the Creative Commons Attribution 4.0 License.
the Creative Commons Attribution 4.0 License.
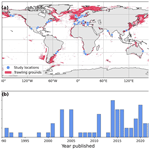
Demersal fishery Impacts on Sedimentary Organic Matter (DISOM): a global harmonized database of studies assessing the impacts of demersal fisheries on sediment biogeochemistry
Justin Tiano
Emil De Borger
Antonio Pusceddu
Clare Bradshaw
Claudia Ennas
Claudia Morys
Marija Sciberras
Marine sediments are among the largest carbon reservoirs on the planet and play a key role in the global cycling of organic matter. Bottom fisheries are the most widespread anthropogenic physical disturbance to seabed habitats, prompting NGOs and governments to act on regulating mobile bottom-contacting fishing gear. However, the scientific evidence of the effects of bottom trawling on sediment biogeochemistry is highly diverse and presents contrasting results. Here we present a global harmonized dataset of 71 independent studies that assess the effects of demersal fisheries on sedimentological (i.e. grain size, porosity) and biogeochemical (i.e. organic carbon, phytopigments, nutrient fluxes) properties: the Demersal fishery Impacts on Sedimentary Organic Matter (DISOM) database (Paradis, 2023; https://doi.org/10.3929/ethz-b-000634336). We identify considerable gaps, namely in the geographical extension of the data; coverage of environmental predictors (i.e. seasons); fishing descriptors such as the availability of true controls, quantification of fishing effort, and distribution of fishing gear types; and biogeochemical variables that study the remineralization of organic matter. Future studies should address these data gaps to enhance the comprehensiveness of the dataset. With this harmonized database, we aim to allow researchers to explore the effects of demersal fisheries in variable environmental settings to disentangle the effects of this disturbance and provide efficient management strategies.
- Article
(9812 KB) - Full-text XML
-
Supplement
(336 KB) - BibTeX
- EndNote
Demersal fishing is one of the most destructive anthropogenic activities in the ocean given its widespread occurrence, covering nearly all continental margins (Amoroso et al., 2018; Kroodsma et al., 2018) and increasing in intensity over time (Watson and Tidd, 2018). Fishing with mobile bottom-contacting fishing gear (e.g. trawls, dredges) alters the composition of benthic communities, with a general decrease in faunal biomass and biodiversity after disturbance, though effects vary in accordance with fishing intensity, type of fishing gear, sediment type, and biological traits of the resident community (e.g. mobility and longevity) (Hiddink et al., 2017, 2019; Sciberras et al., 2018). In addition, the mixing and resuspension of sediment that results from dragging the gears across the seafloor changes the biogeochemical and physical characteristics of fishing grounds (Martín et al., 2014b; Oberle et al., 2018).
Results from trawling studies on sediment biogeochemistry are variable (Epstein et al., 2022). Certain studies have observed that demersal fisheries reduce sedimentary organic matter (Hale et al., 2017; Mayer et al., 1991; Tiano et al., 2019), and others have reported increases (Palanques et al., 2014; Pusceddu et al., 2005a; Sciberras et al., 2016), while some have found statistically insignificant effects (Bhagirathan et al., 2010; Smith et al., 2000). Similarly, variable effects have been observed on the organic matter remineralization rates, with both increases (Paradis et al., 2019; Polymenakou et al., 2005) and decreases (Tiano et al., 2019; Warnken et al., 2003) after fishing disturbance. The inconsistency in these results highlights the fact that the effects of demersal fisheries on biogeochemical processes on the seafloor may be context- and site-specific, and caution must be taken when upscaling the effects to global demersal fishing grounds (Sala et al., 2021; Hiddink et al., 2023).
The wide array of studies assessing the effects of demersal fisheries have their own unique designs and sampling strategies, which could affect the observed outcomes if they are not considered: manipulative experimental studies report the immediate effect of sediment disturbance, whereas comparative studies tend to assess chronic effects of demersal fisheries. In addition, the environmental conditions of the study area such as sediment grain size influence the effect of sediment disturbance. Coarser-grained sediments present in dynamic environments with strong bottom currents are generally characterized by low organic carbon contents and higher permeabilities regimes that lead to lower organic matter accumulation when compared to finer-grained muddy environments (Burdige, 2007; Huettel et al., 2014). In contrast, higher organic carbon contents are found in muddy areas of the seafloor given their higher surface area (Keil et al., 1998; Mayer, 1994). In addition, fishing descriptors such as gear penetration depth, fishing intensity, and frequency between disturbance events determine the effect of bottom fishing on benthic communities (Hiddink et al., 2017; Sciberras et al., 2018), as well as on sediment biogeochemistry (Depestele et al., 2019; Tiano et al., 2019, 2021). Similarly, different results may be obtained if the study is conducted in an area that has been chronically fished, due to the cumulative effects of sediment disturbance (De Borger et al., 2021).
To properly understand the effects of bottom trawling on sediment biogeochemistry in diverse sedimentary environments worldwide, a global harmonized dataset of the studies that investigate the effects of mobile bottom-contacting fishing gear on sediment biogeochemistry is needed. The need for this global harmonized dataset is reflected by the growing attention on the impacts of demersal fisheries on sediment organic carbon stocks (Legge et al., 2020) and the proposal of variable management strategies (Black et al., 2022; Epstein and Roberts, 2022). This harmonized database will allow researchers, managers, and policymakers to determine the biogeochemical effects of bottom trawling within a global context while also considering context-dependent factors. Moreover, it will allow reproducible meta-analyses to be conducted from different perspectives, as well as training and validating models that upscale the biogeochemical impacts of bottom trawling on a global scale.
Here we present the first global dataset that compiles information on the sedimentological (i.e. grain size, porosity) and biogeochemical (i.e. organic carbon, phytopigments, nutrient fluxes) variables. Importantly, this database harmonizes metadata of the different studies, given their inevitable influence on their findings. Finally, we also specify important metadata that should be reported in future studies to allow their inclusion in global meta-analyses.
2.1 Literature review
A literature search was conducted in Web of Science and Scopus for publications that included any of the following keywords: (trawling OR dredging), (sediment OR seafloor), (organic matter OR nutrient OR organic carbon). This search produced 195 studies that spanned 1964 to 2022 (Table S1 in the Supplement). A preliminary screening process was performed using the title and abstract to assess whether the articles studied the effects of sediment disturbance by demersal fishing gear on biogeochemical properties of the seafloor. This preliminary screening discarded 129 studies, either because they were not studying the impact of fishing (87), they were studying the impact of demersal fishing but not on the seafloor (16), or they did not address its effects on sediment biogeochemistry or sedimentology (12). Additional studies were discarded when they attributed demersal fishing as a possible explanation of their results but did not study its effects directly (12) or when the article could not be accessed (2) (Table S1). Only studies with empirical data obtained from sampling and consequent analyses of sediments exposed to demersal fisheries were included in the database. Hence, data obtained from modelling or manipulation experiments in the laboratory were excluded.
From the 66 publications that passed this preliminary screening, only the studies that complied with the following inclusion criteria were retained: (i) studies sampled fished and control sites and/or before and after the fishing disturbance or fishing grounds with different fishing intensities (e.g. gradient studies), (ii) studies provided data of sediment geochemical variables (Table S2), and (iii) studies presented novel data. This last inclusion criterion was added because several studies belong to the same project (e.g. INTERPOL project (Polymenakou et al., 2005; Pusceddu et al., 2005a); RESPONSE project (Cartes et al., 2007; De Juan and Cartes, 2011; Palanques et al., 2014)) and often present duplicate results that could introduce bias to statistical analyses. This second screening process led to 51 publications that were included in the database (Table 1).
If a publication presented data for multiple independent studies, they were separated into several independent studies. This was the case if a publication included different study designs (e.g. Brown et al., 2005), sampled in different geographical locations (e.g. Atkinson et al., 2011; McLaverty et al., 2020a; Muntadas et al., 2015; Rosli et al., 2016), sampled in different environmental settings (e.g. Sciberras et al., 2016, 2017), or compared different fishing gears (e.g. Tiano et al., 2019). This led to 71 independent studies from 51 publications. Mean, measure of variability (i.e. standard deviation, standard error, confidence interval), and sample sizes of sedimentological (e.g. grain size, dry bulk density) and biogeochemical (e.g. organic carbon (OC) content, total nitrogen (TN), oxygen consumption) variables were extracted from these 71 studies. When data were only presented as figures and not in tabular form, the authors were contacted to provide missing data, or the values were extracted using the freely accessible WebPlotDigitizer software (Rohatgi, 2022).
