the Creative Commons Attribution 4.0 License.
the Creative Commons Attribution 4.0 License.
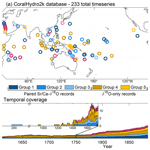
The CoralHydro2k database: a global, actively curated compilation of coral δ18O and Sr ∕ Ca proxy records of tropical ocean hydrology and temperature for the Common Era
Rachel M. Walter
Thomas Felis
Kim M. Cobb
Nerilie J. Abram
Ariella K. Arzey
Alyssa R. Atwood
Logan D. Brenner
Émilie P. Dassié
Kristine L. DeLong
Bethany Ellis
Julien Emile-Geay
Matthew J. Fischer
Nathalie F. Goodkin
Jessica A. Hargreaves
K. Halimeda Kilbourne
Hedwig Krawczyk
Nicholas P. McKay
Andrea L. Moore
Sujata A. Murty
Maria Rosabelle Ong
Riovie D. Ramos
Emma V. Reed
Dhrubajyoti Samanta
Sara C. Sanchez
Jens Zinke
The response of the hydrological cycle to anthropogenic climate change, especially across the tropical oceans, remains poorly understood due to the scarcity of long instrumental temperature and hydrological records. Massive shallow-water corals are ideally suited to reconstructing past oceanic variability as they are widely distributed across the tropics, rapidly deposit calcium carbonate skeletons that continuously record ambient environmental conditions, and can be sampled at monthly to annual resolution. Climate reconstructions based on corals primarily use the stable oxygen isotope composition (δ18O), which acts as a proxy for sea surface temperature (SST), and the oxygen isotope composition of seawater (δ18Osw), a measure of hydrological variability. Increasingly, coral δ18O time series are paired with time series of strontium-to-calcium ratios (), a proxy for SST, from the same coral to quantify temperature and δ18Osw variability through time. To increase the utility of such reconstructions, we present the CoralHydro2k database, a compilation of published, peer-reviewed coral and δ18O records from the Common Era (CE). The database contains 54 paired –δ18O records and 125 unpaired or δ18O records, with 88 % of these records providing data coverage from 1800 CE to the present. A quality-controlled set of metadata with standardized vocabulary and units accompanies each record, informing the use of the database. The CoralHydro2k database tracks large-scale temperature and hydrological variability. As such, it is well-suited for investigations of past climate variability, comparisons with climate model simulations including isotope-enabled models, and application in paleodata-assimilation projects. The CoralHydro2k database is available in Linked Paleo Data (LiPD) format with serializations in MATLAB, R, and Python and can be downloaded from the NOAA National Center for Environmental Information's Paleoclimate Data Archive at https://doi.org/10.25921/yp94-v135 (Walter et al., 2022).
- Article
(5733 KB) - Full-text XML
- BibTeX
- EndNote
The global hydrological cycle is changing in response to ongoing anthropogenic climate change (Held and Soden, 2006; Cheng et al., 2020), yet regional trends in hydrology remain uncertain in many areas of the world (Song et al., 2021; Madakumbura et al., 2021; Ummenhofer et al., 2021). Observed and projected trends in large-scale hydrology are consistent with the “wet get wetter, dry get drier” paradigm (Held and Soden, 2006) as surface ocean fluxes increase as the planet warms. Rising global temperatures mean that the atmosphere can hold more moisture, which contributes to more extreme rainfall across a variety of spatiotemporal scales. In the tropics, many aspects of large-scale hydrology are tied to changes in large-scale coupled ocean–atmosphere dynamics associated with the El Niño–Southern Oscillation (ENSO; Power et al., 2013; Cai et al., 2014), tropical Pacific decadal variability (Gu and Adler, 2013; Dong and Dai, 2015), the Indian Ocean Dipole (Webster et al., 1999; Saji et al., 1999; Cai et al., 2019), and Atlantic Multidecadal Variability (Zhang et al., 2019), to name a few of the most prominent modes.
The detection of potential anthropogenic trends in regional hydrology against a rich background of natural regional hydrological variability is complicated by a dearth of instrumental climate data from across the tropics. In particular, instrumental sea surface temperature (SST) observations are sparse prior to the advent of satellites in 1979 (Reynolds et al., 2002; Rayner et al., 2003; Freeman et al., 2017; Huang et al., 2017; Kennedy et al., 2019), and the vast majority of sea surface salinity (SSS) observations only became available in the 1990s with the advent of the Global Tropical Moored Buoy array (McPhaden et al., 1998, 2010) and the World Ocean Circulation Experiment (WOCE) (Good et al., 2013; Friedman et al., 2017; Cheng et al., 2020; Gould and Cunningham, 2021). Both natural and anthropogenic shifts in regional hydroclimate on interannual to multidecadal timescales have profound impacts on societies, economies, and ecosystems, such that resolving regional trends in past hydrological variability prior to available observational records is a scientific and societal priority.
Shallow-water corals have been extensively used to reconstruct past regional- to oceanic-scale climate variability at data-scarce locations in the tropical and subtropical oceans (as reviewed by Gagan et al., 2000; Corrège, 2006; Lough, 2010; Felis, 2020). Seasonally banded coral skeletons (e.g., Lough and Barnes, 1997) can yield monthly to annually resolved proxy records that can be calibrated to instrumental climate observations and thus used to extend the relatively short instrumental SST and SSS records back to the pre-instrumental era. Most coral-based reconstructions are based on the oxygen isotopic composition (δ18O) and/or strontium-to-calcium ratios () of coral skeletal aragonite. Coral δ18O tracks changes in SST and the oxygen isotopic composition of seawater (δ18Osw) (Epstein et al., 1953; Weber and Woodhead, 1972). Like salinity, variability in δ18Osw reflects the balance of precipitation and evaporation, terrestrial runoff, continental ice melt and formation, and ocean circulation and mixing (e.g., LeGrande and Schmidt, 2006, 2011; Hasson et al., 2013; Conroy et al., 2014). Coral primarily tracks SST variability (Weber, 1973; Smith et al., 1979; Beck et al., 1992) and can be used to decouple the temperature and δ18Osw signals in coral δ18O records (e.g., Gagan et al., 1998; Ren et al., 2003; Corrège, 2006; Cahyarini et al., 2008). As such, paired coral δ18O and records can be used to independently investigate trends in SST and hydrology (Hendy et al., 2002; Linsley et al., 2006; Quinn et al., 2006; Zinke et al., 2008; Felis et al., 2009, 2018; Hetzinger et al., 2010; Nurhati et al., 2011; Cahyarini et al., 2014; Wu et al., 2014; Murty et al., 2017, 2018b; Hennekam et al., 2018; von Reumont et al., 2018; Pfeiffer et al., 2019; Ramos et al., 2019, 2020; Sayani et al., 2019). Coral-based reconstructions have provided much-needed insights into local SST and SSS at many tropical sites; however, the utility of this archive in reconstructing regional- and global-scale signals has been limited by the scarcity of long-term paired coral δ18O and records and the methodological challenges of deriving seawater δ18O changes from these records.
Recent data-synthesis efforts within the international paleoclimate community, under the auspices of the Past Global Changes (PAGES) 2k network, have produced several databases to contextualize modern climate change against the background of natural climate variability over the last ∼ 2000 years, a time interval known as the Common Era (CE) (e.g., PAGES 2k Consortium, 2013; Tierney et al., 2015; PAGES2k Consortium, 2017; Atsawawaranunt et al., 2018; Konecky et al., 2020; Comas-Bru et al., 2020). These data sets, combined with climate simulations, have been instrumental in improving our understanding of CE climate variability and its dynamics (e.g., Abram et al., 2016; Neukom et al., 2019; PAGES 2k Consortium, 2019). Notably, the PAGES Ocean2k project compiled a network of published coral δ18O, , and extension-rate records to reconstruct tropical SST evolution over the past few centuries (Tierney et al., 2015). More recently, the PAGES Iso2k project compiled water isotope records from a variety of terrestrial and marine archives (Konecky et al., 2020), including corals, to investigate temperature-driven changes in the global hydrological cycle (Konecky et al., 2023). Building on these previous efforts, the CoralHydro2k project brought the global coral paleoclimate community together to address existing data-archiving needs and access issues as well as the lack of standardized, best-practice methodology for calibrating coral proxies to climate variables and deriving δ18Osw changes from paired δ18O and records.
Here we present the PAGES CoralHydro2k database, a new, actively curated compilation of coral δ18O and records from the last 2000 years that serve as proxies for near-surface conditions across the tropical and subtropical oceans. This new database employs metadata standards established by the Marine Annually Resolved Proxy Archives (MARPA; Dassié et al., 2017) and the Paleoclimate Community reporTing Standard (PaCTS 1.0; Khider et al., 2019) and is built using the Linked Paleo Data (LiPD) framework (McKay and Emile-Geay, 2016). This first paper from the CoralHydro2k project outlines this new database, its functionality, and plans for active curation of records and future updates. As this database represents the most comprehensive collection of coral records to date, we highlight the existing spatiotemporal coverage and identify opportunities for future data collection.
2.1 Collaborative model
CoralHydro2k was one of nine projects that made up Phase 3 of the PAGES 2k network, a long-standing effort to study climate variability over the last 2000 years (PAGES 2k Network Coordinators, 2017), and continues into Phase 4 of the working group. The CoralHydro2k project was established at the 2017 PAGES Open Science Meeting in Zaragoza, Spain, inspired by the PAGES Hydro2k Workshop in 2016 (PAGES Hydro2k Consortium, 2017). Recurring calls for participation were distributed within the international paleoclimate community to recruit a team with diverse expertise ranging from coral paleothermometry to paleodata assimilation. The resulting CoralHydro2k community is composed of more than 40 volunteer scientists from all academic levels, including undergraduate and graduate students, postdoctoral researchers, and early to senior-level scientists from a variety of international academic and research institutions. Data compilation, initial analysis, and interpretation were done collaboratively and subdivided among thematic working groups as the project progressed. The majority of the work was completed remotely and asynchronously across several virtual platforms (Google Suite, Slack, and Zoom). One in-person meeting with limited remote participation took place in 2019 as a side meeting at the 13th International Conference on Paleoceanography (ICP13) in Sydney, Australia (Hargreaves et al., 2020).
2.2 Record selection and aggregation
Record-selection criteria for the CoralHydro2k database were designed to be as inclusive and comprehensive as possible to develop a versatile database that supports the project's goal of reconstructing tropical hydroclimatic variability on seasonal and longer timescales. The database also supports the broader climate community's need for a uniform global database of coral records for comparison with climate model output over the past 2000 years, especially isotope-enabled models. The CoralHydro2k team selected Common Era coral records that were at least 10 years in length, measured either δ18O, , or both, were published in a peer-reviewed scientific journal, and were archived with an absolute chronology (i.e., time in years CE). For studies where “composite records” or average time series of multiple cores from a single site were publicly available, we included either the composite record or its constituent time series but not both. Composite records are flagged as such in the database.
