the Creative Commons Attribution 4.0 License.
the Creative Commons Attribution 4.0 License.
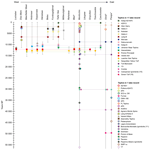
Tephra data from varved lakes of the Last Glacial–Interglacial Transition: towards a global inventory and better chronologies on the Varved Sediments Database (VARDA)
Anna Beckett
Cecile Blanchet
Alexander Brauser
Rebecca Kearney
Celia Martin-Puertas
Ian Matthews
Konstantin Mittelbach
Adrian Palmer
Arne Ramisch
Achim Brauer
The Varved Sediments Database (VARDA) was launched in 2020 and aimed to establish a community database for annually resolved chronological archives with their associated high-resolution proxy records. This resource would support reproducibility through accessible data for the paleoclimate and modelling communities. In this paper, VARDA has been extended by a dataset of European tephra geochemical data and metadata to enable the synchronisation of varve records during the Last Glacial–Interglacial Transition (LGIT; here defined as 25 to 8 ka; Beckett et al., 2022). Geochemical data from 49 known individual tephra layers across 19 lake records have been included, with Lago di Grande Monticchio being the single biggest contributor of geochemical data with 28 tephra layers. The Vedde Ash and Laacher See tephra are the most common layers found in six different records. This highlights the potential of refining the absolute age estimates for these tephra layers using varve chronologies and for synchronising regional paleoclimate archives. This is the first stage in a 5-year plan funded by the Past Global Changes (PAGES) Data Stewardship Scholarship to incorporate a global dataset of tephra geochemical data into varve records. Further stages of this project will focus on different regions and timescales. Data collated for this project are available open access at https://doi.org/10.5880/fidgeo.2023.015 (Beckett et al., 2022).
- Article
(2198 KB) - Full-text XML
- BibTeX
- EndNote
Varved lake sediment records are annually resolved archives of climatic and environmental change (Brauer, 2004; Zolitschka et al., 2015), with comparable resolution to ice cores (Rasmussen et al., 2007). The very nature of these records allows for robust chronologies based on annual layer counts, which can be validated by using independent radiometric dating techniques. Furthermore, other lithological and biological proxy data within these archives can be explored at sub-decadal to seasonal scales (Brauer et al., 2008; Zolitschka et al., 2015). Over the last 2 decades, there has been an increasing focus on (crypto)tephra in varved sediments. Improved techniques for extracting tephra from sediments with a low shard concentration (e.g. Merkt et al., 1993; Blockley et al., 2005; Walsh et al., 2021) have enabled distal tephra horizons to be detected in varve lake records, enabling the application of tephrochronology to improve varve chronologies (e.g. Stihler et al., 1992; Wulf et al., 2004, 2016; Palmer et al., 2020), the use of varve chronologies to generate more precise ages for tephra layers (e.g. Lane et al., 2015; Dräger et al., 2017; Walsh et al., 2021), and the use of varve chronologies as a synchronisation tool to better understand the time-transgressive nature of rapid environmental and climatic change at regional scales (tephra lattices) (Lane et al., 2013; Macleod et al., 2014; Wulf et al., 2016).
Tephra horizons detected within varve sediments are often well constrained and undisturbed and can be precisely dated using the varve chronologies (Lane et al., 2013; Palmer et al., 2020; Walsh et al., 2023). However, a key step in developing a tephrochronology requires a link between the tephra horizon in a sediment archive and an eruption of a known age. This stage is normally undertaken using geochemical data which link the tephra to an eruptive centre (Timms et al., 2019). As more tephra horizons have been detected, there have been important community efforts to improve the accessibility of tephra geochemical datasets. Examples include the RESET database (Bronk Ramsey et al., 2015a) and TephraBase (Newton et al., 2007), which both provide geochemical data and metadata related to the sample analysis. VOLCORE (Mahony et al., 2020) is a more recent addition to tephra databases, providing stratigraphic and geographical data on visible tephra layers discovered in ocean drilling projects.
Further to this, there has been a major increase in the number of varve chronologies reported over the past 30 years and even more recently an increase in papers discussing tephra horizons detected in varve records (see Fig. 1). In 2012, the Varve Working Group (VWG) created a database of varved records in xmsl file format, containing metadata relating to the chronologies of 108 varve lake records, as discussed in Ojala et al. (2012), but this database lacks specific data from proxies and additional chronological control. The recent development of VARDA (Varved Sediments Database 1.0 (Ramisch et al., 2020)) has provided, for the first time, a global database of varve sites that includes metadata on site locations, duration of the varve record and the associated proxy data. In this paper, we present an extensive dataset of tephra horizons identified in varved records, together with their published geochemical datasets and metadata as an update to VARDA. This dataset focuses on European varve records on VARDA, specifically during the Last Glacial–Interglacial Transition (LGIT) because of the abundance of sequences with tephra reported in this region. We discuss the nature of lake identification, data collection and the range of records now available within the database.