2.2 Data harmonization
Given the diverse configurations of individual studies and their context-specific outcomes, it is imperative to harmonize them to facilitate the comparability of findings. Harmonization was conducted for the study designs, environmental factors, and fishing descriptors.
Studies were separated into three main study designs: experimental, comparative control-impact, and comparative gradient studies. Experimental studies are those where areas were experimentally disturbed using a specific fishing gear. These either consisted of collecting samples within and outside an experimentally fished area (control-impact study, CI), before and after an area was experimentally fished (before–after study, BA), or a combination of both (before–after–control-impact study, BACI). Comparative control-impact studies were those where an area known to be fished and at least one undisturbed control site with similar environmental conditions were sampled. Finally, comparative gradient studies collected samples in several sites exposed to different fishing intensities to assess the effect of fishing effort on sediment biogeochemistry.
Table 2Demersal fishing gear types included in DISOM.
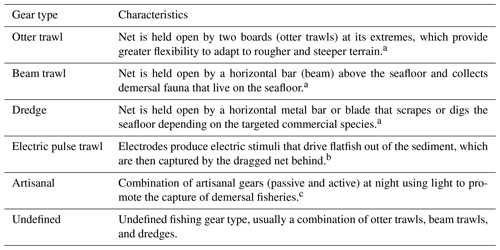
a Martín et al. (2014b). b Tiano et al. (2019). c Silveira et al. (2020).
Categories of environmental factors such as habitat type (sediment type) and seasonality were standardized across all studies. Two different habitat types were defined based on the sedimentological properties of the sites: “muddy” if the percentage of mud (sediment grain size <63 µm) was higher than that of sand or “sandy” if the percentage of sand was higher than mud. If this information was not available, we extracted this classification based on the paper's description or from an associated bibliography of the study area. Seasons varied throughout the different climatic zones encompassed in this database. Although local variations in seasons may exist, temperate zones are usually characterized by four seasons (winter, spring, summer, autumn), and tropical zones usually have two main contrasting seasons (wet and dry); the dataset was harmonized into these different categories.
Regarding the metadata associated with fishing activity, we included several parameters of interest: fishing gear type, fishing effort, time since first and last disturbance, and if the site had been historically fished. Fishing gear type was classified into six main categories: otter trawls, beam trawls, dredges, electric pulse trawl, artisanal fishing gears, and undefined fishing gears (Table 2). If a study did not specify what type of fishing technique was used, it was extracted from other studies or technical reports from the area, and if this information could not be obtained anywhere, it was assigned as “undefined”. While this classification groups together gears with similar properties, it may mask the nuances of the degree of impact each specific fishing gear could have. Hence, we advise future studies to specify, in addition to this general gear type, the model, dimensions, and the weight of the gear type as well as the targeted commercial species, all of which could affect the magnitude of the impacts.
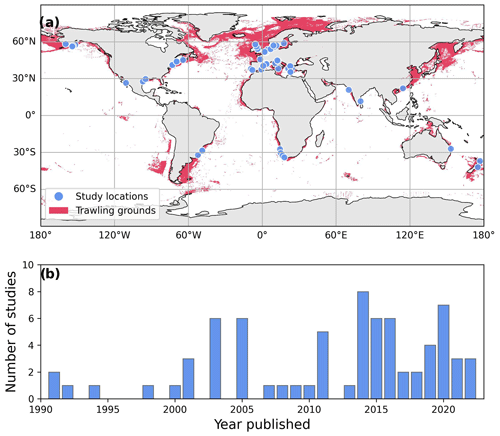
Figure 2Spatial (a) and temporal (b) distribution of number of studies and publications that have addressed the impacts of demersal fishing on sediment biogeochemistry and sedimentology. Fishing grounds were extracted from Global Fishing Watch v.2.0 (Kroodsma et al., 2018). Note that Global Fishing Watch does not distinguish between demersal and pelagic trawling, so this spatial extent should be used informatively.
Fishing effort was defined inconsistently among studies: some reported fishing effort as the density of fishing vessels (Trimmer et al., 2005) and others as the number of hauls per surface area (Paradis et al., 2021) or as the presence of trawl tracks using side-scan sonars (Muntadas et al., 2015). Whenever possible, we harmonized fishing effort to fishing frequency (yr−1), as adopted in several meta-analyses of fishing disturbance (Hiddink et al., 2017; Sciberras et al., 2018). This unit is equivalent to swept area ratio (km2 km−2 yr−1), often employed in fishery studies, and is representative of the number of disturbances that the site is experiencing. In the case of experimental studies, we harmonized the data to the number of times the entire experimental area was fished prior to sampling, considering only the known fishing disturbance by the experimental setup and ignoring fishing effort by commercial demersal fisheries in the area, if applicable. In the case of comparative control-impact and gradient studies, ∼75 % of the studies reported fishing intensity as fishing frequency, and in the remaining cases it was calculated by multiplying the number of hours fished by the speed and the width of the fishing gear, following the methods described by Hiddink et al. (2017).
To account for variations of the cumulative effect of demersal fishing disturbance on the seafloor, as well as the recovery of fishing grounds, additional fishing-related metadata attributes were extracted. For instance, time since fishing can be used to assess the recovery of fishing grounds after its disturbance and is often reported in experimental study designs as part of their research objectives (Brylinsky et al., 1994; Falcão et al., 2003; Silveira et al., 2020). This variable can not only be used for the impacted site but also for control sites. Several studies used areas that have been fished in the past but have not been disturbed recently due to, for example, a temporary ban (Dannheim et al., 2014) or the recent establishment of a marine protected area (MPA; Brown et al., 2005; Sparks-McConkey and Watling, 2001) as their control site, whereas some studies were able to identify areas that had never been fished as their control sites. To account for these contrasting control sites, we extracted the time since fishing, if available, and created an additional column “Historically fished” that classifies whether a site had been fished in the past or not. To account for the cumulative impact of sediment disturbance (De Borger et al., 2021), we also extracted how much time had elapsed since the first disturbance (i.e. when fishing in the area had first begun), and, if this information was not available, a related bibliography describing historical fishing activity of the area was consulted.
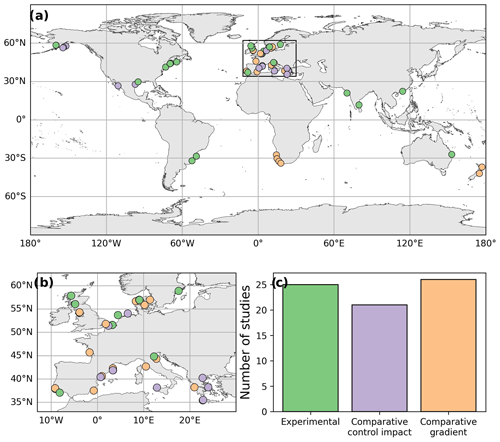
Figure 3Spatial distribution of study designs on global continental margins (a) and on European margins (b) in DISOM. Summary of the different study designs in the dataset (c).
Finally, the different response variables were harmonized, and complementary variables were calculated when possible (Table 3). For instance, although some studies provided a more precise classification of different sand fractions (e.g. fine sand or medium sand; Ramalho et al., 2018; Silveira et al., 2020), the majority of studies simply presented total sand fractions (grain size >63 µm) (Sciberras et al., 2017; Simboura et al., 2008), and these were calculated whenever possible.
Since each study analyses specific variables and reports different information, it would not be efficient to store all the data in a single spreadsheet. Therefore, a relational database was constructed to optimize the data storage capacity, where each table includes data from different categories that are linked together based on identifiers (i.e. primary and foreign keys such as study_id and sample_id) (Fig. 1). These different tables are as follows: study_description, sampling_locations, and response_variables.
The first table, “study_description”, stores information related to the publication, study area, and study design (Table S3). Since each publication can have several independent studies based on the study design or study area (see Sect. 2.2), a unique identifier is given to each independent study.
