) with SST maxima (minima) using QAnalySeries. The seasonal cycle pattern was cross-checked with data. As no nearby in situ instrumental measurements of SST exist for the period included in the coral record, chronology was established using the HadISST dataset (grid square centred on 18.5° S, 147.5° E). No outliers were removed from the dataset.
C3.2 AR24OTI01
GUT12 (AR24OTI01) is a Porites sp. coral that was harvested in May 2012 by Jennie Mallela from the Gutter in the One Tree Island lagoon. The data have not been published previously. GUT12 was previously sliced into ∼7 mm thick slabs, and a section of GUT12 ∼15 cm in length was selected for trace-element analysis by Ariella Arzey (Fig. C4). This section was measured using the AIMS densitometer and examined for diagenetic alteration using a Phenom XL benchtop SEM at the University of Wollongong by Ariella Arzey. Micro-borer holes and secondary aragonite crystals were present along most of the analysis track with patches of trace amounts of secondary aragonite crystal (<2 µm) increasing along the measurement track until 100 % of skeleton was covered in secondary aragonite crystals generally between 0–10 µm length (Fig. C5). Overall, diagenetic alteration is at a low level, and the coral skeleton preservation is fair to good. Coral powder was drilled continuously from a ledge at 0.3–0.6 mm increments using a 2 mm TiN-coated end mill to give a resolution of ∼17–34 (median 24) samples per year. and ratios were measured by inductively coupled atomic emission spectrometry (ICP-AES) at the Australian Nuclear Science and Technology Organisation (ANSTO) with a Thermo Scientific iCAP 7600 series ICP-AES. Data were standardised using measured JCp-1. The coral age model was created using QAnalySeries to align minima (maxima) with SST maxima (minima) using the fortnightly averaged NOAA Reyn_SmithOIv2 weekly SST dataset, grid centred on 23.5° S, 152.5° E.
C3.3 AR24OTI02 and AR24OTI03
OTI 1-3 (AR24OTI02) and OTI 3-1 (AR24OTI03) are Porites spp. corals that were harvested in 2004 from the One Tree Island lagoon by Guy Marion. The corals were part of the Enrichment of Nutrients Coral Reef Experiment (ENCORE) (Koop et al., 2001; Steven and Atkinson, 2003) and were transplanted from the reef slope onto the reef flat on 17 January 1995 and stained with Alizarin Red S on 23 November 1995. OTI 1-3 (Fig. C6) was collected from the reference treatment patch reef at ambient levels of nutrients, while OTI 3-1 (Fig. C7) was from the enriched nitrogen treatment patch reef. Both corals were measured by LA-ICP-MS as per methods described in Lewis et al. (2012); corals were analysed using a Varian 820 ICP-MS, with output data smoothed with a 10-point running mean followed by a 10-point mean. The original age model supplied by Stephen Lewis (where chronology assigned with minima (maxima) matched to 8 February (8 August)) was adjusted to improve correlation with SST by fitting data to match the annual maxima and minima of a fortnightly averaged NOAA Reyn_SmithOIv2 weekly SST dataset centred on 23.5° S, 152.5° E grid. Each piece was examined for diagenetic alteration by Ariella Arzey, although due to the absence of information for both pieces the original LA-ICP-MS track cannot be directly matched to the area examined by the SEM. OTI 1-3 and OTI 3-1 show highly variable diagenetic alteration (Figs. C8 and C9). Both corals show above-average levels of diagenetic alteration compared to other corals examined, which may be due to their handling for or the altered nutrient exposure from the ENCORE experiment. There is scope for future research to determine the cause of the abnormal level of diagenetic alteration. Caution should be exercised if using OTI 1-3 and OTI 3-1 for climate assessment, due to the high levels of diagenesis and the corals' use in the ENCORE experiments.
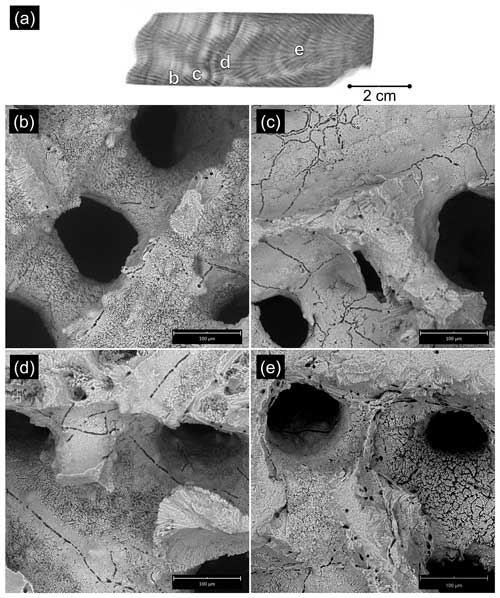
Figure C8OTI 1-3 (AR24OTI02) SEM images. (a) Approximate locations of SEM images labelled (b)–(e) on coral X-radiograph. (b–e) Select SEM images from OTI 1-3; (b) low-level diagenetic alteration with 100 % cover of secondary aragonite needles of 5–15 µm and evidence of micro-borers, (c) No secondary aragonite needles, with evidence of dissolution and micro-borers, (d) Evidence of micro-borers and possibly dissolution. Secondary aragonite needles and calcite crystals (0–10 µm), (e) 90 % surface covered by secondary aragonite needles, left side of coral needles are ≤5 µm and right side of coral needles are ∼5–15 µm. Evidence of micro-borers visible. Scale bar indicates 100 µm (b–e).
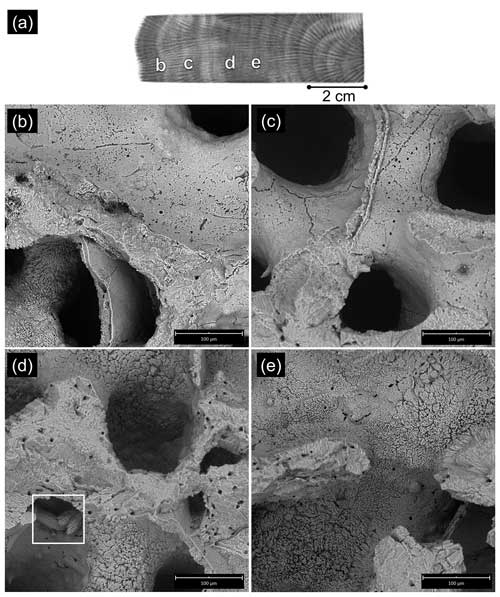
Figure C9OTI 3-1 (AR24OTI03) SEM images. (a) Approximate locations of SEM images labelled (b)–(e) on coral X-ray. (b–e) Select SEM images from OTI 3-1; (b) Secondary aragonite needles (0–10 µm) in lower left of image and possible low Mg-calcite cement on coral surface in upper right of image. Evidence of micro-borers, (c) No secondary aragonite. Evidence of micro-borers and possible minor dissolution of coral surface, (d) 100 % cover with secondary aragonite needles (0–15 µm) with evidence of micro-borers. High Mg-calcite splays occur in pore space (marked by white box), (e) 100 % cover with secondary aragonite needles (∼5–15 µm) with evidence of micro-borers. Scale bar indicates 100 µm (b–e).
C3.4 AR24OTI04 and AR24OTI05
OTI4_6C (OTI-4-19; AR24OTI04) and OTI4_6D (OTI-4-22; AR24OTI05) were collected from the One Tree Island hole 4 reef core matrix (Davies and Hopley, 1983) at a depth listed as between 5.72–7.47 m (OTI Hole 4 section 6) and estimated to be 6.2 and 6.5 m depth down core, respectively. Both corals were measured by LA-ICP-MS as per methods described in Lewis et al. (2012) and as per AR24OTI02 and AR24OTI03 above. An age model was created by matching the maxima with SST minima of a generalised SST time series (minimum SST occurs in August). The age model was further refined by adjusting for best fit of maxima to SST minima. An off-cut of AR24OTI04 (Fig. C10) and alternate slice of AR24OTI05 were dated in July 2022 at the Radiogenic Isotope Facility, University of Queensland, to give an age of CE and CE, respectively (Table D1). An alternate slice of AR24OTI04 was also dated in 2022, but the age used for AR24OTI04 is based on the off-cut of the slice that was measured with LA-ICP-MS as the relationship with the section dated for the alternate slice is not certain. SEM screening of the AR24OTI04 coral off-cut indicated 100 % cover of coral surface with secondary aragonite needles generally between 0–10 µm, although small areas with crystals up to 20 µm were observed (Fig. C11).
C3.5 AR24SLY01
The SR coral (AR24SLY01) was measured for radiocarbon by Supriyo Chakraborty and Ravi Bhushan in 1991 at the Physical Research Laboratory in Ahmedabad, India. Annual bands from the SR coral were analysed as per methods described in Chakraborty (1993) for other coral samples. Briefly, the annual samples were cut from the slice using a <1 mm thickness small diamond wheel operated by an electric hand drill. The cut bands were dried by heating overnight at 80–90 °C, and 15 g of powder was used for the radiocarbon analysis. β counting was carried out using a Packard Tri-Carb liquid scintillator analyser in low-level count mode. The coral X-ray was published in Chakraborty et al. (2000).
