the Creative Commons Attribution 4.0 License.
the Creative Commons Attribution 4.0 License.
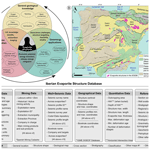
IESDB – the Iberian Evaporite Structure Database
Eloi González-Esvertit
Juan Alcalde
Evaporites flow in the solid state under relatively low differential stress and have unique mechanical properties compared to other sedimentary rocks. Worldwide, they control the structural and stratigraphical architecture of many basins and orogens in ancient and active tectonic settings. Moreover, they host mineral deposits and play a key role in petroleum systems because they typically act as seals due to their low permeability, and their ability to flow results in the formation of structural hydrocarbon traps such as folds and faults in their encasing rocks. Additionally, evaporite structures can be used as subsurface storage sites for geo-energy applications and nuclear waste. The systematic characterisation of subsurface evaporite structures is thus key for the development of geoscience-based technologies to address societal challenges. Owing to their value, massive amounts of surface and subsurface information about (among others) the stratigraphy, structure, geochemistry, and petrophysical properties of evaporite structures and their surrounding rocks have been acquired by Earth scientists, petroleum and mining exploration companies, and geological surveys. However, the data often appear segregated (i.e. in the form of database fragments, scientific articles, and unpublished reports), are not systematically organised, and are sometimes not fully accessible. This contribution presents the Iberian Evaporite Structure Database (IESDB), the first comprehensive assessment that focuses on evaporite structures carried out in any region of the world. The IESDB includes information and figures for 150 evaporite structures and their surrounding rocks inventoried in Spain and Portugal and is sourced from other six thematic databases and more than 1500 published and unpublished scientific documents. The database targets undeformed to slightly deformed evaporite successions, outcropping and buried diapirs, evaporite-cored anticlines, evaporite-detached thrusts, and allochthonous evaporite bodies. Collated data include information about the structure, stratigraphy, event chronology, surface and subsurface data availability, mining activity, and key bibliographic references. The IESDB follows the FAIR principles of database management (Findable, Accessible, Interoperable, and Reusable) and is presented as an interactive web page and an open-access database, where indexed structures can easily be selected from a map or browser and filtered by a search engine. The IESDB intends to be a useful resource for teaching (i.e. pointing out examples of exceptional evaporite outcrops), academic and industry research (i.e. identifying knowledge deficits on specific structures or tectonic settings), and for the sustainable exploration and appraisal of mineral resources and geo-energy applications (i.e. representing a terminus a quo for site selection and suitability assessment). The framework provided by the IESDB is an opportunity to enhance the scientific research on Iberian evaporite structures in Spain and Portugal and to take advantage of their scientific and economic potential to tackle important societal challenges faced by these countries. The IESDB is freely available at https://iesdb.eu (last access: 15 June 2023) and the datasets can be downloaded from https://doi.org/10.20350/digitalCSIC/14586 (González-Esvertit et al., 2022).
- Article
(7312 KB) - Full-text XML
- BibTeX
- EndNote
Evaporites are rocks that form by precipitation from a surface or near-surface saturated brine in hydrologies mainly controlled by solar evaporation (Warren, 2016), both in marine and continental sedimentary environments. They are key components of sedimentary basins and orogens and can control the stratigraphy and structure of many ancient and active extensional, contractional, and strike-slip tectonic settings worldwide (Fig. 1). The mechanical properties of evaporite rocks are unique and differ from those of other sedimentary rocks, such as clastic and carbonate rocks. Evaporites have a unique rheology that allows them to flow in the solid state under relatively low differential stresses, thus behaving in a more viscous manner, whilst their incompressibility makes them one of the least dense materials of the Earth's crust (e.g. Hudec and Jackson, 2007). As a result, evaporitic sequences help to constrain the evolution of sedimentary basins and mountain ranges (Fig. 1), frequently acting as detachments for thrusts and forming structures such as salt diapirs and walls, which typically produce folding and faulting of their encasing rocks. Evaporites tend to be impermeable at shallow depths and hence normally act as barriers to fluid flow. The combination of these particular petrophysical properties and the ability to form hydrocarbon traps in their surrounding rocks make evaporites key components of petroleum systems (Weijermars et al., 1993). Accordingly, they control the reservoir distribution and hydrocarbon migration in some of the world's major hydrocarbon provinces, such as the Persian Gulf, North Sea, or Gulf of Mexico basins, among others (Warren, 2006; Hudec and Jackson, 2012; Jackson and Hudec, 2017, and references therein).
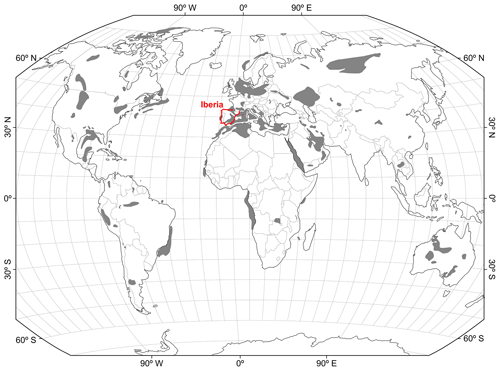
Figure 1Global distribution of deformed evaporite basins, compiled from Hudec and Jackson (2007, 2012) and Warren (2010).
We hereafter use the term “evaporite structure” to refer to any outcropping or buried rock body mappable at the 1:25 000 scale, dominantly composed of evaporite minerals, and geographically limited by geological boundaries such as stratigraphic or diapiric contacts, faults, or fold limbs. The principal evaporite minerals, corresponding to the sulfate and chloride groups, are among the most mined mineral commodities worldwide, with an estimated production of over 140 000 × 106 t in 2019 (US Geological Survey, 2020). In addition, salt caverns built within evaporite structures are considered suitable environments for geo-energy applications, such as the storage of oil, natural gas, compressed air, and hydrogen energy (Lux, 2009; Ozarslan, 2012; Wang et al., 2017) and are one of the key geological repositories of deep nuclear waste (e.g. International Atomic Energy Agency, 2003).
Given the important applications of evaporite rocks for our society, the geological characterisation, classification, and indexation of surface and subsurface evaporite structures are essential for the implementation of new geoscience-based technologies in the near future, especially aiming at the energy transition and climate change mitigation (Tarkowski and Czapowski, 2018; Saucier, 2021; Duffy et al., 2023). In the Iberian Peninsula (hereafter referred to as Iberia when including the Balearic Islands; Figs. 1, 2), a large number of evaporite structures have been historically recognised and studied (e.g. see a review of gypsum resources in Escavy et al., 2012). Iberian evaporites range from Upper Palaeozoic to Quaternary in age and are mainly found as (1) undeformed to slightly deformed sedimentary layers, (2) passive, active, and reactivated diapirs, (3) evaporite-cored anticlines, (4) evaporite-detached thrusts, and (5) allochthonous evaporite bodies. In addition, some deformed structures have evolved through different stages and types of growth and deformation, resulting in complex histories of stacked halokinetic movements. Examples of these complex evolutionary records in the Iberian Peninsula include the Aulet, Montiberri, Adons, and Viu de Llevata evaporite structures in the southern Pyrenees (Saura et al., 2016; Dalton, 2019) or the Bakio, Bermeo, Munguía and Gernika evaporite structures in the Basque Arc (Cámara, 2017; Soto et al., 2017).
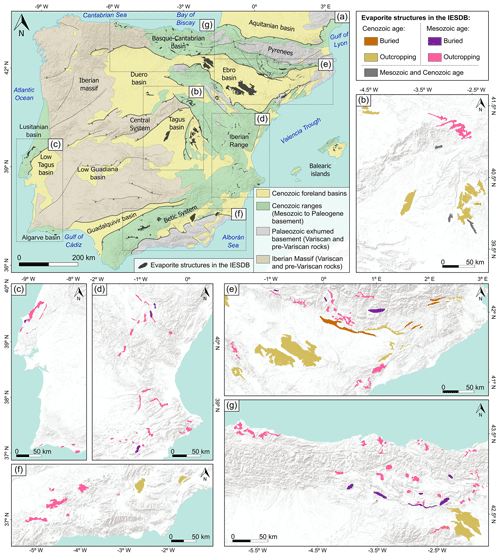
Figure 2(a) Simplified tectonic map of Iberia, after the geological and structural maps of Andeweg (2002), Casas-Sainz and de Vicente (2009), Vergés et al. (2019), and Vergés and Fernàndez (2006). Detail of the evaporite structures indexed in the IESDB (n=150) is provided in central Iberia (b), the Lusitanian and Algarve basins (c), the Iberian range and the eastern Betic System (d), the central and western Betic System (e), and the Basque–Cantabrian basin (f). Base maps are modified from Esri®.