2.1 Lake record identification
This work is an initial stage of a 5-year programme which aims to reach a global scale, and therefore, as a first step, three criteria were required to be met before tephra data were collected in order to develop the framework for later stages of the project. Firstly, we defined a region to collect tephra data from. Since the tephrostratigraphies of different volcanic provinces in Europe are reasonably well developed, it was considered that there were sufficient tephra data to establish the required metadata, and the framework could be tested when developing this part of the database. Secondly, we focused on a specific time period, and, in this case, we chose the LGIT, here defined broadly between 25 and 8 ka. This will enable varved records to be synchronised using tephra during a period of known abrupt climate change during the last deglaciation. Finally, when tephra layers had been identified within a published varve record on VARDA, it was essential that those reported tephra layers included tephra geochemistry and information on the analytical operating conditions including instrument settings and secondary standards.
Using the pre-existing “age within time span” and “search by continent” features in VARDA (Fig. 2a), lake records that were within the determined time period and region were narrowed down to a total of 33 records. The next stage consisted of systematic literature search through the Varve Working Group (VWG) papers and, using Google Scholar, to identify more recent publications for each lake site and to determine which sites contained tephra layers.
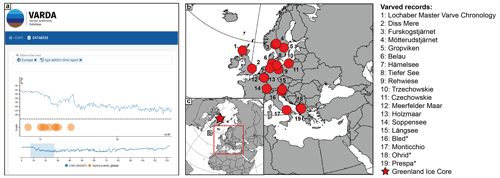
Figure 2(a) Screenshot of the parameters used on VARDA to narrow down the search for lakes within the specified time frame and region (https://varve.gfz-potsdam.de/database, last access: 18 July 2022). (b) Location of all records with tephra geochemical data included in this update. (c) Region where tephra data have been collected, including relative location to the Greenland ice core records. “*” indicates sites that are non-varved.
2.2 Data collection
With the aim of adding new proxy records to VARDA (which is beyond the scope of the present paper), we structured the newly acquired data using fields identified in Ramisch et al. (2020). Where necessary, new fields were adopted in the Beckett et al. (2022) dataset to create a standard approach for documenting and compiling tephra geochemical data in line with established tephra community standards (e.g. Timms et al., 2019; Wallace et al., 2022) and metadata related to the tephra layer as identified by the authors (Tables 1 and 2). This process generates the relevant information for each individual tephra layer and the sites it has been identified in.
Of the parameters in Table 1, “Correlation” and “Source” are mandatory but can be recorded as “Unknown”. This allows for (1) the input of tephra geochemical data from unknown eruptions and therefore not correlated to a named tephra layer and (2) the input of tephra layers with an unknown or unconfirmed volcanic source. Tephra layers without a known source or correlation can still be valuable isochronous marker horizons; therefore making these fields mandatory was deemed appropriate.
Table 2 outlines all the relevant information published with the geochemical data and provides context to the major element geochemistry. This includes providing age estimates and the methods used for dating each layer, which aids in distinguishing identical geochemical signatures based on age. It must be noted that the “age cal BP” provided on the database may vary for the same tephra layer across different sites; defining the “best” age for a tephra layer is subjective, and therefore this project has taken the approach to use the date quoted in the paper publishing the geochemical data. This allows for recalculating ages of the tephra horizon using the most recent 14C calibration curve, if appropriate. In addition, there has been a recent drive in the tephra community for reporting the analytical conditions used for obtaining geochemistry and including the standard materials used for calibrating the analytical device. This metadata information enables the data to be reproducible and consistent for future tephra investigations and was therefore collected from the literature for each tephra layer, with future additions to include the published average and 2-standard-deviation- measured major and minor element oxide values for secondary standards to ensure quality assurance and accurate tephra correlations.
Of the 33 lakes of suitable age and location on VARDA, 22 contained tephra layers, but only 19 of those have published geochemical data of the tephra layers (locations displayed in Fig. 2b). The lake archives with tephra geochemical data are (Figs. 3, 4) Belau (Dörfler et al., 2012), Bled (Lane et al., 2011b), Czechowskie (Wulf et al., 2016), Diss Mere (Martin-Puertas et al., 2021; Walsh et al., 2021), Furskogstjärnet (Zillén et al., 2002), Gropviken (Macleod et al., 2014), Hämelsee (Jones et al., 2018), Holzmaar (Wulf et al., 2013), Längsee (Schmidt et al., 2002), Lochaber (Palmer et al., 2020), Meerfelder Maar (Lane et al., 2015), Lago di Grande Monticchio (Wulf et al., 2004, 2008), Mötterudstjärnet (Zillén et al., 2002), Ohrid (Vogel et al., 2010), Prespa (Wagner et al., 2012), Rehwiese (Wulf et al., 2013), Soppensee (Lane et al., 2011a), Tiefer See (Wulf et al., 2016) and Trzechowskie (Wulf et al., 2013). Where applicable, if only part of the lake record fell within the time frame, all tephra layers found in the record, including pre-25 ka and/or post-8 ka, were compiled to create a consistent approach for each lake record.