The second table, “sampling_location”, stores metadata associated with the sampling site such as its coordinates, sampling date, habitat type, seasonality, climate zone, and other variables related to fishing activity (e.g. fishing gear, fishing intensity, time since disturbance) which would help discern the effect of demersal fisheries (Table S4).
The third table, “response_variables”, stores the different sedimentological (i.e. grain size and porosity) and biogeochemical (i.e. OC, nitrogen, phytopigment, nutrient fluxes) variables measured for each individual study and sampling location, as well as the sample's metadata, such as sediment sectioning and how the data were collected (Table S5).
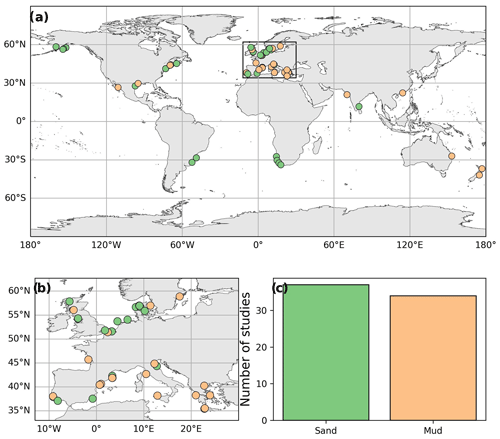
Figure 4Spatial distribution of habitat types on global continental margins (a) and on European margins (b) in DISOM. Summary of the different habitat types in the dataset (c).
4.1 Spatiotemporal coverage of the data
This database compiled data from 71 studies that covered a total of 907 independent locations where a series of sedimentological and biogeochemical proxies were analysed to assess the impacts of demersal fisheries. The majority of the 71 studies included in the database were conducted in European waters, namely in the Mediterranean Sea (18) and in north European seas (e.g. North Sea, Irish Sea, Baltic Sea) (21) (Fig. 2a). Several studies were conducted in North America (15), mainly in the eastern margin, as well as along the Alaskan margin, the Gulf of Mexico, and the Gulf of California (Fig. 2a). Few studies were conducted in the Atlantic Iberian margin (4), southeastern African margin (4), India (2), Brazil (3), New Zealand (2), Australia (1), and east Asia (1) (Fig. 1a). Despite the wide extension of fishing grounds along the southwestern South American and the east Asian margins, only two studies were identified in these widespread fished margins. Although demersal fisheries occur in all continental margins, we did not find peer-reviewed studies on trawling impacts and biogeochemistry along western South America and western Canada, nor along the western North African margin or the eastern Russian margin (Fig. 2a).
The first reported studies that assessed the impacts of bottom trawling on sediment biogeochemistry were published in the 1990s, with five independent studies during that decade (Fig. 2b). An increase in the number of studies was observed in the following decade, with 18 independent studies published between 2000 and 2010, whereas 35 studies were published during the last decade (2010–2020). In the last few years (2020–2022), we have identified 13 studies that have addressed the impacts of demersal fisheries on biogeochemistry, which indicates a growing interest in understanding whether and how demersal fisheries may be altering biogeochemical cycles.
4.2 Distribution of study designs
The distribution of study designs stored in DISOM is relatively equal, with a similar representation of experimental (25), comparative control-impact (20), and comparative gradient (26) studies (Fig. 3). Studies conducted on the European continental margins include an equal number of the three study designs (Fig. 3). In North America, experimental and comparative control-impact studies have been conducted equally, but no comparative gradient studies were carried out (Fig. 3). Studies conducted in South America, Africa, Asia, and Oceania only presented one typology of study design (Fig. 3). Future studies should be carried out with all three of the typologies of study designs in order to deepen the knowledge of acute and chronic impacts and the effects of different fishing intensity on organic matter in these regions.
4.3 Distribution of environmental conditions
Studies of the effects of different bottom fishing gear in different habitat types on sediment biogeochemistry are essential to understand fishing impacts using global models. The number of independent studies in sandy and muddy habitats is equally represented in DISOM, with 37 and 34 independent studies in sandy and muddy habitat types, respectively (Fig. 4). However, except for the European and American margin, there is an unequal spatial distribution of the studies on sandy and muddy habitats: Brazilian and southwest African margins only presented studies on sandy environments whereas Chinese, Australian, and New Zealand margins only had studies on muddy areas of the seafloor (Fig. 4).
The majority of studies were conducted in shallow environments, at <50 m depth, whereas few studies were conducted at deeper environments (>200 m) (Fig. 5). The highly skewed nature of the dataset towards shallow environments should be considered when modelling the effects of demersal fisheries, especially considering the continuous expansion of fishing grounds to deeper environments (Norse et al., 2012; Watson and Morato, 2013).
Finally, one of the factors that should be considered when studying the impacts of demersal fisheries is the sampling season, since the impacts caused by this anthropogenic activity could be masked if they coexist with seasonal storms or cycles of high net primary productivity. For instance, seasonal storms can resuspend as much sediment and particulate organic carbon as bottom trawling (Arjona-Camas et al., 2021, 2022; Durrieu de Madron et al., 2005; Ferré et al., 2008; Mengual et al., 2016; Paradis et al., 2022; Pusceddu et al., 2005b, 2015), whereas seasonal variations in organic matter inputs to the seafloor could offset the effects of trawling (Daly et al., 2018; Rajesh et al., 2019; Smith et al., 2000). Although several studies (20) conducted their sampling during several seasons to account for the effect of seasonality, the majority of the studies included in DISOM only sampled during a specific season, usually during summer (30), which could skew the global results when assessing the impacts of demersal fisheries on sediment biogeochemistry (Fig. 6).
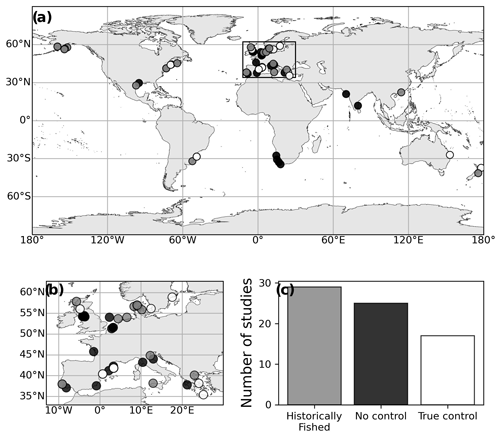
Figure 8Spatial distribution of presence of a control site for each study on global continental margins (a) and on European margins (b) in DISOM. Summary of the different types of controls in the dataset (c).
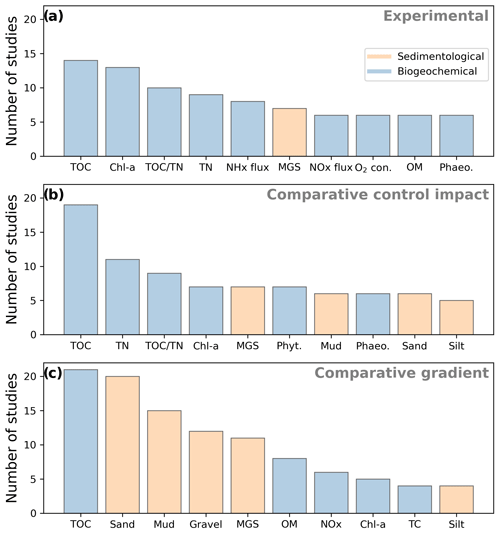
Figure 9Number of studies that analysed each response variable in each of the three study designs: (a) experimental, (b) comparative control-impact, and (c) comparative gradient studies. For clarity, only the 10 most common response variables were included, and variables were grouped based on the property they represent (sedimentological or biogeochemical). A full description of the different biogeochemical and sedimentological variables can be found in Table S2.
4.4 Distribution of fishing descriptors
Although we harmonized the different fishing gear types, it is important to note that there is a wide variety of configurations for each gear type with different effects on the seafloor (Martín et al., 2014b). For instance, the size and weight of otter trawls tend to increase with the water depth at the fishing ground, so an otter trawl that operates in shallow environments will be different than one that operates at greater depths (Ragnarsson and Steingrimsson, 2003).
In this dataset, there was an unequal distribution of demersal fishing gear types, which could skew statistical analyses. The most common fishing gear type identified was otter trawls (39), followed by towed dredges (18) and beam trawls (7) (Fig. 7). The type of fishing gear was sometimes undefined in certain studies (7). In these situations, a literature search indicated that demersal fishing fleets consisted of a mixture of otter trawls, dredges, and beam trawls (Fig. 7). The effects of electric pulse trawls and artisanal gears were only assessed by one study each, and these were spatially limited: the study on the electric pulse trawl technique was only conducted in the North Sea, while artisanal gears were only studied in southern Brazil (Fig. 7).