Iberian evaporite structures have mostly been studied individually and with a certain lack of regional correlation, and only a few review articles summarise their occurrence and significance from a basin- or orogen-scale perspective (Cámara, 2017; Cámara and Flinch, 2017; Martínez del Olmo et al., 2015). Moreover, neither inventories nor databases focused on evaporite structures are available in Iberia or, to the best of our knowledge, in other worldwide regions, despite the existence of large amounts of valuable and open access, surface- and subsurface-derived stratigraphic and structural data. In order to fill this gap, and to foster the use of evaporite resources for the energy transition (both as subsurface storage sites for geo-energy applications and as mineral resources), here we present the Iberian Evaporite Structure Database (IESDB), which is an overall comprehensive assessment of evaporite structures in Iberia. The IESDB includes information on the location, type, structure, stratigraphy, event chronology, seismic and well data availability, and mining activity (historical and active) of 150 evaporite structures of Iberia and their surrounding rocks (Fig. 2). The database also includes a comprehensive literature review (more than 1500 published and unpublished references), in addition to a set of geological maps, sketches, and cross sections (1200 figures, with 8 for each indexed evaporite structure). The IESDB is sourced from the past 50-year-long efforts of the scientific and industrial community in gathering data from evaporite structures. The database has been built and formatted with rigorous data selection and data ordering criteria and is presented here as a freely available, open-to-feedback website (https://iesdb.eu, last access: 15 June 2023). The IESDB follows the FAIR principles of database management (Findable, Accessible, Interoperable, and Reusable; Wilkinson et al., 2016) and can be operated as a geographic information system (GIS)-based interactive tool, allowing the user to navigate through the indexed evaporite structures by direct map selection or multi-criteria filtering.
The IESDB aims to provide the research and industrial communities with a user-friendly “evaporite radar” for any scientific, industrial, societal, or teaching purpose. The IESDB aims to be a collaborative project in which users can provide feedback on the information collected, propose new evaporite structures to be indexed or discuss the geological aspects of those already characterised. The database will be periodically updated as new data become available. Hence, more evaporite structures can be added in the IESDB as they are discovered, and new published data that provide reinterpretations or represent a significant knowledge widening of the already inventoried structures will also be integrated in the IESDB during the scheduled updates.
Iberia is located at the western end of the Himalayan–Alpine collision zone, a Cenozoic belt formed after the Paleo-Tethys closure during the Alpine orogeny (Rosenbaum et al., 2002). However, Iberia records older (nearly 600 Ma) geodynamic events that make it a geologically diverse region (Fig. 2). The main events that affected this region can be broadly summarised as the (1) Early Cambrian to Early Permian compression, magmatism, and metamorphism (Variscan cycle; e.g. Simancas, 2019, and references therein), (2) Mesozoic rifting and basin formation (early Alpine cycle; e.g. Gómez et al., 2019, and references therein), and (3) Cenozoic compression and mountain range development by the inversion of Mesozoic rift basins (Alpine orogeny; e.g. Rosenbaum et al., 2002; Vergés et al., 2019, and references therein). Since no Palaeozoic evaporites are present in Iberia, we hereafter focus on the Iberian geodynamics and evaporite rock evolution from the early Mesozoic to the Quaternary.
The Mesozoic evolution of Iberia was governed by widespread rifting periods along its northern, eastern, and western margins, ending with the continental break-up along the Bay of Biscay, Ligurian Tethys, and Atlantic plate boundaries, respectively (Salas and Casas, 1993; Casas-Sainz and de Vicente, 2009; Vergés et al., 2019). As a result, shallow to deep marine sedimentary basins formed at the main rifting zones (Fig. 2a). These are (1) the intracratonic Iberian basin, represented nowadays by the Iberian range, Maestrat Basin, Cameros Massif, and Catalan Coastal Ranges (Salas and Casas, 1993; Vergés and Fernàndez, 2006), (2) the Basque–Cantabrian–Aquitanian–Pyrenean basin, which currently forms the Basque–Cantabrian basin and the Pyrenees (Vergés and Fernàndez, 2006; Soto et al., 2008; López-Gómez et al., 2019), (3) the Atlantic Margin rift basins, represented onshore by the Lusitanian basin (Rasmussen et al., 1998), and (4) the Atlantic–Ligurian Tethys linkage zone, which currently forms the external Prebetic and Subbetic cordilleras within the Betic System (García-Hernández et al., 1980; Peper and Cloetingh, 1992) and the Algarve basin (Ramos et al., 2016, 2020).
Mesozoic evaporites played an important role during the Mesozoic extension and Cenozoic compression periods. Lower Mesozoic evaporites can be found within the epicontinental Triassic rocks of the Buntsandstein, Muschelkalk, and Keuper facies (Ortí et al., 2017). The uppermost Buntsandstein Rot and the Intermediate Muschelkalk M2 evaporites are scarce and restricted to the north-east of Iberia (Virgili et al., 1977; Marzo, 1980; Jurado, 1990; López-Gómez et al., 1993; Fig. 2c, d), while the Upper Triassic Keuper facies are the most abundant Mesozoic evaporite deposits and can be found in all the Mesozoic basins (Ortí et al., 2017; Fig. 2). Jurassic evaporites (mainly Upper Rhaetian–Hettangian) include the Anhydrite member (Ortí, 1987; Ortí et al., 2017) along northern and eastern Iberia (Fig. 2c, d, f), the Dagorda Formation (Rasmussen et al., 1998; Wilson et al., 1989) in the Lusitanian basin, and the Hettangian evaporites (Ramos et al., 2016, 2020) in the Algarve basin (Fig. 2b). Cretaceous evaporites are scarce and geographically restricted (Segura et al., 2006; Escavy et al., 2012; Quijada et al., 2013), so, to our knowledge, no stratigraphic correlations between units have been reported to date.
Mesozoic evaporite deformation mainly happened in the form of Upper Triassic–Lower Jurassic salt mobilisation and diapiric raise between the Early Jurassic and the Late Cretaceous in all the Iberian Mesozoic basins (Vergés et al., 2019). Diapirs with a nearly circular outcrop geometry are found within the Basque–Cantabrian (Cámara, 2017; Roca et al., 2021) and Algarve (Ramos et al., 2016, 2017) basins (Fig. 2b, f), while halokinetic structures and stratal geometries have been overprinted during the Alpine compression in other Iberian domains (e.g. Escosa et al., 2018; Burrel and Teixell, 2021; Fig. 2c, d, e). Thus, most of the evaporite structures nowadays correspond to anticline cores, detachment horizons, and allochthonous evaporite bodies (McClay et al., 2004; Martínez del Olmo et al., 2015; Vergés et al., 2020).
Cenozoic geodynamics in Iberia have been governed by contractional tectonics as a result of the convergence between the European, Iberian, and African plates since the late Santonian–early Campanian (Puigdefàbregas and Souquet, 1986; Macchiavelli et al., 2017; Vergés et al., 2019). Asynchronous deformation occurred along the Iberian margins and within intraplate domains. This caused the inversion of Mesozoic rift basins, giving rise to the Cenozoic ranges and foreland basins that shape the current geological configuration and landscape of Iberia. The origin and evolution of the Cenozoic ranges at the plate boundaries (i.e. the Pyrenees and the Betic System; Fig. 2d, e) and within the plate's interior (i.e. the Iberian range; Fig. 2c) were controlled by the distribution of evaporite deposits of the Mesozoic to Paleogene age. These rocks acted as detachment levels during thrust development (Vergés and Fernàndez, 2006; Muñoz et al., 2018). In summary, evaporite successions that were deposited from the early Mesozoic to the Paleogene have been involved in different tectonic processes and are nowadays deformed. On the contrary, Neogene to Quaternary evaporites within the Cenozoic foreland basins have practically been unaffected by deformation.
As a result of uplifting during the Cenozoic, most of the large Cenozoic basins of Iberia evolved from exorheic to endorheic drainage conditions during the Paleogene and Neogene, except for the Guadalquivir basin that remained connected to the Atlantic Ocean. This stage produced intense evaporation and favoured evaporite deposition, mostly in the Duero, Ebro, and High Tagus foreland basins (Escavy et al., 2012; Fig. 2a, d, f). Furthermore, the (minor and more restricted) Neogene to Quaternary basins of the Betic System have also been isolated from the Atlantic Ocean and the Mediterranean Sea since the late Miocene, giving rise to a diachronic formation of evaporites, followed by a complete disconnection and basin continentalisation (Fig. 2e; Galdeano and Vera, 1992; Playà et al., 2000; Galindo-Zaldívar et al., 2019; García-Veigas et al., 2020, and references therein). The Baza basin, the largest Neogene–Quaternary intra-mountain basin of the eastern Betic chain, evolved from endorheic to exorheic after being captured by the drainage network of the Guadalquivir River since the latest middle Pleistocene (Gibert et al., 2007; García-Tortosa et al., 2008).
