the Creative Commons Attribution 4.0 License.
the Creative Commons Attribution 4.0 License.
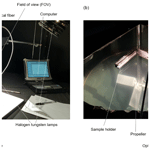
Hyperspectral reflectance dataset of pristine, weathered, and biofouled plastics
Ana I. Catarino
Liesbeth De Keukelaere
Mattias Bossaer
Els Knaeps
Gert Everaert
This work presents a hyperspectral reflectance dataset of macroplastic samples acquired using Analytical Spectral Devices (ASD) FieldSpec 4. Samples analyzed consisted of pristine, artificially weathered, and biofouled plastic items and plastic debris samples collected in the docks of the Port of Antwerp and in the river Scheldt near Temse Bridge (Belgium). The hyperspectral signal of each sample was measured in controlled dry conditions in an optical calibration facility at the Vlaamse Instelling voor Technologisch Onderzoek (VITO; Flemish Institute for Technological Research) and, for a subset of plastics, under wet and submerged conditions in a silo tank at Flanders Hydraulics. The wet and submerged hyperspectral signals were measured in a mesocosm setting that mimicked environmentally relevant concentrations of freshwater microalgae and suspended sediment. The ASD was equipped with an 8∘ field of view at the calibration facility, and a 1∘ field of view was used in the mesocosm setting. The dataset obtained complies with the FAIR (Findable, Accessible, Interoperable, Reusable) principles and is available in the open-access repository Marine Data Archive (https://doi.org/10.14284/530, Leone et al., 2021).
- Article
(1681 KB) - Full-text XML
-
Supplement
(1053 KB) - BibTeX
- EndNote
The spectral reflectance measurements, collected in the framework of the Plastic Flux for Innovation and Business Opportunities in Flanders (PLUXIN) project, contribute to the current knowledge on the detection of plastics using remote sensing techniques.
In recent years, focus has been placed on the detection of plastic litter using remote sensing techniques such as optical sensors on satellites, aircraft, and drones (Garaba and Dierssen, 2018; Martínez-Vicente et al., 2019). With the increasing demand for these technologies, it is crucial to generate knowledge on the diagnostic spectral properties of not only pristine but also weathered and biofouled plastics (Moshtaghi et al., 2021) that are representative of the variety of environmental plastics. Currently, the spectral reflectance of dry plastics is known and is already applied in the field of material recycling (Moroni et al., 2015), but it is restricted to items assessed for dry measurements. To be able to identify plastic litter in aquatic environments such as rivers, harbors, and oceans, we require the acquisition of the spectral features when plastics are either wet or submerged (Moshtaghi et al., 2021), as water absorbs the light in both the near (NIR) and shortwave infrared (SWIR). In addition, other water constituents such as sediment or algae could further impact the reflected signal of plastic items (Moshtaghi et al., 2021).
To date, only a limited number of high-quality datasets consisting of hyperspectral measurements of wet and submerged plastic litter have been published in open-access repositories (e.g., Garaba and Dierssen, 2018, 2020; Knaeps et al., 2021). As plastics in our environment are so diverse in polymer type, color, transparency, thickness, state (pristine, biofouled, weathered, wrinkled), and wetness (dry, wet, submerged), it is critical to generate, within the scientific community, substantiated datasets which represent plastics in many different facets. The collected spectra can serve as references or endmember spectra in future remote sensing plastic detection techniques and help to understand the complexity of plastic detection through spectral analysis. It is recognized that not all possible scenarios can be measured in an experimental way, therefore the dataset can further be used to compare with and complement numerical simulations. The dataset described in the current paper aims to complement the existing datasets by adding new information about the hyperspectral reflectance of pristine plastic items, harvested plastic litter, and artificially weathered and biofouled plastic samples. In addition, the dataset reports the optical features of plastics acquired in various water turbidity conditions obtained by adding sediment or algae to the water at selected concentrations. The dry spectral reflectance of plastic specimens, consisting of different polymers, was measured using an Analytical Spectral Device (ASD) FieldSpec 4. For a selection of these samples, the spectral reflectance was also collected in wet and submerged settings.