From the 71 independent studies, ∼75 % did not have a true control site (i.e. where bottom fishing was completely absent) to compare the effect of this anthropogenic disturbance in relation to natural baseline conditions (Fig. 8). Either these studies did not have any control site (no control), or their control site had been disturbed in the past (historically fished) and may not have recovered completely to its baseline conditions.
Studies with no true control site were either experimental studies that sampled before and after disturbance in an impacted site (Bhagirathan et al., 2010; Tiano et al., 2021) or were comparative gradient studies that sampled in areas with different fishing intensities (Atkinson et al., 2011; Hale et al., 2017; Sciberras et al., 2016). Considering that demersal fishing grounds essentially cover all continental margins, it is not surprising that a representative control site with similar environmental conditions to the disturbed site is most often not available (Fig. 8). Indeed, only 17 independent studies had true control sites due to specific conditions: (i) experimental studies were conducted in marine protected areas that had never been fished (Ferguson et al., 2020; Morys et al., 2021; Tuck et al., 1998); (ii) control sites were far from harbours, and fishers still had not explored those areas (Paradis et al., 2019; Simboura et al., 2008; Watling et al., 2001); or (iii) specific features prevented the access of fishing vessels to certain areas (Cartes et al., 2007; Martín et al., 2014a; Palanques et al., 2014; Paradis et al., 2021).
To overcome this lack of true controls, certain studies chose sites that were not currently disturbed but had been historically fished as pseudo-control sites (“Historically fished” in Fig. 8). These sites were not being disturbed during the study because they were conducted during a seasonal fishing closure (Fiordelmondo et al., 2003; Meseck et al., 2014; Polymenakou et al., 2005; Pusceddu et al., 2005a; Silveira et al., 2020; Tiano et al., 2019) or because the site had been recently banned from fishing activities (Brown et al., 2005; Dannheim et al., 2014). However, since these pseudo-controls had been disturbed by demersal fisheries in the past, they may not reflect true baseline conditions, which could affect the outcomes of the study. For instance, a recent global meta-analysis on the effects of demersal fisheries on benthic communities revealed that the history of fishing disturbance prior to an experimental study influenced the outcome of the result, masking the impacts in comparison to studies on undisturbed sites (Sciberras et al., 2018).
Finally, only 45 studies provided information on fishing frequency, which eventually will limit the possibility to use these data to fully understand how fishing frequency affects the magnitude of the sedimentological or biogeochemical parameter measured. The majority of the 26 studies that did not include fishing frequency are comparative control-impact studies that designed their studies to compare the biogeochemical properties between impacted and control sites, without considering the influence of fishing frequency. In addition, many of these studies were conducted prior to the installation of geographical positioning systems in fishing vessels, such as an automatic identification system (AIS) or vessel monitoring system (VMS), which facilitate the estimation of fishing frequency over an area. With the increasing availability of harmonized fishing frequency data such as through the efforts of Global Fishing Watch, we will be able to include this information without the need to harmonize fishing effort.
4.5 Response variables
We identified 90 different sedimentological and biogeochemical variables reported in all studies, and the 10 most reported ones are shown in Fig. 9. These are separated based on the different study designs (experimental, comparative control-impact, and comparative gradient studies) and the type of information they provide (sedimentological and biogeochemical). Unsurprisingly, the most widely measured response variable for all study designs was total organic carbon (TOC) (Fig. 9). Total nitrogen and proxies of algal material (e.g. chlorophyll a, phaeopigments, and the total phytopigment concentration), which provide a useful measure of the fishing effect on the nutritional quality of organic matter (i.e. its food availability for the benthos; Pusceddu et al., 2009), were commonly reported in both experimental and comparative control-impact study designs (Fig. 9). However, other organic compounds such as proteins, lipids, and lignin phenols were not sufficiently represented across studies, hindering the capacity to study the effects of demersal fisheries on a wider range of organic compounds with different reactivities and origins.
The effect of sediment disturbance on sedimentological variables, such as grain size, was represented in all study designs, but these variables were more prominent in comparative gradient studies.
Biogeochemical variables which measure remineralization processes (e.g. nutrient concentrations and fluxes, as well as oxygen consumption) were more commonly reported in manipulative and comparative gradient studies than in comparative control-impact ones (Fig. 9). The unbalanced representation of these variables among study designs may bias our understanding of the effect of fishing disturbance on remineralization processes in marine sediments, which has become a matter of debate when modelling the amount of CO2 emitted from the seafloor due to this sediment disturbance (Sala et al., 2021; Hiddink et al., 2023). Hence, more studies that assess the effect on organic matter remineralization should be performed.
4.6 General remarks
As illustrated in the Sankey diagram (Fig. 10), each individual study in this compilation has a unique combination of environmental conditions (e.g. habitats, depths, and seasonality) and demersal fishing descriptors (e.g. fishing gear type, presence of control site, information on fishing effort), which need to be considered when comparing their results. For instance, the majority of the comparative gradient studies did not have any control site given the difficulty of finding a representative control site in the area under scrutiny. Given their nature, beam trawls were almost exclusively used in sandy environments, and no comparative gradient study was performed using this gear. Hence, researchers that aim to use this database should take into account that the heterogeneity of the large number of influential variables (i.e. study design, environmental conditions, fishing descriptors) will reduce the degrees of freedom needed to conduct meaningful statistical analyses.
These unique combinations of study design, environmental conditions, and fishing descriptors (Fig. 10) may limit what is currently known on the effect of demersal fishery on biogeochemical processes on the seafloor, producing contrasting results in their biogeochemical effects on the seafloor (Epstein et al., 2022). The reasons behind these contrasting results are beyond the scope of this paper and should be addressed in a thorough meta-analysis (Tiano et al., 2024).
While this database only gathered data from studies published in peer-reviewed papers, there is a wealth of information available in grey literature that could further improve our understanding of the effect of demersal fisheries on global biogeochemical processes in marine sediments. Moreover, future versions of this database should incorporate data of benthic community abundance and composition, since alterations of benthic communities can also affect the biogeochemical and sedimentological properties of the seafloor. Finally, considering that demersal fisheries modify sedimentary dynamics in the water column and the composition of suspended sediment and nutrients in the overlying water column, these effects should be included to properly understand the global effect of demersal fisheries on biogeochemical cycles in the marine realm. As the number of these studies increases, DISOM will be expanded to include studies that address the effect of demersal fisheries on the water column.
The database is available for download as an Excel file, where each separate sheet stores the individual tables (Paradis, 2023; https://doi.org/10.3929/ethz-b-000634336).
DISOM is the first comprehensive open-access database that compiles and harmonizes data of sedimentological and biogeochemical variables of peer-reviewed studies that address the effects of demersal fisheries on the seafloor. We also present important metadata on environmental and fishing descriptors that need to be reported to ensure the comparability between studies and facilitate holistic meta-analyses. While this database harmonizes data from 71 independent studies located in a wide variety of continental margins, we have identified geographical gaps along the African, South American, Canadian, and Asian margins. Studies presented an unbalanced seasonal sampling strategy, with the majority of studies conducted during summer in temperate regions. Regarding fishing descriptors, we highlight the scarcity of true controls given the global extension of demersal fishing grounds as well as the unbalanced distribution of demersal fishing gear types studied. Finally, our comprehension of the impact of demersal fishing disturbance on organic matter remineralization may be constrained by the limited availability of studies quantifying these variables. Hence, more studies should be conducted considering these data gaps to obtain a more comprehensive dataset. Given the complexity of influential variables, researchers that aim to use this harmonized dataset to understand the biogeochemical impacts of demersal fisheries should account for the heterogeneity of study designs, environmental conditions, and fishing descriptors.
The supplement related to this article is available online at: https://doi.org/10.5194/essd-16-3547-2024-supplement.
SP performed the first screening step, while all authors performed the second screening step. All authors devised a methodology to objectively extract the data and compiled the data of assigned manuscripts. SP harmonized the data across studies. SP wrote the manuscript with the contribution of all co-authors.