The IESDB includes a complete review of 150 evaporite structures and their surrounding rocks that crop out or are buried onshore Iberia, including undeformed to slightly deformed successions, evaporite-cored anticlines, diapirs, evaporite-detached thrusts, and allochthonous evaporite bodies (Fig. 2). The structure of the IESDB is based on a process of selection, compilation, and classification of the evaporite structures and their geological information. Thus, and considering that this workflow could be adapted and applied for inventorying other geological structures and replicated in other regions of the world, an overview of the IESDB structure is presented in this section, together with their building process and final datasets.
3.1 Outline
To ensure that the database includes only relevant entries, the evaporite structures included in the IESDB were selected according with the following criteria: (1) they are located onshore of Iberia, (2) when cropping out, they should have a minimum exposed area of 0.5 km2, (3) when buried, seismic and/or well data should be available, and they should have a minimum horizontal intersection area of 1.5 km2 at 0.5 km depth, (4) the structure or deposit should be mentioned in at least 10 peer-reviewed articles, and (5) the stratigraphy and structure of the evaporite deposit and, when applicable, the overburden, halokinetic, or post-kinematic sequences should be directly addressed in at least one peer-reviewed article or industry or government report. Each inventoried structure includes written and visual information about the geometry, stratigraphy, and structural evolution of the evaporites and their surrounding rocks together with (when possible) the evaporite flow driving mechanisms and their approximate age.
The database can be accessed and inter-operated by two different procedures. The first is the bulk download of the raw datasets, where information is categorised according to its type and nature (see Sect. 3.3), and the second is through the dynamic and open-access IESDB web page (Fig. 3), where information is classified in 150 unique web sections that correspond to the indexed evaporite structures. The latter method has been conceived as being interactive and user-friendly. This means that the indexed evaporite structures can be easily selected on a map (Fig. 3c) or can be browsed through a search engine by the following classification criterions: structure type, deformed/undeformed, geological setting, cropping out/buried, evaporite unit(s) names, evaporite unit(s) age, evaporite unit(s) origin, salt structure classification, according to the schemes of Jackson and Talbot (1991) and Hudec and Jackson (2007), and age of evaporite flow (Fig. 3b). The IESDB web page structure is inspired by e-commerce management tools to treat the inventoried structures as online products for sale, so each evaporite structure has a unique web section in which written and illustrated information is displayed over several sub-sections, following a common layout (Fig. 3a). A guide section is also provided on the IESDB web site and includes guidelines for inter-operating the database, in addition to information about the origin and meaning of the different types of data related to the evaporite structures.
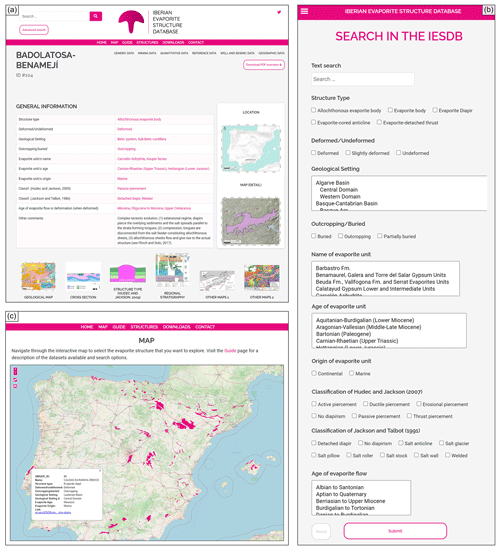
Figure 3Screenshots of the IESDB web page (https://iesdb.eu, last access: 15 June 2023). (a) Unique web section of the Badolatosa–Benamejí evaporite structure (ID no. 104; web version). (b) Filtering criteria included in the IESDB advanced search engine (mobile version). (c) Interactive map, with all the evaporite structures included in the IESDB; by clicking on one evaporite structure, the user will see a pop-up overview of the information and the corresponding link to the unique web section of the selected structure. Base map in panel (c) modified from © OpenStreetMap contributors 2022. Distributed under the Open Data Commons Open Database License (ODbL) v1.0.
The IESDB aims to be a dynamic project open to collaboration and with constant improvement and expansion. We encourage users to contact the database managers and submit new information or suggest corrections to expand and improve the database. New evaporite structures can be added as new research articles or public reports are published to provide new surface or subsurface information. Moreover, new data and reinterpretations that concern the already-inventoried structures, in addition to feedback provided by users via a contact page, will be considered to implement the data updates.
3.2 Data sources
The IESDB is sourced from over 1500 classified references and six thematic open-access databases. Classified references include, among others, published articles and conference abstracts, books, book chapters, doctoral theses and published and unpublished industry and government reports. Geological maps and cross sections provided by IGME (Instituto Geológico y Minero de España or Spanish Geological Survey; http://info.igme.es/cartografiadigital/geologica/Magna50.aspx, last access: 15 June 2023), LNEG (Laboratorio Nacional de Energia e Geologia or Portuguese Geological Survey; https://geoportal.lneg.pt/mapa/, last access: 15 June 2023), and ICGC (Institut Cartogràfic I Geològic de Catalunya or Catalonian Geological Survey; https://www.icgc.cat/Administracio-i-empresa/Descarregues/Cartografia-geologica-i-geotematica, last access: 15 June 2023) have also been used as source layers for data mining. The location and geometry of the available seismic and well data for each structure was obtained from the SeisDARE database (DeFelipe et al., 2021), together with the GEODE map series (IGME; https://info.igme.es/cartografiadigital/geologica/Geode.aspx, last access: 15 June 2023), the SIGEOF database (IGME; https://info.igme.es/SIGEOF/, last access: 15 June 2023) and the well data catalogue (IGME; https://info.igme.es/Litoteca/, last access: 15 June 2023), and integrated in a GIS-based environment. Mining inventories included in the Spanish National Mining Cadastre (Ministerio para la Transición Ecológica y el Reto Demográfico, Spanish Government; https://energia.gob.es/mineria/Paginas/catastro.aspx, last access: 15 June 2023) and in the Panorama Minero inventory (IGME; http://www.igme.es/PanoramaMinero/PMLin.htm, last access: 15 June 2023) have also been used to obtain the mining-related information of each structure. A detailed overview of the references related to each inventoried evaporite structure is provided as one of the IESDB datasets (see Sect. 3.3) and as a web sub-section on the IESDB web page. In the latter case, hyperlinks to each listed reference are included in the IESDB web page, aiming to facilitate the data query from the original data sources.
3.3 Database structure
Up to 454 information fields for each evaporite structure were manually filled by means of classification and curation of the information found in the data sources (see Sect. 3.2). The information fields were classified into seven datasets according to the type of information that they contain (Fig. 4), namely generic data (31 fields), mining data (20 fields), well and seismic data (228 fields), geographic data (120 fields), quantitative data (9 fields), reference data (38 fields), and image data (8 fields). The structures are correlated over these datasets through a unique ID that has been randomly assigned to them.
The quality and quantity of the information fields of each structure depend on its type, actual knowledge, and geoscientific interest. Thus, there are evaporite structures with complete information in all the datasets, while some information types may be missing for other structures, depending on the data availability. We established the information-filling percentage (IFP) to address the completeness of database fields for each evaporite structure. The IFP is calculated by normalising the fields that contain information with respect to the total number of fields for each dataset (Figs. 4, 5). The IFP of all the inventoried structures is over 75 % for the reference-dependent datasets, while it is more variable for the mining, well and seismic, and (partially) geographic datasets. This is because these latter fields depend totally or partially on the performance and availability of borehole and seismic surveys (Fig. 5). We describe below the nature and type of information that are included in each of the IESDB datasets.
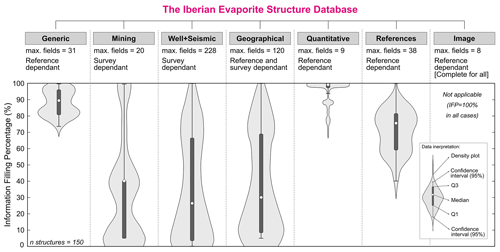
Figure 5Datasets included in IESDB with their respective statistics on the percentage of filled fields (information-filling percentage or IFP). The IFP is calculated for each structure by normalising the fields which contain information relative to the total fields of each dataset to 100 %. Note that the survey-dependent datasets show a significatively higher variability when compared with the reference-dependent datasets.