The presented data can be used to generate insights into the spectral properties of plastic litter and how these features change when plastics are exposed to natural agents or different depths. The dataset was compiled following the FAIR (Findable, Accessible, Interoperable, Reusable; https://www.go-fair.org/fair-principles/, last access: 24 April 2022; GO FAIR, last update 2022) principles, and it is publicly available at https://doi.org/10.14284/530 (Leone et al., 2021).
Data collection consisted of measuring the spectral reflectance of different plastic specimens using the ASD. The measurements were conducted in two different settings: at an optical calibration facility located at the Vlaamse Instelling voor Technologisch Onderzoek (VITO; Flemish Institute for Technological Research; Mol, Belgium), and at a silo tank at the Flanders Hydraulics Research facility (Antwerp, Belgium). The spectral reflectance of each plastic specimen was collected at least 5 times (“pseudo-replicates”) within 1 min. These pseudo-replicates are measurements taken by slightly changing the position of the plastic sample. Therefore, very homogeneous plastics will have less variation in the pseudo-replicates' reflectance.
2.1 Plastic specimens
We aimed to collect the spectral reflectance of plastic items as representative as possible of commonly found plastics in the environment (Li et al., 2016) and to provide additional information on their reflectance when exposed to natural agents such as sunlight (UV radiation) or biofilm growth. To do so, we have selected and analyzed a total of 10 polymer types. The selected polymers were polyethylene (PE), polypropylene (PP), polystyrene (PS), two types of polyethylene terephthalate (PET) – crystalline and amorphous – fluorocarbon, thermoplastic elastomer, polyvinyl chloride (PVC), nylon 6 (PA6), and paraffin (Table 1; S1). In addition, we further artificially weathered and biofouled a selection of six plastic polymer types. The polymer discrimination was based on the available information from the supplier or marked on the plastic itself (e.g., plastic bottles or bags with an identifiable polymer tag). In addition, for a set of samples, we confirmed the polymer type using a micro-Fourier transform infrared spectroscopy (micro-FTIR, PerkinElmer, FTIR spectrometer Frontier). The percentage of matching scores with a library was recorded, and it is reported in the dataset. Table 1 provides an overview of measured plastic types within this study and compared them to existing datasets. This dataset adds additional information on plastics spectral reflectance of similar polymers to what is already available in the literature, allowing comparison but also novel conditions and treatments (Table 1).
Table 1Comparison of polymers and conditions analyzed in this dataset and in the literature. (D: dry; W: wet; S: submerged; PE: polyethylene; PP: polypropylene; PS: polystyrene; PET: polyethylene terephthalate; PVC: polyvinyl chloride; PA6: polyamide 6; PA 6.6: polyamide 66; LD-PE: low-density polyethylene; FEB: fluorinated ethylene propylene Teflon; ABS: terpolymer lustran 752; and PMMA: polymethyl methacrylate.)
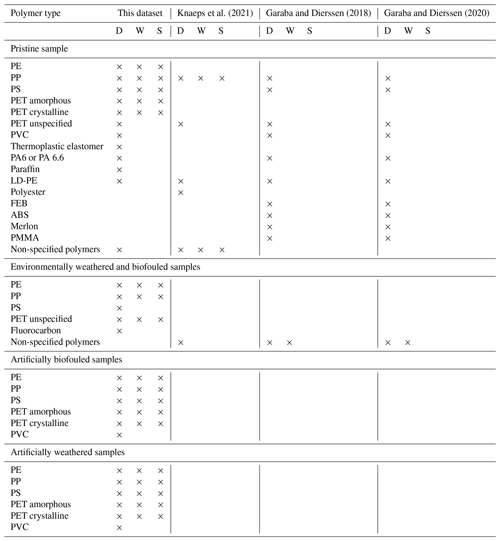
2.1.1 Pristine plastic specimens
To enhance the physio-chemical properties of an end plastic product, plastic manufacturers often incorporate additive substances into the pristine polymer (Garaba et al., 2021). Additives included in plastics, such as colored plastics, may be influencing the spectra (Garaba et al., 2021). Thus, to avoid confounding measurements, sheets of 6 × 6 cm were obtained from a commercial supplier (Carat, Germany, https://www.carat-lab.com, last access: 24 April 2022) as pure pristine plastic polymers without any additional additive for the following polymers: PP, PE, PS, PET amorphous, and PET crystalline. In addition, no color or stains were added to the commercial plastics, and as such, these selected samples were transparent or white. The other pristine plastic samples were either purchased from a local shop (Ostend, Belgium) or obtained from other institutes (Marine Remote Sensing Group, University of the Aegean in Plastic Litter Projects, 2021–2022, The Ocean Cleanup).