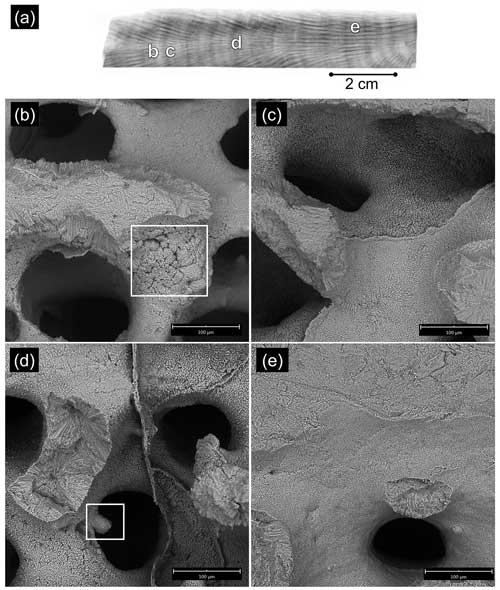
Figure C11OTI4_6C (OTI-4-19; AR24OTI04) SEM images. (a) Approximate locations of SEM images labelled (b)–(e) on coral X-ray. (b–e) Select SEM images from OTI4_6C (AR24OTI04); (b) 100 % cover of secondary aragonite needles generally <10 µm, but section with uneven surface (marked by white box) indicates ∼10–20 µm crystals, (c) 100 % cover of secondary aragonite needles of <10 µm, (d) 100 % cover of secondary aragonite needles of 5–10 µm. Includes secondary aragonite covering likely fungal growth or part of borer tube that occurred when coral was alive (marked by white box), (e) 80 % cover of trace (<5 µm) crystals of secondary aragonite. Scale bar indicates 100 µm (b–e).
Table D1Uranium–thorium age data obtained using a Nu Plasma II multicollector inductively coupled plasma mass spectrometer of fossil corals from the Great Barrier Reef, Australia. Note that AA47_OTI-4-19_4_Th and AA_OTI4_6C_1 samples are alternate slices of the same coral piece linked to the GBRCD record AR24OTI04.

∗ (D2)
Values in parentheses are activity ratios calculated from atomic ratios using decay constants of Cheng et al. (2000). All values have been corrected for laboratory procedural blanks. All errors reported as 2σ. Uncorrected 230Th age (ka) was calculated using the Isoplot/Ex 3.75 software (Ludwig, 2003), where ka denotes thousand years since the date of chemistry.
To account for non-radiogenic or initial 230Th0, two correction factors were applied:
- (a)
A bulk-Earth correction assuming a non-radiogenic 230Th/232Th0 activity value = 0.825+/−50%, with 238U, 234U, 232Th, and 230Th in secular equilibrium.
- (b)
A two-component mixing correction value determined using the equation of Clark et al. (2014) that accounts for both detrital and hydrogenous shown in Eq. (D1):
where 232Thdead is the measured 232Th value (ppb) in the individual dead coral sample. 232Thlive is 0.77 ppb, being the mean 232Th value of 43 live Porites coral samples from the inshore Great Barrier Reef with a corresponding activity ratio of 1.066±0.063 (20 %) (Clark et al., 2012). activity ratio representative of the insoluble Th component incorporated post-mortem or as particulates during growth is assumed to be 0.61±0.12 (20 %). This value is determined from the average y intercept values of five versus isochrons obtained from dead Porites corals sampled from the Palm Islands region (Clark et al., 2014).
The values in Table D1 are those determined after calculating the two-component correction. The “Bulk-Earth corr. age (ka)” is supplied to indicate the difference in age calculated between the two corrections (∼4–6 years).
AIMS loggers: derived data based on Australian Institute of Marine Science data (https://data.aims.gov.au/aimsrtds/datatool.xhtml, last access: 28 March 2021).
Hadley Centre Sea Ice and Sea Surface Temperature (HadISST) (Rayner et al., 2003; https://coastwatch.pfeg.noaa.gov/erddap/griddap/erdHadISST.html, last access: 29 February 2024).
NOAA REYN_SmithOIv2 (Reynolds et al., 2002; http://iridl.ldeo.columbia.edu/SOURCES/.NOAA/.NCEP/.EMC/.CMB/.GLOBAL/.Reyn_SmithOIv2/, last access: 27 February 2023).
Reefs and shoals – Queensland: Queensland Department of Resources (© State of Queensland (Department of Resources) 2023 (http://qldspatial.information.qld.gov.au/catalogue/custom/search.page?q=%22Reefs and shoals - Queensland%22, last access: 20 September 2023).
Drainage basins – Queensland (© State of Queensland (Department of Resources) 2023 (http://qldspatial.information.qld.gov.au/catalogue/custom/search.page?q=%22Drainage basins - Queensland%22, last access: 4 August 2023).
Major watercourse lines – Queensland (© State of Queensland (Department of Resources) 2023 (http://qldspatial.information.qld.gov.au/catalogue/custom/search.page?q=%22Major watercourse lines - Queensland%22, last access: 11 September 2023).
Populated places – Queensland (© State of Queensland (Department of Resources) 2023 (http://qldspatial.information.qld.gov.au/catalogue/custom/search.page?q=%22Populated%20places%20-%20Queensland%22, last access: 4 August 2023).
Mainland – Queensland (© State of Queensland (Department of Resources) 2023 (https://qldspatial.information.qld.gov.au/catalogue/custom/search.page?q=%22Mainland - Queensland%22, last access: 20 September 2023).
AKA and HVM conceived the study and designed the database. AKA built and curated the database, and database testing was conducted by AKA and HWF. TRC contributed data analysis and advice for U-Th dating. AKA, SEL, JM, JMW, SC, and TBR provided coral data and associated technical information. NPM facilitated converting the database to LiPD format, and AKA and HWF provided example scripts for using the database in R and Python. AKA and HVM wrote the manuscript with input from TRC, JMW, SEL, JM, NPM, HWF, SC, TBR, and MJF.
The contact author has declared that none of the authors has any competing interests.
Publisher's note: Copernicus Publications remains neutral with regard to jurisdictional claims made in the text, published maps, institutional affiliations, or any other geographical representation in this paper. While Copernicus Publications makes every effort to include appropriate place names, the final responsibility lies with the authors.
We would like to thank all the researchers whose publicly archived data or whose data were archived in supplementary material were included in the GBRCD, as well as the researchers who supplied us with their data (Appendix A).
We are thankful for the software and data sources that made this project possible. Sea surface temperature data (HadISST1.1) were provided by the Met Office and accessed from https://coastwatch.pfeg.noaa.gov/erddap/info/erdHadISST/index.html (last access: 29 April 2024).
Figure 1 was generated using QGIS 3.22.11 (https://www.qgis.org, last access: 8 January 2024), with GIS layers sourced from the State of Queensland (Department of Resources), found at http://qldspatial.information.qld.gov.au/catalogue/ (last access: 12 February 2024). The full list of GIS layers is available in Appendix E.
Figures 3–5 were generated using R 4.3.1 (https://www.R-project.org/, last access: 29 February 2024) with the ggplot2 3.4.3, sf 1.0-14, and ozmaps 0.4.5 R packages found at https://cran.r-project.org/web/packages/ggplot2/index.html (last access: 7 January 2024), https://cran.r-project.org/web/packages/sf/index.html (last access: 18 December 2023), and https://cran.r-project.org/web/packages/ozmaps/index.html (last access: 18 December 2023).
We would also like to thank the NOAA National Centers for Environmental Information, especially Edward Gille, and LiPD teams for facilitating archiving and distribution of the GBRCD.
Jennie Mallela's fieldwork was undertaken with the permission of the Great Barrier Reef Marine Park Authority (GBRMPA permit number G12.35021.1) and One Tree Island Research Station.
This research was funded by an Australian Government Research Training Program scholarship. This research was supported by an AINSE Ltd. Post Graduate Research Award (PGRA) (Ariella K. Arzey). This research was funded by an Australian Research Council (ARC) Discovery Project (DP200100206) to Helen V. McGregor, Jody M. Webster, and Tara R. Clark. This work was supported by ARC Special Research Initiative in Excellence in Antarctic Science (SRIEAS) (SR200100005), Securing Antarctica's Environmental Future, and ARC Future Fellowship (FT140100286) to Helen V. McGregor. Tara R. Clark and Jennie Mallela were funded by ARC Discovery Early Career Researcher Awards (DECRA) (DE180100017 and DE120101998, respectively).
This paper was edited by Sebastiaan van de Velde and reviewed by Niels de Winter and one anonymous referee.
Alibert, C. and McCulloch, M. T.: Strontium/calcium ratios in modern Porites corals From the Great Barrier Reef as a proxy for sea surface temperature: Calibration of the thermometer and monitoring of ENSO, Paleoceanography, 12, 345–363, https://doi.org/10.1029/97PA00318, 1997.
Alibert, C., Kinsley, L., Fallon, S. J., McCulloch, M. T., Berkelmans, R., and McAllister, F.: Source of trace element variability in Great Barrier Reef corals affected by the Burdekin flood plumes, Geochim. Cosmochim. Ac., 67, 231–246, https://doi.org/10.1016/S0016-7037(02)01055-4, 2003.
Arzey, A. K., McGregor, H. V., Clark, T. R., Webster, J. M., Lewis, S. E., Mallela, J., McKay, N. P., Fahey, H. W., Chakraborty, S., Razak, T. B., and Fischer, M. J.: The Great Barrier Reef Coral Skeletal Records Database (2024), NOAA National Centers for Environmental Information [data set], https://doi.org/10.25921/hqxk-8h74, 2024.
Australian Institute of Marine Science: Long-term Reef Monitoring Program – Annual Summary Report on coral reef condition for 2016/17, https://www.aims.gov.au/reef-monitoring/gbr-condition-summary-2016-2017 (last access: 5 August 2023), 2017.
Australian Institute of Marine Science: Long-Term Monitoring Program Annual Summary Report of Coral Reef Condition 2021/22, https://www.aims.gov.au/monitoring-great-barrier-reef/gbr-condition-summary-2021-22 (last access: 16 August 2023), 2022.
Barnes, D. J. and Lough, J. M.: Systematic variations in the depth of skeleton occupied by coral tissue in massive colonies of Porites from the Great barrier reef, J. Exp. Mar. Biol. Ecol., 159, 113–128, https://doi.org/10.1016/0022-0981(92)90261-8, 1992.
Barnes, D. J., Taylor, R. B., and Lough, J. M.: Measurement of luminescence in coral skeletons, J. Exp. Mar. Biol. Ecol., 295, 91–106, https://doi.org/10.1016/s0022-0981(03)00274-0, 2003.