3.3.1 Generic data
Generic data (median IFP of 89 %; Fig. 5) include, for each inventoried structure, general information about the regional geological framework and of the structure and stratigraphy of the evaporites and their surrounding rocks (Fig. 4). Data corresponding to the evaporite units refer to the formation/facies names, the depositional environment, the lithological composition, the type of evaporite structure (according to Jackson and Talbot, 1991, and Hudec and Jackson, 2007), and the evaporite flow characteristics, including the age and number of deformation stages, their triggering mechanisms, and their halokinetic structures. The structural and stratigraphic information of the surrounding rocks includes the name, age, and lithological composition of the stratigraphic formation/facies, which are categorised according to whether they are post-evaporite sedimentation and pre-kinematic (e.g. overburden sequences), syn-kinematic, or post-kinematic.
Names of boreholes and seismic profiles that crosscut or are within 5 and 3 km of each structure, respectively, are also provided in the generic data, but their information is further detailed in the well and seismic and geographical data datasets described below. Historical and active mining activities in the inventoried structures include information about the exploitation methods, start and end dates of mining, and the products or sub-products that have been or are being extracted. Finally, a miscellaneous section is included to gather other relevant information about the inventoried structures. This includes the relationships between neighbouring subsurface evaporite bodies, details about their deformation history, or interpretations of their structural evolution, according to different studies.
3.3.2 Mining data
Mining data (median IFP of 41 %; Fig. 5) contain more detailed information about the mining exploitation activities in the inventoried structures (Fig. 4). A 2 km multi-ring buffer (constant distance) around the inventoried structures was used to obtain information of the open-pit mining activities above them, in addition to of the underground galleries that exploited the evaporites in the subsurface. Mining companies typically acquire and compile information on the structural, stratigraphic, and geochemical characteristics of the products and sub-products they exploit. Therefore, the names of the companies that own the concessions of active mines are also presented, together with the exploitation ID indexed in the Spanish National Mining Cadastre (Ministerio para la Transición Ecológica y el Reto Demográfico, Spanish Government; https://energia.gob.es/mineria/Paginas/catastro.aspx, last access: 15 June 2023).
3.3.3 Well and seismic data
Borehole and seismic data are key information sources of the subsurface stratigraphy and structure of the inventoried structures and their surroundings. Thus, well and seismic data (median IFP of 27 %; Fig. 5) contain expanded information about boreholes and seismic profiles located within a 5 and 3 km multi-ring buffer (constant distance) around the evaporite structures, respectively (Fig. 4). An important part of this dataset is extracted from the aforementioned IGME and SeisDARE databases (see Sect. 3.2), whereas other (private access) information was obtained from published articles and industry and government reports. The seismic line information includes the ID and name of the profiles and the name and year of the seismic acquisition campaign. Additionally, the availability of each profile in SEG-Y format in the SIGEOF catalogue (IGME) or in SeisDARE database (DeFelipe et al., 2021) is also provided. Well information includes the names of the boreholes and the company that executed them, together with the targets of their execution (i.e. subsurface substances or structures).
3.3.4 Geographical data
Geographical data (median IFP of 31 %; Fig. 5) contain geographical information (in EPSG:4326 – WGS 84 coordinates) of the datasets described above (Fig. 4). Evaporite structures are positioned with respect to the central, minimum, and maximum latitude and longitude coordinates of their shape. Boreholes related to the indexed structures are listed as point data and referenced by their x and y coordinates, while seismic lines also include their minimum and maximum latitude and longitude values. An Esri® shapefile that includes the georeferenced shape of the inventoried evaporites is available at IESDB website for direct download and visualisation in a GIS-based environment.
3.3.5 Quantitative data
Quantitative data (median IFP of 99 %; Fig. 5) include a summary of the geometry and event chronology for each structure (Fig. 4). For the geometrical characterisation, outcropping or (when buried) horizontal intersection areas of the evaporite structures are included, in addition to their maximum thickness and maximum width and length values. The chronology of deformation episodes is only addressed for the deformed structures and comprises the maximum and minimum age of evaporite deformation (i.e. folding, thrusting, or diapirism), in addition to the number of deformation phases or diapiric growth stages.
3.3.6 Reference data
Reference data (median IFP of 76 %; Fig. 5) provides an extensive literature review of each evaporite structure indexed in the IESDB (Fig. 4). References consist of more than 1500 conference abstracts, scientific articles, books, book chapters, PhD theses and unpublished industry and government reports, all of them cited with the American Psychological Association (APA) format edition and linked to their online versions with hyperlinks (i.e. digital object identifiers or, when not available, generic URLs). Some sources address the evaporite structures regionally and multidisciplinarily, while others only focus on individual geological features. Thus, references are categorised by their main knowledge area, and some of them fit in two or more study fields. Altogether, 14 geoscience-related disciplines that include up to 38 references for each evaporite structure have been established to offer an overall literature assessment. Selected disciplines or categories, with the respective number of references that they include, are as follows: cross-sectional source (1), available well data (2), stratigraphy (6), regional stratigraphy (1), available seismic data (3), structure (6), analogue modelling (3), gravimetry and/or tomography (3), geochemistry (4), petrophysics and/or palaeomagnetism (2), MAGNA50 map series (IGME) sheet number (1), other maps (2), and other references (4).
3.3.7 Image data
Image data (median IFP of 100 % and therefore not included in Fig. 5) contain newly created and previously published figures of the inventoried evaporite structures (Fig. 4). Newly created figures include (1) a general location map of each structure, (2) a detailed map of their outcropping or (when buried) horizontal intersection shape, and (3) a cross section showing their subsurface structure. Additionally, a simplified sketch of the structure type, according to the Hudec and Jackson (2007) classification, is included for the deformed evaporites (when applicable). The already-published figures consist of (1) a stratigraphic correlation panel or column that represents the regional stratigraphy of evaporites and surrounding rocks (referenced in the “Regional stratigraphy” category of the reference data), (2) a portion of the IGME, LNEG, or ICGC geological map and (3) two geological maps from the literature (referenced in the “Other maps 1 and 2” categories of the reference data), with the first at basin scale (i.e. focused on regional geology of the evaporite structure) and the second at the most detailed scale available. The rights holder's copyright permissions have been checked for all the already-published figures reproduced on the IESDB website, and hence the availability, accuracy, and scale of the figures are restricted to those that are open access and that provide permission to be reproduced. This fact also prevented us from sharing the most up-to-date illustrated data on some of the structures, and therefore, in some cases, the reproduced figures show fewer details than the IESDB could (and would like to) offer.
3.3.8 Summarised index cards
Besides the seven datasets described above, a downloadable portable document format (*.pdf) format index card has been created to provide a comprehensive overview of each evaporite structure (Fig. 6). These summarised index cards are conceived as a summary of the stratigraphic and structural properties, subsurface data availability, and main references of each evaporite structure. They follow a common layout and include four figures from the image data (see Sect. 3.2.7), corresponding to the location, shape, geological map, and cross section of the evaporite structure they represent. The summarised index cards can be downloaded separately from the unique web section of each evaporite structure or jointly from the data repository as a complete dataset.
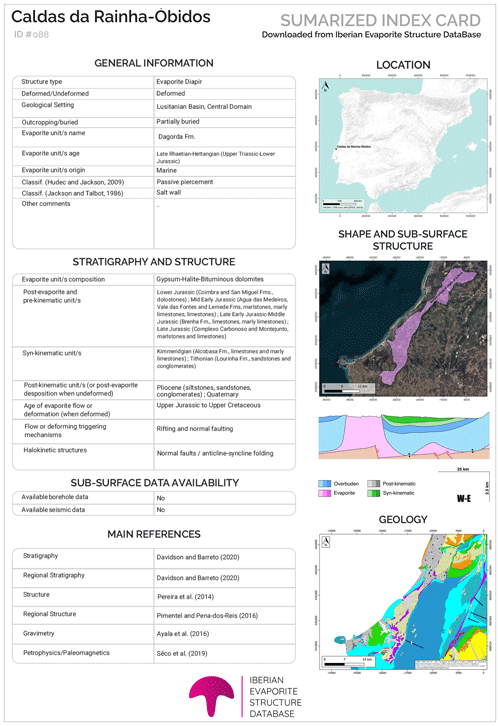
Figure 6Example of the index cards included in IESDB for each indexed evaporite structure. They include a summarised and illustrated overview of the general information, structure, stratigraphy, subsurface data availability, and the main literature references. Base maps are modified from Esri®; geological map is modified from Laboratório Nacional de Energia e Geologia (LNEG), Government of Portugal.