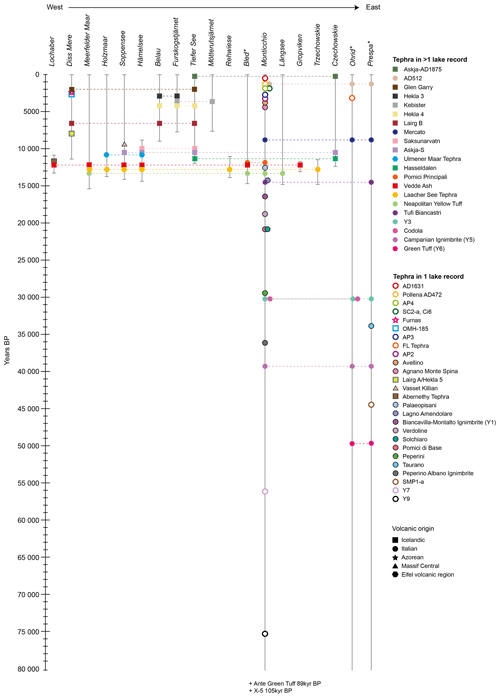
Figure 3Connectivity of tephra layers between varved lake records, with dashed lines connecting the same layer between records. Ages used are as detailed in the compiled database. * Records that are non-varved but are included for good chronological control – see Ramisch et al. (2020) for further details.
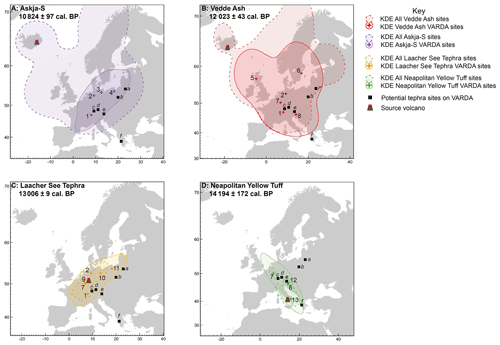
Figure 4Kernel density estimation plots (Bronk Ramsey et al., 2015a) of four tephra layers present in four or more varve records comparing the RESET database supplemented by a selection of more recent identifications that extend the range (dashed line) with the spatial range using the VARDA (solid line). KDE provides a 95 % confidence interval on the dispersal range of tephra using the spatial distribution of sites queried. Age estimations sourced from (a) Kearney et al. (2018), (b) Bronk Ramsey et al. (2015b), (c) Reinig et al. (2021) and (d) Bronk Ramsey et al. (2015b). These are the current most precise age estimates for the specific tephra horizons and may not correspond with age estimates in the database. Tephra sites are as follows: (1) Soppensee, (2) Hämelsee, (3) Tiefer See, (4) Czechowskie, (5) Lochaber Master Varve Chronology, (6) Gropviken, (7) Meerfelder Maar, (8) Bled, (9) Holzmaar, (10) Rehwiese, (11) Trzechowskie, (12) Längsee and (13) Lago di Grande Monticchio. Potential tephra sites are (a) Hancza, (b) Gosciaz, (c) Schleinsee, (d) Ammersee, (e) Mondsee and (f) Lagoon Etoliko.
Figure 3 displays the interconnections established between the archives through the correlated tephra layers. Within these 19 lake archives, there are 49 individual known tephra layers, each with at least one lake archive providing geochemical data. The volcanic source regions for these tephra layers found in Europe are Iceland, Eifel, Massif Central, the Hellenic Arc and Italy, including multiple tephra layers from the Somma–Vesuvius and Campi Flegrei volcanic complexes. There are an additional 24 tephra layers with “unknown” correlations that have been included in the database. The Vedde Ash (Iceland) and Laacher See tephra (Eifel) layers are the most commonly found and, if combined, allow us to synchronise nine records (Fig. 3). Geographically, the Vedde Ash (Katla, Iceland) is the most widespread tephra layer in the database, reaching from Scotland in the west to Sweden and Slovenia in the east (Fig. 4b). Both the Askja-S tephra layer (Askja, Iceland) and Neapolitan Yellow Tuff (Campi Flegrei, Italy) are found in four records across Europe (Fig. 4a and d). Lago di Grande Monticchio is the site with the most identified tephra layers at present; there are 28 tephra layers (all originating from Italy or the Hellenic Arc) within the time period of 0–100 ka included in the database, but additional layers have been identified earlier in the record (See: Wulf et al., 2012), which will be added to the database in the next steps of the project.