Beck, J. W., Edwards, R. L., Ito, E., Taylor, F. W., Recy, J., Rougerie, F., Joannot, P., and Henin, C.: Sea-Surface Temperature from Coral Skeletal Strontium/Calcium Ratios, Science, 257, 644–647, https://doi.org/10.1126/science.257.5070.644, 1992.
Bosscher, H.: Computerized tomography and skeletal density of coral skeletons, Coral Reefs, 12, 97–103, https://doi.org/10.1007/BF00302109, 1993.
Boto, K. and Isdale, P.: Fluorescent bands in massive corals result from terrestrial fulvic acid inputs to nearshore zone, Nature, 315, 396–397, https://doi.org/10.1038/315396a0, 1985.
Brenner, L. D., Linsley, B. K., and Potts, D. C.: A modern -ä18O-sea surface temperature calibration for Isopora corals on the Great Barrier Reef, Paleoceanography, 32, 182–194, https://doi.org/10.1002/2016pa002973, 2017.
Calvo, E., Marshall, J. F., Pelejero, C., McCulloch, M. T., Gagan, M. K., and Lough, J. M.: Interdecadal climate variability in the Coral Sea since 1708 A.D, Palaeogeogr. Palaeoclimatol., 248, 190–201, https://doi.org/10.1016/j.palaeo.2006.12.003, 2007.
Cantin, N. E. and Lough, J. M.: Surviving Coral Bleaching Events: Porites Growth Anomalies on the Great Barrier Reef, PLOS ONE, 9, e88720, https://doi.org/10.1371/journal.pone.0088720, 2014.
Cantin, N. E., Fallon, S. J., Wu, Y., and Lough, J. M.: Project ISP019: Calcification and geochemical signatures of industrial development of the Gladstone Harbour from century old coral skeletons, Australian Institute of Marine Science, Townsville, Qld, 40, 2018.
Chakraborty, S.: Environmental significance of isotopic and trace elemental variations in banded corals, PhD Thesis (unpublished), The Maharaja Sayajirao University of Baroda, Vadodara, India, 119 pp., 1993.
Chakraborty, S. and Ramesh, R.: Monsoon-induced sea surface temperature changes recorded in Indian corals, Terra Nova, 5, 545–551, https://doi.org/10.1111/j.1365-3121.1993.tb00303.x, 1993.
Chakraborty, S. and Ramesh, R.: Environmental significance of carbon and oxygen isotope ratios of banded corals from Lakshadweep, India, Quatern. Int., 37, 55–65, https://doi.org/10.1016/1040-6182(96)00028-6, 1997.
Chakraborty, S., Ramesh, R., and Lough, J. M.: Effect of intraband variability on stable isotope and density time series obtained from banded corals, J. Earth Syst. Sci., 109, 145–151, https://doi.org/10.1007/BF02719158, 2000.
Chen, X., Deng, W., Kang, H., Zeng, T., Zhang, L., Zhao, J.-X., and Wei, G.: A Replication Study on Coral ä11B and and Their Variation in Modern and Fossil Porites: Implications for Coral Calcifying Fluid Chemistry and Seawater pH Changes Over the Last Millennium, Paleoceanogr. Paleocl., 36, e2021PA004319, https://doi.org/10.1029/2021PA004319, 2021.
Cheng, H., Edwards, R. L., Hoff, J., Gallup, C. D., Richards, D. A., and Asmerom, Y.: The half-lives of uranium-234 and thorium-230, Chem. Geol., 169, 17–33, https://doi.org/10.1016/S0009-2541(99)00157-6, 2000.
Clark, T. R., Zhao, J.-X., Feng, Y.-X., Done, T. J., Jupiter, S., Lough, J., and Pandolfi, J. M.: Spatial variability of initial in modern Porites from the inshore region of the Great Barrier Reef, Geochim. Cosmochim. Ac., 78, 99–118, https://doi.org/10.1016/j.gca.2011.11.032, 2012.
Clark, T. R., Roff, G., Zhao, J.-X., Feng, Y.-x., Done, T. J., and Pandolfi, J. M.: Testing the precision and accuracy of the U–Th chronometer for dating coral mortality events in the last 100 years, Quat. Geochronol., 23, 35–45, https://doi.org/10.1016/j.quageo.2014.05.002, 2014.
Clark, T. R., Roff, G., Zhao, J. X., Feng, Y. X., Done, T. J., McCook, L. J., and Pandolfi, J. M.: U-Th dating reveals regional-scale decline of branching Acropora corals on the Great Barrier Reef over the past century, P. Natl. Acad. Sci. USA, 114, 10350–10355, https://doi.org/10.1073/pnas.1705351114, 2017.
Crook, E. D., Cohen, A. L., Rebolledo-Vieyra, M., Hernandez, L., and Paytan, A.: Reduced calcification and lack of acclimatization by coral colonies growing in areas of persistent natural acidification, P. Natl. Acad. Sci. USA, 110, 11044–11049, https://doi.org/10.1073/pnas.1301589110, 2013.
Dassié, E., DeLong, K. L., Kilbourne, K. H., Williams, B., Abram, N. J., Brenner, L. D., Brahmi, C., Cobb, K. M., Corrège, T., Dissard, D., Emile-Geay, J., Evangelista, H., Evans, M. N., Farmer, J., Felis, T., Gagan, M., Gillikin, D. P., Goodkin, N. F., Khodri, M., Lavagnino, A. C., LaVigne, M., Lazareth, C. E., Linsley, B., Lough, J., McGregor, H., Nurhati, I. S., Ouellette, G., Perrin, L., Raymo, M., Rosenheim, B., Sandstrom, M., Schöne, B. R., Sifeddine, A., Stevenson, S., Thompson, D. M., Waite, A., Wanamaker, A., and Wu, H.: Saving our marine archives, Eos, 98, 32–36, https://doi.org/10.1029/2017EO068159, 2017.
Davies, P. J. and Hopley, D.: Growth fabrics and growth-rates of Holocene reefs in the Great Barrier-Reef, BMR J. Aust. Geol. Geop., 8, 237–251, 1983.
Davies, P. J., Marshall, J. F., and Hopley, D.: Relationships between reef growth and sea level in the Great Barrier Reef., Proceedings of the Fifth International Coral Reef Congress, 27 May–1 June 1985, Tahiti, 95–103, 1985.
De'ath, G., Lough, J. M., and Fabricius, K. E.: Declining Coral Calcification on the Great Barrier Reef, Science, 323, 116–119, https://doi.org/10.1126/science.1165283, 2009.
De'ath, G., Fabricius, K. E., Sweatman, H., and Puotinen, M.: The 27-year decline of coral cover on the Great Barrier Reef and its causes, P. Natl. Acad. Sci. USA, 109, 17995–17999, https://doi.org/10.1073/pnas.1208909109, 2012.
DeCarlo, T. M., Cohen, A. L., Barkley, H. C., Cobban, Q., Young, C., Shamberger, K. E., Brainard, R. E., and Golbuu, Y.: Coral macrobioerosion is accelerated by ocean acidification and nutrients, Geology, 43, 7–10, https://doi.org/10.1130/g36147.1, 2015.
DeCarlo, T. M., Gaetani, G. A., Cohen, A. L., Foster, G. L., Alpert, A. E., and Stewart, J. A.: Coral Sr-U thermometry, Paleoceanography, 31, 626–638, https://doi.org/10.1002/2015PA002908, 2016.
Dechnik, B., Webster, J. M., Davies, P. J., Braga, J.-C., and Reimer, P. J.: Holocene “turn-on” and evolution of the Southern Great Barrier Reef: Revisiting reef cores from the Capricorn Bunker Group, Mar. Geol., 363, 174–190, https://doi.org/10.1016/j.margeo.2015.02.014, 2015.
Dechnik, B., Webster, J. M., Webb, G. E., Nothdurft, L., and Zhao, J.-X.: Successive phases of Holocene reef flat development: Evidence from the mid- to outer Great Barrier Reef, Palaeogeogr. Palaeocl., 466, 221–230, https://doi.org/10.1016/j.palaeo.2016.11.030, 2017.
DeLong, K. L., Quinn, T. M., and Taylor, F. W.: Reconstructing twentieth-century sea surface temperature variability in the southwest Pacific: A replication study using multiple coral records from New Caledonia, Paleoceanography, 22, PA4212, https://doi.org/10.1029/2007PA001444, 2007.
Deng, W., Wei, G., McCulloch, M., Xie, L., Liu, Y., and Zeng, T.: Evaluation of annual resolution coral geochemical records as climate proxies in the Great Barrier Reef of Australia, Coral Reefs, 33, 965–977, https://doi.org/10.1007/s00338-014-1203-9, 2014.
D'Olivo, J. P. and McCulloch, M. T.: Response of coral calcification and calcifying fluid composition to thermally induced bleaching stress, Sci. Rep.-UK, 7, 2207, https://doi.org/10.1038/s41598-017-02306-x, 2017.
D'Olivo, J. P. and McCulloch, M.: Impact of European settlement and land use changes on Great Barrier Reef river catchments reconstructed from long-term coral records, Sci. Total Environ., 830, 154461, https://doi.org/10.1016/j.scitotenv.2022.154461, 2022.
D'Olivo, J. P., McCulloch, M. T., and Judd, K.: Long-term records of coral calcification across the central Great Barrier Reef: assessing the impacts of river runoff and climate change, Coral Reefs, 32, 999–1012, https://doi.org/10.1007/s00338-013-1071-8, 2013.
D'Olivo, J. P., McCulloch, M. T., Eggins, S. M., and Trotter, J.: Coral records of reef-water pH across the central Great Barrier Reef, Australia: assessing the influence of river runoff on inshore reefs, Biogeosciences, 12, 1223–1236, https://doi.org/10.5194/bg-12-1223-2015, 2015.