Evaporites have unique properties that make them stand out from other sedimentary rocks (e.g. Hudec and Jackson, 2007; Jackson and Hudec, 2017). Their ability to flow in the solid state under relatively low differential stress makes their study key for understanding the formation of sedimentary basins and orogens and characterising their role in controlling deformation processes at multiple scales (salt tectonics). Exceptionally well-exposed evaporites in Iberia allow us to study and interpret their halokinetic structures and growth strata (e.g. Rubinat et al., 2013, at the Bicorb–Quesa diapir in the eastern Prebetic zone and Roca et al., 2021, at the Bakio diapir in the Basque–Cantabrian basin), while an array of detailed (and often untapped) subsurface information constitutes an opportunity for understanding the extent and geometry of subsurface evaporite bodies and their relationship with the regional structure and stratigraphy (as demonstrated, for example, in the regional reviews of Martínez del Olmo et al., 2015, in the Prebetic Cordillera and of Carrillo et al., 2017, in the southeastern Pyrenees). The surface and subsurface stratigraphical, structural, and geophysical data for each evaporite structure are provided in the IESDB, so the website can be used as a search engine to find examples of, among others, the types and timing of salt mobilisation processes or the halokinetic sequences in the salt encasing rocks. The IESDB is thus a powerful tool for screening structures with geological features of interest, a resource for detecting missing information and setting research goals, and a geoscience teaching tool that allows us to look for examples to illustrate stratigraphic and tectonic processes in which evaporites are involved.
In addition, the properties of evaporites and their capacity to act as geological traps for hydrocarbons and other fluids have fostered their use in different geo-energy applications. Salt caverns are widely used as seasonal and long-lasting reservoirs for hydrocarbon products (e.g. oil crude and natural gas) and, in some cases, have been considered to be potential repositories for nuclear waste (e.g. Ozarslan, 2012; Wang et al., 2017; Warren, 2017). Furthermore, they have been proposed as suitable sites for renewable energy storage (e.g. in the form of green hydrogen and compressed air energy storage). Thus, understanding the deformation kinematics, flow history, and actual geometry of evaporite structures is crucial not only for the exploration and production of hydrocarbons but also for the future development of cleaner energy storage activities that are required to secure the energy transition to zero carbon. Onshore buried evaporite structures from the southern Pyrenees, Basque–Cantabrian basin, Prebetic Cordillera, and Lusitanian basin (all of them indexed in the IESDB; Fig. 2) have already been proposed as suitable candidate sites for underground energy storage (Carneiro et al., 2019; Caglayan et al., 2020; Martínez del Olmo, 2021). It is worth noting that Spain ranks third in the European national storage potential classification, after Germany and Poland (Caglayan et al., 2020), and evaporite structures are fundamental for this.
Other studies also appraised the suitability of some of the evaporite structures indexed in the IESDB to be used as seals for CO2 geological storage facilities (Sun et al., 2021), particularly in the Lusitanian basin (Pereira et al., 2014, 2021), the Betic System (Urquiza, 2012), and the Basque–Cantabrian basin (Llamas et al., 2017; Fig. 2). Finally, evaporite structures have also been postulated as being suitable hosts for low- to high-level nuclear waste repositories, initially by the U.S. Committee of Waste Disposal (Heroy, 1957) and more recently by other organisations (e.g. International Atomic Energy Agency, 2003). The site election for development of this facilities must be, however, based on multiple criteria to ensure their safety and durability (e.g. Warren, 2017). For example, numerous projects led by ENRESA (Empresa Nacional de Residuos Radiactivos, S. A.), a public and non-profit organisation responsible for the management of radioactive waste in Spain, were funded between 1986 and 1997 to study evaporite formations as potential hosts for deep nuclear waste storage. Given the wide range of applications that they offer, knowledge on the geologic features of evaporite structures will thus be crucial in the near future for energy storage, climate change mitigation, and waste management, and the overall assessment provided by the IESDB represents an initiative that could be replicated in other evaporite-rich world regions (Fig. 1).
The IESDB database is freely available at https://iesdb.eu (last access: 15 June 2023) Datasets and summarised index cards can be downloaded from the DIGITAL.CSIC data repository at https://doi.org/10.20350/digitalCSIC/14586 (González-Esvertit et al., 2022).
Evaporite rocks have attracted great scientific and industrial interest during the last century. Earth scientists and hydrocarbon and mining companies have studied evaporite structures worldwide aiming to understand, among others, their origin, significance, and influence on basin and orogen architecture. Surface and subsurface geoscientific information derived from these aims remain useful nowadays but are often untapped because of access issues, segregation, and scarce data dissemination. The IESDB includes a painstaking compilation of 150 evaporite structures and their surrounding rocks from Iberia and is sourced from an extensive database and literature review of scientific articles, published maps, and reports. This database represents a comprehensive multidisciplinary review of Iberian evaporites, in addition to the first overall assessment of evaporite structures carried out in any region of the world. The IESDB is presented as an open access, user-friendly, and an open-to-feedback web page, where the inventoried structures can be selected from a map or sorted by multi-criteria filtering, and the workflow used to build it could be replicated in other world regions for the indexation of evaporite or other types of geologic structures. The database stores systematically organised information about the structure, stratigraphy, event chronology, seismic and well data availability, and mining activity (historical and active) of 150 evaporite structures. The compilation and assessment provided by the IESDB will serve as a starting point for improving the regional and local geological understanding of the inventoried structures and can also act as an evaporite radar for the identification of suitable sites for energy, carbon, or nuclear waste storage facilities.
JA and EGR conceptualised the original idea, indexing criteria and data compilation methods. EGE collected, filtered, and organised the data and images, with contributions from all co-authors. JA managed the data upload to the DIGITAL.CSIC data repository and the DOI assignation. EGR provided the project funding and managed the website hosting on the Universitat de Barcelona servers. EGE, JA, and EGR designed the web page and carried out the data uploading. EGE drafted the paper, with contributions from all co-authors.
The contact author has declared that none of the authors has any competing interests.
Publisher’s note: Copernicus Publications remains neutral with regard to jurisdictional claims in published maps and institutional affiliations.
In loving memory of our friend and colleague Victoriano (“Vito”) Pineda, who passed away in the Súria potash mine on 9 March 2023, at the age of 29. We gratefully acknowledge Dan Micrea Tamas, Ricardo Pereira and an anonymous reviewer for their insightful and constructive comments and suggestions, and Kirsten Elger for the editorial guidance. We are thankful to Geosciences Barcelona (GEO3BCN-CSIC) for providing space at the Digital.CSIC data repository. The geological surveys of Spain (IGME), Portugal (LNEG), and Catalonia (ICGC) are acknowledged for providing access to borehole, seismic, and mining data and the geological maps of evaporite structures and surroundings.
This research has been funded by the Ramón y Cajal fellowship RyC-2018-026335-I to Enrique Gomez-Rivas (MCIN/AEI/10.13039/501100011033 and the European Social Fund – Investing in Your Future) and the DGICYT Spanish Project PID2020-118999GB-I00 (MCIN/AEI/10.13039/501100011033). This work is a contribution to the 2021 SGR 00349 Geologia Sedimentària consolidated research group (Agència de Gestió d’Ajuts Universitaris i de Recerca). Juan Alcalde has been funded by MCIN/AEI/10.13039/501100011033 and the European Union NextGenerationEU/PRTR for the Ramón y Cajal fellowship (grant no. RYC2021-033872-I).
This paper was edited by Kirsten Elger and reviewed by Dan Mircea Tamas and one anonymous referee.
Andeweg, B.: Cenozoic tectonic evolution of the Iberian Peninsula: effects and causes of changing stress fields, unpublished thesis, Vrije Universiteit, Amsterdam, 178 pp., 2002.
Burrel, L. and Teixell, A.: Contractional salt tectonics and role of pre-existing diapiric structures in the South Pyrenean foreland fold-and-thrust belt (Montsec and Serres Marginals), Journal of the Geological Society, 178, jgs2020-085, https://doi.org/10.1144/jgs2020-085, 2021.
Caglayan, D. G., Weber, N., Heinrichs, H. U., Linßen, J., Robinius, M., Kukla, P. A., and Stolten, D.: Technical potential of salt caverns for hydrogen storage in Europe, Int. J. Hydrogen Energ., 45, 6793–6805, https://doi.org/10.1016/j.ijhydene.2019.12.161, 2020.