Coral records were sourced from past PAGES 2k data compilations with more restrictive selection criteria, such as Ocean2k (Tierney et al., 2015) and Iso2k (Konecky et al., 2020), and from public repositories such as the World Data Center PANGAEA (https://www.pangaea.de/, last access: 19 May 2022) and the NOAA National Centers for Environmental Information (NCEI) World Data Service for Paleoclimatology (https://www.ncei.noaa.gov/products/paleoclimatology, last access: 19 May 2022). For a few studies where data were not archived in public repositories, we retrieved the records from publications and supplemental information or contacted the corresponding authors. In addition to being compiled in the CoralHydro2k database, 27 previously unarchived records were submitted to the NOAA's NCEI database for archival by CoralHydro2k project members.
2.3 Database organization
Coral records in the database are organized into seven groups based on the availability of paired proxy time series, temporal coverage, and record resolution (Table 1). Groups 1–3 contain records with paired –δ18O time series. Group 1 records have monthly to bimonthly temporal resolution and cover at least 80 % of the 20th century. Records in Group 2 are similar in resolution to records in Group 1 but cover less than 80 % of the 20th century. Group 3 records contain any paired –δ18O time series that have lower than bimonthly resolution. Group 4 records are δ18O-only time series with monthly to bimonthly resolution, while Group 5 records are δ18O-only time series with lower than bimonthly resolution. Groups 6 and 7 mirror Groups 4 and 5, respectively, but for -only records.
Following the Iso2k database protocols (Konecky et al., 2020), each record in the CoralHydro2k database is assigned a unique nine-digit alphanumeric identifier. These unique identifiers are generated using the first two letters of the lead author surname (AN), the last two digits of the publication year (01), a three-letter code indicating the location of the record (ABC), and a two-digit core-ID number (02). The two-digit core-ID number begins at “01” by default and increases with each successive record from the same site and publication. Identifiers have the final format “AN01ABC02”. For example, record AB08MEN01 was published by Abram et al. (2008), is a record from the Mentawai Islands, and is the first core from that study.
2.4 Metadata
The CoralHydro2k database contains 55 metadata fields that inform the use of each coral record: 32 metadata fields are standardized and quality-controlled, while 23 fields are unstructured. Standardized metadata fields use controlled vocabulary or numeric information with uniform units, making them easily searchable by database users. Unstructured metadata are free-form text entries that are less rigorously quality-controlled but are included to aid the interpretation of the coral records. The names of standardized metadata fields are italicized in Tables 2 to 6, while the names of unstructured metadata fields are not.
Table 2Entity metadata. Information relating directly to the coral proxy record, including location, core name, species, and time span. Fields containing standardized data, e.g., uniform units, format, or controlled vocabulary, are italicized.
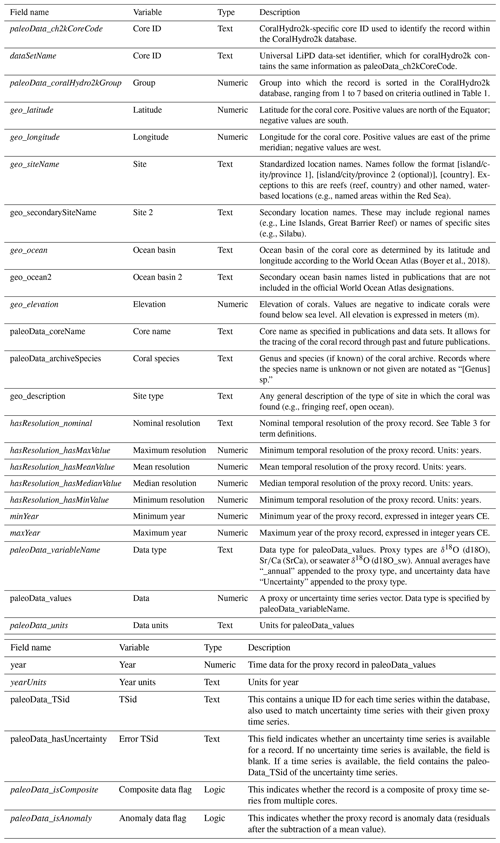
Table 3Nominal resolution descriptors. Defines the number of “data points per year” that were used to determine the nominal resolution label for each proxy record. Fields containing standardized data, e.g., uniform units or controlled vocabulary, are italicized.
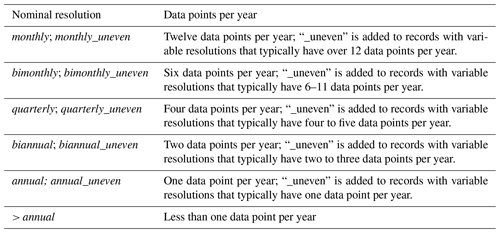
Metadata included in the CoralHydro2k database are organized into four categories (entity, publication, analysis, and calibration) based on standards recommended by MARPA (Dassié et al., 2017) and PaCTS1.0 (Khider et al., 2019). Entity metadata provide identifying information for each coral record (Table 2), including geographic coordinates, location names, water depth of the coral colony, coral species, and any core names included in the original publications. Also included in entity metadata are resolution information and the start and end years of each record. Record resolution is provided as the minimum, maximum, mean, and median data points per year for each record. A nominal label for resolution (monthly, bimonthly, quarterly, biannual, annual, or > annual, described in Table 3), based on the modal resolution of a record, is also included to allow users to easily search for records. The term “_uneven” is appended to the nominal label for records that have a variable resolution. Care should be used when interpolating these records to even sampling resolution for analysis because, although most are relatively evenly sampled, some records have sections of substantially higher or lower resolution.
Table 4Publication metadata. Details on publication information for up to three publications associated with each coral record. Fields containing standardized data, e.g., uniform units or controlled vocabulary, are italicized.
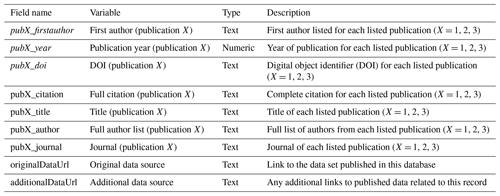
Publication metadata (Table 4) contain bibliographical information for each coral record, including digital object identifiers (DOIs) for publications and links to the public repository from which the data were retrieved. For records featured in multiple publications, bibliographical information for publications is stored in the order established by the source data repository. The first citations are found in the pub1 metadata fields, and subsequent citations are found in pub2 and pub3.
Table 5Analysis metadata. Coral sampling information, units used, and any additional notes on the coral record. Fields containing standardized data, e.g., uniform units or controlled vocabulary, are italicized.
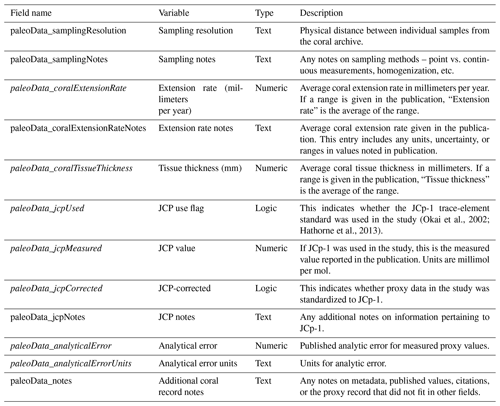
Table 6Calibration metadata. Any published information on the calibration of the coral record to sea surface temperature. There are no standardized metadata fields in this table.
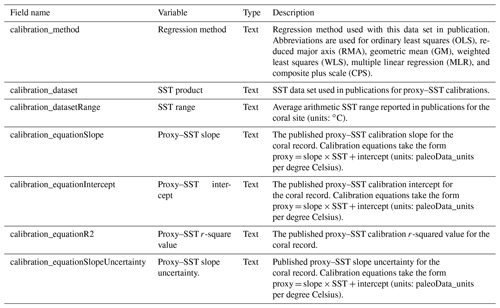
Analysis metadata (Table 5) provide information about the laboratory analysis of the samples, including (when available) information related to subsampling the cores, coral extension rate, and tissue thickness, the units of reported variables, and the analytical precision for geochemical time series. When available, information on the measurement of the international coral reference material JCp-1 (Okai et al., 2002; Hathorne et al., 2013) is included for records. Calibration metadata (Table 6) include any proxy–SST slopes, intercepts, correlations, and information about regression methods used, as reported in the original publications. These calibration metadata may differ from the standardized calibration results that we calculate across the whole database and report in Sect. 3.2 below.
2.5 Quality control and validation
As records included in the CoralHydro2k database are published in peer-reviewed scientific journals, our quality-control efforts were focused on the consistency of metadata and the accurate integration of records into the database. More specifically, the quality-control team worked to ensure that (i) metadata and proxy time series were entered correctly into the database, (ii) metadata followed a standardized vocabulary or format, and (iii) records were sorted into the correct group based on the types of proxies available, length, and resolution. For sites where coral records were either extended or revised in subsequent studies, we include the most recent version of the record in the database and include citation information and other metadata from previous studies. A quality-control checklist was used to ensure each field was in a standard format and contained information consistent with that in original publications and other online repositories. When information was unavailable, the corresponding fields were left blank.
Users of the database should not view the inclusion of a record as an endorsement of its fidelity by CoralHydro2k for reconstructing a climate parameter, as non-climatic factors (e.g., coral skeletal structure or growth rate) can complicate the extraction of climate signals from geochemical records (see Reed et al., 2021; DeLong et al., 2013, 2016). We strongly suggest users further assess records and original publications or consult the original author or a coral paleoclimate expert if they have questions or concerns.
2.6 Relation to other PAGES 2k products
CoralHydro2k was inspired by PAGES (2k) compilations of marine and hydrological proxy records such as Ocean2k (Tierney et al., 2015; McGregor et al., 2015), SISAL (Atsawawaranunt et al., 2018; Comas-Bru et al., 2020), and Iso2k (Konecky et al., 2020) but was created to address a different set of research questions. As the database is designed specifically for coral-based proxy records, we employ more inclusive record-selection criteria that allow us to include records that do not meet the length requirements of previous PAGES 2k data compilations but are important to contextualize ongoing climate change during the Common Era. The CoralHydro2k database also contains new, updated, or extended records that were published after previous PAGES efforts and will continue to be actively curated and updated annually. With a more comprehensive coral database, the CoralHydro2k project will investigate methodological differences in proxy–SST calibrations, explore methodologies for deriving coral-based δ18Osw reconstructions, refine proxy-system models for coral and δ18O time series that enable proxy data and climate model intercomparison, and provide a denser proxy network for paleodata-assimilation efforts.
3.1 Spatial and temporal coverage
The CoralHydro2k database includes 233 proxy time series from 124 unique locations sorted into seven groups (Fig. 1a). The proxy time series are stored as “records”, with 54 records containing paired and δ18O time series, 79 records containing only δ18O time series, and 46 records containing only time series. For 19 of the paired δ18O and records, we also include in the database the coral-derived δ18Osw time series calculated by the authors of the original publication. Records in the CoralHydro2k database extend from 33∘ N to 28∘ S and across all tropical oceans. The majority of these records are concentrated in the Indo-Pacific Warm Pool and the western tropical Atlantic, as conditions there are favorable for coral growth and reefs are more accessible to researchers. Record density is low in the eastern tropical Pacific and eastern tropical Atlantic, where cooler and/or more variable ocean conditions are generally unfavorable for coral growth.