D'Olivo, J. P., Sinclair, D. J., Rankenburg, K., and McCulloch, M. T.: A universal multi-trace element calibration for reconstructing sea surface temperatures from long-lived Porites corals: Removing “vital-effects”, Geochim. Cosmochim. Ac., 239, 109–135, https://doi.org/10.1016/j.gca.2018.07.035, 2018.
Druffel, E. R. M. and Griffin, S.: Large variations of surface ocean radiocarbon: Evidence of circulation changes in the southwestern Pacific, J. Geophys. Res.-Oceans, 98, 20249–20259, https://doi.org/10.1029/93JC02113, 1993.
Druffel, E. R. M. and Griffin, S.: Regional Variability of Surface Ocean Radiocarbon from Southern Great Barrier Reef Corals, Radiocarbon, 37, 517–524, https://doi.org/10.1017/S0033822200031003, 1995.
Druffel, E. R. M. and Griffin, S.: Variability of surface ocean radiocarbon and stable isotopes in the southwestern Pacific, J. Geophys. Res.-Oceans, 104, 23607–23613, https://doi.org/10.1029/1999JC900212, 1999.
Dutton, A., Rubin, K., McLean, N., Bowring, J., Bard, E., Edwards, R. L., Henderson, G. M., Reid, M. R., Richards, D. A., Sims, K. W. W., Walker, J. D., and Yokoyama, Y.: Data reporting standards for publication of U-series data for geochronology and timescale assessment in the earth sciences, Quat. Geochronol., 39, 142–149, https://doi.org/10.1016/j.quageo.2017.03.001, 2017.
Ellis, B., Grant, K., Mallela, J., and Abram, N.: Is XRF core scanning a viable method for coral palaeoclimate temperature reconstructions?, Quatern. Int,, 514, 97–107, https://doi.org/10.1016/j.quaint.2018.11.044, 2019.
Erler, D. V., Wang, X. T., Sigman, D. M., Scheffers, S. R., Martínez-García, A., and Haug, G. H.: Nitrogen isotopic composition of organic matter from a 168 year-old coral skeleton: Implications for coastal nutrient cycling in the Great Barrier Reef Lagoon, Earth Planet. Sc. Lett., 434, 161–170, https://doi.org/10.1016/j.epsl.2015.11.023, 2016.
Erler, D. V., Farid, H. T., Glaze, T. D., Carlson-Perret, N. L., and Lough, J. M.: Coral skeletons reveal the history of nitrogen cycling in the coastal Great Barrier Reef, Nat. Commun., 11, 1500, https://doi.org/10.1038/s41467-020-15278-w, 2020.
Fallon, S. J.: Environmental Records from Corals and Coralline Sponges, PhD Thesis, Research School of Earth Sciences, Australian National University, https://doi.org/10.25911/5d778a502c764, 2000.
Fallon, S. J., McCulloch, M. T., and Alibert, C.: Examining water temperature proxies in Porites corals from the Great Barrier Reef: a cross-shelf comparison, Coral Reefs, 22, 389–404, https://doi.org/10.1007/s00338-003-0322-5, 2003.
Felis, T., McGregor, H. V., Linsley, B. K., Tudhope, A. W., Gagan, M. K., Suzuki, A., Inoue, M., Thomas, A. L., Esat, T. M., Thompson, W. G., Tiwari, M., Potts, D. C., Mudelsee, M., Yokoyama, Y., and Webster, J. M.: Intensification of the meridional temperature gradient in the Great Barrier Reef following the Last Glacial Maximum, Nat. Commun., 5, 4102, https://doi.org/10.1038/ncomms5102, 2014.
Gagan, M. K., Chivas, A. R., and Isdale, P. J.: High-resolution isotopic records from corals using ocean temperature and mass-spawning chronometers, Earth Planet. Sc. Lett., 121, 549–558, https://doi.org/10.1016/0012-821X(94)90090-6, 1994.
Gagan, M. K., Ayliffe, L. K., Hopley, D., Cali, J. A., Mortimer, G. E., Chappell, J., McCulloch, M. T., and Head, M. J.: Temperature and Surface-Ocean Water Balance of the Mid-Holocene Tropical Western Pacific, Science, 279, 1014–1018, https://doi.org/10.1126/science.279.5353.1014, 1998.
Gagan, M. K., Ayliffe, L. K., Opdyke, B. N., Hopley, D., Scott-Gagan, H., and Cowley, J.: Coral oxygen isotope evidence for recent groundwater fluxes to the Australian Great Barrier Reef, Geophys. Res. Lett., 29, 1982, https://doi.org/10.1029/2002GL015336, 2002.
Gagan, M. K., Dunbar, G. B., and Suzuki, A.: The effect of skeletal mass accumulation in Porites on coral andä18O paleothermometry, Paleoceanography, 27, 16, https://doi.org/10.1029/2011pa002215, 2012.
GBRCD: GBR Coral Skeletal Records Database, GitHub repository [code]: https://github.com/arzeyak/GBR-Coral-Skeletal-Records-Database, last access: August 2024.
Grove, C. A., Rodriguez-Ramirez, A., Merschel, G., Tjallingii, R., Zinke, J., Macia, A., and Brummer, G.-J. A.: UV-Spectral Luminescence Scanning: Technical Updates and Calibration Developments, in: Micro-XRF Studies of Sediment Cores: Applications of a non-destructive tool for the environmental sciences, edited by: Croudace, I. W., and Rothwell, R. G., Springer Netherlands, Dordrecht, 563–581, https://doi.org/10.1007/978-94-017-9849-5_23, 2015.
Guan, Y., Hohn, S., Wild, C., and Merico, A.: Vulnerability of global coral reef habitat suitability to ocean warming, acidification and eutrophication, Glob. Change Biol., 26, 5646–5660, https://doi.org/10.1111/gcb.15293, 2020.
Hathorne, E. C., Gagnon, A., Felis, T., Adkins, J., Asami, R., Boer, W., Caillon, N., D, C., Cobb, K. M., Douville, E., deMenocal, P., Eisenhauer, A., Garbe-Schönberg, D., Geibert, W., Goldstein, S., Hughen, K., Inoue, M., Kawahata, H., Kölling, M., Cornec, F. L., Linsley, B. K., McGregor, H. V., Montagna, P., Nurhati, I. S., Quinn, T. M., Raddatz, J., Rebaubier, H., Robinson, L., Sadekov, A., Sherrell, R., Sinclair, D., Tudhope, A. W., Wei, G., Wong, H., Wu, H. C., and You, C.-F.: Interlaboratory study for coral and other element/Ca ratio measurements, Geochem. Geophy. Geosy., 14, 3730–3750, https://doi.org/10.1002/ggge.20230, 2013.
Heaton, T. J., Köhler, P., Butzin, M., Bard, E., Reimer, R. W., Austin, W. E. N., Bronk Ramsey, C., Grootes, P. M., Hughen, K. A., Kromer, B., Reimer, P. J., Adkins, J., Burke, A., Cook, M. S., Olsen, J., and Skinner, L. C.: Marine20—The Marine Radiocarbon Age Calibration Curve (0–55,000 cal BP), Radiocarbon, 62, 779–820, https://doi.org/10.1017/RDC.2020.68, 2020.
Hendy, E. J., Gagan, M. K., Alibert, C. A., McCulloch, M. T., Lough, J. M., and Isdale, P. J.: Abrupt Decrease in Tropical Pacific Sea Surface Salinity at End of Little Ice Age, Science, 295, 1511–1514, https://doi.org/10.1126/science.1067693, 2002.
Hendy, E. J., Gagan, M. K., and Lough, J. M.: Chronological control of coral records using luminescent lines and evidence for non-stationary ENSO teleconnections in northeast Australia, The Holocene, 13, 187–199, https://doi.org/10.1191/0959683603hl606rp, 2003a.
Hendy, E. J., Lough, J. M., and Gagan, M. K.: Historical mortality in massive Porites from the central Great Barrier Reef, Australia: evidence for past environmental stress?, Coral Reefs, 22, 207–215, https://doi.org/10.1007/s00338-003-0304-7, 2003b.
Hendy, E. J., Gagan, M. K., Lough, J. M., McCulloch, M., and deMenocal, P. B.: Impact of skeletal dissolution and secondary aragonite on trace element and isotopic climate proxies in Porites corals, Paleoceanography, 22, PA4101, https://doi.org/10.1029/2007PA001462, 2007.
Hendy, E. J., Tomiak, P. J., Collins, M. J., Hellstrom, J., Tudhope, A. W., Lough, J. M., and Penkman, K. E. H.: Assessing amino acid racemization variability in coral intra-crystalline protein for geochronological applications, Geochim. Cosmochim. Ac., 86, 338–353, https://doi.org/10.1016/j.gca.2012.02.020, 2012.
Henley, B. J., McGregor, H. V., King, A. D., Hoegh-Guldberg, O., Arzey, A. K., Karoly, D. J., Lough, J. M., DeCarlo, T. M., and Linsley, B. K.: Highest ocean heat in four centuries places Great Barrier Reef in danger, Nature, 632, 320–326, https://doi.org/10.1038/s41586-024-07672-x, 2024.
Hopley, D., Smithers, S. G., and Parnell, K.: The Geomorphology of the Great Barrier Reef: Development, Diversity and Change, Cambridge University Press, Cambridge, https://doi.org/10.1017/CBO9780511535543, 2007.
Hughes, T. P., Kerry, J. T., Álvarez-Noriega, M., Álvarez-Romero, J. G., Anderson, K. D., Baird, A. H., Babcock, R. C., Beger, M., Bellwood, D. R., Berkelmans, R., Bridge, T. C., Butler, I. R., Byrne, M., Cantin, N. E., Comeau, S., Connolly, S. R., Cumming, G. S., Dalton, S. J., Diaz-Pulido, G., Eakin, C. M., Figueira, W. F., Gilmour, J. P., Harrison, H. B., Heron, S. F., Hoey, A. S., Hobbs, J.-P. A., Hoogenboom, M. O., Kennedy, E. V., Kuo, C.-y., Lough, J. M., Lowe, R. J., Liu, G., McCulloch, M. T., Malcolm, H. A., McWilliam, M. J., Pandolfi, J. M., Pears, R. J., Pratchett, M. S., Schoepf, V., Simpson, T., Skirving, W. J., Sommer, B., Torda, G., Wachenfeld, D. R., Willis, B. L., and Wilson, S. K.: Global warming and recurrent mass bleaching of corals, Nature, 543, 373–377, https://doi.org/10.1038/nature21707, 2017.