2.1.2 Weathered plastics
To mimic the effect of solar radiation on plastic items in the environment, pristine plastics without additives were exposed to UV radiation in an Atlas SunTest CPS+ weathering chamber, simulating 1 solar year in central Europe (Gewert et al., 2018) (Table 2). Prior to the treatment, plastic specimens were cut, using a hot knife, into 2 × 10 cm sheets in order to fit them into closed quartz cuvettes. Although UV exposure in a laboratory cannot perfectly mimic environmental conditions, to reproduce them as accurately as possible, we have tested different treatments for weathering conditions: i.e., dry UV exposure, seawater UV exposure (35 PSU), and dark controls (dry and wet), and each treatment consisted of a set of three independent replicates for each polymer type (Table 4).
2.1.3 Biofouled plastics
To test the effects of biofilm attached to the surface of plastic items on their spectral reflectance, we induced biofilm growth on the two surfaces of pristine samples. Since surface roughness can affect biofilm growth and its survival (Rodriguez et al., 2012), one of the two surfaces of the commercial plastic specimens was manually treated for approximately 10 s with sandpaper (grain size 80) to create a rougher surface compared to the smooth and untouched one. An aquarium was filled with unfiltered seawater collected from the port of Ostend (Belgium), which was renewed every 2 weeks, kept at 20 ∘C, and aerated with an air pump. Plastic specimens were suspended in the aquarium with paper clippers and rope (Fig. 1) to allow the biofilm to form on all of the surfaces.
2.1.4 Field plastic items
Plastic specimens were collected from the Port of Antwerp and in the Scheldt River, near Temse Bridge (Belgium), and are naturally exposed to weathering and biofouling and are, compared to pristine polymers, non-homogeneous. The plastic coming from the river Scheldt was collected by a plastic collector installed by the Belgian company Dredging, Environmental and Marine Engineering NV (DEME) Environmental Contractors (DEC) on behalf of Vlaamse Waterweg (the Flemish authority responsible for waterways in Flanders, Belgium).
2.2 Spectral measurements of plastic items
The spectral reflectance measurements of plastic specimens were performed using an Analytical Spectral Device (ASD) Field-Spec4 (Table 3). The reflectance was automatically derived and normalized to a 99 % Labsphere Spectralon® Lambertian panel. In the silo tank, the Spectralon panel was positioned on the holder of the adjustable arm at the same distance from the ASD field of view as the dry plastic specimen.
Following the procedure described by Knaeps et al. (2021), we measured the spectral reflectance of the different plastic samples at two different facilities: (1) measurements done in dry conditions were performed at the optical calibration laboratory of the Flemish Institute for Technological Research (VITO), Belgium; and (2) the series of wet and submerged measurements were acquired in a mesocosm setting, with experiments being performed in a conical shaped silo tank at the Flanders Hydraulics Research facility in Antwerp, Belgium. In both laboratory and silo tank setups (Fig. 2), we have attached a laser pen to the ASD pistol grip to ensure that, at all times, the fiber optics of the ASD were pointing at the plastic samples.
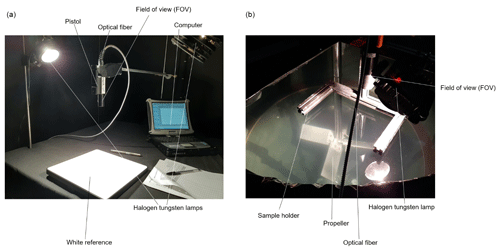
Figure 2Experimental setup; (a) laboratory setup and (b) silo tank setup. Pictures were taken by Giulia Leone.