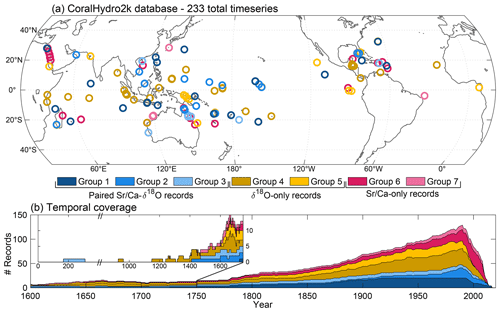
Figure 1CoralHydro2k database records are divided across Groups 1–7 based on their available proxy information. See Table 1 for a summary of group descriptions. (a) Spatial distribution of all records in the CoralHydro2k database. (b) Temporal coverage of all records in the CoralHydro2k database. Inset shows earlier records (0–1750 CE).
The majority of records in the database fall between 1800 and 2010 CE (Fig. 1b). Approximately 28 % of records in the database cover time intervals earlier than the 1800s, with most of these records coming from corals that are dead when collected (often referred to as “fossil” corals), which provide short, discrete time series often spanning several decades that have been used to reconstruct preindustrial tropical climate variability (e.g., Cobb et al., 2003a; Wu et al., 2017; Abram et al., 2020). The oldest such record in the database is a coral from Hainan Island in the South China Sea that covers 167–309 CE (Xiao et al., 2017).
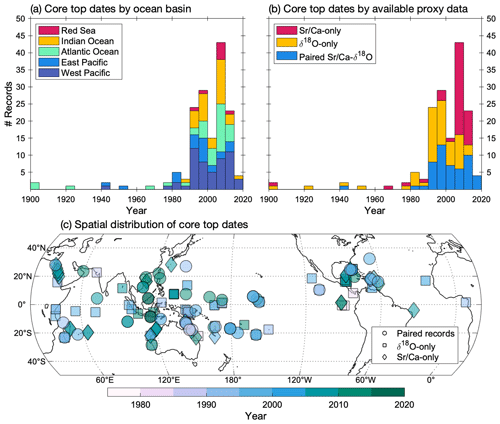
Figure 2Total number of records with core-top dates between 1900 and 2020 CE, sorted into 5-year bins and organized by (a) ocean basin and (b) available proxy data. Records with core-top dates prior to 1900 CE are not shown. The eastern and western Pacific Ocean are split at 180∘ longitude. (c) Global core-top date spatial distribution of coral records in the CoralHydro2k database. Records with core-top dates prior to 1975 CE are not shown (31 records).
A surge in coral-based proxy-record generation began in the early 1990s and is reflected in the most common core-top ages occurring in the period from 1990 to 2015 CE (Fig. 2a–b). Peak record density occurs between the late 1980s and early 1990s (Fig. 1b), reflecting increased coral-coring efforts in all tropical oceans from 1985 to 2015 CE (Fig. 2) that increase data coverage across this interval. Record density precipitously drops after 1998 CE (Fig. 1b), which may simply reflect the 5- to 15-year delay between core collection and record publication. However, we observe that fewer new records are available from more remote regions of the tropics (Fig. 2c). The availability of and paired records began in the late 1990s (Fig. 2b) with the development of a rapid, high-precision, and cost-effective method for measuring using inductively coupled plasma optical emission spectrometry (ICP-OES) (Schrag, 1999). and paired records in the database that have core-top dates prior to the late 1990s typically represent updates or extensions to previously published δ18O records (e.g., Felis et al., 2000, 2018) or fossil records.
A majority of the records included in the CoralHydro2k database offer seasonal or subseasonal resolution: 76 % of the records in the CoralHydro2k database have monthly or bimonthly resolution, 6 % have quarterly to biannual resolution, and 18 % have annual or lower resolution (Fig. 3).
3.2 Relationship with sea surface temperature
Proxy records in the CoralHydro2k database capture SST variability on seasonal and longer timescales. To highlight relationships between temperature and proxy records in CoralHydro2k, we calculate Pearson correlation coefficients between the records and local SST (2∘ grid area) from the NOAA ERSSTv5 data set (Huang et al., 2017). Significance is assessed here at the 90 % confidence level: for bimonthly average data, 92 % of and 96 % of δ18O records have a significant correlation with SST. Significant absolute correlations range from 0.23 to 0.94 (–SST) and from 0.13 to 0.89 (δ18O–SST) for the interval 1950–2020 CE, with a median correlation of 0.74 for –SST and 0.59 for δ18O–SST (Fig. 4a, c). Bimonthly average correlations are generally stronger at higher latitudes, where the seasonal range in temperature is larger.
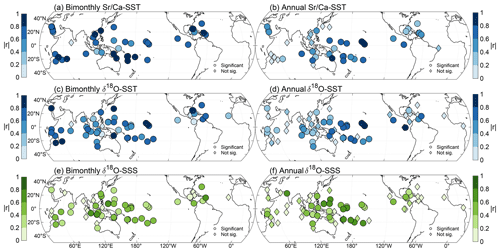
Figure 4Absolute correlations between coral and δ18O and local sea surface temperature (SST) from 1950 to 2020 CE (a–d) and between coral δ18O and local sea surface salinity (SSS) (e–f) from 1970 to 2010 CE at bimonthly (a, c, e) and annual (April–March; b, d, f) resolutions. SST and SSS were taken from the grid box nearest to each coral record in the NOAA ERSSTv5 (Huang et al., 2017) and Hadley EN4 (Good et al., 2013) data sets, respectively. Significant correlations are denoted by circles (greater than 90 % confidence interval), and nonsignificant correlations are denoted by diamonds. We note that significance can vary based on the choice of gridded data set and grid box, annual-averaging period, and correlation interval, and as such, the values shown here may differ from those reported in the original publications for each record. Correlations are shown as absolute values for ease of visualization, but we note that the linear relationship between SST and coral or δ18O is negative.
Significant absolute correlations between annual-average proxy time series and local SST range from 0.26 to 0.89 for data and from 0.26 to 0.92 for δ18O data, with medians of 0.50 for –SST and 0.57 for δ18O–SST correlations (Fig. 4b, d). For annual-average data, 43 % of –SST and 56 % of δ18O–SST correlations are significant. Here, we use the April–March tropical year for annual averages to avoid splitting large-scale tropical variability between years (Ropelewski and Halpert, 1987). The higher annual proxy–SST correlations occur near the Equator, particularly in the central and western tropical Pacific, where the ENSO drives large SST changes on interannual timescales.
We note that significant discrepancies exist among gridded SST data products (e.g., HadISST, ERSST, OISST) due to the scarcity of observations across space and time and the different statistical techniques used to infill missing data in each SST data product (e.g., Deser et al., 2010; Freeman et al., 2017; Kennedy et al., 2019). Thus, the proxy–SST correlations presented here may deviate from those stated in each record's original publication.
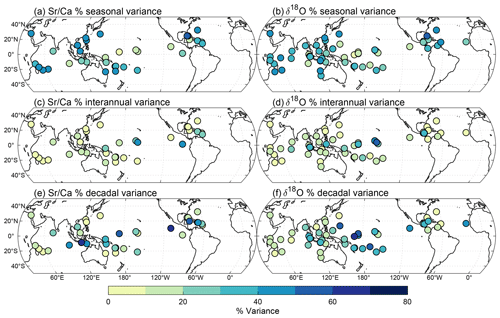
Figure 5Percent variance of coral (a, c, e) and δ18O (b, d, f) records calculated as the fraction of variance that each timescale of variability contributes to total time series variance. Variance is calculated across the full length of each coral record. (a–b) High-pass variability calculated using a 13-month filter. (c–d) The 2–7-year bandpass percent variability that includes interannual variance driven by the El Niño–Southern Oscillation (ENSO). (e–f) The 10-year low-pass variability. All percent variability was calculated only for records at least 30 years in length.
Patterns observed in proxy–SST correlations are also mirrored in the dominant frequency of variability displayed by each record. To examine the relative contributions of seasonal, interannual, and decadal variability in coral records, we apply a 13-month high-pass (seasonal), 2–7-year bandpass (interannual), and 10-year low-pass filter to all monthly and bimonthly records that are at least 30 years long. Filtering was performed using a sixth-order Butterworth filter in MATLAB, with the filter order used to optimize filtering in the decadal band. Variance for each filtered series is normalized by the proxy record variance determined for the entire record length to enable comparison between δ18O and (Fig. 5). The seasonal variance in both proxies increases with latitude (Fig. 5a–b), with records in the subtropics exhibiting greater seasonal variance than records close to the Equator. Conversely, records in the Indo-Pacific Warm Pool contain higher proportions of interannual variance (Fig. 5c–d). This pattern is more apparent among longer coral δ18O records, as several records in the database do not meet the 30-year length requirement for bandpass filtering.
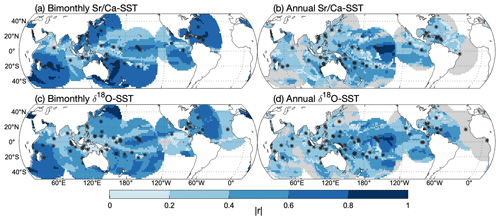
Figure 6Median absolute correlation between SST (2∘ × 2∘ grid boxes, ERSSTv5) and coral proxy records within a 3000 km radius. Bimonthly (a, c) and annual (April–March; b, d) correlations shown are significant at the 90 % confidence level. Grid boxes with records within 3000 km but no significant correlations are shaded gray. Correlations are calculated using available data from 1950 to 2020 CE. Record locations are indicated by black asterisks.
For global or regional climate reconstructions, it is useful to consider the relationship of gridded SST data products with the proxy network as opposed to the relationship of individual records with the nearest grid point in those data products. To assess the reconstruction potential of the CoralHydro2k proxy network, we calculate the median absolute correlation between each ERSSTv5 grid box and all available records in the database within a 3000 km radius (Fig. 6). We find significant (assuming a 90 % confidence interval) annual –SST correlations across 56 % of the tropical and subtropical oceans (Fig. 6b) and significant annual δ18O–SST correlations across 60 % (Fig. 6d). Consistent with previous results, bimonthly correlations between SST and both proxies are higher (Fig. 6a, c) due to the seasonal cycle. While non-climatic factors, such as age–model errors (Comboul et al., 2014; Lawman et al., 2020b; Loope et al., 2020), may lower the correlation between coral proxies and SST in some regions, significant correlations observed here highlight the fact that the CoralHydro2k database captures regional to global patterns of climate variability and thus is suitable for reconstructing SST variability across much of the tropical and subtropical oceans. Reconstruction potential is limited in the eastern Pacific and eastern Atlantic due to the scarcity of corals from those regions.