The contact author has declared that none of the authors has any competing interests.
Publisher's note: Copernicus Publications remains neutral with regard to jurisdictional claims made in the text, published maps, institutional affiliations, or any other geographical representation in this paper. While Copernicus Publications makes every effort to include appropriate place names, the final responsibility lies with the authors.
We would like to acknowledge Albert Palanques, Ciarán McLaverty, Rachel Hale, Irini Tsikopoulou, Mark Trimmer, Christopher J. Smith, and Lawrence M. Mayer, who provided data in tabular format when they were not available in the publications.
Marija Sciberras was supported by the Children's Investment Fund Foundation (grant no. 2206-07755 (Ocean Carbon DA)).
This paper was edited by François G. Schmitt and reviewed by Craig Smeaton and three anonymous referees.
Amoroso, R. O., Pitcher, C. R., Rijnsdorp, A. D., McConnaughey, R. A., Parma, A. M., Suuronen, P., Eigaard, O. R., Bastardie, F., Hintzen, N. T., Althaus, F., Baird, S. J., Black, J., Buhl-Mortensen, L., Campbell, A. B., Catarino, R., Collie, J., Cowan, J. H., Durholtz, D., Engstrom, N., Fairweather, T. P., Fock, H. O., Ford, R., Gálvez, P. A., Gerritsen, H., Góngora, M. E., González, J. A., Hiddink, J. G., Hughes, K. M., Intelmann, S. S., Jenkins, C., Jonsson, P., Kainge, P., Kangas, M., Kathena, J. N., Kavadas, S., Leslie, R. W., Lewis, S. G., Lundy, M., Makin, D., Martin, J., Mazor, T., Gonzalez-Mirelis, G., Newman, S. J., Papadopoulou, N., Posen, P. E., Rochester, W., Russo, T., Sala, A., Semmens, J. M., Silva, C., Tsolos, A., Vanelslander, B., Wakefield, C. B., Wood, B. A., Hilborn, R., Kaiser, M. J., and Jennings, S.: Bottom trawl fishing footprints on the world's continental shelves., P. Natl. Acad. Sci. USA, 115(43), E10275–E10282, https://doi.org/10.1073/pnas.1802379115, 2018.
Arjona-Camas, M., Puig, P., Palanques, A., Durán, R., White, M., Paradis, S., and Emelianov, M.: Natural vs. trawling-induced water turbidity and suspended sediment transport variability within the Palamós Canyon (NW Mediterranean), Mar. Geophys. Res., 42, 38, https://doi.org/10.1007/s11001-021-09457-7, 2021.
Arjona-Camas, M., Puig, P., De Leo, F. C., Garner, G., Paradis, S., Durán, R., and Palanques, A.: Influence of Natural Processes and Bottom Trawling in the Nepheloid Layer Structure Off Vancouver Island (British Columbia, Canada, NE Pacific), Front. Mar. Sci., 8, 770280, https://doi.org/10.3389/fmars.2021.770280, 2022.
Atkinson, L., Field, J., and Hutchings, L.: Effects of demersal trawling along the west coast of southern Africa: multivariate analysis of benthic assemblages, Mar. Ecol. Prog. Ser., 430, 241–255, https://doi.org/10.3354/meps08956, 2011.
Bhagirathan, U., Meenakumari, B., Jayalakshmy, K. V., Panda, S. K., Madhu, V. R., and Vaghela, D. T.: Impact of bottom trawling on sediment characteristics-a study along inshore waters off Veraval coast, India, Environ. Monit. Assess., 160, 355–369, https://doi.org/10.1007/s10661-008-0700-0, 2010.
Black, K. E., Smeaton, C., Turrell, W. R., and Austin, W. E. N.: Assessing the potential vulnerability of sedimentary carbon stores to bottom trawling disturbance within the UK EEZ, Front. Mar. Sci., 9, 892892, https://doi.org/10.3389/fmars.2022.892892, 2022.
Brown, E. J., Finney, B., Dommisse, M., and Hills, S.: Effects of commercial otter trawling on the physical environment of the southeastern Bering Sea, Cont. Shelf Res., 25, 1281–1301, https://doi.org/10.1016/j.csr.2004.12.005, 2005.
Brylinsky, M., Gibson, J., and Gordon Jr., D. C.: Impacts of Flounder Trawls on the Intertidal Habitat and Community of the Minas Basin, Bay of Fundy, Can. J. Fish. Aquat. Sci., 51, 650–661, https://doi.org/10.1139/f94-066, 1994.
Burdige, D. J.: Preservation of Organic Matter in Marine Sediments: Controls, Mechanisms, and an Imbalance in Sediment Organic Carbon Budgets?, Chem. Rev., 107, 467–485, https://doi.org/10.1021/CR050347Q, 2007.
Cartes, J. E., Papiol, V., Palanques, A., Guillén, J., and Demestre, M.: Dynamics of suprabenthos off the Ebro Delta (Catalan Sea: western Mediterranean): Spatial 13 and temporal patterns and relationships with environmental factors, Estuar. Coast. Shelf Sci., 75, 501–515, https://doi.org/10.1016/j.ecss.2007.05.047, 2007.
Costa, K. G. and Netto, S. A.: Effects of small-scale trawling on benthic communities of estuarine vegetated and non-vegetated habitats, Biodivers. Conserv., 23, 1041–1055, https://doi.org/10.1007/s10531-014-0652-3, 2014.
Daly, E., Johnson, M. P., Wilson, A. M., Gerritsen, H. D., Kiriakoulakis, K., Allcock, A. L., and White, M.: Bottom trawling at Whittard Canyon: Evidence for seabed modification, trawl plumes and food source heterogeneity, Prog. Oceanogr., 169, 227–240, https://doi.org/10.1016/j.pocean.2017.12.010, 2018.
Dannheim, J., Brey, T., Schröder, A., Mintenbeck, K., Knust, R., and Arntz, W. E.: Trophic look at soft-bottom communities – Short-term effects of trawling cessation on benthos, J. Sea Res., 85, 18–28, https://doi.org/10.1016/j.seares.2013.09.005, 2014.
De Borger, E., Tiano, J., Braeckman, U., Rijnsdorp, A. D., and Soetaert, K.: Impact of bottom trawling on sediment biogeochemistry: a modelling approach, Biogeosciences, 18, 2539–2557, https://doi.org/10.5194/bg-18-2539-2021, 2021.
De Juan, S. and Cartes, J. E.: Influence of environmental factors on the dynamics of macrobenthic crustaceans on soft-bottoms of the Ebro Delta continental shelf (northwestern Mediterranean), Sci. Mar., 75, 691–700, https://doi.org/10.3989/scimar.2011.75n4691, 2011.
Depestele, J., Degrendele, K., Esmaeili, M., Ivanović, A., Kröger, S., O'Neill, F. G., Parker, R., Polet, H., Roche, M., Teal, L. R., Vanelslander, B., and Rijnsdorp, A. D.: Comparison of mechanical disturbance in soft sediments due to tickler-chain SumWing trawl vs. electro-fitted PulseWing trawl, edited by: M. Pol, ICES J. Mar. Sci., 76, 312–329, https://doi.org/10.1093/icesjms/fsy124, 2019.
Dolmer, P., Kristensen, T., Christiansen, M. L., Petersen, M. F., Kristensen, P. S., and Hoffmann, E.: Short-term impact of blue mussel dredging (Mytilus edulis L.) on a benthic community, Hydrobiologia, 465, 115–127, https://doi.org/10.1023/A:1014549026157, 2001.
Durrieu de Madron, X., Ferré, B., Le Corre, G., Grenz, C., Conan, P., Pujo-Pay, M., Buscail, R., and Bodiot, O.: Trawling-induced resuspension and dispersal of muddy sediments and dissolved elements in the Gulf of Lion (NW Mediterranean), Cont. Shelf Res., 25, 2387–2409, https://doi.org/10.1016/j.csr.2005.08.002, 2005.
Eleftheriou, A. and Robertson, M. R.: The effects of experimental scallop dredging on the fauna and physical environment of a shallow sandy community, Netherlands J. Sea Res., 30, 289–299, https://doi.org/10.1016/0077-7579(92)90067-O, 1992.