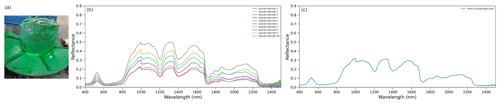
Figure 3Spectra of field sample and figure of the object from which the spectra were obtained (a). Spectrum (b) shows the pseudo-replicates of the low homogeneous field samples. Spectrum (c) is the mean of all the pseudo-replicates. No splice correction was applied. The picture was taken by Giulia Leone.
Table 4Overview of the polymer and treatment performed during the study (SSC: suspended sediment concentration).
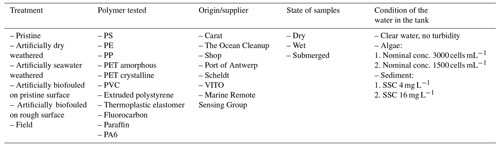
3.1 Laboratory setup
All the plastic specimens were measured in the optical calibration facility (VITO, Belgium). This laboratory consisted of a dark room, where a desk equipped with two halogen tungsten lamps and a holder for the ASD field of view allowed us to collect the measurements.
3.2 Silo tank setup
The water silo tank (diameter: 2 m, depth: 3 m), at Flanders Hydraulics Research (Belgium), was equipped with a controlled mixer with a double pitch blade impeller that permitted the mixing of the water to obtain suspensions of sediment or algae. No information about the rpm (revolutions per minute) was available on the metal impeller at the water tank. We attached a tailor-made aluminum frame to the water tank for the mounting of the spectroradiometer detector and light, together with a plexiglass sample holder to lower the plastic specimens in the tank and measure their spectral reflectance at different water depths. The plexiglass was cut to fit the plastic samples which were held by black plastic paper clips. In the current study, we acquired data using a single lamp attached to the frame, with an angle of 40∘. To reduce undesired stray light, a dark environment was created with a black plastic cover around the experimental setup, and in addition, black plastic bags were held against the tank by means of wooden rings with weights and waterproof tape. In the tank, samples attached to the plexiglass sample holder were first measured at dry conditions just above the water level and then carefully lowered in the water at fixed depths. The water level above the sample was measured with a ruler and the chosen depths were: 1, 2, 4, and 8 cm. After the submersion, the plastic was measured again above the water level as a wetted sample. The same steps were performed in clear and turbid water.
3.2.1 Water with added sediment
Natural sediment was collected using a manual Van Veen grab by the Flanders Marine Institute (VLIZ) during a sampling campaign as part of the PLUXIN project in Nieuwpoort (Belgium). The sediment was transported to the laboratory (VLIZ), placed into a metal container, and dried in the oven for 4 d at 60 ∘C, and was afterward manually crushed using a mortar and stored in the dark until further use. To add the crushed sediment into the tank, before any measurements, the tank metal impeller was activated for 1.5 min to allow the sediment to stay in suspension in the water. This action was repeated approximately every 30 min to maintain the sediment in suspension. The actual concentration of sediment added to the tank was measured by Flanders Hydraulics. The suspended sediment concentration (SSC) was determined gravimetrically, after filtration of the sample through a filter with a pore size of 0.45 µm and drying at a temperature of 105 ∘C. The results showed a low concentration of sediment of 4 mg L−1 and a higher concentration of 16 mg L−1.
3.2.2 Water with added microalgae
To evaluate how the readings of spectral reflectance of plastic specimens can be affected by turbidity in the water due to microalgae suspension, measurements were performed using two concentrations of freshwater Pseudokirchneriella subcapitata. The concentrations were selected to mimic the natural conditions of spring and late summer seasonal concentrations of green algae for a western European river (Ibelings et al., 1998). The nominal concentrations of microalgae were 1500 and 3000 cells mL−1. The microalgae P. subcapitata was cultured at the Research unit “Health” at VITO, and the stock solution of 8.18 × 106 algae mL−1 was kept for 11 d in a cold room and the dark before being used in the experimental setup. After pouring 450 mL of stock solution, before any measurements, the metal impeller was activated for 1.5 min to allow the algae to suspend in the water. This action was repeated approximately every 30 min to maintain the algae in suspension. To obtain a higher concentration of algae that would mimic a nominal concentration of 3000 cells mL−1, another 450 mL of stock solution was then added into the tank.