3.3 Relationship with hydrology
Coral δ18O records capture combined changes in local SST and δ18Osw, with the latter reflecting the balance among hydrological processes (e.g., precipitation, evaporation, horizontal and vertical ocean advection). Since observed δ18Osw data coverage is limited through space and time (e.g., LeGrande and Schmidt, 2006; Boyer et al., 2018; Breitkreuz et al., 2018), we compare each coral δ18O record to SSS from the nearest Hadley EN4.2.1 grid box (Good et al., 2013; Gouretski and Reseghetti, 2010) as both SSS and δ18Osw variability are driven by similar hydrological processes. However, we do note that the relationship between these two variables may not be spatiotemporally constant (Conroy et al., 2014, 2017). Significant absolute correlations between coral δ18O and SSS between 1970 and 2010 range from 0.16 to 0.69 at bimonthly resolution and from 0.28 to 0.79 at annual resolution (Fig. 4e–f). The highest correlations occur in the Western Pacific Warm Pool region, where there is stronger SSS variability due to factors that do not strongly covary with temperature such as terrestrial runoff and ocean mixing (Qu et al., 2014; Murty et al., 2017, 2018b). For western Pacific sites further away from the Maritime Continent, higher SSS–δ18O correlations may reflect the strong covariance between SSS and SST, especially on interannual timescales. In contrast, coral δ18O records from sites close to the Equator in the Indian and central equatorial Pacific oceans exhibit lower δ18O–SSS correlations, which suggests that SSS variability at these sites is smaller relative to SST or may point to potential biases in gridded SSS data products.
Many δ18O–SSS correlations at annual resolution are not significant; however, this may be more reflective of the SSS data set used here rather than the integrity of records in the database. Historical SSS observational records are much shorter and sparser than SST before the satellite era (Good et al., 2013; Boyer et al., 2018; Friedman et al., 2017), especially in the tropical and subtropical oceans. Consequently, much larger discrepancies exist among gridded SSS data sets than those found between gridded SST products (Carton et al., 2018, 2019; Zweng et al., 2019). New and emerging salinity products such as NASA's Soil Moisture Active Passive (SMAP) Sea Surface Salinity (Vazquez-Cuervo and Gomez-Valdes, 2018), the ESA's Soil Moisture and Ocean Salinity Mission (SMOS; Boutin et al., 2016), Aquarius (Drucker and Riser, 2014), and Argo (Schmid et al., 2007) will be important calibration data sets for future coral studies or reconstructions that cover the years since 2011 CE. Nonetheless, the lack of long, historical SSS records highlights the need for independent coral-based constraints on long-term hydrological trends across the tropical and subtropical oceans.
3.4 Local reproducibility of and δ18O records
We assess the “local” reproducibility of coral records in the database by comparing each proxy record to records of the same type within a 50 km radius with at least 20 years in common (Fig. 7). As the CoralHydro2k database represents the most comprehensive coral-based proxy compilation effort to date, approximately 36 % of the records are within a 50 km radius of one to five contemporaneous records. Bimonthly absolute correlations for records within 50 km of each other range from 0.11 to 0.95 (Fig. 7a), and bimonthly δ18O correlations range from 0.24 to 0.79 (Fig. 7c). Similarly, annual correlations for records within 50 km of each other range from 0.04 to 0.59 (Fig. 7b), and annual correlations for δ18O records range from 0.01 to 0.87 (Fig. 7d). While we observe good reproducibility at most sites, the highest degree of local reproducibility among both and δ18O records occurs in more open ocean settings (e.g., the central Pacific), where there is less spatial variability in growth environments, ocean advection patterns, local SST, and SSS across short distances.
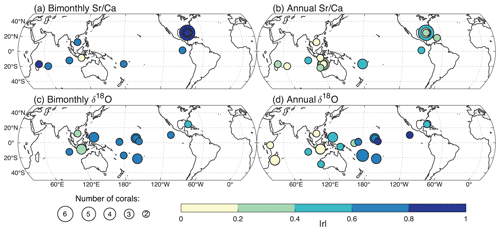
Figure 7Median absolute correlation between coral proxy records located within a 50 km radius. Correlation was calculated over the common time period between overlapping records, provided that there was a minimum of 20 years of overlap. Records that are not within 50 km of another record are not shown. Marker size indicates the number of records used in the median correlation calculation at each site (largest: N = 6).
Reproducibility studies show that proxy records from corals growing on the same reef can exhibit inconsistent mean values (e.g., Giry et al., 2012; Felis et al., 2003, 2004, 2014; DeLong et al., 2007, 2013; Sayani et al., 2021) or proxy–SST relationships (e.g., DeLong et al., 2012; Sayani et al., 2019). The exact causes of intercolony variability are not known. Intercolony differences in mean values are often attributed to subtle differences in reef environments, unresolved interspecies differences, or “vital effects”: a catch-all term used to describe a myriad of unknown physiological and/or metabolic processes that impact the incorporation of oxygen isotopes and trace elements into coral skeletons (Weber, 1973; Weber and Woodhead, 1972; McConnaughey, 1989). Proxy–SST relationships may also vary among sites due to different coral microsampling methods (e.g., punch/spot drilling versus continuous micro-milling), the lack of standardized analytical methods for measuring prior to the use of the coral reference material JCp-1 (Hathorne et al., 2013), differences in regression methods and instrumental data sets used to calculate regressions (Corrège, 2006), and other regional parameters (Murty et al., 2018a). Despite these intercolony differences, contemporaneous proxy records often exhibit similar seasonal and interannual variability (e.g., Felis et al., 2004; Giry et al., 2012; DeLong et al., 2012, 2014; Kuffner et al., 2017; Sayani et al., 2019), highlighting that coral records are indeed capturing common climate signals and can be used to reconstruct regional and global climate trends and variability.
While intercolony variability has mostly been studied in massive Porites spp. corals, which are widely distributed throughout the Indian and Pacific oceans and most commonly used in paleoclimate reconstructions, some species (e.g., Siderastrea siderea, found in the Atlantic Ocean) exhibit more reproducibility among coral colonies in , δ18O, and calibration equations (Maupin et al., 2008; DeLong et al., 2014, 2016; Kuffner et al., 2017; Weerabaddana et al., 2021). More work is needed to both quantify intercolony variability in different coral species and understand the impact of the calibration method on coral-based temperature reconstructions.
4.1 General applications
The CoralHydro2k database is the most comprehensive compilation of coral δ18O and records to date. The database offers extensive coverage of monthly to annually resolved marine proxy records that can be used to investigate near-surface hydrology and temperature variability across the global tropics and subtropics. Comparable information at similarly high resolution is rarely available with other marine paleo-archives. Paired coral –δ18O records allow for independent reconstruction and investigation of preindustrial temperature and hydrologic changes at seasonal, interannual, and decadal timescales. The inclusion of both unpaired and short proxy records, many of which did not meet the selection criteria of previous PAGES 2k data compilations, allows the CoralHydro2k database to be used for applications beyond large-scale temperature and hydrology reconstructions. This includes, and is certainly not limited to, proxy calibration studies, proxy-system model development, and paleodata-assimilation efforts. Records in the CoralHydro2k database can also be compared to model outputs, either by converting coral into temperature for direct comparison or by using proxy-system modeling to estimate proxy composition from climate model output. Coral δ18O records and coral-derived δ18Osw records can also be directly compared with new simulations from isotope-enabled models. A brief overview of how to access, query, and cite the database is provided in the sections below.
4.2 Searching the CoralHydro2k database
All three serializations of the CoralHydro2k database store data in two main containers. The first container, labeled “D”, stores records and metadata under each record's unique identifier as described in Sect. 2.3. The second container, labeled “TS”, is a “flattened” form of the database, where information for all records is stored in a format that resembles a spreadsheet. The unique identifier for the coral record each time series belongs to can be found in the dataSetName and paleoData_ch2kCoreCode fields within this flat format. MATLAB and R serializations also have an “sTS” container to be consistent with other PAGES2k data sets, which contains the same information as container “TS”. Proxy records stored in the CoralHydro2k database can be searched for using a variety of keywords or parameters. Users can search, filter, or create subsets of the database in a number of different ways by creating their own scripts in MATLAB, R, or Python. One potential starting point is to filter or create a subset of records based on the paleoData_coralHydro2kGroup field, which categorizes records based on the predefined proxy, resolution, and temporal coverage groupings (Table 1) described in Sect. 2.3. Additional ways to query the database include but are not limited to the following.
-
Proxy type, using the field paleoData_variableName to select either Sr Ca or δ18O records.
-
Temporal coverage, using minYear and maxYear to search for proxy records that fall within a specific time period.
-
Record resolution, which can be searched by nominal temporal resolution (Table 3) using hasResolution_nominal or numerically (minimum, mean, median, maximum) using fields beginning with “hasResolution_”. See Table 2 for more information.
-
Location, using geographic coordinates (geo_latitude and geo_longitude), site name (geo_siteName), or ocean basin (geo_ocean). geo_ocean is the level-one ocean basin listed in the World Ocean Atlas (Boyer et al., 2018) for the geographic coordinates of the record. Coral species, using paleoData_archiveSpecies to search for records from a particular coral species, e.g., Porites spp.
A Python demo and example MATLAB and R scripts are archived with the database to help guide users on how to access and search the database in their preferred programming language.
5.1 The CoralHydro2k database
The development of the CoralHydro2k database was guided by FAIR data principles (Wilkinson et al., 2016), which strive to make scholarly data findable, accessible, interoperable, and reusable. Thus, the CoralHydro2k database employs the LiPD framework (McKay and Emile-Geay, 2016), a standardized, machine-readable format for archiving and describing paleoclimate data, with serializations for MATLAB, R, and Python. The database is archived on the NOAA NCEI World Data Service for Paleoclimatology. To access the database, visit https://doi.org/10.25921/yp94-v135 (Walter et al., 2022) and click on “NOAA Study Page”. Alternatively, the NOAA Study Page can be accessed at https://www.ncei.noaa.gov/access/paleo-search/study/35453 (last access: 19 May 2022). Also available on the database website and CoralHydro2k GitHub (https://github.com/CoralHydro2k/; CoralHydro2k, 2022) are example scripts to help new users search the database.
One of CoralHydro2k's core goals is to create an actively curated coral database. We encourage the community to submit newly published coral δ18O and records using the data submission form located on the repository website linked above. Newly published records submitted by record generators and sourced by the CoralHydro2k team from public archives will be compiled and added to the database on an annual basis. Updates to the database will follow the versioning scheme used by the PAGES2k database (PAGES2k Consortium, 2017). The first release of the CoralHydro2k database is version 1.0.0. The version number has three counters in the following form: C1.C2.C3. The first counter, C1, is updated with each publication of a formal update of the data set. The second counter, C2, is updated when a record is added or removed. The third counter, C3, is updated when a modification is made to the data or metadata. It is anticipated that future versions and a change log describing updates with each new version will be made available at the same location as the original data release.