Epstein, G. and Roberts, C. M.: Identifying priority areas to manage mobile bottom fishing on seabed carbon in the UK, edited by: Nagai, R. H., PLOS Clim., 1, e0000059, https://doi.org/10.1371/journal.pclm.0000059, 2022.
Epstein, G., Middelburg, J. J., Hawkins, J. P., Norris, C. R., and Roberts, C. M.: The impact of mobile demersal fishing on carbon storage in seabed sediments, Glob. Chang. Biol., 28, 2875–2894, https://doi.org/10.1111/gcb.16105, 2022.
Falcão, M., Gaspar, M., Caetano, M., Santos, M., and Vale, C.: Short-term environmental impact of clam dredging in coastal waters (south of Portugal): chemical disturbance and subsequent recovery of seabed, Mar. Environ. Res., 56, 649–664, https://doi.org/10.1016/S0141-1136(03)00069-2, 2003.
Ferguson, A. J. P., Oakes, J., and Eyre, B. D.: Bottom trawling reduces benthic denitrification and has the potential to influence the global nitrogen cycle, Limnol. Oceanogr. Lett., 5, lol2.10150, https://doi.org/10.1002/lol2.10150, 2020.
Ferré, B., Durrieu de Madron, X., Estournel, C., Ulses, C., and Le Corre, G.: Impact of natural (waves and currents) and anthropogenic (trawl) resuspension on the export of particulate matter to the open ocean, Cont. Shelf Res., 28, 2071–2091, https://doi.org/10.1016/j.csr.2008.02.002, 2008.
Fiordelmondo, C., Manini, E., Gambi, C., and Pusceddu, A.: Short-Term Impact of Clam Harvesting on Sediment Chemistry, Benthic Microbes and Meiofauna in the Goro Lagoon (Italy), Chem. Ecol., 19, 173–187, https://doi.org/10.1080/0275754031000119924, 2003.
Goldberg, R., Rose, J. M., Mercaldo-Allen, R., Meseck, S. L., Clark, P., Kuropat, C., and Pereira, J. J.: Effects of hydraulic dredging on the benthic ecology and sediment chemistry on a cultivated bed of the Northern quahog, Mercenaria mercenaria, Aquaculture, 428–429, 150–157, https://doi.org/10.1016/j.aquaculture.2014.03.012, 2014.
Hale, R., Godbold, J. A., Sciberras, M., Dwight, J., Wood, C., Hiddink, J. G., and Solan, M.: Mediation of macronutrients and carbon by post-disturbance shelf sea sediment communities, Biogeochemistry, 135, 121–133, https://doi.org/10.1007/s10533-017-0350-9, 2017.
Hiddink, J. G., Jennings, S., Sciberras, M., Szostek, C. L., Hughes, K. M., Ellis, N., Rijnsdorp, A. D., McConnaughey, R. A., Mazor, T., Hilborn, R., Collie, J. S., Pitcher, C. R., Amoroso, R. O., Parma, A. M., Suuronen, P., and Kaiser, M. J.: Global analysis of depletion and recovery of seabed biota after bottom trawling disturbance, P. Natl. Acad. Sci. USA, 114, 8301–8306, https://doi.org/10.1073/pnas.1618858114, 2017.
Hiddink, J. G., Jennings, S., Sciberras, M., Bolam, S. G., Cambiè, G., McConnaughey, R. A., Mazor, T., Hilborn, R., Collie, J. S., Pitcher, C. R., Parma, A. M., Suuronen, P., Kaiser, M. J., and Rijnsdorp, A. D.: Assessing bottom trawling impacts based on the longevity of benthic invertebrates, edited by V. Trenkel, J. Appl. Ecol., 56, 1075–1084, https://doi.org/10.1111/1365-2664.13278, 2019.
Hiddink, J. G., van de Velde, S. J., McConnaughey, R. A., De Borger, E., Tiano, J., Kaiser, M. J., Sweetman, A. K., and Sciberras, M.: Quantifying the carbon benefits of ending bottom trawling, Nature, 617, E1–E2, https://doi.org/10.1038/s41586-023-06014-7, 2023.
Huettel, M., Berg, P., and Kostka, J. E.: Benthic Exchange and Biogeochemical Cycling in Permeable Sediments, Ann. Rev. Mar. Sci., 6, 23–51, https://doi.org/10.1146/annurev-marine-051413-012706, 2014.
Keil, R. G., Tsamakis, E., Giddings, J. C., and Hedges, J. I.: Biochemical distributions (amino acids, neutral sugars, and lignin phenols) among size-classes of modern marine sediments from the Washington coast, Geochim. Cosmochim. Ac., 62, 1347–1364, https://doi.org/10.1016/S0016-7037(98)00080-5, 1998.
Kroodsma, D. A., Mayorga, J., Hochberg, T., Miller, N. A., Boerder, K., Ferretti, F., Wilson, A., Bergman, B., White, T. D., Block, B. A., Woods, P., Sullivan, B., Costello, C., and Worm, B.: Tracking the global footprint of fisheries, Science, 359, 904–908, https://doi.org/10.1126/science.aao5646, 2018.
Lamarque, B., Deflandre, B., Galindo Dalto, A., Schmidt, S., Romero-Ramirez, A., Garabetian, F., Dubosq, N., Diaz, M., Grasso, F., Sottolichio, A., Bernard, G., Gillet, H., Cordier, M.-A., Poirier, D., Lebleu, P., Derriennic, H., Danilo, M., Murilo Barboza Tenório, M., and Grémare, A.: Spatial Distributions of Surface Sedimentary Organics and Sediment Profile Image Characteristics in a High-Energy Temperate Marine RiOMar: The West Gironde Mud Patch, J. Mar. Sci. Eng., 9, 242, https://doi.org/10.3390/jmse9030242, 2021.
Legge, O., Johnson, M., Hicks, N., Jickells, T., Diesing, M., Aldridge, J., Andrews, J., Artioli, Y., Bakker, D. C. E., Burrows, M. T., Carr, N., Cripps, G., Felgate, S. L., Fernand, L., Greenwood, N., Hartman, S., Kröger, S., Lessin, G., Mahaffey, C., Mayor, D. J., Parker, R., Queirós, A. M., Shutler, J. D., Silva, T., Stahl, H., Tinker, J., Underwood, G. J. C., Van Der Molen, J., Wakelin, S., Weston, K., and Williamson, P.: Carbon on the Northwest European Shelf: Contemporary Budget and Future Influences, Front. Mar. Sci., 7, 143, https://doi.org/10.3389/fmars.2020.00143, 2020.
Liu, X.-S., Xu, W.-Z., Cheung, S. G., and Shin, P. K. S.: Response of meiofaunal community with special reference to nematodes upon deployment of artificial reefs and cessation of bottom trawling in subtropical waters, Hong Kong, Mar. Pollut. Bull., 63, 376–384, https://doi.org/10.1016/j.marpolbul.2010.11.019, 2011.
Martín, J., Puig, P., Masqué, P., Palanques, A., and Sánchez-Gómez, A.: Impact of bottom trawling on deep-sea sediment properties along the flanks of a submarine canyon, PLoS One, 9, e104536, https://doi.org/10.1371/journal.pone.0104536, 2014a.
Martín, J., Puig, P., Palanques, A., and Giamportone, A.: Commercial bottom trawling as a driver of sediment dynamics and deep seascape evolution in the Anthropocene, Anthropocene, 7, 1–15, https://doi.org/10.1016/j.ancene.2015.01.002, 2014b.
Mayer, L. M.: Surface area control of organic carbon accumulation in continental shelf sediments, Geochim. Cosmochim. Ac., 58, 1271–1284, https://doi.org/10.1016/0016-7037(94)90381-6, 1994.
Mayer, L. M., Schick, D. F., Findlay, R. H., and Rice, D. L.: Effects of commercial dragging on sedimentary organic matter, Mar. Environ. Res., 31, 249–261, https://doi.org/10.1016/0141-1136(91)90015-Z, 1991.
McLaverty, C., Eigaard, O., Dinesen, G., Gislason, H., Kokkalis, A., Erichsen, A., and Petersen, J.: High-resolution fisheries data reveal effects of bivalve dredging on benthic communities in stressed coastal systems, Mar. Ecol. Prog. Ser., 642, 21–38, https://doi.org/10.3354/meps13330, 2020a.