Isdale, P.: Fluorescent bands in massive corals record centuries of coastal rainfall, Nature, 310, 578–579, https://doi.org/10.1038/310578a0, 1984.
Isdale, P. J., Stewart, B. J., Tickle, K. S., and Lough, J. M.: Palaeohydrological variation in a tropical river catchment: a reconstruction using fluorescent bands in corals of the Great Barrier Reef, Australia, The Holocene, 8, 1–8, https://doi.org/10.1191/095968398670905088, 1998.
Jupiter, S.: Coral rare earth element tracers of terrestrial exposure in nearshore corals of the Great Barrier Reef, Proceedings of the 11th International Coral Reef Symposium, 7–11 July 2008, Fort Lauderdale, Florida, 102–106, 2008.
Jupiter, S., Roff, G., Marion, G., Henderson, M., Schrameyer, V., McCulloch, M., and Hoegh-Guldberg, O.: Linkages between coral assemblages and coral proxies of terrestrial exposure along a cross-shelf gradient on the southern Great Barrier Reef, Coral Reefs, 27, 887–903, https://doi.org/10.1007/s00338-008-0422-3, 2008.
Jupiter, S. D.: From Cane to Coral Reefs: Ecosystem Connectivity and Downstream Responses to Land Use Intensification, University of California, Santa Cruz, 600 pp., 2006.
Kamber, B. S., Greig, A., and Collerson, K. D.: A new estimate for the composition of weathered young upper continental crust from alluvial sediments, Queensland, Australia, Geochim. Cosmochim. Ac., 69, 1041–1058, https://doi.org/10.1016/j.gca.2004.08.020, 2005.
Kaufman, D. S. and PAGES 2k special-issue editorial team: Technical note: Open-paleo-data implementation pilot – the PAGES 2k special issue, Clim. Past, 14, 593–600, https://doi.org/10.5194/cp-14-593-2018, 2018.
Khider, D., Emile-Geay, J., McKay, N. P., Gil, Y., Garijo, D., Ratnakar, V., Alonso-Garcia, M., Bertrand, S., Bothe, O., Brewer, P., Bunn, A., Chevalier, M., Comas-Bru, L., Csank, A., Dassié, E., DeLong, K., Felis, T., Francus, P., Frappier, A., Gray, W., Goring, S., Jonkers, L., Kahle, M., Kaufman, D., Kehrwald, N. M., Martrat, B., McGregor, H., Richey, J., Schmittner, A., Scroxton, N., Sutherland, E., Thirumalai, K., Allen, K., Arnaud, F., Axford, Y., Barrows, T., Bazin, L., Pilaar Birch, S. E., Bradley, E., Bregy, J., Capron, E., Cartapanis, O., Chiang, H.-W., Cobb, K. M., Debret, M., Dommain, R., Du, J., Dyez, K., Emerick, S., Erb, M. P., Falster, G., Finsinger, W., Fortier, D., Gauthier, N., George, S., Grimm, E., Hertzberg, J., Hibbert, F., Hillman, A., Hobbs, W., Huber, M., Hughes, A. L. C., Jaccard, S., Ruan, J., Kienast, M., Konecky, B., Le Roux, G., Lyubchich, V., Novello, V. F., Olaka, L., Partin, J. W., Pearce, C., Phipps, S. J., Pignol, C., Piotrowska, N., Poli, M.-S., Prokopenko, A., Schwanck, F., Stepanek, C., Swann, G. E. A., Telford, R., Thomas, E., Thomas, Z., Truebe, S., von Gunten, L., Waite, A., Weitzel, N., Wilhelm, B., Williams, J., Williams, J. J., Winstrup, M., Zhao, N., and Zhou, Y.: PaCTS 1.0: A Crowdsourced Reporting Standard for Paleoclimate Data, Paleoceanogr. Paleocl., 34, 1570–1596, https://doi.org/10.1029/2019PA003632, 2019.
Konecky, B. L., McKay, N. P., Churakova (Sidorova), O. V., Comas-Bru, L., Dassié, E. P., DeLong, K. L., Falster, G. M., Fischer, M. J., Jones, M. D., Jonkers, L., Kaufman, D. S., Leduc, G., Managave, S. R., Martrat, B., Opel, T., Orsi, A. J., Partin, J. W., Sayani, H. R., Thomas, E. K., Thompson, D. M., Tyler, J. J., Abram, N. J., Atwood, A. R., Cartapanis, O., Conroy, J. L., Curran, M. A., Dee, S. G., Deininger, M., Divine, D. V., Kern, Z., Porter, T. J., Stevenson, S. L., von Gunten, L., and Iso2k Project Members: The Iso2k database: a global compilation of paleo-δ18O and δ2H records to aid understanding of Common Era climate, Earth Syst. Sci. Data, 12, 2261–2288, https://doi.org/10.5194/essd-12-2261-2020, 2020.
Koop, K., Booth, D., Broadbent, A., Brodie, J., Bucher, D., Capone, D., Coll, J., Dennison, W., Erdmann, M., Harrison, P., Hoegh-Guldberg, O., Hutchings, P., Jones, G. B., Larkum, A. W. D., O'Neil, J., Steven, A., Tentori, E., Ward, S., Williamson, J., and Yellowlees, D.: ENCORE: The Effect of Nutrient Enrichment on Coral Reefs. Synthesis of Results and Conclusions, Mar. Pollut. Bull., 42, 91–120, https://doi.org/10.1016/S0025-326X(00)00181-8, 2001.
Lawrence, M. G., Greig, A., Collerson, K. D., and Kamber, B. S.: Rare Earth Element and Yttrium Variability in South East Queensland Waterways, Aquat. Geochem., 12, 39–72, https://doi.org/10.1007/s10498-005-4471-8, 2006.
Leonard, N. D., Welsh, K. J., Lough, J. M., Feng, Y. X., Pandolfi, J. M., Clark, T. R., and Zhao, J. X.: Evidence of reduced mid-Holocene ENSO variance on the Great Barrier Reef, Australia, Paleoceanography, 31, 1248–1260, https://doi.org/10.1002/2016PA002967, 2016.
Leonard, N. D., Welsh, K. J., Nguyen, A. D., Sadler, J., Pandolfi, J. M., Clark, T. R., Zhao, J. X., Feng, Y. x., and Webb, G. E.: High resolution geochemical analysis of massive Porites spp. corals from the Wet Tropics, Great Barrier Reef: rare earth elements, yttrium and barium as indicators of terrigenous input, Mar. Pollut. Bull., 149, 110634, https://doi.org/10.1016/j.marpolbul.2019.110634, 2019.
Leonard, N. D., Lepore, M. L., Zhao, J.-X., Rodriguez-Ramirez, A., Butler, I. R., Clark, T. R., Roff, G., McCook, L., Nguyen, A. D., Feng, Y., and Pandolfi, J. M.: Re-evaluating mid-Holocene reef “turn-off” on the inshore Southern Great Barrier Reef, Quaternary Sci. Rev., 244, 106518, https://doi.org/10.1016/j.quascirev.2020.106518, 2020.
Lewis, S. E.: Environmental Trends in GBR lagoon and Burdekin River catchment during the mid-Holocene and since European settlement using Porites coral records, Magnetic Island, QLD, PhD Thesis, School of Earth Sciences, James Cook University, Townsville, QLD, 2005.
Lewis, S. E., Shields, G. A., Kamber, B. S., and Lough, J. M.: A multi-trace element coral record of land-use changes in the Burdekin River catchment, NE Australia, Palaeogeogr. Palaeoclimatol., 246, 471–487, https://doi.org/10.1016/j.palaeo.2006.10.021, 2007.
Lewis, S. E., Brodie, J. E., McCulloch, M. T., Mallela, J., Jupiter, S. D., Williams, H. S., Lough, J. M., and Matson, E. G.: An assessment of an environmental gradient using coral geochemical records, Whitsunday Islands, Great Barrier Reef, Australia, Mar. Pollut. Bull., 65, 306–319, https://doi.org/10.1016/j.marpolbul.2011.09.030, 2012.
Lewis, S. E., Sloss, C. R., Murray-Wallace, C. V., Woodroffe, C. D., and Smithers, S. G.: Post-glacial sea-level changes around the Australian margin: a review, Quaternary Sci. Rev., 74, 115–138, https://doi.org/10.1016/j.quascirev.2012.09.006, 2013.
Lewis, S. E., Lough, J. M., Cantin, N. E., Matson, E. G., Kinsley, L., Bainbridge, Z. T., and Brodie, J. E.: A critical evaluation of coral , and ratios as indicators of terrestrial input: New data from the Great Barrier Reef, Australia, Geochim. Cosmochim. Ac., 237, 131–154, https://doi.org/10.1016/j.gca.2018.06.017, 2018.
Li, Y., Liao, X., Bi, K., Han, T., Chen, J., Lu, J., He, C., and Lu, Z.: Micro-CT reconstruction reveals the colony pattern regulations of four dominant reef-building corals, Ecol. Evol., 11, 16266–16279, https://doi.org/10.1002/ece3.8308, 2021.
Linsley, B. K., Dunbar, R. B., Dassié, E. P., Tangri, N., Wu, H. C., Brenner, L. D., and Wellington, G. M.: Coral carbon isotope sensitivity to growth rate and water depth with paleo-sea level implications, Nat. Commun., 10, 2056, https://doi.org/10.1038/s41467-019-10054-x, 2019.