Researchers utilizing the whole CoralHydro2k database or a significant portion of the database should cite this paper and the paper describing the most recent version of the database. When using any subset of the CoralHydro2k database, researchers are strongly encouraged to cite the original and all associated publications for each record used, provided that this does not cause the publication to exceed the reference limit for the target journal. Citation information associated with each record in the database, including a full bibliography and DOIs and a link to the original public archive of each data set, is included in the metadata to facilitate users in crediting the original data generators in their use of the coral data.
5.2 Underlying data and sources
The figures and comparisons presented in this paper rely on data obtained from the following sources. Sea surface temperature data were obtained from ERSSTv5 (Huang et al., 2017), which is archived by the NOAA/OAR/ESRL Physical Sciences Laboratory in Boulder, Colorado, USA, and is available on their website at https://psl.noaa.gov/data/gridded/data.noaa.ersst.v5.html (last access: 19 May 2022). Salinity data were obtained from the Met Office Hadley Centre's EN4.2.1 dataset (Good et al., 2013) and are available on their website at http://www.metoffice.gov.uk/hadobs (last access: 19 May 2022). All maps displayed in Figs. 1–7 were generated using the M_Map MATLAB package (Pawlowicz, 2020) available at https://www.eoas.ubc.ca/~rich/map.html (last access: 19 May 2022).
Shallow-water corals provide monthly to annually resolved climate records from data-scarce locations across the tropical and subtropical oceans and are incredibly useful for extending modern-day observations back into the preindustrial era, contextualizing anthropogenic climate trends, and improving the skill of future climate projections. The PAGES CoralHydro2k project was formed to facilitate the use of coral paleoclimate records by the broader scientific community. Our first effort on this front is the CoralHydro2k database: a mostly unfunded endeavor representing the collective efforts of more than 40 researchers across different career stages, institutes, and time zones, meeting monthly to biweekly and working asynchronously over the past 5 years. Subsequent publications from the CoralHydro2k project will use this database to evaluate proxy–SST calibrations and methodological differences used in coral-based climate reconstructions and investigate past tropical ocean hydroclimate trends using data assimilation and comparison to isotope-enabled models. Furthermore, the CoralHydro2k team has also been collecting instrumental seawater δ18O data as part of our database-compilation efforts. That database will be released in the near future – also following the FAIR standards – and will also be maintained with active curation (see DeLong et al., 2022). While the fruits of the CoralHydro2k database are likely to come over the next 5–10 years, continuing to invest as a community in compiling standardized data sets will inevitably elevate the utility of each record.
The CoralHydro2k database is a comprehensive, machine-readable, standardized, and actively curated database of coral δ18O and records. Records in the CoralHydro2k database track large-scale regional SST and hydrology signals across seasonal, interannual, and decadal timescales with a high degree of reproducibility. As such, the records in the database can be used for investigating tropical and subtropical SST and hydrology variability on societally relevant timescales and can be combined with large networks of terrestrial paleo-archives of climate variability such as tree rings, ice cores, or speleothems to investigate past and present ocean–atmosphere–land interactions. Moreover, the database enables global-scale comparisons of coral-based paleoclimate reconstructions with state-of-the-art climate models, either through the use of forward models (Thompson et al., 2011; Dee et al., 2015, 2017; Tardif et al., 2019) or directly in the case of isotope-enabled models (Konecky et al., 2020). The comprehensive and high-resolution nature of the CoralHydro2k database also makes it ideally suited as an input database for paleoclimate data-assimilation efforts such as the Last Millennium Reanalysis (Hakim et al., 2016; Steiger et al., 2018; Tardif et al., 2019; Sanchez et al., 2021).
The “CoralHydro2k Project Members” author group includes all named contributors as well as Sarah S. Eggleston (Past Global Changes, 3012 Bern, Switzerland), Nicholas T. Hitt (School of Geography, Environment, and Earth Sciences, Victoria University of Wellington, Wellington, 6012, New Zealand), Belen Martrat (Department of Environmental Chemistry, Spanish Council for Scientific Research (CSIC), Institute of Environmental Assessment and Water Research (IDAEA), 08034 Barcelona, Spain), Helen V. McGregor (School of Earth and Environmental Sciences, University of Wollongong, Wollongong, 2522, Australia), and Feng Zhu (School of Atmospheric Sciences, Nanjing University of Information Science & Technology, Nanjing, 211544, China).
HRS, TF, KMC, and NJA directed the CoralHydro2k project. RMW built and managed the CoralHydro2k database with supervision from HRS, technical assistance from MJF, and assistance with conversion to LiPD format from NPM. All the coauthors contributed to the design of the database, which includes the database format, record-inclusion criteria, and metadata selection and standardization. Data-curation efforts were led by HRS, RMW, BE, JAH, LDB, and KHK, with RMW, HRS, TF, NJA, AKA, ARA, LDB, EPD, KLD, BE, MJF, NFG, JAH, KHK, HK, SAM, RDR, EVR, DS, SCS, and JZ populating either data sets and/or metadata included in the CoralHydro2k database. Quality-control efforts were led by RMW, HK, BE, MJF, and KHK, with assistance from AKA, LDB, ARA, EPD, KLD, TF, NFG, SAM, RDR, EVR, HRS, and JZ. The manuscript text was written by HRS and RMW, with significant contributions from TF, KHK, KLD, NFG, and JZ and with all the coauthors providing edits and feedback throughout the process. RMW generated all figures for this paper with inputs from HRS, TF, KMC, NJA, ARA, KLD, BE, MJF, NFG, KHK, HK, DS, and SCS and with all the coauthors providing feedback on design and interpretation. RMW, JEG, RDR, ALM, and MRO created example scripts for accessing the database in MATLAB, R, and Python. HRS, RMW, and TF facilitated group meetings and workflows. TF, BE, and JAH organized the ICP13 in-person/online workshop held in Sydney, Australia, in 2019.
The contact author has declared that none of the authors has any competing interests.
Publisher’s note: Copernicus Publications remains neutral with regard to jurisdictional claims in published maps and institutional affiliations.
CoralHydro2k is a contribution to Phases 3 and 4 of the PAGES 2k network. We would like to thank PAGES IPO for providing logistical, technical, and financial support for community-driven projects such as CoralHydro2k. More specifically, we would like to extend our gratitude to Sarah Eggleston, Belen Martrat, Angela Wade, and the PAGES2k coordinators for helping facilitate various aspects of this project over the last 4 years. Most importantly, we would like to thank the original data generators of each coral-based proxy record for making their data publicly available via the World Data Center PANGAEA, the NOAA NCEI World Data Service for Paleoclimatology, or other means, without whom this effort would not be possible.
We would like to thank all the researchers whose publicly archived data were included in the CoralHydro2k database (Appendix A). We would also like to thank the following researchers who provided data that were not previously archived or that were archived in places other than NOAA or PANGAEA and are now included in CoralHydro2k and NOAA: Tianran Chen, Wenfeng Deng, Juan P. D'Olivo, Heitor Evangelista, Jennifer A. Flannery, Eberhard Gischler, Nathalie F. Goodkin, Y. Kawakubo, K. Halimeda Kilbourne, Braddock K. Linsley, Christopher R. Maupin, Hussein R. Sayani, Sujata A. Murty, David Storz, Takaaki K. Watanabe, Henry C. Wu, Hangfang Xiao.
We are also grateful for the software and data sources that made this project possible. Sea surface temperature data (ERSSTv5) were provided by the NOAA/OAR/ESRL Physical Sciences Laboratory in Boulder, Colorado, USA, from their website at https://psl.noaa.gov/data/gridded/data.noaa.ersst.v5.html (last access: 19 May 2022). Salinity data (EN4.2.1) were provided by the Met Office Hadley Centre (http://www.metoffice.gov.uk/hadobs, last access: 19 May 2022)). All maps displayed in Figs. 1–7 were generated using the M_Map MATLAB package found at https://www.eoas.ubc.ca/~rich/map.html (last access: 19 May 2022). Lastly, we would like to thank NOAA NCEI and the LiPD team for facilitating archival and distribution of the CoralHydro2k database.
This work was partially funded by Georgia Tech's President's Undergraduate Research Award (PURA) and the Rutt Bridges Undergraduate Research Award to Rachel M. Walter. Kristine L. DeLong was partially funded by National Science Foundation Award 2102931 and Department of the Interior South Central Climate Adaptation Science Center Cooperative Agreement G19AC00086. Nerilie J. Abram, Bethany Ellis, and Jessica A. Hargreaves received funding from the Australian Research Council (grant nos. FT160100029 and CE170100023).
This paper was edited by Alessio Rovere and reviewed by Oliver Bothe and one anonymous referee.
Abram, N. J., Gagan, M. K., Cole, J. E., Hantoro, W. S., and Mudelsee, M.: Recent intensification of tropical climate variability in the Indian Ocean, Nat. Geosci., 1, 849–853, https://doi.org/10.1038/ngeo357, 2008.
Abram, N. J., Dixon, B. C., Rosevear, M. G., Plunkett, B., Gagan, M. K., Hantoro, W. S., and Phipps, S. J.: Optimized coral reconstructions of the Indian Ocean Dipole: An assessment of location and length considerations, Paleoceanography, 30, 1391–1405, https://doi.org/10.1002/2015PA002810, 2015.
Abram, N. J., McGregor, H. V., Tierney, J. E., Evans, M. N., McKay, N. P., Kaufman, D. S., and PAGES 2k Consortium: Early onset of industrial-era warming across the oceans and continents, Nature, 536, 411–418, https://doi.org/10.1038/nature19082, 2016.
Abram, N. J., Wright, N. M., Ellis, B., Dixon, B. C., Wurtzel, J. B., England, M. H., Ummenhofer, C. C., Philibosian, B., Cahyarini, S. Y., Yu, T.-L., Shen, C.-C., Cheng, H., Edwards, R. L., and Heslop, D.: Coupling of Indo-Pacific climate variability over the last millennium, Nature, 579, 385–392, https://doi.org/10.1038/s41586-020-2084-4, 2020.
Alpert, A. E., Cohen, A. L., Oppo, D. W., DeCarlo, T. M., Gaetani, G. A., Hernandez-Delgado, E. A., Winter, A., and Gonneea, M. E.: Twentieth century warming of the tropical Atlantic captured by Sr-U paleothermometry, Paleoceanography, 32, 146–160, https://doi.org/10.1002/2016PA002976, 2017.
Asami, R., Yamada, T., Iryu, Y., Quinn, T. M., Meyer, C. P., and Paulay, G.: Interannual and decadal variability of the western Pacific sea surface condition for the years 1787–2000: Reconstruction based on stable isotope record from a Guam coral, J. Geophys. Res.-Oceans, 110, C05018, https://doi.org/10.1029/2004JC002555, 2005.
Atsawawaranunt, K., Comas-Bru, L., Amirnezhad Mozhdehi, S., Deininger, M., Harrison, S. P., Baker, A., Boyd, M., Kaushal, N., Ahmad, S. M., Ait Brahim, Y., Arienzo, M., Bajo, P., Braun, K., Burstyn, Y., Chawchai, S., Duan, W., Hatvani, I. G., Hu, J., Kern, Z., Labuhn, I., Lachniet, M., Lechleitner, F. A., Lorrey, A., Pérez-Mejías, C., Pickering, R., Scroxton, N., and SISAL Working Group Members: The SISAL database: a global resource to document oxygen and carbon isotope records from speleothems, Earth Syst. Sci. Data, 10, 1687–1713, https://doi.org/10.5194/essd-10-1687-2018, 2018.