The collection of this information is helpful to identify both the temporal (Fig. 3) and spatial range of the tephra layers in predominantly varved (and three non-varved) sediment records across Europe (Fig. 4). Clearly, there is a concentration of tephra layers reported around the Late Glacial period (∼ 15–11 ka), most likely reflecting the wealth of studies focusing on investigating this period of abrupt climate change and its impact on the temperate mid-latitudes of Europe. Nonetheless, there is considerable scope to extend these studies to the period immediately after the Last Glacial Maximum in Europe. Recent investigations in Middle and Late Holocene tephra layers in European varves show potential for a more robust Holocene tephrostratigraphic framework in the North Atlantic sector (Dräger et al., 2017; Walsh et al., 2021, 2023). Extending the spatial reach of the tephra database will allow us to build tephra lattices that will help in connecting/synchronising climate records on a global scale.
Comparison of varve records to non-varved records shows where varved sediments with tephra are lacking but will also provide important information on the potential of finding cryptotephra in varve sequences across Europe based on the likely passage of the tephra dispersal at the time of the eruption. For an example with comparison to other well-known tephra databases, Fig. 4 displays a kernel density estimation (KDE) of the extent of the Askja-S, Vedde Ash, Laacher See and Neapolitan Yellow Tuff tephra layers using all known records in the RESET database (Bronk Ramsey et al., 2015a) and additional more recent sites that extend the known limit of tephra dispersal (Wulf et al., 2013; Haflidason et al., 2019; Jones et al., 2020). The KDE, in this instance, is used purely statistically to broadly estimate the 95 % confidence interval for spatial distribution of sites containing each tephra layer (Bronk Ramsey et al., 2015a). Superimposed over this is a KDE of the tephra dispersal using only the sites containing these tephra layers in VARDA (Ramisch et al., 2020). Furthermore, the location of six additional sites with varve chronologies (Ammersee (von Grafenstein et al., 1998, 1999), Gosciaz (Bonk et al., 2021; Müller et al., 2021), Hancza (Lauterbach et al., 2011b), Lagoon Etoliko (Haenssler et al., 2013), Mondsee (Lauterbach et al., 2011a; Swierczynski et al., 2013) and Schleinsee (Clark et al., 1989)), which have high potential for cryptotephra investigations, is highlighted (Fig. 4). These sites have been identified through a simple query using VARDA search functions for sites within Europe and within the appropriate time span.
Tephra geochemical data compiled for this project are available open access at the GFZ Data Services at https://doi.org/10.5880/fidgeo.2023.015 (Beckett et al., 2022) or via https://varve.gfz-potsdam.de (Ramisch et al., 2020).
There is much potential in detecting (crypto)tephra in varved sediment records as they act as one of the most precise forms of isochronous marker horizons that can help in better understanding the rates of regional climatic responses to global perturbations. By concentrating on the European tephrostratigraphy during the LGIT, we have initiated the inclusion of these important datasets, in particular the geochemical information and metadata to improve accessibility. Further iterations of this expanded database are planned through the Past Global Changes (PAGES) Data Stewardship Scholarship by extending the spatial coverage and temporal range for tephra horizons in varved sediments. Expanding the collection of tephra geochemistry provides opportunities to explore novel and emerging data analysis techniques to identify unknown tephra layers based on their geochemical signatures, potential dispersal and estimated age. Finally, further research into tephrochronology in varved records should focus on exploring other regions and time periods with as much intensity as has been given to the LGIT in Europe.
AnB: data curation, investigation, validation, visualisation, manuscript writing (original draft and review and editing). CB: visualisation, project administration, manuscript writing (review and editing). AlB: database administration, data curation, manuscript writing (review and editing), software. RK: manuscript writing (review and editing). CMP: conceptualisation, funding acquisition, manuscript writing (review and editing), project administration. IM: visualisation, manuscript writing (review and editing). KM: database administration, software. AP: conceptualisation, funding acquisition, manuscript writing (review and editing), project administration, supervision. AR: conceptualisation, project administration. ACB: manuscript writing (review and editing), conceptualisation.
The contact author has declared that none of the authors has any competing interests.
Publisher's note: Copernicus Publications remains neutral with regard to jurisdictional claims made in the text, published maps, institutional affiliations, or any other geographical representation in this paper. While Copernicus Publications makes every effort to include appropriate place names, the final responsibility lies with the authors.
We acknowledge the Past Global Changes (PAGES) Data Stewardship Scholarship (no. 102) for financial support in the generation and inclusion of the tephra datasets into the database. This work was supported by the German Federal Ministry of Education and Research (BMBF) as a Research for Sustainability initiative (FONA; http://www.fona.de, last access: 10 November 2022) through the Palmod project. Simon Blockley is thanked for his help accessing and navigating the RESET database.