Lough, J., Barnes, D., and McAllister, F.: Luminescent lines in corals from the Great Barrier Reef provide spatial and temporal records of reefs affected by land runoff, Coral Reefs, 21, 333–343, https://doi.org/10.1007/s00338-002-0253-6, 2002.
Lough, J. M.: A strategy to improve the contribution of coral data to high-resolution paleoclimatology, Palaeogeogr. Palaeoclimatol., 204, 115–143, https://doi.org/10.1016/S0031-0182(03)00727-2, 2004.
Lough, J. M.: Tropical river flow and rainfall reconstructions from coral luminescence: Great Barrier Reef, Australia, Paleoceanography, 22, PA2218, https://doi.org/10.1029/2006pa001377, 2007.
Lough, J. M.: Great Barrier Reef coral luminescence reveals rainfall variability over northeastern Australia since the 17th century, Paleoceanography, 26, 14, https://doi.org/10.1029/2010pa002050, 2011a.
Lough, J. M.: Measured coral luminescence as a freshwater proxy: comparison with visual indices and a potential age artefact, Coral Reefs, 30, 169–182, https://doi.org/10.1007/s00338-010-0688-0, 2011b.
Lough, J. M. and Barnes, D. J.: Possible relationships between environmental variables and skeletal density in a coral colony from the central Great Barrier Reef, J. Exp. Mar. Biol. Ecol., 134, 221–241, https://doi.org/10.1016/0022-0981(89)90071-3, 1990.
Lough, J. M. and Barnes, D. J.: Comparisons of skeletal density variations in Porites from the central Great Barrier Reef, J. Exp. Mar. Biol. Ecol., 155, 1–25, https://doi.org/10.1016/0022-0981(92)90024-5, 1992.
Lough, J. M. and Barnes, D. J.: Several centuries of variation in skeletal extension, density and calcification in massive Porites colonies from the Great Barrier Reef: a proxy for seawater temperature and a background of variability against which to identify unnatural change, J. Exp. Mar. Biol. Ecol., 211, 29–67, https://doi.org/10.1016/S0022-0981(96)02710-4, 1997.
Lough, J. M. and Barnes, D. J.: Environmental controls on growth of the massive coral Porites, J. Exp. Mar. Biol. Ecol., 245, 225–243, https://doi.org/10.1016/S0022-0981(99)00168-9, 2000.
Lough, J. M., Llewellyn, L. E., Lewis, S. E., Turney, C. S. M., Palmer, J. G., Cook, C. G., and Hogg, A. G.: Evidence for suppressed mid-Holocene northeastern Australian monsoon variability from coral luminescence, Paleoceanography, 29, 581–594, https://doi.org/10.1002/2014pa002630, 2014.
Lough, J. M., Lewis, S. E., and Cantin, N. E.: Freshwater impacts in the central Great Barrier Reef: 1648–2011, Coral Reefs, 34, 739–751, https://doi.org/10.1007/s00338-015-1297-8, 2015.
Ludwig, K. R.: Mathematical–Statistical Treatment of Data and Errors for 230Th/U Geochronology, in: Uranium-Series Geochemistry, edited by: Bourdon, B., Henderson, G. M., Lundstrom, C. C., and Turner, S. P., The Mineralogical Society of America, Washington, DC, 631–656, https://doi.org/10.2113/0520631, 2003.
Madin, J. S., Anderson, K. D., Andreasen, M. H., Bridge, T. C. L., Cairns, S. D., Connolly, S. R., Darling, E. S., Diaz, M., Falster, D. S., Franklin, E. C., Gates, R. D., Harmer, A. M. T., Hoogenboom, M. O., Huang, D., Keith, S. A., Kosnik, M. A., Kuo, C.-Y., Lough, J. M., Lovelock, C. E., Luiz, O., Martinelli, J., Mizerek, T., Pandolfi, J. M., Pochon, X., Pratchett, M. S., Putnam, H. M., Roberts, T. E., Stat, M., Wallace, C. C., Widman, E., and Baird, A. H.: The Coral Trait Database, a curated database of trait information for coral species from the global oceans, Sci. Data, 3, 160017, https://doi.org/10.1038/sdata.2016.17, 2016.
Marchitto, T. M., Bryan, S. P., Doss, W., McCulloch, M. T., and Montagna, P.: A simple biomineralization model to explain Li, Mg, and Sr incorporation into aragonitic foraminifera and corals, Earth Planet. Sc. Lett., 481, 20–29, https://doi.org/10.1016/j.epsl.2017.10.022, 2018.
Marion, G. S., Jupiter, S. D., Radice, V. Z., Albert, S., and Hoegh-Guldberg, O.: Linking isotopic signatures of nitrogen in nearshore coral skeletons with sources in catchment runoff, Mar. Pollut. Bull., 173, 113054, https://doi.org/10.1016/j.marpolbul.2021.113054, 2021.
Marshall, J. F.: Decadal-scale, high resolution records of sea surface temperature in the eastern Indian and south western Pacific Oceans from proxy records of the strontium/calcium ratio of massive porites corals, PhD thesis, Australian National University, https://doi.org/10.25911/5d63bfacacc9f, 2000.
Marshall, J. F. and McCulloch, M. T.: An assessment of the ratio in shallow water hermatypic corals as a proxy for sea surface temperature, Geochim. Cosmochim. Ac., 66, 3263–3280, https://doi.org/10.1016/S0016-7037(02)00926-2, 2002.
Maynard, J., van Hooidonk, R., Eakin, C. M., Puotinen, M., Garren, M., Williams, G., Heron, S. F., Lamb, J., Weil, E., Willis, B., and Harvell, C. D.: Projections of climate conditions that increase coral disease susceptibility and pathogen abundance and virulence, Nat. Clim. Change, 5, 688–694, https://doi.org/10.1038/nclimate2625, 2015.
McCulloch, M., Fallon, S., Wyndham, T., Hendy, E., Lough, J., and Barnes, D.: Coral record of increased sediment flux to the inner Great Barrier Reef since European settlement, Nature, 421, 727, https://doi.org/10.1038/nature01361, 2003.
McCulloch, M. T., Gagan, M. K., Mortimer, G. E., Chivas, A. R., and Isdale, P. J.: A high-resolution and ä18O coral record from the Great Barrier Reef, Australia, and the 1982–1983 El Niño, Geochim. Cosmochim. Ac., 58, 2747–2754, https://doi.org/10.1016/0016-7037(94)90142-2, 1994.
McCulloch, M. T., D'Olivo, J. P., Falter, J., Holcomb, M., and Trotter, J. A.: Coral calcification in a changing World and the interactive dynamics of pH and DIC upregulation, Nat. Commun., 8, 15686, https://doi.org/10.1038/ncomms15686, 2017.
McGregor, H. V. and Abram, N. J.: Images of diagenetic textures in Porites corals from Papua New Guinea and Indonesia, Geochem. Geophys. Geosyst., 9, Q10013, https://doi.org/10.1029/2008GC002093, 2008.
McGregor, H. V. and Gagan, M. K.: Diagenesis and geochemistry of porites corals from Papua New Guinea: Implications for paleoclimate reconstruction, Geochim. Cosmochim. Ac., 67, 2147–2156, https://doi.org/10.1016/S0016-7037(02)01050-5, 2003.
McKay, N. P. and Emile-Geay, J.: Technical note: The Linked Paleo Data framework – a common tongue for paleoclimatology, Clim. Past, 12, 1093–1100, https://doi.org/10.5194/cp-12-1093-2016, 2016.
Min, G. R., Edwards, R. L., Taylor, F. W., Recy, J., Gallup, C. D., and Beck, J. W.: Annual cycles of UCa in coral skeletons and UCa thermometry, Geochim. Cosmochim. Ac., 59, 2025–2042, https://doi.org/10.1016/0016-7037(95)00124-7, 1995.
Mollica, N. R., Guo, W., Cohen, A. L., Huang, K.-F., Foster, G. L., Donald, H. K., and Solow, A. R.: Ocean acidification affects coral growth by reducing skeletal density, P. Natl. Acad. Sci. USA, 115, 1754–1759, https://doi.org/10.1073/pnas.1712806115, 2018.
Morrill, C., Thrasher, B., Lockshin, S. N., Gille, E. P., McNeill, S., Shepherd, E., Gross, W. S., and Bauer, B. A.: The Paleoenvironmental Standard Terms (PaST) Thesaurus: Standardizing Heterogeneous Variables in Paleoscience, Paleoceanogr. Paleocl., 36, e2020PA004193, https://doi.org/10.1029/2020PA004193, 2021.
Murphy, R., Webster, J. M., Nothdurft, L., Dechnik, B., and McGregor, H. V.: High-resolution hyperspectral imaging of diagenesis and clays in fossil coral reef material: a nondestructive tool for improving environmental and climate reconstructions, Geochem. Geophys. Geosysy., 18, 3209–3230, 10.1002/2017GC006949, 2017.
Nguyen, A. D., Zhao, J. x., Feng, Y. x., Hu, W. p., Yu, K. f., Gasparon, M., Pham, T. B., and Clark, T. R.: Impact of recent coastal development and human activities on Nha Trang Bay, Vietnam: evidence from a Porites lutea geochemical record, Coral Reefs, 32, 181–193, https://doi.org/10.1007/s00338-012-0962-4, 2013.
Nothdurft, L. D. and Webb, G. E.: Earliest diagenesis in scleractinian coral skeletons: implications for palaeoclimate-sensitive geochemical archives, Facies, 55, 161–201, https://doi.org/10.1007/s10347-008-0167-z, 2009.
Nothdurft, L. D., Webb, G. E., Bostrom, T., and Rintoul, L.: Calcite-filled borings in the most recently deposited skeleton in live-collected Porites (Scleractinia): Implications for trace element archives, Geochim. Cosmochim. Ac., 71, 5423–5438, https://doi.org/10.1016/j.gca.2007.09.025, 2007.