Cámara, P.: Salt and Strike-Slip Tectonics as Main Drivers in the Structural Evolution of the Basque-Cantabrian Basin, Spain, in: Permo-Triassic Salt Provinces of Europe, North Africa and the Atlantic Margins, Elsevier, 371–393, https://doi.org/10.1016/B978-0-12-809417-4.00018-5, 2017.
Cámara, P. and Flinch, J. F.: The Southern Pyrenees, in: Permo-Triassic Salt Provinces of Europe, North Africa and the Atlantic Margins, Elsevier, 395–415, https://doi.org/10.1016/B978-0-12-809417-4.00019-7, 2017.
Carneiro, J. F., Matos, C. R., and van Gessel, S.: Opportunities for large-scale energy storage in geological formations in mainland Portugal, Renew. Sust. Energ. Rev., 99, 201–211, https://doi.org/10.1016/j.rser.2018.09.036, 2019.
Carrillo, E., Koyi, H. A., and Nilfouroushan, F.: Structural significance of an evaporite formation with lateral stratigraphic heterogeneities (Southeastern Pyrenean Basin, NE Spain), Mar. Petrol. Geol., 86, 1310–1326, https://doi.org/10.1016/j.marpetgeo.2017.07.024, 2017.
Casas-Sainz, A. M. and de Vicente, G.: On the tectonic origin of Iberian topography, Tectonophysics, 474, 214–235, https://doi.org/10.1016/j.tecto.2009.01.030, 2009.
Dalton, C. E. G.: Stratigraphic and Structural Characterization and Evolution of Exposed Megaflaps Flanking Salt Diapirs, PhD thesis, The University of Texas, El Paso, 273 pp., https://scholarworks.utep.edu/open_etd/71/ (last access: 15 June 2023), 2019.
DeFelipe, I., Alcalde, J., Ivandic, M., Martí, D., Ruiz, M., Marzán, I., Diaz, J., Ayarza, P., Palomeras, I., Fernandez-Turiel, J.-L., Molina, C., Bernal, I., Brown, L., Roberts, R., and Carbonell, R.: Reassessing the lithosphere: SeisDARE, an open-access seismic data repository, Earth Syst. Sci. Data, 13, 1053–1071, https://doi.org/10.5194/essd-13-1053-2021, 2021.
Duffy, O., Hudec, M., Peel, F., Apps, G., Bump, A., Moscardelli, L., Dooley, T., Bhattacharya, S., Wisian, K., and Shuster, M.: The Role of Salt Tectonics in the Energy Transition: An Overview and Future Challenges, Tektonika, 1, 18–48, https://doi.org/10.55575/tektonika2023.1.1.11, 2023.
Escavy, J. I., Herrero, M. J., and Arribas, M. E.: Gypsum resources of Spain: Temporal and spatial distribution, Ore Geol. Rev., 13, 72–84, https://doi.org/10.1016/j.oregeorev.2012.09.001, 2012.
Escosa, F. O., Ferrer, O., and Roca, E.: Geology of the Eastern Prebetic Zone at the Jumilla region (SE Iberia), J. Maps, 14, 77–86, https://doi.org/10.1080/17445647.2018.1433562, 2018.
Galdeano, C. S. and Vera, J. A.: Stratigraphic record and palaeogeographical context of the Neogene basins in the Betic Cordillera, Spain, Basin Res., 4, 21–36, https://doi.org/10.1111/j.1365-2117.1992.tb00040.x, 1992.
Galindo-Zaldívar, J., Braga, J. C., Marín-Lechado, C., Ercilla, G., Martín, J. M., Pedrera, A., Casas, D., Aguirre, J., Ruiz-Constán, A., Estrada, F., Puga-Bernabéu, Á., Sanz de Galdeano, C., Juan, C., García-Alix, A., Vázquez, J. T., and Alonso, B.: Extension in the Western Mediterranean, in: The Geology of Iberia: A Geodynamic Approach, edited by: Quesada, C. and Oliveira, J. T., Springer International Publishing, Cham, 61–103, https://doi.org/10.1007/978-3-030-11190-8_3, 2019.
García-Hernández, M., López-Garrido, A. C., Rivas, P., Sanz de Galdeano, C., and Vera, J. A.: Mesozoic palaeogeographic evolution of the External Zones of the Betic Cordillera, Geol. Mijnbouw, 59, 155–168, 1980.
García-Tortosa, F. J., Alfaro, P., Galindo-Zaldívar, J., Gibert, L., López-Garrido, A. C., Sanz de Galdeano, C., and Ureña, M.: Geomorphologic evidence of the active Baza Fault (Betic Cordillera, South Spain), Geomorphology, 97, 374–391, https://doi.org/10.1016/j.geomorph.2007.08.007, 2008.
García-Veigas, J., Gibert, L., Cendón, D. I., Artiaga, D., Corbí, H., Soria, J. M., Lowenstein, T. K., and Sanz, E.: Late Miocene evaporite geochemistry of Lorca and Fortuna basins (Eastern Betics, SE Spain): Evidence of restriction and continentalization, Basin Res., 32, 916–948, https://doi.org/10.1111/bre.12408, 2020.
Gibert, L., Ortí, F., and Rosell, L.: Plio-Pleistocene lacustrine evaporites of the Baza Basin (Betic Chain, SE Spain), Sediment. Geol., 28, 89–116, https://doi.org/10.1016/j.sedgeo.2007.03.003, 2007.
Gómez, J. J., Sandoval, J., Aguado, R., O'Dogherty, L., and Osete, M. L.: The Alpine Cycle in Eastern Iberia: Microplate Units and Geodynamic Stages, in: The Geology of Iberia: A Geodynamic Approach, edited by: Quesada, C. and Oliveira, J. T., Springer International Publishing, Cham, 15–27, https://doi.org/10.1007/978-3-030-11295-0_2, 2019.
González-Esvertit, E., Alcalde, J., and Gomez-Rivas, E.: IESDB – The Iberian Evaporite Structures DataBase. An interactive atlas of evaporite structures in Iberia, Digital.CSIC [data set], https://doi.org/10.20350/digitalCSIC/14586, 2022.
Heroy, W. B.: Disposal of radioactive waste in salt cavities, in: The Disposal of Radioactive Waste on Land. Report prepared for the Report prepared for the Committee on Waste Disposal in Geologic Structures. Division on Earth and Life Studies, The National Academies Press, Washington, DC, https://doi.org/10.17226/10294, 1957.
Hudec, M. R. and Jackson, M. P. A.: Terra infirma: Understanding salt tectonics, Earth-Sci. Rev., 82, 1–28, https://doi.org/10.1016/j.earscirev.2007.01.001, 2007.
Hudec, M. R. and Jackson, M. P. A.: De Re Salica: Fundamental principles of salt tectonics, in: Regional Geology and Tectonics: Phanerozoic Passive Margins, Cratonic Basins and Global Tectonic Maps, Elsevier, 18–41, https://doi.org/10.1016/B978-0-444-56357-6.00001-9, 2012.
International Atomic Energy Agency (Ed.): Scientific and technical basis for the geological disposal of radioactive waste, International Atomic Energy Agency, Vienna, 80 pp., ISBN 92-0-100103-7, 2003.
Jackson, M. P. A. and Hudec, M. R.: Salt Tectonics: Principles and Practice, Cambridge University Press, Cambridge, https://doi.org/10.1017/9781139003988, 2017.
Jackson, M. P. A. and Talbot, C. J.: A Glossary of Salt Tectonics, The University of Texas at Austin, Bureau of Economic Geology, Geological Circular, 44 pp., https://doi.org/10.23867/GC9104D, 1991.
Jurado, M. J.: El Triásico y el Liásico basal evaporíticos del subsuelo de la Cuenca del Ebro, in: Formaciones Evaporíticas de la Cuenca del Ebro y Cadenas Periféricas, y de la Zona de Levante, edited by: Ortí, F. and Salvany, J. M., Universitat de Barcelona, ISBN mkt0006759737, 1990.
Llamas, B., Cruz Castañeda, M., Laín, C., and Pous, J.: Study of the Basque–Cantabrian basin as a suitable region for the implementation of an energy storage system based on compressed air energy storage (CAES), Environ. Earth Sci., 76, 204, https://doi.org/10.1007/s12665-017-6515-y, 2017.