This research has been supported by the Past Global Changes (PAGES) Data Stewardship Scholarship no. 102 and the BMBF Federal Ministry of Education and Science, PalMod project (German Climate Modelling Initiative) – phase II (grant no. 01LP19[14-26][A-E]).
This paper was edited by Alessio Rovere and reviewed by Christine Lane and one anonymous referee.
Beckett, A., Blanchet, C., Brauser, A., Kearney, R., Martin-Puertas, C., Matthews, I., Mittelbach, K., Palmer, A., Ramisch, A., and Brauer, A.: Major Element and Trace Element Compositions of Tephra Layers Found in European Varved Lake Records, V. 2.0, GFZ Data Services [data set], https://doi.org/10.5880/fidgeo.2023.015, 2022.
Blockley, S. P. E., Pyne-O'Donnell, S. D. F., Lowe, J. J., Matthews, I. P., Stone, A., Pollard, A. M., Turney, C. S. M., and Molyneux, E. G.: A new and less destructive laboratory procedure for the physical separation of distal glass tephra shards from sediments, Quaternary Sci. Rev., 24, 1952–1960, https://doi.org/10.1016/j.quascirev.2004.12.008, 2005.
Bonk, A., Müller, D., Ramisch, A., Kramkowski, M. A., Noryśkiewicz, A. M., Sekudewicz, I., Gąsiorowski, M., Luberda-Durnaś, K., Słowiński, M., Schwab, M., Tjallingii, R., Brauer, A., and Błaszkiewicz, M.: Varve microfacies and chronology from a new sediment record of Lake Gościąż (Poland), Quaternary Sci. Rev., 251, 106715, https://doi.org/10.1016/j.quascirev.2020.106715, 2021.
Brauer, A.: Annually Laminated Lake Sediments and Their Palaeoclimatic Relevance, in: The Climate in Historical Times: Towards a Synthesis of Holocene Proxy Data and Climate Models, edited by: Fischer, H., Kumke, T., Lohmann, G., Flöser, G., Miller, H., von Storch, H., and Negendank, J. F. W., Springer, Berlin, Heidelberg, 109–127, https://doi.org/10.1007/978-3-662-10313-5_7, 2004.
Brauer, A., Haug, G. H., Dulski, P., Sigman, D. M., and Negendank, J. F. W.: An abrupt wind shift in western Europe at the onset of the Younger Dryas cold period, Nat. Geosci., 1, 520–523, https://doi.org/10.1038/ngeo263, 2008.
Bronk Ramsey, C., Housley, R. A., Lane, C. S., Smith, V. C., and Pollard, A. M.: The RESET tephra database and associated analytical tools, Quaternary Sci. Rev., 118, 33–47, https://doi.org/10.1016/j.quascirev.2014.11.008, 2015a.
Bronk Ramsey, C., Albert, P. G., Blockley, S. P., Hardiman, M., Housley, R. A., Lane, C. S., Lee, S., Matthews, I. P., Smith, V. C., and Lowe, J. J.: Improved age estimates for key Late Quaternary European tephra horizons in the RESET lattice, Quaternary Sci. Rev., 118, 18–32, https://doi.org/10.1016/j.quascirev.2014.11.007, 2015b.
Clark, J. S., Merkt, J., and Muller, H.: Post-Glacial Fire, Vegetation, and Human History on the Northern Alpine Forelands, South-Western Germany, J. Ecol., 77, 897–925, https://doi.org/10.2307/2260813, 1989.
Dörfler, W., Feeser, I., van den Bogaard, C., Dreibrodt, S., Erlenkeuser, H., Kleinmann, A., Merkt, J., and Wiethold, J.: A high-quality annually laminated sequence from Lake Belau, Northern Germany: Revised chronology and its implications for palynological and tephrochronological studies, Holocene, 22, 1413–1426, https://doi.org/10.1177/0959683612449756, 2012.
Dräger, N., Theuerkauf, M., Szeroczyńska, K., Wulf, S., Tjallingii, R., Plessen, B., Kienel, U., and Brauer, A.: Varve microfacies and varve preservation record of climate change and human impact for the last 6000 years at Lake Tiefer See (NE Germany), Holocene, 27, 450–464, https://doi.org/10.1177/0959683616660173, 2017.
Haenssler, E., Nadeau, M.-J., Vött, A., and Unkel, I.: Natural and human induced environmental changes preserved in a Holocene sediment sequence from the Etoliko Lagoon, Greece: New evidence from geochemical proxies, Quatern. Int., 308–309, 89–104, https://doi.org/10.1016/j.quaint.2012.06.031, 2013.