In this study, we created a dataset with the spectral reflectance of 10 plastic polymers that had undergone two treatments (i.e., artificial weathering and artificial biofouling), in addition to field-collected samples and pristine plastics (Table 4). All data included in the presented dataset were curated before submission and consisted of the raw reflectance data of the samples. A metadata section, included in the dataset, explains the data more in detail. For instance, information on the polymer type, micro-FTIR results with the corresponding library matching score, the origin of the sample, date, and time of collection. The sample code was created following the naming convention of Knaeps et al. (2021). To ensure that end users can correctly interpret each section of the metadata, a README file is available together with the dataset. From the presented dataset, it is possible to visually show the spectral reflectance of different plastic polymers and compare the different conditions experimentally tested (Figs. S1, S2). For instance, it is possible to derive the effect of weathering and biofouling on the spectral reflectance of a polymer when compared with the same pristine one.
We suggest that users of this dataset perform splice correction on the data using Python or any suitable software. This processing step will lead to the obtention of spectra that do not present radiometric steps at the joints of the detectors. In addition, pseudo-replicates of each plastic sample were taken, and therefore, we advise that users calculate the mean of these measurements to obtain a single spectrum for each observed item (Fig. 3).
The data are available in the open access repository Marine Data Archive at https://doi.org/10.14284/530 (Leone et al., 2021).
The use of remote sensing technologies can be used in the detection, observation, and monitoring of marine plastic pollution. However, due to a lack of knowledge of the optical features of environmental plastics, small steps can be made in designing algorithms to appropriately detect plastic pollution. The presented hyperspectral dataset is a step forward in the knowledge of the optical features of plastic litter when exposed to natural agents such as UV radiations or the growth of biofilm. In addition, from the data presented it is possible to investigate the effects that biofouling and weathering have on different polymers. Lastly, the conditions in which a plastic polymer is (i.e., dry, wet, or submerged with different turbidity) are also described and assessed in the presented dataset. Therefore, we anticipate that this dataset will contribute to the definition of optical spectral bands and assist in the development of algorithms for the observation, monitoring, and discrimination of plastics in a (semi-) operational environment.
The supplement related to this article is available online at: https://doi.org/10.5194/essd-15-745-2023-supplement.
GL: conceptualization, methodology, writing – (original draft and review and editing), visualization, funding acquisition, data curation, and investigation. AIC: conceptualization, methodology, writing (review and editing), and supervision. LDK: conceptualization and writing (review and editing). MB: investigation, methodology, and writing (review and editing). EK: conceptualization, methodology, writing (review and editing), supervision, and funding acquisition. GE: conceptualization, methodology, funding acquisition, supervision, and writing (review and editing).
The contact author has declared that none of the authors has any competing interests.
Publisher’s note: Copernicus Publications remains neutral with regard to jurisdictional claims in published maps and institutional affiliations.
We would like to thank Flanders Hydraulics for the use of the silo tank and for the sediment concentration measurements, and Hilda Witters and Guy Geukens of the research Unit Health at VITO (Belgium) for culturing the freshwater algae. Special thanks to the Marine Remote Sensing Group, University of the Aegean (Greece), and The Ocean Cleanup (the Netherlands) for sharing plastic specimens with us.
This research has been supported by the cSBO Plastic Flux for Innovation and Business Opportunities in Flanders (PLUXIN, HBC.2019.2904) project, financed by Flanders Innovation & Entrepreneurship (VLAIO) and supported by The Blue Cluster, a Flemish members organization for sustainable entrepreneurship in blue growth (Belgium). Since November 2021, Giulia Leone is supported by the Research Foundation of Flanders (FWO), as a PhD grant strategic basic research, application number 1S13522N (Belgium).
This paper was edited by David Carlson and reviewed by Chuanmin Hu and one anonymous referee.