Bagnato, S., Linsley, B. K., Howe, S. S., Wellington, G. M., and Salinger, J.: Evaluating the use of the massive coral Diploastrea heliopora for paleoclimate reconstruction, Paleoceanography, 19, PA1032, https://doi.org/10.1029/2003PA000935, 2004.
Beck, J. W., Edwards, R. L., Ito, E., Taylor, F. W., Recy, J., Rougerie, F., Joannot, P., and Henin, C.: Sea-Surface Temperature from Coral Skeletal Strontium/Calcium Ratios, Science, 257, 644–647, https://doi.org/10.1126/science.257.5070.644, 1992.
Boiseau, M., Juillet-Leclerc, A., Yiou, P., Salvat, B., Isdale, P., and Guillaume, M.: Atmospheric and oceanic evidences of El Niño-Southern Oscillation events in the south central Pacific Ocean from coral stable isotopic records over the last 137 years, Paleoceanography, 13, 671–685, https://doi.org/10.1029/98PA02502, 1998.
Boiseau, M., Ghil, M., and Juillet-Leclerc, A.: Climatic trends and interdecadal variability from south-central Pacific coral records, Geophys. Res. Lett., 26, 2881–2884, https://doi.org/10.1029/1999GL900595, 1999.
Bolton, A., Goodkin, N. F., Hughen, K., Ostermann, D. R., Vo, S. T., and Phan, H. K.: Paired Porites coral and δ18O from the western South China Sea: Proxy calibration of sea surface temperature and precipitation, Palaeogeogr. Palaeocl., 410, 233–243, https://doi.org/10.1016/j.palaeo.2014.05.047, 2014.
Boutin, J., Chao, Y., Asher, W. E., Delcroix, T., Drucker, R., Drushka, K., Kolodziejczyk, N., Lee, T., Reul, N., Reverdin, G., Schanze, J., Soloviev, A., Yu, L., Anderson, J., Brucker, L., Dinnat, E., Santos-Garcia, A., Jones, W. L., Maes, C., Meissner, T., Tang, W., Vinogradova, N., and Ward, B.: Satellite and In Situ Salinity: Understanding Near-Surface Stratification and Subfootprint Variability, B. Am. Meteorol. Soc., 97, 1391–1407, https://doi.org/10.1175/BAMS-D-15-00032.1, 2016.
Boyer, T. P., Baranova, O. K., Coleman, C., Garcia, H. E., Grodsky, A., Locarnini, R. A., Mishonov, A. V., Paver, C. R., Reagan, J. R., Seidov, D., Smolyar, I. V., Weathers, K. W., and Zweng, M. M.: World Ocean Database 2018, edited by: Mishonov, A. V., NOAA Atlas NESDIS 87, https://www.ncei.noaa.gov/sites/default/files/2020-04/wod_intro_0.pdf (last access: 19 May 2022), 2018.
Breitkreuz, C., Paul, A., Kurahashi-Nakamura, T., Losch, M., and Schulz, M.: A Dynamical Reconstruction of the Global Monthly Mean Oxygen Isotopic Composition of Seawater, J. Geophys. Res.-Oceans, 123, 7206–7219, https://doi.org/10.1029/2018JC014300, 2018.
Bryan, S. P., Hughen, K. A., Karnauskas, K. B., and Farrar, J. T.: Two Hundred Fifty Years of Reconstructed South Asian Summer Monsoon Intensity and Decadal-Scale Variability, Geophys. Res. Lett., 46, 3927–3935, https://doi.org/10.1029/2018GL081593, 2019.
Cahyarini, S. Y., Pfeiffer, M., Timm, O., Dullo, W.-C., and Schönberg, D. G.: Reconstructing seawater δ18O from paired coral δ18O and ratios: Methods, error analysis and problems, with examples from Tahiti (French Polynesia) and Timor (Indonesia), Geochim. Cosmochim. Ac., 72, 2841–2853, https://doi.org/10.1016/j.gca.2008.04.005, 2008.
Cahyarini, S. Y., Pfeiffer, M., Nurhati, I. S., Aldrian, E., Dullo, W.-C., and Hetzinger, S.: Twentieth century sea surface temperature and salinity variations at Timor inferred from paired coral δ18O and measurements, J. Geophys. Res.-Oceans, 119, 4593–4604, https://doi.org/10.1002/2013JC009594, 2014.
Cai, W., Borlace, S., Lengaigne, M., van Rensch, P., Collins, M., Vecchi, G., Timmermann, A., Santoso, A., McPhaden, M. J., Wu, L., England, M. H., Wang, G., Guilyardi, E., and Jin, F.-F.: Increasing frequency of extreme El Niño events due to greenhouse warming, Nat. Clim. Change, 4, 111–116, https://doi.org/10.1038/nclimate2100, 2014.
Cai, W., Wu, L., Lengaigne, M., Li, T., McGregor, S., Kug, J.-S., Yu, J.-Y., Stuecker, M. F., Santoso, A., Li, X., Ham, Y.-G., Chikamoto, Y., Ng, B., McPhaden, M. J., Du, Y., Dommenget, D., Jia, F., Kajtar, J. B., Keenlyside, N., Lin, X., Luo, J.-J., Martín-Rey, M., Ruprich-Robert, Y., Wang, G., Xie, S.-P., Yang, Y., Kang, S. M., Choi, J.-Y., Gan, B., Kim, G.-I., Kim, C.-E., Kim, S., Kim, J.-H., and Chang, P.: Pantropical climate interactions, Science, 363, eaav4236, https://doi.org/10.1126/science.aav4236, 2019.
Calvo, E., Marshall, J. F., Pelejero, C., McCulloch, M. T., Gagan, M. K., and Lough, J. M.: Interdecadal climate variability in the Coral Sea since 1708 A.D., Palaeogeogr. Palaeocl., 248, 190–201, https://doi.org/10.1016/j.palaeo.2006.12.003, 2007.
Carilli, J. E., Charles, C. D., Garren, M., McField, M., and Norris, R. D.: Baseline shifts in coral skeletal oxygen isotopic composition: a signature of symbiont shuffling?, Coral Reefs, 32, 559–571, https://doi.org/10.1007/s00338-012-1004-y, 2013.
Carilli, J. E., McGregor, H. V., Gaudry, J. J., Donner, S. D., Gagan, M. K., Stevenson, S., Wong, H., and Fink, D.: Equatorial Pacific coral geochemical records show recent weakening of the Walker Circulation, Paleoceanography, 29, 1031–1045, https://doi.org/10.1002/2014PA002683, 2014.
Carton, J. A., Chepurin, G. A., and Chen, L.: SODA3: A New Ocean Climate Reanalysis, J. Climate, 31, 6967–6983, https://doi.org/10.1175/JCLI-D-18-0149.1, 2018.
Carton, J. A., Penny, S. G., and Kalnay, E.: Temperature and Salinity Variability in the SODA3, ECCO4r3, and ORAS5 Ocean Reanalyses, J. Climate, 32, 2277–2293, https://doi.org/10.1175/JCLI-D-18-0605.1, 2019.
Chakraborty, S. and Ramesh, R.: Stable isotope variations in a coral (Favia speciosa) from the Gulf of Kutch during 1948–1989 A.D.: Environmental implications, P. Indian. A. S.-Earth, 107, 331–341, https://doi.org/10.1007/BF02841599, 1998.
Charles, C. D., Hunter, D. E., and Fairbanks, R. G.: Interaction Between the ENSO and the Asian Monsoon in a Coral Record of Tropical Climate, Science, 277, 925–928, https://doi.org/10.1126/science.277.5328.925, 1997.
Charles, C. D., Cobb, K., Moore, M. D., and Fairbanks, R. G.: Monsoon–tropical ocean interaction in a network of coral records spanning the 20th century, Mar. Geol., 201, 207–222, https://doi.org/10.1016/S0025-3227(03)00217-2, 2003.
Chen, T., Cobb, K. M., Roff, G., Zhao, J., Yang, H., Hu, M., and Zhao, K.: Coral-Derived Western Pacific Tropical Sea Surface Temperatures During the Last Millennium, Geophys. Res. Lett., 45, 3542–3549, https://doi.org/10.1002/2018GL077619, 2018.
Cheng, L., Trenberth, K. E., Gruber, N., Abraham, J. P., Fasullo, J. T., Li, G., Mann, M. E., Zhao, X., and Zhu, J.: Improved Estimates of Changes in Upper Ocean Salinity and the Hydrological Cycle, J. Climate, 33, 10357–10381, https://doi.org/10.1175/JCLI-D-20-0366.1, 2020.
Cobb, K. M., Charles, C. D., and Hunter, D. E.: A central tropical Pacific coral demonstrates Pacific, Indian, and Atlantic decadal climate connections, Geophys. Res. Lett., 8, 2209–2212, https://doi.org/10.1029/2001GL012919, 2001.
Cobb, K. M., Charles, C. D., Cheng, H., and Edwards, R. L.: El Niño/Southern Oscillation and tropical Pacific climate during the last millennium, Nature, 424, 271–276, https://doi.org/10.1038/nature01779, 2003a.
Cobb, K. M., Charles, C. D., Cheng, H., Kastner, M., and Edwards, R. L.: U/Th-dating living and young fossil corals from the central tropical Pacific, Earth Planet. Sc. Lett., 210, 91–103, https://doi.org/10.1016/S0012-821X(03)00138-9, 2003b.
Cole, J. E., Fairbanks, R. G., and Shen, G. T.: Recent Variability in the Southern Oscillation: Isotopic Results from a Tarawa Atoll Coral, Science, 260, 1790–1793, https://doi.org/10.1126/science.260.5115.1790, 1993.
Cole, J. E., Dunbar, R. B., McClanahan, T. R., and Muthiga, N. A.: Tropical Pacific Forcing of Decadal SST Variability in the Western Indian Ocean over the Past Two Centuries, Science, 287, 617–619, https://doi.org/10.1126/science.287.5453.617, 2000.
Comas-Bru, L., Rehfeld, K., Roesch, C., Amirnezhad-Mozhdehi, S., Harrison, S. P., Atsawawaranunt, K., Ahmad, S. M., Brahim, Y. A., Baker, A., Bosomworth, M., Breitenbach, S. F. M., Burstyn, Y., Columbu, A., Deininger, M., Demény, A., Dixon, B., Fohlmeister, J., Hatvani, I. G., Hu, J., Kaushal, N., Kern, Z., Labuhn, I., Lechleitner, F. A., Lorrey, A., Martrat, B., Novello, V. F., Oster, J., Pérez-Mejías, C., Scholz, D., Scroxton, N., Sinha, N., Ward, B. M., Warken, S., Zhang, H., and SISAL Working Group members: SISALv2: a comprehensive speleothem isotope database with multiple age–depth models, Earth Syst. Sci. Data, 12, 2579–2606, https://doi.org/10.5194/essd-12-2579-2020, 2020.