Haflidason, H., Regnéll, C., Pyne-O'Donnell, S., and Svendsen, J. I.: Extending the known distribution of the Vedde Ash into Siberia: occurrence in lake sediments from the Timan Ridge and the Ural Mountains, northern Russia, Boreas, 48, 444–451, https://doi.org/10.1111/bor.12354, 2019.
Jones, G., Lane, C. S., Brauer, A., Davies, S. M., de Bruijn, R., Engels, S., Haliuc, A., Hoek, W. Z., Merkt, J., Sachse, D., Turner, F., and Wagner-Cremer, F.: The Lateglacial to early Holocene tephrochronological record from Lake Hämelsee, Germany: a key site within the European tephra framework, Boreas, 47, 28–40, https://doi.org/10.1111/bor.12250, 2018.
Jones, G., Davies, S. M., Staff, R. A., Loader, N. J., Davies, S. J., and Walker, M. J. C.: Traces of volcanic ash from the Mediterranean, Iceland and North America in a Holocene record from south Wales, UK, J. Quaternary Sci., 35, 163–174, https://doi.org/10.1002/jqs.3141, 2020.
Kearney, R., Albert, P. G., Staff, R. A., Pál, I., Veres, D., Magyari, E., and Ramsey, C. B.: Ultra-distal fine ash occurrences of the Icelandic Askja-S Plinian eruption deposits in Southern Carpathian lakes: New age constraints on a continental scale tephrostratigraphic marker, Quaternary Sci. Rev., 188, 174–182, https://doi.org/10.1016/j.quascirev.2018.03.035, 2018.
Lane, C. S., Blockley, S. P. E., Bronk Ramsey, C., and Lotter, A. F.: Tephrochronology and absolute centennial scale synchronisation of European and Greenland records for the last glacial to interglacial transition: A case study of Soppensee and NGRIP, Quatern. Int., 246, 145–156, https://doi.org/10.1016/j.quaint.2010.11.028, 2011a.
Lane, C. S., Andrič, M., Cullen, V. L., and Blockley, S. P. E.: The occurrence of distal Icelandic and Italian tephra in the Lateglacial of Lake Bled, Slovenia, Quaternary Sci. Rev., 30, 1013–1018, https://doi.org/10.1016/j.quascirev.2011.02.014, 2011b.
Lane, C. S., Brauer, A., Blockley, S. P. E., and Dulski, P.: Volcanic ash reveals time-transgressive abrupt climate change during the Younger Dryas, Geology, 41, 1251–1254, https://doi.org/10.1130/G34867.1, 2013.
Lane, C. S., Brauer, A., Martín-Puertas, C., Blockley, S. P. E., Smith, V. C., and Tomlinson, E. L.: The Late Quaternary tephrostratigraphy of annually laminated sediments from Meerfelder Maar, Germany, Quaternary Sci. Rev., 122, 192–206, https://doi.org/10.1016/j.quascirev.2015.05.025, 2015.
Lauterbach, S., Brauer, A., Andersen, N., Danielopol, D. L., Dulski, P., Hüls, M., Milecka, K., Namiotko, T., Obremska, M., Von Grafenstein, U., and Participants, D.: Environmental responses to Lateglacial climatic fluctuations recorded in the sediments of pre-Alpine Lake Mondsee (northeastern Alps), J. Quaternary Sci., 26, 253–267, https://doi.org/10.1002/jqs.1448, 2011a.
Lauterbach, S., Brauer, A., Andersen, N., Danielopol, D. L., Dulski, P., Hüls, M., Milecka, K., Namiotko, T., Plessen, B., Grafenstein, U. V., and Participants, D.: Multi-proxy evidence for early to mid-Holocene environmental and climatic changes in northeastern Poland, Boreas, 40, 57–72, https://doi.org/10.1111/j.1502-3885.2010.00159.x, 2011b.
Macleod, A., Brunnberg, L., Wastegård, S., Hang, T., and Matthews, I. P.: Lateglacial cryptotephra detected within clay varves in Östergötland, south-east Sweden, J. Quaternary Sci., 29, 605–609, https://doi.org/10.1002/jqs.2738, 2014.
Mahony, S. H., Barnard, N. H., Sparks, R. S. J., and Rougier, J. C.: VOLCORE, a global database of visible tephra layers sampled by ocean drilling, Sci. Data, 7, 330, https://doi.org/10.1038/s41597-020-00673-1, 2020.
Martin-Puertas, C., Walsh, A. A., Blockley, S. P. E., Harding, P., Biddulph, G. E., Palmer, A., Ramisch, A., and Brauer, A.: The first Holocene varve chronology for the UK: Based on the integration of varve counting, radiocarbon dating and tephrostratigraphy from Diss Mere (UK), Quat. Geochronol., 61, 101134, https://doi.org/10.1016/j.quageo.2020.101134, 2021.