López-Gómez, J., Mas, R., and Arche, A.: The evolution of the Middle Triassic (Muschelkalk) carbonate ramp in the SE Iberian Ranges, eastern Spain: sequence stratigraphy, dolomitization processes and dynamic controls, Sediment. Geol., 87, 165–193, https://doi.org/10.1016/0037-0738(93)90003-N, 1993.
López-Gómez, J., Alonso-Azcárate, J., Arche, A., Arribas, J., Fernández Barrenechea, J., Borruel-Abadía, V., Bourquin, S., Cadenas, P., Cuevas, J., De la Horra, R., Díez, J. B., Escudero-Mozo, M. J., Fernández-Viejo, G., Galán-Abellán, B., Galé, C., Gaspar-Escribano, J., Gisbert Aguilar, J., Gómez-Gras, D., Goy, A., Gretter, N., Heredia Carballo, N., Lago, M., Lloret, J., Luque, J., Márquez, L., Márquez-Aliaga, A., Martín-Algarra, A., Martín-Chivelet, J., Martín-González, F., Marzo, M., Mercedes-Martín, R., Ortí, F., Pérez-López, A., Pérez-Valera, F., Pérez-Valera, J. A., Plasencia, P., Ramos, E., Rodríguez-Méndez, L., Ronchi, A., Salas, R., Sánchez-Fernández, D., Sánchez-Moya, Y., Sopeña, A., Suárez-Rodríguez, Á., Tubía, J. M., Ubide, T., Valero Garcés, B., Vargas, H., and Viseras, C.: Permian-Triassic Rifting Stage, in: The Geology of Iberia: A Geodynamic Approach, edited by: Quesada, C. and Oliveira, J. T., Springer International Publishing, Cham, 29–112, https://doi.org/10.1007/978-3-030-11295-0_3, 2019.
Lux, K.-H.: Design of salt caverns for the storage of natural gas, crude oil and compressed air: Geomechanical aspects of construction, operation and abandonment, Geological Society, London, Special Publications, 313, 93–128, https://doi.org/10.1144/SP313.7, 2009.
Macchiavelli, C., Vergés, J., Schettino, A., Fernàndez, M., Turco, E., Casciello, E., Torne, M., Pierantoni, P. P., and Tunini, L.: A New Southern North Atlantic Isochron Map: Insights Into the Drift of the Iberian Plate Since the Late Cretaceous, J. Geophys. Res., 122, 9603–9626, https://doi.org/10.1002/2017JB014769, 2017.
Martínez del Olmo, W.: Almacenamiento de Hidrógeno en España, Revista de la Sociedad Geológica de España, 34, 53–59, 2021.
Martínez del Olmo, W., Motis, K., and Martín, D.: El papel del diapirismo de la sal Triásica en la estructuración del Prebético (SE de España), Revista de la Sociedad Geológica de España, 28, 3–24, 2015.
Marzo, M.: El Buntsandstein de los Catalánides, Universitat de Barcelona, unpublished PhD thesis, 317 pp., http://hdl.handle.net/10803/1579 (last access: 20 June 2023), 1980.
McClay, K., Muñoz, J.-A., and García-Senz, J.: Extensional salt tectonics in a contractional orogen: A newly identified tectonic event in the Spanish Pyrenees, Geology, 32, 737–740, https://doi.org/10.1130/G20565.1, 2004.
Muñoz, J. A., Mencos, J., Roca, E., Carrera, N., Gratacós, O., Ferrer, O., and Fernández, O.: The structure of the South-Central-Pyrenean fold and thrust belt as constrained by subsurface data, Geol. Acta, 22, 439–460, https://doi.org/10.1344/GeologicaActa2018.16.4.7, 2018.
Ortí, F.: Aspectos sedimentológicos de las evaporitas del Triásico y del Liásico inferior en el E de la Península Ibérica, Cuadernos de Geología Ibérica, 11, 837–858, 1987.
Ortí, F., Pérez-López, A., and Salvany, J. M.: Triassic evaporites of Iberia: Sedimentological and palaeogeographical implications for the western Neotethys evolution during the Middle Triassic-Earliest Jurassic, Palaeogeogr. Palaeocl., 471, 157–180, https://doi.org/10.1016/j.palaeo.2017.01.025, 2017.
Ozarslan, A.: Large-scale hydrogen energy storage in salt caverns, Int. J. Hydrogen Energ., 37, 14265–14277, https://doi.org/10.1016/j.ijhydene.2012.07.111, 2012.
Peper, T. and Cloetingh, S.: Lithosphere dynamics and tectono-stratigraphic evolution of the Mesozoic Betic rifted margin (southeastern Spain), Tectonophysics, 203, 345–361, https://doi.org/10.1016/0040-1951(92)90231-T, 1992.
Pereira, N., Carneiro, J. F., Araújo, A., Bezzeghoud, M., and Borges, J.: Seismic and structural geology constraints to the selection of CO2 storage sites – The case of the onshore Lusitanian basin, Portugal, J. Appl. Geophys., 102, 21–38, https://doi.org/10.1016/j.jappgeo.2013.12.001, 2014.
Pereira, P., Ribeiro, C., and Carneiro, J.: Identification and characterisation of geological formations with CO2 storage potential in Portugal, Petrol. Geosci., 27, petgeo2020-123, https://doi.org/10.1144/petgeo2020-123, 2021.
Playà, E., Ortıì, F., and Rosell, L.: Marine to non-marine sedimentation in the upper Miocene evaporites of the Eastern Betics, SE Spain: sedimentological and geochemical evidence, Sediment. Geol., 133, 135–166, https://doi.org/10.1016/S0037-0738(00)00033-6, 2000.
Puigdefàbregas, C. and Souquet, P.: Tectono-sedimentary cycles and depositional sequences of the Mesozoic and Tertiary from the Pyrenees, Tectonophysics, 129, 173–203, 1986.
Quijada, I. E., Suarez-Gonzalez, P., Benito, M. I., and Mas, R.: Depositional Depth of Laminated Carbonate Deposits: Insights From the Lower Cretaceous Valdeprado Formation (Cameros Basin, Northern Spain), J. Sediment. Res., 83, 241–257, https://doi.org/10.2110/jsr.2013.23, 2013.
Ramos, A., Fernández, O., Terrinha, P., and Muñoz, J. A.: Extension and inversion structures in the Tethys–Atlantic linkage zone, Algarve Basin, Portugal, Int. J. Earth Sci., 105, 1663–1679, https://doi.org/10.1007/s00531-015-1280-1, 2016.
Ramos, A., Fernández, O., Muñoz, J. A., and Terrinha, P.: Impact of basin structure and evaporite distribution on salt tectonics in the Algarve Basin, Southwest Iberian margin, Mar. Petrol. Geol., 88, 961–984, https://doi.org/10.1016/j.marpetgeo.2017.09.028, 2017.
Ramos, A., Fernández, O., Terrinha, P., Muñoz, J. A., and Arnaiz, Á.: Paleogeographic evolution of a segmented oblique passive margin: the case of the SW Iberian margin, Int. J. Earth Sci., 109, 1871–1895, https://doi.org/10.1007/s00531-020-01878-w, 2020.
Rasmussen, E. S., Lomholt, S., Andersen, C., and Vejbæk, O. V.: Aspects of the structural evolution of the Lusitanian Basin in Portugal and the shelf and slope area offshore Portugal, Tectonophysics, 300, 199–225, https://doi.org/10.1016/S0040-1951(98)00241-8, 1998.
Roca, E., Ferrer, O., Rowan, M. G., Muñoz, J. A., Butillé, M., Giles, K. A., Arbués, P., and de Matteis, M.: Salt tectonics and controls on halokinetic-sequence development of an exposed deepwater diapir: The Bakio Diapir, Basque-Cantabrian Basin, Pyrenees, Mar. Petrol. Geol., 123, 104770, https://doi.org/10.1016/j.marpetgeo.2020.104770, 2021.
Rosenbaum, G., Lister, G. S., and Duboz, C.: Relative motions of Africa, Iberia and Europe during Alpine orogeny, Tectonophysics, 359, 117–129, https://doi.org/10.1016/S0040-1951(02)00442-0, 2002.
Rubinat, M., Roca, E., Escalas, M., Queralt, P., Ferrer, O., and Ledo, J. J.: The influence of basement structure on the evolution of the Bicorb-Quesa Diapir (eastern Betics, Iberian Peninsula): contractive thin-skinned deformation above a pre-existing extensional basement fault, Int. J. Earth Sci., 102, 25–41, https://doi.org/10.1007/s00531-012-0789-9, 2013.
Salas, R. and Casas, A.: Mesozoic extensional tectonics, stratigraphy and crustal evolution during the Alpine cycle of the eastern Iberian basin, Tectonophysics, 228, 33–55, https://doi.org/10.1016/0040-1951(93)90213-4, 1993.