Comboul, M., Emile-Geay, J., Evans, M. N., Mirnateghi, N., Cobb, K. M., and Thompson, D. M.: A probabilistic model of chronological errors in layer-counted climate proxies: applications to annually banded coral archives, Clim. Past, 10, 825–841, https://doi.org/10.5194/cp-10-825-2014, 2014.
Conroy, J. L., Cobb, K. M., Lynch-Stieglitz, J., and Polissar, P. J.: Constraints on the salinity–oxygen isotope relationship in the central tropical Pacific Ocean, Mar. Chem., 161, 26–33, https://doi.org/10.1016/j.marchem.2014.02.001, 2014.
Conroy, J. L., Thompson, D. M., Cobb, K. M., Noone, D., Rea, S., and Legrande, A. N.: Spatiotemporal variability in the δ18O-salinity relationship of seawater across the tropical Pacific Ocean, Paleoceanography, 32, 484–497, https://doi.org/10.1002/2016PA003073, 2017.
CoralHydro2k: CoralHydro2k, GitHub repository [code], https://github.com/CoralHydro2k, last access: 19 May 2022.
Corrège, T.: Sea surface temperature and salinity reconstruction from coral geochemical tracers, Palaeogeogr. Palaeocl., 232, 408–428, https://doi.org/10.1016/j.palaeo.2005.10.014, 2006.
Damassa, T. D., Cole, J. E., Barnett, H. R., Ault, T. R., and McClanahan, T. R.: Enhanced multidecadal climate variability in the seventeenth century from coral isotope records in the western Indian Ocean, Paleoceanography, 21, PA2016, https://doi.org/10.1029/2005PA001217, 2006.
Dassié, E., DeLong, K., Kilbourne, H., Williams, B., Abram, N., Brenner, L., Brahmi, C., Cobb, K. M., Corrège, T., Dissard, D., Emile-Geay, J., Evangelista, H., Evans, M. N., Farmer, J., Felis, T., Gagan, M., Gillikin, D. P., Goodkin, N., Khodri, M., Lavagnino, A. C., LaVigne, M., Lazareth, C., Linsley, B., Lough, J., McGregor, H., Nurhati, I. S., Ouellette, G., Perrin, L., Raymo, M., Rosenheim, B., Sandstrom, M., Schöne, B. R., Sifeddine, A., Stevenson, S., Thompson, D. M., Waite, A., Wanamaker, A., and Wu, H.: Saving Our Marine Archives, Eos, 98, https://doi.org/10.1029/2017EO068159, 2017.
DeCarlo, T. M., Gaetani, G. A., Cohen, A. L., Foster, G. L., Alpert, A. E., and Stewart, J. A.: Coral Sr-U thermometry, Paleoceanography, 31, 626–638, https://doi.org/10.1002/2015PA002908, 2016.
Dee, S., Emile-Geay, J., Evans, M. N., Allam, A., Steig, E. J., and Thompson, D. M.: PRYSM: An open-source framework for PRoxY System Modeling, with applications to oxygen-isotope systems, J. Adv. Model Earth Sy., 7, 1220–1247, https://doi.org/10.1002/2015MS000447, 2015.
Dee, S. G., Parsons, L. A., Loope, G. R., Overpeck, J. T., Ault, T. R., and Emile-Geay, J.: Improved spectral comparisons of paleoclimate models and observations via proxy system modeling: Implications for multi-decadal variability, Earth Planet. Sc. Lett., 476, 34–46, https://doi.org/10.1016/j.epsl.2017.07.036, 2017.
DeLong, K. L., Quinn, T. M., and Taylor, F. W.: Reconstructing twentieth-century sea surface temperature variability in the southwest Pacific: A replication study using multiple coral records from New Caledonia, Paleoceanography, 22, PA4212, https://doi.org/10.1029/2007PA001444, 2007.
DeLong, K. L., Flannery, J. A., Maupin, C. R., Poore, R. Z., and Quinn, T. M.: A coral calibration and replication study of two massive corals from the Gulf of Mexico, Palaeogeogr. Palaeocl., 307, 117–128, https://doi.org/10.1016/j.palaeo.2011.05.005, 2011.
DeLong, K. L., Quinn, T. M., Taylor, F. W., Lin, K., and Shen, C.-C.: Sea surface temperature variability in the southwest tropical Pacific since AD 1649, Nat. Clim. Change, 2, 799–804, https://doi.org/10.1038/nclimate1583, 2012.
DeLong, K. L., Quinn, T. M., Taylor, F. W., Shen, C.-C., and Lin, K.: Improving coral-base paleoclimate reconstructions by replicating 350 years of coral variations, Palaeogeogr. Palaeocl., 373, 6–24, https://doi.org/10.1016/j.palaeo.2012.08.019, 2013.
DeLong, K. L., Flannery, J. A., Poore, R. Z., Quinn, T. M., Maupin, C. R., Lin, K., and Shen, C.-C.: A reconstruction of sea surface temperature variability in the southeastern Gulf of Mexico from 1734 to 2008 C.E. using cross-dated records from the coral Siderastrea siderea, Paleoceanography, 29, 403–422, https://doi.org/10.1002/2013PA002524, 2014.
DeLong, K. L., Maupin, C. R., Flannery, J. A., Quinn, T. M., and Shen, C.-C.: Refining temperature reconstructions with the Atlantic coral Siderastrea siderea, Palaeogeogr. Palaeocl., 462, 1–15, https://doi.org/10.1016/j.palaeo.2016.08.028, 2016.
DeLong, K. L., Atwood, A. R., Moore, A. L., and Sanchez, S. C.: Clues from the Sea Build a Picture of Earth's Water Cycle, Eos, 103, https://doi.org/10.1029/2022EO220231, 2022.
Deng, W., Wei, G., Xie, L., Ke, T., Wang, Z., Zeng, T., and Liu, Y.: Variations in the Pacific Decadal Oscillation since 1853 in a coral record from the northern South China Sea, J. Geophys. Res.-Oceans, 118, 2358–2366, https://doi.org/10.1002/jgrc.20180, 2013.
Deser, C., Alexander, M. A., Xie, S.-P., and Phillips, A. S.: Sea Surface Temperature Variability: Patterns and Mechanisms, Annu. Rev. Mar. Sci., 2, 115–143, https://doi.org/10.1146/annurev-marine-120408-151453, 2010.
D'Olivo, J. P., Sinclair, D. J., Rankenburg, K., and McCulloch, M. T.: A universal multi-trace element calibration for reconstructing sea surface temperatures from long-lived Porites corals: Removing `vital-effects,' Geochim. Cosmochim. Ac., 239, 109–135, https://doi.org/10.1016/j.gca.2018.07.035, 2018.
Dong, B. and Dai, A.: The influence of the Interdecadal Pacific Oscillation on Temperature and Precipitation over the Globe, Clim. Dynam., 45, 2667–2681, https://doi.org/10.1007/s00382-015-2500-x, 2015.
Draschba, S., Pätzold, J., and Wefer, G.: North Atlantic climate variability since AD 1350 recorded in δ18O and skeletal density of Bermuda corals, Int. J. Earth Sci., 88, 733–741, https://doi.org/10.1007/s005310050301, 2000.
Drucker, R. and Riser, S. C.: Validation of Aquarius sea surface salinity with Argo: Analysis of error due to depth of measurement and vertical salinity stratification, J. Geophys. Res.-Oceans, 119, 4626–4637, https://doi.org/10.1002/2014JC010045, 2014.
Druffel, E. R. M. and Griffin, S.: Large variations of surface ocean radiocarbon: Evidence of circulation changes in the southwestern Pacific, J. Geophys. Res.-Oceans, 98, 20249–20259, https://doi.org/10.1029/93JC02113, 1993.
Druffel, E. R. M. and Griffin, S.: Variability of surface ocean radiocarbon and stable isotopes in the southwestern Pacific, J. Geophys. Res.-Oceans, 104, 23607–23613, https://doi.org/10.1029/1999JC900212, 1999.
Dunbar, R. B., Wellington, G. M., Colgan, M. W., and Glynn, P. W.: Eastern Pacific sea surface temperature since 1600 A.D.: The δ18O record of climate variability in Galápagos Corals, Paleoceanography, 9, 291–315, https://doi.org/10.1029/93PA03501, 1994.
Epstein, S., Buchsbaum, R., Lowenstam, H. A., and Urey, H. C.: Revised carbonate-water isotopic temperature scale, Geol. Soc. Am. Bull., 64, 1315–1326, https://doi.org/10.1130/0016-7606(1953)64[1315:RCITS]2.0.CO;2, 1953.
Evangelista, H., Sifeddine, A., Corrège, T., Servain, J., Dassié, E. P., Logato, R., Cordeiro, R. C., Shen, C.-C., Le Cornec, F., Nogueira, J., Segal, B., Castagna, A., and Turcq, B.: Climatic Constraints on Growth Rate and Geochemistry ( and U/Ca) of the Coral Siderastrea stellata in the Southwest Equatorial Atlantic (Rocas Atoll, Brazil), Geochem. Geophy. Geosy., 19, 772–786, https://doi.org/10.1002/2017GC007365, 2018.
Evans, M. N., Fairbanks, R. G., and Rubenstone, J. L.: A proxy index of ENSO teleconnections, Nature, 394, 732–733, https://doi.org/10.1038/29424, 1998.
Felis, T.: Extending the Instrumental Record of Ocean-Atmosphere Variability into the Last Interglacial Using Tropical Corals, Oceanography, 33, 68–79, https://doi.org/10.5670/oceanog.2020.209, 2020.
Felis, T., Pätzold, J., Loya, Y., Fine, M., Nawar, A. H., and Wefer, G.: A coral oxygen isotope record from the northern Red Sea documenting NAO, ENSO, and North Pacific teleconnections on Middle East climate variability since the year 1750, Paleoceanography, 15, 679–694, https://doi.org/10.1029/1999PA000477, 2000.
Felis, T., Pätzold, J., and Loya, Y.: Mean oxygen-isotope signatures in Porites spp. corals: inter-colony variability and correction for extension-rate effects, Coral Reefs, 22, 328–336, https://doi.org/10.1007/s00338-003-0324-3, 2003.
Felis, T., Lohmann, G., Kuhnert, H., Lorenz, S. J., Scholz, D., Pätzold, J., Al-Rousan, S. A., and Al-Moghrabi, S. M.: Increased seasonality in Middle East temperatures during the last interglacial period, Nature, 429, 164–168, https://doi.org/10.1038/nature02546, 2004.
Felis, T., Suzuki, A., Kuhnert, H., Dima, M., Lohmann, G., and Kawahata, H.: Subtropical coral reveals abrupt early-twentieth-century freshening in the western North Pacific Ocean, Geology, 37, 527–530, https://doi.org/10.1130/G25581A.1, 2009.