McLaverty, C., Eigaard, O. R., Gislason, H., Bastardie, F., Brooks, M. E., Jonsson, P., Lehmann, A., and Dinesen, G. E.: Using large benthic macrofauna to refine and improve ecological indicators of bottom trawling disturbance, Ecol. Indic., 110, 105811, https://doi.org/10.1016/j.ecolind.2019.105811, 2020b.
Mengual, B., Cayocca, F., Le Hir, P., Draye, R., Laffargue, P., Vincent, B., and Garlan, T.: Influence of bottom trawling on sediment resuspension in the `Grande-Vasière' area (Bay of Biscay, France), Ocean Dynam., 66, 1181–1207, https://doi.org/10.1007/s10236-016-0974-7, 2016.
Meseck, S. L., Mercaldo-Allen, R., Rose, J. M., Clark, P., Kuropat, C., Pereira, J. J., and Goldberg, R.: Effects of Hydraulic Dredging for Mercenaria mercenaria, Northern Quahog, on Sediment Biogeochemistry, J. World Aquac. Soc., 45, 301–311, https://doi.org/10.1111/jwas.12114, 2014.
Morys, C., Brüchert, V., and Bradshaw, C.: Impacts of bottom trawling on benthic biogeochemistry in muddy sediments: Removal of surface sediment using an experimental field study, Mar. Environ. Res., 169, 105384, https://doi.org/10.1016/j.marenvres.2021.105384, 2021.
Muntadas, A., de Juan, S., and Demestre, M.: Integrating the provision of ecosystem services and trawl fisheries for the management of the marine environment, Sci. Total Environ., 506–507, 594–603, https://doi.org/10.1016/j.scitotenv.2014.11.042, 2015.
Norse, E. A., Brooke, S., Cheung, W. W. L., Clark, M. R., Ekeland, I., Froese, R., Gjerde, K. M., Haedrich, R. L., Heppell, S. S., Morato, T., Morgan, L. E., Pauly, D., Sumaila, R., and Watson, R.: Sustainability of deep-sea fisheries, Mar. Policy, 36, 307–320, https://doi.org/10.1016/J.MARPOL.2011.06.008, 2012.
Oberle, F. K. J., Puig, P., and Martín, J.: Fishing Activities, in Submarine Geomorphology, edited by: Micallef, A., Krastel, S., and Savini, A., 503–534, Springer, Cham., 2018.
Palanques, A., Puig, P., Guillén, J., Demestre, M., and Martín, J.: Effects of bottom trawling on the Ebro continental shelf sedimentary system (NW Mediterranean), Cont. Shelf Res., 72, 83–98, https://doi.org/10.1016/j.csr.2013.10.008, 2014.
Paradis, S.: Demersal fishery Impacts on Sedimentary Organic Matter (DISOM), ETH Zürich [data set], https://doi.org/10.3929/ethz-b-000634336, 2023.
Paradis, S., Pusceddu, A., Masqué, P., Puig, P., Moccia, D., Russo, T., and Lo Iacono, C.: Organic matter contents and degradation in a highly trawled area during fresh particle inputs (Gulf of Castellammare, southwestern Mediterranean), Biogeosciences, 16, 4307–4320, https://doi.org/10.5194/bg-16-4307-2019, 2019.
Paradis, S., Goñi, M., Masqué, P., Durán, R., Arjona-Camas, M., Palanques, A., and Puig, P.: Persistence of Biogeochemical Alterations of Deep-Sea Sediments by Bottom Trawling, Geophys. Res. Lett., 48, e2020GL091279, https://doi.org/10.1029/2020GL091279, 2021.
Paradis, S., Arjona-Camas, M., Goñi, M., Palanques, A., Masqué, P., and Puig, P.: Contrasting particle fluxes and composition in a submarine canyon affected by natural sediment transport events and bottom trawling, Front. Mar. Sci., 9, 1017052, https://doi.org/10.3389/fmars.2022.1017052, 2022.
Polymenakou, P. N., Pusceddu, A., Tselepides, A., Polychronaki, T., Giannakourou, A., Fiordelmondo, C., Hatziyanni, E., and Danovaro, R.: Benthic microbial abundance and activities in an intensively trawled ecosystem (Thermaikos Gulf, Aegean Sea), Cont. Shelf Res., 25, 2570–2584, https://doi.org/10.1016/J.CSR.2005.08.018, 2005.
Pusceddu, A., Fiordelmondo, C., Polymenakou, P., Polychronaki, T., Tselepides, A., and Danovaro, R.: Effects of bottom trawling on the quantity and biochemical composition of organic matter in coastal marine sediments (Thermaikos Gulf, northwestern Aegean Sea), Cont. Shelf Res., 25, 2491–2505, https://doi.org/10.1016/j.csr.2005.08.013, 2005a.
Pusceddu, A., Grémare, A., Escoubeyrou, K., Amouroux, J. M., Fiordelmondo, C., and Danovaro, R.: Impact of natural (storm) and anthropogenic (trawling) sediment resuspension on particulate organic matter in coastal environments, Cont. Shelf Res., 25, 2506–2520, https://doi.org/10.1016/j.csr.2005.08.012, 2005b.
Pusceddu, A., Dell'Anno, A., Fabiano, M., and Danovaro, R.: Quantity and bioavailability of sediment organic matter as signatures of benthic trophic status, Mar. Ecol. Prog. Ser., 375, 41–52, https://doi.org/10.3354/meps07735, 2009.
Pusceddu, A., Bianchelli, S., Martin, J., Puig, P., Palanques, A., Masque, P., and Danovaro, R.: Chronic and intensive bottom trawling impairs deep-sea biodiversity and ecosystem functioning, P. Natl. Acad. Sci. USA, 111, 8861–8866, https://doi.org/10.1073/pnas.1405454111, 2014.
Pusceddu, A., Bianchelli, S., and Danovaro, R.: Quantity and biochemical composition of particulate organic matter in a highly trawled area (Thermaikos Gulf, Eastern Mediterranean Sea), Adv. Oceanogr. Limnol., 6, 21–32, https://doi.org/10.4081/aiol.2015.5448, 2015.
Ragnarsson, S. and Steingrimsson, S. A.: Spatial distribution of otter trawl effort in Icelandic waters: comparison of measures of effort and implications for benthic community effects of trawling activities, ICES J. Mar. Sci., 60, 1200–1215, https://doi.org/10.1016/S1054-3139(03)00143-7, 2003.
Rajesh, N., Muthuvelu, S., Mahadevan, G., and Murugesan, P.: Impact of bottom trawling on water and sediment characteristics of Cuddalore and Parangipettai coastal waters, Indian J. Geo-Marine Sci., 48, 639–646, 2019.
Ramalho, S. P., Almeida, M., Esquete, P., Génio, L., Ravara, A., Rodrigues, C. F., Lampadariou, N., Vanreusel, A., and Cunha, M. R.: Bottom-trawling fisheries influence on standing stocks, composition, diversity and trophic redundancy of macrofaunal assemblages from the West Iberian Margin, Deep-Sea Res. Pt. I, 138, 131–145, https://doi.org/10.1016/j.dsr.2018.06.004, 2018.
Ramalho, S. P., Lins, L., Soetaert, K., Lampadariou, N., Cunha, M. R., Vanreusel, A., and Pape, E.: Ecosystem Functioning Under the Influence of Bottom-Trawling Disturbance: An Experimental Approach and Field Observations From a Continental Slope Area in the West Iberian Margin, Front. Mar. Sci., 7, 457, https://doi.org/10.3389/fmars.2020.00457, 2020.
Rohatgi, A.: Webplotdigitizer, https://automeris.io/WebPlotDigitizer (last access: 16 March 2022), 2022.
Rosli, N., Leduc, D., Rowden, A. A., Clark, M. R., Probert, P. K., Berkenbusch, K., and Neira, C.: Differences in meiofauna communities with sediment depth are greater than habitat effects on the New Zealand continental margin: implications for vulnerability to anthropogenic disturbance, PeerJ, 4, e2154, https://doi.org/10.7717/peerj.2154, 2016.