Merkt, J., Müller, H., Knabe, W., Müller, P., and Weiser, T.: The early Holocene Saksunarvatn tephra found in lake sediments in NW Germany, Boreas, 22, 93–100, https://doi.org/10.1111/j.1502-3885.1993.tb00168.x, 1993.
Müller, D., Tjallingii, R., Płóciennik, M., Luoto, T. P., Kotrys, B., Plessen, B., Ramisch, A., Schwab, M. J., Błaszkiewicz, M., Słowiński, M., and Brauer, A.: New insights into lake responses to rapid climate change: the Younger Dryas in Lake Gościąż, central Poland, Boreas, 50, 535–555, https://doi.org/10.1111/bor.12499, 2021.
Newton, A. J., Dugmore, A. J., and Gittings, B. M.: Tephrabase: tephrochronology and the development of a centralised European database, J. Quaternary Sci., 22, 737–743, https://doi.org/10.1002/jqs.1094, 2007.
Ojala, A. E. K., Francus, P., Zolitschka, B., Besonen, M., and Lamoureux, S. F.: Characteristics of sedimentary varve chronologies – A review, Quaternary Sci. Rev., 43, 45–60, https://doi.org/10.1016/j.quascirev.2012.04.006, 2012.
Palmer, A. P., Matthews, I. P., Lowe, J. J., MacLeod, A., and Grant, R.: A revised chronology for the growth and demise of Loch Lomond Readvance (“Younger Dryas”) ice lobes in the Lochaber area, Scotland, Quaternary Sci. Rev., 248, 106548, https://doi.org/10.1016/j.quascirev.2020.106548, 2020.
Ramisch, A., Brauser, A., Dorn, M., Blanchet, C., Brademann, B., Köppl, M., Mingram, J., Neugebauer, I., Nowaczyk, N., Ott, F., Pinkerneil, S., Plessen, B., Schwab, M. J., Tjallingii, R., and Brauer, A.: VARDA (VARved sediments DAtabase) – providing and connecting proxy data from annually laminated lake sediments, Earth Syst. Sci. Data, 12, 2311–2332, https://doi.org/10.5194/essd-12-2311-2020, 2020.
Rasmussen, S. O., Vinther, B. M., Clausen, H. B., and Andersen, K. K.: Early Holocene climate oscillations recorded in three Greenland ice cores, Quaternary Sci. Rev., 26, 1907–1914, https://doi.org/10.1016/j.quascirev.2007.06.015, 2007.
Reinig, F., Wacker, L., Jöris, O., Oppenheimer, C., Guidobaldi, G., Nievergelt, D., Adolphi, F., Cherubini, P., Engels, S., Esper, J., and Land, A.: Precise date for the Laacher See eruption synchronizes the Younger Dryas. Nature, 595, 66–69, https://doi.org/10.1038/s41586-021-03608-x, 2021.
Schmidt, R., van den Bogaard, C., Merkt, J., and Müller, J.: A new Lateglacial chronostratigraphic tephra marker for the south-eastern Alps: The Neapolitan Yellow Tuff (NYT) in Längsee (Austria) in the context of a regional biostratigraphy and palaeoclimate, Quatern. Int., 88, 45–56, https://doi.org/10.1016/S1040-6182(01)00072-6, 2002.
Stihler, S. D., Stone, D. B., and Beget, J. E.: “Varve” counting vs. tephrochronology and 137Cs and 210Pb dating: A comparative test at Skilak Lake, Alaska, Geology, 20, 1019–1022, https://doi.org/10.1130/0091-7613(1992)020<1019:VCVTAC>2.3.CO;2, 1992.
Swierczynski, T., Lauterbach, S., Dulski, P., and Brauer, A.: Late Neolithic Mondsee Culture in Austria: living on lakes and living with flood risk?, Clim. Past, 9, 1601–1612, https://doi.org/10.5194/cp-9-1601-2013, 2013.
Timms, R. G. O., Matthews, I. P., Lowe, J., Palmer, A. P., Weston, D. J., MacLeod, A., and Blockley, S. P. E.: Establishing tephrostratigraphic frameworks to aid the study of abrupt climatic and glacial transitions: a case study of the Last Glacial-Interglacial Transition in the British Isles (c. 16–8 ka BP), Earth-Sci. Rev., 192, 34–64, https://doi.org/10.1016/j.earscirev.2019.01.003, 2019.