Garaba, S. P. and Dierssen, H. M.: An airborne remote sensing case study of synthetic hydrocarbon detection using short wave infrared absorption features identified from marine-harvested macro- and microplastics, Remote Sens. Environ., 205, 224–235, https://doi.org/10.1016/j.rse.2017.11.023, 2018.
Garaba, S. P. and Dierssen, H. M.: Hyperspectral ultraviolet to shortwave infrared characteristics of marine-harvested, washed-ashore and virgin plastics, Earth Syst. Sci. Data, 12, 77–86, https://doi.org/10.5194/essd-12-77-2020, 2020.
Garaba, S. P., Arias, M., Corradi, P., Harmel, T., de Vries, R., and Lebreton, L.: Concentration, anisotropic and apparent colour effects on optical reflectance properties of virgin and ocean-harvested plastics, J. Hazard. Mater., 406, 124290, https://doi.org/10.1016/j.jhazmat.2020.124290, 2021.
Gewert, B., Plassmann, M., Sandblom, O., and Macleod, M.: Identification of Chain Scission Products Released to Water by Plastic Exposed to Ultraviolet Light, Environ. Sci. Technol. Lett., 5, 272–276, https://doi.org/10.1021/acs.estlett.8b00119, 2018.
GO FAIR: GO FAIR [website], https://www.go-fair.org (last access: 24 April 2022), last update 2022.
Ibelings, B., Admiraal, W., Bijkerk, R., Ietswaart, T., and Prins, H.: Monitoring of algae in Dutch rivers: does it meet its goals?, J. Appl. Phycol., 10, 171–181, https://doi.org/10.1023/A:1008049000764, 1998.
Knaeps, E., Sterckx, S., Strackx, G., Mijnendonckx, J., Moshtaghi, M., Garaba, S. P., and Meire, D.: Hyperspectral-reflectance dataset of dry, wet and submerged marine litter, Earth Syst. Sci. Data, 13, 713–730, https://doi.org/10.5194/essd-13-713-2021, 2021.
Leone, G., Catarino, A., De Keukelaere, L., Bossaer, M., Knaeps, E., and Everaert, G.: Hyperspectral reflectance dataset for dry, wet and submerged plastics in clear and turbid water, Marine Data Archive [data set], https://doi.org/10.14284/530, 2021.
Li, W. C., Tse, H., and Fok, L.: Plastic waste in the marine environment: A review of sources, occurrence and effects, Sci. Total Environ., 566–567, 333–349, https://doi.org/10.1016/j.scitotenv.2016.05.084, 2016.
Martínez-Vicente, V., Clark, J. R., Corradi, P., Aliani, S., Arias, M., Bochow, M., Bonnery, G., Cole, M., Cózar, A., Donnelly, R., Echevarría, F., Galgani, F., Garaba, S. P., Goddijn-Murphy, L., Lebreton, L., Leslie, H. A., Lindeque, P. K., Maximenko, N., Martin-Lauzer, F.-R., Moller, D., Murphy, P., Palombi, L., Raimondi, V., Reisser, J., Romero, L., Simis, S. G. H., Sterckx, S., Thompson, R. C., Topouzelis, K. N., van Sebille, E. van, Veiga, J. M., and Vethaak, A. D.: Measuring Marine Plastic Debris from Space: Initial Assessment of Observation Requirements, Remote Sensing, 11, 2443, https://doi.org/10.3390/rs11202443, 2019.
Moroni, M., Mei, A., Leonardi, A., Lupo, E., and Marca, F.: PET and PVC Separation with Hyperspectral Imagery, Sensors, 15, 2205–2227, https://doi.org/10.3390/s150102205, 2015.
Moshtaghi, M., Knaeps, E., Sterckx, S., Garaba, S., and Meire, D.: Spectral reflectance of marine macroplastics in the VNIR and SWIR measured in a controlled environment, Sci. Rep., 11, 5436, https://doi.org/10.1038/s41598-021-84867-6, 2021.
Rodriguez, D., Einarsson, B., and Carpio, A.: Biofilm growth on rugose surfaces, Phys. Rev. E Stat. Nonlin. Soft Matter Phys., 86, 061914, https://doi.org/10.1103/PhysRevE.86.061914, 2012.