Sala, E., Mayorga, J., Bradley, D., Cabral, R. B., Atwood, T. B., Auber, A., Cheung, W., Costello, C., Ferretti, F., Friedlander, A. M., Gaines, S. D., Garilao, C., Goodell, W., Halpern, B. S., Hinson, A., Kaschner, K., Kesner-Reyes, K., Leprieur, F., McGowan, J., Morgan, L. E., Mouillot, D., Palacios-Abrantes, J., Possingham, H. P., Rechberger, K. D., Worm, B., and Lubchenco, J.: Protecting the global ocean for biodiversity, food and climate, Nature, 592, 397–402, https://doi.org/10.1038/s41586-021-03371-z, 2021.
Sánchez, A., Aguíñiga, S., Lluch-Belda, D., Camalich-Carpizo, J., Del Monte-Luna, P., Ponce-Díaz, G., and Arreguín-Sánchez, F.: Geoquímica sedimentaria en áreas de pesca de arrastre y no arrastre de fondo en la costa de Sinaloa-Sonora, Golfo de California, Boletín la Soc. Geológica Mex., 61, 25–30, http://www.scielo.org.mx/scielo.php?script=sci_arttext&pid=S1405-33222009000100004&lng=es&nrm=iso&tlng=es (last access: 23 November 2022), 2009.
Sañé, E., Martín, J., Puig, P., and Palanques, A.: Organic biomarkers in deep-sea regions affected by bottom trawling: pigments, fatty acids, amino acids and carbohydrates in surface sediments from the La Fonera (Palamós) Canyon, NW Mediterranean Sea, Biogeosciences, 10, 8093–8108, https://doi.org/10.5194/bg-10-8093-2013, 2013.
Sciberras, M., Parker, R., Powell, C., Robertson, C., Kröger, S., Bolam, S., and Geert Hiddink, J.: Impacts of bottom fishing on the sediment infaunal community and biogeochemistry of cohesive and non-cohesive sediments, Limnol. Oceanogr., 61, 2076–2089, https://doi.org/10.1002/lno.10354, 2016.
Sciberras, M., Tait, K., Brochain, G., Hiddink, J. G., Hale, R., Godbold, J. A., and Solan, M.: Mediation of nitrogen by post-disturbance shelf communities experiencing organic matter enrichment, Biogeochemistry, 135, 135–153, https://doi.org/10.1007/s10533-017-0370-5, 2017.
Sciberras, M., Hiddink, J. G., Jennings, S., Szostek, C. L., Hughes, K. M., Kneafsey, B., Clarke, L. J., Ellis, N., Rijnsdorp, A. D., McConnaughey, R. A., Hilborn, R., Collie, J. S., Pitcher, C. R., Amoroso, R. O., Parma, A. M., Suuronen, P., and Kaiser, M. J.: Response of benthic fauna to experimental bottom fishing: A global meta-analysis, Fish Fish., 19, 698–715, https://doi.org/10.1111/faf.12283, 2018.
Sheridan, P. and Doerr, J.: Short-Term Effects of the Cessation of Shrimp Trawling on Texas Benthic Habitats, Am. Fish. Soc. Symp., 41, 571–578, 2005.
Silveira, S., Ortega, I., and Dumont, L. F. C.: Artisanal trawling impact over prey availability and diet of estuarine megabenthic organisms in southern Brazil, Estuar. Coast. Shelf Sci., 237, 106682, https://doi.org/10.1016/j.ecss.2020.106682, 2020.
Simboura, N., Zenetos, A., Pancucci-Papadopoulou, M.-A., Thessalou-Legaki, M., and Papaspyrou, S.: A Baseline Study on Benthic Species Distribution in Two Neighbouring Gulfs, With and Without Access to Bottom Trawling, Mar. Ecol., 19, 293–309, https://doi.org/10.1111/j.1439-0485.1998.tb00469.x, 2008.
Smith, C., Papadopoulou, K. N., and Diliberto, S.: Impact of otter trawling on an eastern Mediterranean commercial trawl fishing ground, ICES J. Mar. Sci., 57, 1340–1351, https://doi.org/10.1006/jmsc.2000.0927, 2000.
Sparks-McConkey, P. J. and Watling, L.: Effects on the ecological integrity of a soft-bottom habitat from a trawling disturbance, Hydrobiologia, 456, 73–85, https://doi.org/10.1023/A:1013071629591, 2001.
Stone, R. P., Masuda, M., and Baldwin, A. P.: Characteristics of Benthic Sediments From Areas Open and Closed to Bottom Trawling in the Gulf of Alaska, U.S. Department of Commerce, NOAA technical memorandum NMFS-AFSC, 140, https://repository.library.noaa.gov/view/noaa/22851 (last access: 21 May 2022), 2003.
Tiano, J. C., Witbaard, R., Bergman, M. J. N., van Rijswijk, P., Tramper, A., van Oevelen, D., and Soetaert, K.: Acute impacts of bottom trawl gears on benthic metabolism and nutrient cycling, edited by S. Degraer, ICES J. Mar. Sci., 76, 1917–1930, https://doi.org/10.1093/icesjms/fsz060, 2019.
Tiano, J. C., De Borger, E., O'Flynn, S., Cheng, C. H., van Oevelen, D., and Soetaert, K.: Physical and electrical disturbance experiments uncover potential bottom fishing impacts on benthic ecosystem functioning, J. Exp. Mar. Bio. Ecol., 545, 151628, https://doi.org/10.1016/j.jembe.2021.151628, 2021.
Tiano, J. C., Depestele, J., Van Hoey, G., Fernandes, J., van Rijswijk, P., and Soetaert, K.: Trawling effects on biogeochemical processes are mediated by fauna in high-energy biogenic-reef-inhabited coastal sediments, Biogeosciences, 19, 2583–2598, https://doi.org/10.5194/bg-19-2583-2022, 2022.
Tiano, J. C., De Borger, E., Paradis, S., Bradshaw, C., Morys, C., Pusceddu, A., Ennas, C., Soetaert, K., Puig, P., Masqué, P., and Sciberras, M.: Global meta-analysis of demersal fishing impacts on organic carbon and associated biogeochemistry, Fish Fish., accepted, https://doi.org/10.1111/faf.12855, 2024.
Trimmer, M., Petersen, J., Sivyer, D., Mills, C., Young, E., and Parker, E.: Impact of long-term benthic trawl disturbance on sediment sorting and biogeochemistry in the southern North Sea, Mar. Ecol. Prog. Ser., 298, 79–94, https://doi.org/10.3354/meps298079, 2005.
Tsikopoulou, I., Smith, C. J., Papadopoulou, K. N., and Austen, M. C.: Linking species functional traits to specific biogeochemical processes under trawling pressure, Biology, 11, 1378, https://doi.org/10.3390/biology11101378, 2022.
Tuck, I. D., Hall, S. J., Robertson, M. R., Armstrong, E., and Basford, D. J.: Effects of physical trawling disturbance in a previously unfished sheltered Scottish sea loch, Mar. Ecol. Prog. Ser., 162, 227–242, https://doi.org/10.3354/meps162227, 1998.
van de Velde, S., Van Lancker, V., Hidalgo-Martinez, S., Berelson, W. M., and Meysman, F. J. R.: Anthropogenic disturbance keeps the coastal seafloor biogeochemistry in a transient state, Sci. Rep., 8, 5582, https://doi.org/10.1038/s41598-018-23925-y, 2018.
Warnken, K. W., Gill, G. A., Dellapenna, T. M., Lehman, R. D., Harper, D. E., and Allison, M. A.: The effects of shrimp trawling on sediment oxygen consumption and the fluxes of trace metals and nutrients from estuarine sediments, Estuar. Coast. Shelf Sci., 57, 25–42, https://doi.org/10.1016/S0272-7714(02)00316-5, 2003.
Watling, L., Findlay, R. H., Mayer, L. M., and Schick, D. F.: Impact of a scallop drag on the sediment chemistry, microbiota, and faunal assemblages of a shallow subtidal marine benthic community, J. Sea Res., 46, 309–324, https://doi.org/10.1016/S1385-1101(01)00083-1, 2001.
Watson, R. A. and Morato, T.: Fishing down the deep: Accounting for within-species changes in depth of fishing, Fish. Res., 140, 63–65, https://doi.org/10.1016/J.FISHRES.2012.12.004, 2013.
Watson, R. A. and Tidd, A.: Mapping nearly a century and a half of global marine fishing: 1869–2015, Mar. Policy, 93, 171–177, https://doi.org/10.1016/j.marpol.2018.04.023, 2018.