Vogel, H., Zanchetta, G., Sulpizio, R., Wagner, B., and Nowaczyk, N.: A tephrostratigraphic record for the last glacial–interglacial cycle from Lake Ohrid, Albania and Macedonia, J. Quaternary Sci., 25, 320–338, https://doi.org/10.1002/jqs.1311, 2010.
von Grafenstein, U., Erlenkeuser, H., Müller, J., Jouzel, J., and Johnsen, S.: The cold event 8200 years ago documented in oxygen isotope records of precipitation in Europe and Greenland, Clim. Dynam., 14, 73–81, https://doi.org/10.1007/s003820050210, 1998.
von Grafenstein, U., Erlenkeuser, H., Brauer, A., Jouzel, J., and Johnsen, S. J.: A Mid-European Decadal Isotope-Climate Record from 15,500 to 5000 Years B.P., Science, 284, 1654–1657, https://doi.org/10.1126/science.284.5420.1654, 1999.
Wagner, B., Aufgebauer, A., Vogel, H., Zanchetta, G., Sulpizio, R., and Damaschke, M.: Late Pleistocene and Holocene contourite drift in Lake Prespa (Albania/F.Y.R. of Macedonia/Greece), Quatern. Int., 274, 112–121, https://doi.org/10.1016/j.quaint.2012.02.016, 2012.
Wallace, K. L., Bursik, M. I., Kuehn, S., Kurbatov, A. V., Abbott, P., Bonadonna, C., Cashman, K., Davies, S. M., Jensen, B., Lane, C., Plunkett, G., Smith, V. C., Tomlinson, E., Thordarsson, T., and Walker, J. D.: Community established best practice recommendations for tephra studies – from collection through analysis, Sci. Data, 9, 447, https://doi.org/10.1038/s41597-022-01515-y, 2022.
Walsh, A. A., Blockley, S. P. E., Milner, A. M., Matthews, I. P., and Martin-Puertas, C.: Complexities in European Holocene cryptotephra dispersal revealed in the annually laminated lake record of Diss Mere, East Anglia, Quat. Geochronol., 66, 101213, https://doi.org/10.1016/j.quageo.2021.101213, 2021.
Walsh, A. A., Blockley, S. P. E., Milner, A. M., and Martin-Puertas, C.: Updated age constraints on key tephra markers for NW Europe based on a high-precision varve lake chronology, Quaternary Sci. Rev., 300, 107897, https://doi.org/10.1016/j.quascirev.2022.107897, 2023.
Wulf, S., Kraml, M., Brauer, A., Keller, J., and Negendank, J. F. W.: Tephrochronology of the 100 ka lacustrine sediment record of Lago Grande di Monticchio (southern Italy), Quatern. Int., 122, 7–30, https://doi.org/10.1016/j.quaint.2004.01.028, 2004.
Wulf, S., Kraml, M., and Keller, J.: Towards a detailed distal tephrostratigraphy in the Central Mediterranean: The last 20,000 yrs record of Lago Grande di Monticchio, J. Volcanol. Geoth. Res., 177, 118–132, https://doi.org/10.1016/j.jvolgeores.2007.10.009, 2008.
Wulf, S., Keller, J., Paterne, M., Mingram, J., Lauterbach, S., Opitz, S., Sottili, G., Giaccio, B., Albert, P. G., Satow, C., Tomlinson, E. L., Viccaro, M., and Brauer, A.: The 100–133 ka record of Italian explosive volcanism and revised tephrochronology of Lago Grande di Monticchio, Quaternary Sci. Rev., 58, 104–123, https://doi.org/10.1016/j.quascirev.2012.10.020, 2012.
Wulf, S., Ott, F., Słowiński, M., Noryśkiewicz, A. M., Dräger, N., Martin-Puertas, C., Czymzik, M., Neugebauer, I., Dulski, P., Bourne, A. J., Błaszkiewicz, M., and Brauer, A.: Tracing the Laacher See Tephra in the varved sediment record of the Trzechowskie palaeolake in central Northern Poland, Quaternary Sci. Rev. 76, 129–139, https://doi.org/10.1016/j.quascirev.2013.07.010, 2013.
Wulf, S., Dräger, N., Ott, F., Serb, J., Appelt, O., Guðmundsdóttir, E., van den Bogaard, C., Słowiński, M., Błaszkiewicz, M., and Brauer, A.: Holocene tephrostratigraphy of varved sediment records from Lakes Tiefer See (NE Germany) and Czechowskie (N Poland), Quaternary Sci. Rev., 132, 1–14, https://doi.org/10.1016/j.quascirev.2015.11.007, 2016.
Zillén, L. M., Wastegård, S., and Snowball, I. F.: Calendar year ages of three mid-Holocene tephra layers identified in varved lake sediments in west central Sweden, Quaternary Sci. Rev., 21, 1583–1591, https://doi.org/10.1016/S0277-3791(02)00036-7, 2002.
Zolitschka, B., Francus, P., Ojala, A. E. K., and Schimmelmann, A.: Varves in lake sediments – a review, Quaternary Sci. Rev., 117, 1–41, https://doi.org/10.1016/j.quascirev.2015.03.019, 2015.