Saucier, H.: Geoscientists Will Be the Backbone of the Energy Transition, AAPG Explorer, https://explorer.aapg.org/story/articleid/61004/ (last access: 15 March 2022), 2021.
Saura, E., Ardèvol i Oró, L., Teixell, A., and Vergés, J.: Rising and falling diapirs, shifting depocenters, and flap overturning in the Cretaceous Sopeira and Sant Gervàs subbasins (Ribagorça Basin, southern Pyrenees): Southern Pyrenees Cretaceous Diapirism, Tectonics, 35, 638–662, https://doi.org/10.1002/2015TC004001, 2016.
Segura, M., Polo, T., García-Hidalgo, J. F., Gil, J., Carenas, B., and García, Á.: The Upper Cretaceous in the Tagus Basin (Central Spain): sequential analysis based on oil-well data and outcrop correlation, Geological Society, London, Special Publications, 262, 231–244, https://doi.org/10.1144/GSL.SP.2006.262.01.14, 2006.
Simancas, J. F.: Variscan Cycle, in: The Geology of Iberia: A Geodynamic Approach, edited by: Quesada, C. and Oliveira, J. T., Springer International Publishing, Cham, 1–25, https://doi.org/10.1007/978-3-030-10519-8_1, 2019.
Soto, R., Villalaín, J. J., and Casas-Sainz, A. M.: Remagnetizations as a tool to analyze the tectonic history of inverted sedimentary basins: A case study from the Basque-Cantabrian basin (north Spain), Tectonics, 27, TC1017, https://doi.org/10.1029/2007TC002208, 2008.
Soto, R., Beamud, E., Roca, E., Carola, E., and Almar, Y.: Distinguishing the effect of diapir growth on magnetic fabrics of syn-diapiric overburden rocks: Basque–Cantabrian basin, Northern Spain, Terra Nova, 29, 191–201, https://doi.org/10.1111/ter.12262, 2017.
Sun, X., Alcalde, J., Bakhtbidar, M., Elío, J., Vilarrasa, V., Canal, J., Ballesteros, J., Heinemann, N., Haszeldine, S., Cavanagh, A., Vega-Maza, D., Rubiera, F., Martínez-Orio, R., Johnson, G., Carbonell, R., Marzan, I., Travé, A., and Gomez-Rivas, E.: Hubs and clusters approach to unlock the development of carbon capture and storage – Case study in Spain, Appl. Energ., 300, 117418, https://doi.org/10.1016/j.apenergy.2021.117418, 2021.
Tarkowski, R. and Czapowski, G.: Salt domes in Poland – Potential sites for hydrogen storage in caverns, Int. J. Hydrogen Energ., 43, 21414–21427, https://doi.org/10.1016/j.ijhydene.2018.09.212, 2018.
Urquiza, M. R.: Estudio de las formaciones favorables para almacenamiento de CO2 en un sector del borde meridional de las Béticas orientales, PhD thesis, Universidad de Oviedo, 262 pp., http://hdl.handle.net/10651/13206 (last access: 20 June 2023), 2012.
U.S. Geological Survey: Mineral commodity summaries 2020, U.S. Geological Survey, 200 pp., https://doi.org/10.3133/mcs2020, 2020.
Vergés, J. and Fernàndez, M.: Ranges and basins in the Iberian Peninsula: their contribution to the present topography, Geological Society, London, Memoirs, 32, 223–234, https://doi.org/10.1144/GSL.MEM.2006.032.01.13, 2006.
Vergés, J., Kullberg, J. C., Casas-Sainz, A., de Vicente, G., Duarte, L. V., Fernàndez, M., Gómez, J. J., Gómez-Pugnaire, M. T., Jabaloy Sánchez, A., López-Gómez, J., Macchiavelli, C., Martín-Algarra, A., Martín-Chivelet, J., Muñoz, J. A., Quesada, C., Terrinha, P., Torné, M., and Vegas, R.: An Introduction to the Alpine Cycle in Iberia, in: The Geology of Iberia: A Geodynamic Approach, edited by: Quesada, C. and Oliveira, J. T., Springer International Publishing, Cham, 1–14, https://doi.org/10.1007/978-3-030-11295-0_1, 2019.
Vergés, J., Poprawski, Y., Almar, Y., Drzewiecki, P. A., Moragas, M., Bover-Arnal, T., Macchiavelli, C., Wright, W., Messager, G., Embry, J., and Hunt, D.: Tectono-sedimentary evolution of Jurassic–Cretaceous diapiric structures: Miravete anticline, Maestrat Basin, Spain, Basin Res., 32, 1653–1684, https://doi.org/10.1111/bre.12447, 2020.
Virgili, C., Sopeña, A., and Ramos, A.: Problemas de la cronoestratigrafía del Trías en España, Cuadernos de Geología Ibérica, 4, 57–88, 1977.
Wang, J., Lu, K., Ma, L., Wang, J., Dooner, M., Miao, S., Li, J., and Wang, D.: Overview of Compressed Air Energy Storage and Technology Development, Energies, 10, 991, https://doi.org/10.3390/en10070991, 2017.
Warren, J. K.: Evaporites: Sediments, Resources and Hydrocarbons, Springer Berlin, 1035 pp., ISBN 978-3-540-32344-0, https://doi.org/10.1007/3-540-32344-9, 2006.
Warren, J. K.: Evaporites through time: Tectonic, climatic and eustatic controls in marine and nonmarine deposits, Earth-Sci. Rev., 98, 217–268, https://doi.org/10.1016/j.earscirev.2009.11.004, 2010.
Warren, J. K.: Interpreting Evaporite Textures, in: Evaporites, Springer International Publishing, Cham, 1–83, https://doi.org/10.1007/978-3-319-13512-0_1, 2016.
Warren, J. K.: Salt usually seals, but sometimes leaks: Implications for mine and cavern stabilities in the short and long term, Earth-Sci. Rev., 165, 302–341, https://doi.org/10.1016/j.earscirev.2016.11.008, 2017.
Weijermars, R., Jackson, M. P. A., and Vendeville, B.: Rheological and tectonic modeling of salt provinces, Tectonophysics, 217, 143–174, https://doi.org/10.1016/0040-1951(93)90208-2, 1993.
Wilkinson, M. D., Dumontier, M., Aalbersberg, Ij. J., Appleton, G., Axton, M., Baak, A., Blomberg, N., Boiten, J.-W., da Silva Santos, L. B., Bourne, P. E., Bouwman, J., Brookes, A. J., Clark, T., Crosas, M., Dillo, I., Dumon, O., Edmunds, S., Evelo, C. T., Finkers, R., Gonzalez-Beltran, A., Gray, A. J. G., Groth, P., Goble, C., Grethe, J. S., Heringa, J., 't Hoen, P. A. C., Hooft, R., Kuhn, T., Kok, R., Kok, J., Lusher, S. J., Martone, M. E., Mons, A., Packer, A. L., Persson, B., Rocca-Serra, P., Roos, M., van Schaik, R., Sansone, S.-A., Schultes, E., Sengstag, T., Slater, T., Strawn, G., Swertz, M. A., Thompson, M., van der Lei, J., van Mulligen, E., Velterop, J., Waagmeester, A., Wittenburg, P., Wolstencroft, K., Zhao, J., and Mons, B.: The FAIR Guiding Principles for scientific data management and stewardship, Sci. Data, 3, 160018, https://doi.org/10.1038/sdata.2016.18, 2016.
Wilson, R. C. L., Hiscott, R. N., Willis, M. G., and Gradstein, F. M.: The Lusitanian Basin of West-Central Portugal: Mesozoic and Tertiary Tectonic, Stratigraphic, and Subsidence History, in: Extensional Tectonics and Stratigraphy of the North Atlantic Margins, ISBN 9781629811291, https://doi.org/10.1306/M46497C22, 1989.
- Abstract
- Introduction
- Geological setting
- The Iberian Evaporite Structure Database
- The IESDB applications on geology, geo-resources, and geo-energy
- Data availability
- Conclusions and final remarks
- Author contributions
- Competing interests
- Disclaimer
- Acknowledgements
- Financial support
- Review statement
- References
- Abstract
- Introduction
- Geological setting
- The Iberian Evaporite Structure Database
- The IESDB applications on geology, geo-resources, and geo-energy
- Data availability
- Conclusions and final remarks
- Author contributions
- Competing interests
- Disclaimer
- Acknowledgements
- Financial support
- Review statement
- References