Okai, T., Suzuki, A., Kawahata, H., Terashima, S., and Imai, N.: Preparation of a New Geological Survey of Japan Geochemical Reference Material: Coral JCp-1, Geostandard. Newslett., 26, 95–99, https://doi.org/10.1111/j.1751-908X.2002.tb00627.x, 2002.
Ortiz, J.-C., Wolff, N. H., Anthony, K. R. N., Devlin, M., Lewis, S., and Mumby, P. J.: Impaired recovery of the Great Barrier Reef under cumulative stress, Sci. Adv., 4, eaar6127, https://doi.org/10.1126/sciadv.aar6127, 2018.
Palmer, J. G., Cook, E. R., Turney, C. S. M., Allen, K., Fenwick, P., Cook, B. I., O'Donnell, A., Lough, J., Grierson, P., and Baker, P.: Drought variability in the eastern Australia and New Zealand summer drought atlas (ANZDA, CE 1500–2012) modulated by the Interdecadal Pacific Oscillation, Environ. Res. Lett., 10, 124002, https://doi.org/10.1088/1748-9326/10/12/124002, 2015.
Pelejero, C., Calvo, E., McCulloch, M. T., Marshall, J. F., Gagan, M. K., Lough, J. M., and Opdyke, B. N.: Preindustrial to Modern Interdecadal Variability in Coral Reef pH, Science, 309, 2204–2207, https://doi.org/10.1126/science.1113692, 2005.
Prouty, N. G., Cohen, A., Yates, K. K., Storlazzi, C. D., Swarzenski, P. W., and White, D.: Vulnerability of Coral Reefs to Bioerosion From Land-Based Sources of Pollution, J. Geophys. Res.-Oceans, 122, 9319–9331, https://doi.org/10.1002/2017JC013264, 2017.
Quinn, T. M. and Taylor, F. W.: SST artifacts in coral proxy records produced by early marine diagenesis in a modern coral from Rabaul, Papua New Guinea, Geophys. Res. Lett., 33, L04601, https://doi.org/10.1029/2005GL024972, 2006.
Rayner, N. A., Parker, D. E., Horton, E. B., Folland, C. K., Alexander, L. V., Rowell, D. P., Kent, E. C., and Kaplan, A.: Global analyses of sea surface temperature, sea ice, and night marine air temperature since the late nineteenth century, J. Geophys. Res.-Atmos., 108, 4407, https://doi.org/10.1029/2002JD002670, 2003.
Razak, T. B., Mumby, P. J., Nguyen, A. D., Zhao, J.-X., Lough, J. M., Cantin, N. E., and Roff, G.: Use of skeletal ratios to determine growth patterns in a branching coral Isopora palifera, Mar. Biol., 164, 96, https://doi.org/10.1007/s00227-017-3099-8, 2017.
Reed, E. V., Cole, J. E., Lough, J. M., Thompson, D., and Cantin, N. E.: Linking climate variability and growth in coral skeletal records from the Great Barrier Reef, Coral Reefs, 38, 29–43, https://doi.org/10.1007/s00338-018-01755-8, 2019.
Reynolds, R. W., Rayner, N. A., Smith, T. M., Stokes, D. C., and Wang, W.: An Improved In Situ and Satellite SST Analysis for Climate, J. Climate, 15, 1609–1625, https://doi.org/10.1175/1520-0442(2002)015<1609:AIISAS>2.0.CO;2, 2002.
Roche, R. C., Perry, C. T., Smithers, S. G., Leng, M. J., Grove, C. A., Sloane, H. J., and Unsworth, C. E.: Mid-Holocene sea surface conditions and riverine influence on the inshore Great Barrier Reef, The Holocene, 24, 885–897, https://doi.org/10.1177/0959683614534739, 2014.
Rodriguez-Ramirez, A., Grove, C. A., Zinke, J., Pandolfi, J. M., and Zhao, J.-X.: Coral Luminescence Identifies the Pacific Decadal Oscillation as a Primary Driver of River Runoff Variability Impacting the Southern Great Barrier Reef, PLOS ONE, 9, e84305, https://doi.org/10.1371/journal.pone.0084305, 2014.
Ryan, E. J., Smithers, S. G., Lewis, S. E., Clark, T. R., and Zhao, J.-X.: The Variable Influences of Sea Level, Sedimentation and Exposure on Holocene Reef Development over a Cross-Shelf Transect, Central Great Barrier Reef, Diversity, 10, 110, https://doi.org/10.3390/d10040110, 2018.
Sadler, J., Webb, G. E., Nothdurft, L. D., and Dechnik, B.: Geochemistry-based coral palaeoclimate studies and the potential of `non-traditional' (non-massive Porites) corals: Recent developments and future progression, Earth-Sci. Rev., 139, 291–316, https://doi.org/10.1016/j.earscirev.2014.10.002, 2014.
Sadler, J., Nguyen, A. D., Leonard, N. D., Webb, G. E., and Nothdurft, L. D.: Acropora interbranch skeleton ratios: Evaluation of a potential new high-resolution paleothermometer, Paleoceanography, 31, 505–517, https://doi.org/10.1002/2015PA002898, 2016a.
Sadler, J., Webb, G. E., Leonard, N. D., Nothdurft, L. D., and Clark, T. R.: Reef core insights into mid-Holocene water temperatures of the southern Great Barrier Reef, Paleoceanography, 31, 1395–1408, https://doi.org/10.1002/2016pa002943, 2016b.
Saha, N., Rodriguez-Ramirez, A., Nguyen, A. D., Clark, T. R., Zhao, J. X., and Webb, G. E.: Seasonal to decadal scale influence of environmental drivers on and in coral aragonite from the southern Great Barrier Reef, Sci. Total Environ., 639, 1099–1109, https://doi.org/10.1016/j.scitotenv.2018.05.156, 2018a.
Saha, N., Webb, G. E., Zhao, J.-X., Leonard, N. D., and Nguyen, A. D.: Influence of marine biochemical cycles on seasonal variation of in the near-shore coral Cyphastrea, Rat Island, southern Great Barrier Reef, Chem. Geol., 499, 71–83, https://doi.org/10.1016/j.chemgeo.2018.09.005, 2018b.
Saha, N., Webb, G. E., Christy, A. G., and Zhao, J.-X.: Vanadium in the massive coral Porites: A potential proxy for historical wood clearing and burning, Earth Planet. Sc. Lett., 527, 115793, https://doi.org/10.1016/j.epsl.2019.115793, 2019a.
Saha, N., Webb, G. E., Zhao, J.-X., Nguyen, A. D., Lewis, S. E., and Lough, J. M.: Coral-based high-resolution rare earth element proxy for terrestrial sediment discharge affecting coastal seawater quality, Great Barrier Reef, Geochim. Cosmochim. Ac., 254, 173–191, https://doi.org/10.1016/j.gca.2019.04.004, 2019b.
Saha, N., Webb, G. E., Zhao, J.-X., Lewis, S. E., Nguyen, A. D., and Feng, Y.: Spatiotemporal variation of rare earth elements from river to reef continuum aids monitoring of terrigenous sources in the Great Barrier Reef, Geochim. Cosmochim. Ac., 299, 85–112, https://doi.org/10.1016/j.gca.2021.02.014, 2021.
Sammarco, P. W., Risk, M. J., Schwarcz, H. P., and Heikoop, J. M.: Cross-continental shelf trends in coral ä15N on the Great Barrier Reef: further consideration of the reef nutrient paradox, Mar. Ecol. Prog. Ser., 180, 131–138, 1999.
Sanborn, K. L., Webster, J. M., Webb, G. E., Braga, J. C., Humblet, M., Nothdurft, L., Patterson, M. A., Dechnik, B., Warner, S., Graham, T., Murphy, R. J., Yokoyama, Y., Obrochta, S. P., Zhao, J.-X., and Salas-Saavedra, M.: A new model of Holocene reef initiation and growth in response to sea-level rise on the Southern Great Barrier Reef, Sediment. Geol., 397, 105556, https://doi.org/10.1016/j.sedgeo.2019.105556, 2020.
Sayani, H. R., Cobb, K. M., Cohen, A. L., Elliott, W. C., Nurhati, I. S., Dunbar, R. B., Rose, K. A., and Zaunbrecher, L. K.: Effects of diagenesis on paleoclimate reconstructions from modern and young fossil corals, Geochim. Cosmochim. Ac., 75, 6361–6373, https://doi.org/10.1016/j.gca.2011.08.026, 2011.
Sinclair, D. J., Kinsley, L. P. J., and McCulloch, M. T.: High resolution analysis of trace elements in corals by laser ablation ICP-MS, Geochim. Cosmochim. Ac., 62, 1889–1901, https://doi.org/10.1016/S0016-7037(98)00112-4, 1998.
Spencer, T., Brown, B., Hamylton, S., and McLean, R.: `A Close and Friendly Alliance': Biology, Geology and the Great Barrier Reef Expedition of 1928–1929, in: Oceanography and Marine Biology edited by: Hawkins, S. J., 89–138, https://doi.org/10.1201/9781003138846-2, 2021.
Steinberg, C.: Impacts of climate change on the physical oceanography of the Great Barrier Reef, in: Climate change and the Great Barrier Reef: a vulnerability assessment, The Great Barrier Reef Marine Park Authority, https://hdl.handle.net/11017/536 (last access: 21 December 2020), 2007.
Steven, A. D. L. and Atkinson, M. J.: Nutrient uptake by coral-reef microatolls, Coral Reefs, 22, 197–204, https://doi.org/10.1007/s00338-003-0303-8, 2003.
Stuiver, M. and Reimer, P. J.: Extended 14C Data Base and Revised CALIB 3.0 14C Age Calibration Program, Radiocarbon, 35, 215–230, https://doi.org/10.1017/S0033822200013904, 1993.
Suzuki, A., Gagan, M. K., Fabricius, K., Isdale, P. J., Yukino, I., and Kawahata, H.: Skeletal isotope microprofiles of growth perturbations in Porites corals during the 1997–1998 mass bleaching event, Coral Reefs, 22, 357–369, https://doi.org/10.1007/s00338-003-0323-4, 2003.
Takada, N., Suzuki, A., Ishii, H., Hironaka, K., and Hironiwa, T.: Thermoluminescence of coral skeletons: a high-sensitivity proxy of diagenetic alteration of aragonite, Sci. Rep.-UK, 7, 17969, https://doi.org/10.1038/s41598-017-18269-y, 2017.
Thompson, D., McCulloch, M., Cole, J. E., Reed, E. V., D'Olivo, J. P., Dyez, K., Lofverstrom, M., Lough, J., Cantin, N., Tudhope, A. W., Cheung, A. H., Vetter, L., and Edwards, R. L.: Marginal Reefs Under Stress: Physiological Limits Render Galápagos Corals Susceptible to Ocean Acidification and Thermal Stress, AGU Adv., 3, e2021AV000509, https://doi.org/10.1029/2021AV000509, 2022.
Thompson, D. M.: Environmental records from coral skeletons: A decade of novel insights and innovation, WIREs Clim. Change, 13, e745, https://doi.org/10.1002/wcc.745, 2022.
Vines, T. H., Albert, A. Y. K., Andrew, R. L., Débarre, F., Bock, D. G., Franklin, M. T., Gilbert, K. J., Moore, J.-S., Renaut, S., and Rennison, D. J.: The Availability of Research Data Declines Rapidly with Article Age, Curr. Biol., 24, 94–97, https://doi.org/10.1016/j.cub.2013.11.014, 2014.
Walter, R. M., Sayani, H. R., Felis, T., Cobb, K. M., Abram, N. J., Arzey, A. K., Atwood, A. R., Brenner, L. D., Dassié, É. P., DeLong, K. L., Ellis, B., Emile-Geay, J., Fischer, M. J., Goodkin, N. F., Hargreaves, J. A., Kilbourne, K. H., Krawczyk, H., McKay, N. P., Moore, A. L., Murty, S. A., Ong, M. R., Ramos, R. D., Reed, E. V., Samanta, D., Sanchez, S. C., Zinke, J., and the PAGES CoralHydro2k Project Members: The CoralHydro2k database: a global, actively curated compilation of coral δ18O and proxy records of tropical ocean hydrology and temperature for the Common Era, Earth Syst. Sci. Data, 15, 2081–2116, https://doi.org/10.5194/essd-15-2081-2023, 2023.
Walther, B. D., Kingsford, M. J., and McCulloch, M. T.: Environmental Records from Great Barrier Reef Corals: inshore versus offshore drivers, PLoS One, 8, e77091, https://doi.org/10.1371/journal.pone.0077091, 2013.
Wang, Z., Li, J., Wei, G., Deng, W., Chen, X., Zeng, T., Wang, X., Ma, J., Zhang, L., Tu, X., Wang, Q., and McCulloch, M.: Biologically controlled Mo isotope fractionation in coral reef systems, Geochim. Cosmochim. Ac., 262, 128–142, https://doi.org/10.1016/j.gca.2019.07.037, 2019.
Weber, J. N.: Incorporation of strontium into reef coral skeletal carbonate, Geochim. Cosmochim. Ac., 37, 2173–2190, https://doi.org/10.1016/0016-7037(73)90015-X, 1973.
Weber, J. N. and Woodhead, P. M. J.: Factors affecting the carbon and oxygen isotopic composition of marine carbonate sediments – II. Heron Island, Great Barrier Reef, Australia, Geochim. Cosmochim. Ac., 33, 19–38, https://doi.org/10.1016/0016-7037(69)90090-8, 1969.
Weber, J. N. and Woodhead, P. M. J.: Carbon and Oxygen Isotope Fractionation in the Skeletal Carbonate of Reef-Building Corals, Chem. Geol., 6, 93–117, https://doi.org/10.1016/0009-2541(70)90009-4, 1970.
Weber, J. N. and Woodhead, P. M. J.: Temperature dependence of oxygen-18 concentration in reef coral carbonates, J. Geophys. Res., 77, 463–473, https://doi.org/10.1029/JC077i003p00463, 1972.
Webster, J. M., Braga, J. C., Humblet, M., Potts, D. C., Iryu, Y., Yokoyama, Y., Fujita, K., Bourillot, R., Esat, T. M., Fallon, S., Thompson, W. G., Thomas, A. L., Kan, H., McGregor, H. V., Hinestrosa, G., Obrochta, S. P., and Lougheed, B. C.: Response of the Great Barrier Reef to sea-level and environmental changes over the past 30,000 years, Nat. Geosci., 11, 426–432, https://doi.org/10.1038/s41561-018-0127-3, 2018.
Weerabaddana, M. M., Thompson, D. M., Reed, E. V., Farfan, G. A., Kirk, J. D., Kojima, A. C., Dettman, D. L., de Brum, K., Kabua, E., and Edwards, F.: Impact of Intra-Skeletal Calcite on the Preservation of Coral Geochemistry and Implications for Paleoclimate Reconstruction, Paleoceanogr. Paleocl., 39, e2023PA004730, https://doi.org/10.1029/2023PA004730, 2024.
Wei, G., McCulloch, M. T., Mortimer, G., Deng, W., and Xie, L.: Evidence for ocean acidification in the Great Barrier Reef of Australia, Geochim. Cosmochim. Ac., 73, 2332–2346, https://doi.org/10.1016/j.gca.2009.02.009, 2009.
Wei, G., Wang, Z., Ke, T., Liu, Y., Deng, W., Chen, X., Xu, J., Zeng, T., and Xie, L.: Decadal variability in seawater pH in the West Pacific: Evidence from coral ä11B records, J. Geophys. Res.-Oceans, 120, 7166–7181, https://doi.org/10.1002/2015JC011066, 2015.
Wilkinson, M. D., Dumontier, M., Aalbersberg, I. J., Appleton, G., Axton, M., Baak, A., Blomberg, N., Boiten, J.-W., da Silva Santos, L. B., Bourne, P. E., Bouwman, J., Brookes, A. J., Clark, T., Crosas, M., Dillo, I., Dumon, O., Edmunds, S., Evelo, C. T., Finkers, R., Gonzalez-Beltran, A., Gray, A. J. G., Groth, P., Goble, C., Grethe, J. S., Heringa, J., 't Hoen, P. A. C., Hooft, R., Kuhn, T., Kok, R., Kok, J., Lusher, S. J., Martone, M. E., Mons, A., Packer, A. L., Persson, B., Rocca-Serra, P., Roos, M., van Schaik, R., Sansone, S.-A., Schultes, E., Sengstag, T., Slater, T., Strawn, G., Swertz, M. A., Thompson, M., van der Lei, J., van Mulligen, E., Velterop, J., Waagmeester, A., Wittenburg, P., Wolstencroft, K., Zhao, J., and Mons, B.: The FAIR Guiding Principles for scientific data management and stewardship, Sci. Data, 3, 160018, https://doi.org/10.1038/sdata.2016.18, 2016.
Wu, Y., Fallon, S. J., Cantin, N. E., and Lough, J. M.: Surface ocean radiocarbon from a Porites coral record in the Great Barrier Reef: 1945–2017, Radiocarbon, 63, 1193–1203, https://doi.org/10.1017/RDC.2020.141, 2021a.
Wu, Y., Fallon, S. J., Cantin, N. E., and Lough, J. M.: Assessing multiproxy approaches (, , , and ) to reconstruct sea surface temperature from coral skeletons throughout the Great Barrier Reef, Sci. Total Environ., 786, 147393, https://doi.org/10.1016/j.scitotenv.2021.147393, 2021b.
Wyndham, T., McCulloch, M., Fallon, S., and Alibert, C.: High-resolution coral records of rare earth elements in coastal seawater: biogeochemical cycling and a new environmental proxy, Geochim. Cosmochim. Ac., 68, 2067–2080, https://doi.org/10.1016/j.gca.2003.11.004, 2004.
Xiao, H., Deng, W., Wei, G., Chen, J., Zheng, X., Shi, T., Chen, X., Wang, C., Liu, X., and Zeng, T.: A Pilot Study on Zinc Isotopic Compositions in Shallow-Water Coral Skeletons, Geochem. Geophys. Geosyst., 21, e2020GC009430, https://doi.org/10.1029/2020GC009430, 2020.
Yokoyama, Y., Webster, J. M., Cotterill, C., Braga, J. C., Jovane, L., Mills, H., Morgan, S., Suzuki, A., and the IODP Expedition 325 Scientists: IODP Expedition 325: Great Barrier Reefs Reveals Past Sea-Level, Climate and Environmental Changes Since the Last Ice Age, Sci. Dril., 12, 32–45, https://doi.org/10.2204/iodp.sd.12.04.2011, 2011.
- Abstract
- Introduction
- Overview of the GBR Coral Skeletal Records Database
- Database use and citation
- Characterising the database
- Discussion
- Code and data availability
- Conclusions
- Appendix A: GBRCD records
- Appendix B: GBRCD to LiPD field translation
- Appendix C: GBRCD additional coral information
- Appendix D: Uranium–thorium dating information
- Appendix E: GBRCD data resources
- Author contributions
- Competing interests
- Disclaimer
- Acknowledgements
- Financial support
- Review statement
- References
- Abstract
- Introduction
- Overview of the GBR Coral Skeletal Records Database
- Database use and citation
- Characterising the database
- Discussion
- Code and data availability
- Conclusions
- Appendix A: GBRCD records
- Appendix B: GBRCD to LiPD field translation
- Appendix C: GBRCD additional coral information
- Appendix D: Uranium–thorium dating information
- Appendix E: GBRCD data resources
- Author contributions
- Competing interests
- Disclaimer
- Acknowledgements
- Financial support
- Review statement
- References