Felis, T., McGregor, H. V., Linsley, B. K., Tudhope, A. W., Gagan, M. K., Suzuki, A., Inoue, M., Thomas, A. L., Esat, T. M., Thompson, W. G., Tiwari, M., Potts, D. C., Mudelsee, M., Yokoyama, Y., and Webster, J. M.: Intensification of the meridional temperature gradient in the Great Barrier Reef following the Last Glacial Maximum, Nat. Commun., 5, 4102, https://doi.org/10.1038/ncomms5102, 2014.
Felis, T., Ionita, M., Rimbu, N., Lohmann, G., and Kölling, M.: Mild and Arid Climate in the Eastern Sahara-Arabian Desert During the Late Little Ice Age, Geophys. Res. Lett., 45, 7112–7119, https://doi.org/10.1029/2018GL078617, 2018.
Flannery, J. A. and Poore, R. Z.: Proxy Sea-Surface Temperature Reconstructions from Modern and Holocene Montastraea faveolata Specimens from the Dry Tortugas National Park, Florida, USA, J. Coastal Res., 63, 20–31, https://doi.org/10.2112/SI63-003.1, 2013.
Flannery, J. A., Richey, J. N., Thirumalai, K., Poore, R. Z., and DeLong, K. L.: Multi-species coral -based sea-surface temperature reconstruction using Orbicella faveolata and Siderastrea siderea from the Florida Straits, Palaeogeogr. Palaeocl., 466, 100–109, https://doi.org/10.1016/j.palaeo.2016.10.022, 2017.
Flannery, J. A., Richey, J. N., Toth, L. T., Kuffner, I. B., and Poore, R. Z.: Quantifying Uncertainty in -Based Estimates of SST From the Coral Orbicella faveolata, Paleoceanography and Paleoclimatology, 33, 958–973, https://doi.org/10.1029/2018PA003389, 2018.
Fleitmann, D., Dunbar, R. B., McCulloch, M., Mudelsee, M., Vuille, M., McClanahan, T. R., Cole, J. E., and Eggins, S.: East African soil erosion recorded in a 300 year old coral colony from Kenya, Geophys. Res. Lett., 34, L04401, https://doi.org/10.1029/2006GL028525, 2007.
Freeman, E., Woodruff, S. D., Worley, S. J., Lubker, S. J., Kent, E. C., Angel, W. E., Berry, D. I., Brohan, P., Eastman, R., Gates, L., Gloeden, W., Ji, Z., Lawrimore, J., Rayner, N. A., Rosenhagen, G., and Smith, S. R.: ICOADS Release 3.0: a major update to the historical marine climate record, Int. J. Climatol., 37, 2211–2232, https://doi.org/10.1002/joc.4775, 2017.
Friedman, A. R., Reverdin, G., Khodri, M., and Gastineau, G.: A new record of Atlantic sea surface salinity from 1896 to 2013 reveals the signatures of climate variability and long-term trends, Geophys. Res. Lett., 44, 1866–1876, https://doi.org/10.1002/2017GL072582, 2017.
Gagan, M. K., Ayliffe, L. K., Hopley, D., Cali, J. A., Mortimer, G. E., Chappell, J., McCulloch, M. T., and Head, M. J.: Temperature and Surface-Ocean Water Balance of the Mid-Holocene Tropical Western Pacific, Science, 279, 1014–1018, https://doi.org/10.1126/science.279.5353.1014, 1998.
Gagan, M. K., Ayliffe, L. K., Beck, J. W., Cole, J. E., Druffel, E. R. M., Dunbar, R. B., and Schrag, D. P.: New views of tropical paleoclimates from corals, Quaternary Sci. Rev., 19, 45–64, https://doi.org/10.1016/S0277-3791(99)00054-2, 2000.
Gagan, M. K., Sosdian, S. M., Scott-Gagan, H., Sieh, K., Hantoro, W. S., Natawidjaja, D. H., Briggs, R. W., Suwargadi, B. W., and Rifai, H.: Coral 13C/12C records of vertical seafloor displacement during megathrust earthquakes west of Sumatra, Earth Planet. Sc. Lett., 432, 461–471, https://doi.org/10.1016/j.epsl.2015.10.002, 2015.
Giry, C., Felis, T., Kölling, M., Scholz, D., Wei, W., Lohmann, G., and Scheffers, S.: Mid- to late Holocene changes in tropical Atlantic temperature seasonality and interannual to multidecadal variability documented in southern Caribbean corals, Earth Planet. Sc. Lett., 331–332, 187–200, https://doi.org/10.1016/j.epsl.2012.03.019, 2012.
Good, S. A., Martin, M. J., and Rayner, N. A.: EN4: Quality controlled ocean temperature and salinity profiles and monthly objective analyses with uncertainty estimates, J. Geophys. Res.-Oceans, 118, 6704–6716, https://doi.org/10.1002/2013JC009067, 2013 (data available at: http://www.metoffice.gov.uk/hadobs, last access: 19 May 2022).
Goodkin, N. F.: Geochemistry of slow-growing corals: reconstructing sea surface temperature, salinity and the North Atlantic Oscillation, Thesis, Massachusetts Institute of Technology, http://hdl.handle.net/1721.1/40969, 2007.
Goodkin, N. F., Hughen, K. A., Cohen, A. L., and Smith, S. R.: Record of Little Ice Age sea surface temperatures at Bermuda using a growth-dependent calibration of coral , Paleoceanography, 20, PA4016, https://doi.org/10.1029/2005PA001140, 2005.
Goodkin, N. F., Hughen, K. A., Curry, W. B., Doney, S. C., and Ostermann, D. R.: Sea surface temperature and salinity variability at Bermuda during the end of the Little Ice Age, Paleoceanography, 23, PA3203, https://doi.org/10.1029/2007PA001532, 2008.
Goodkin, N. F., Samanta, D., Bolton, A., Ong, M. R., Hoang, P. K., Vo, S. T., Karnauskas, K. B., and Hughen, K. A.: Natural and Anthropogenic Forcing of Multi-Decadal to Centennial Scale Variability of Sea Surface Temperature in the South China Sea, Paleoceanography and Paleoclimatology, 36, e2021PA004233, https://doi.org/10.1029/2021PA004233, 2021.
Gorman, M. K., Quinn, T. M., Taylor, F. W., Partin, J. W., Cabioch, G., Austin Jr., J. A., Pelletier, B., Ballu, V., Maes, C., and Saustrup, S.: A coral-based reconstruction of sea surface salinity at Sabine Bank, Vanuatu from 1842 to 2007 CE, Paleoceanography, 27, PA3226, https://doi.org/10.1029/2012PA002302, 2012.
Gould, W. J. and Cunningham, S. A.: Global-scale patterns of observed sea surface salinity intensified since the 1870s, Communications Earth & Environment, 2, 76, https://doi.org/10.1038/s43247-021-00161-3, 2021.
Gouretski, V. and Reseghetti, F.: On depth and temperature biases in bathythermograph data: Development of a new correction scheme based on analysis of a global ocean database, Deep-Sea Res. Pt. I, 57, 812–833, https://doi.org/10.1016/j.dsr.2010.03.011, 2010.
Grove, C. A., Kasper, S., Zinke, J., Pfeiffer, M., Garbe-Schönberg, D., and Brummer, G.-J. A.: Confounding effects of coral growth and high SST variability on skeletal : Implications for coral paleothermometry, Geochem. Geophy. Geosy., 14, 1277–1293, https://doi.org/10.1002/ggge.20095, 2013.
Gu, G. and Adler, R. F.: Interdecadal variability/long-term changes in global precipitation patterns during the past three decades: global warming and/or pacific decadal variability?, Clim. Dynam., 40, 3009–3022, https://doi.org/10.1007/s00382-012-1443-8, 2013.
Guilderson, T. P. and Schrag, D. P.: Reliability of coral isotope records from the Western Pacific Warm Pool: A comparison using age-optimized records, Paleoceanography, 14, 457–464, https://doi.org/10.1029/1999PA900024, 1999.
Hakim, G. J., Emile-Geay, J., Steig, E. J., Noone, D., Anderson, D. M., Tardif, R., Steiger, N., and Perkins, W. A.: The last millennium climate reanalysis project: Framework and first results, J. Geophys. Res.-Atmos., 121, 6745–6764, https://doi.org/10.1002/2016JD024751, 2016.
Hargreaves, J., DeLong, K., Felis, T., Abram, N., Cobb, K., and Sayani, H.: Tropical ocean hydroclimate and temperature from coral archives, PAGES Magazine, 28, 29, https://doi.org/10.22498/pages.28.1.29, 2020.
Hasson, A. E. A., Delcroix, T., and Dussin, R.: An assessment of the mixed layer salinity budget in the tropical Pacific Ocean. Observations and modelling (1990–2009), Ocean. Dynam., 63, 179–194, https://doi.org/10.1007/s10236-013-0596-2, 2013.
Hathorne, E. C., Gagnon, A., Felis, T., Adkins, J., Asami, R., Boer, W., Caillon, N., Case, D., Cobb, K. M., Douville, E., deMenocal, P., Eisenhauer, A., Garbe-Schönberg, D., Geibert, W., Goldstein, S., Hughen, K., Inoue, M., Kawahata, H., Kölling, M., Cornec, F. L., Linsley, B. K., McGregor, H. V., Montagna, P., Nurhati, I. S., Quinn, T. M., Raddatz, J., Rebaubier, H., Robinson, L., Sadekov, A., Sherrell, R., Sinclair, D., Tudhope, A. W., Wei, G., Wong, H., Wu, H. C., and You, C.-F.: Interlaboratory study for coral and other element/Ca ratio measurements, Geochem. Geophy. Geosy., 14, 3730–3750, https://doi.org/10.1002/ggge.20230, 2013.
Held, I. M. and Soden, B. J.: Robust Responses of the Hydrological Cycle to Global Warming, J. Climate, 19, 5686–5699, https://doi.org/10.1175/JCLI3990.1, 2006.
Hendy, E. J., Gagan, M. K., Alibert, C. A., McCulloch, M. T., Lough, J. M., and Isdale, P. J.: Abrupt Decrease in Tropical Pacific Sea Surface Salinity at End of Little Ice Age, Science, 295, 1511–1514, https://doi.org/10.1126/science.1067693, 2002.
Hennekam, R., Zinke, J., van Sebille, E., ten Have, M., Brummer, G.-J. A., and Reichart, G.-J.: Cocos (Keeling) Corals Reveal 200 Years of Multidecadal Modulation of Southeast Indian Ocean Hydrology by Indonesian Throughflow, Paleoceanography and Paleoclimatology, 33, 48–60, https://doi.org/10.1002/2017PA003181, 2018.
Hereid, K. A., Quinn, T. M., Taylor, F. W., Shen, C.-C., Edwards, R. L., and Cheng, H.: Coral record of reduced El Nino activity in the early 15th to middle 17th centuries, Geology, 41, 51–54, https://doi.org/10.1130/G33510.1, 2013.
Hetzinger, S., Pfeiffer, M., Dullo, W.-C., Ruprecht, E., and Garbe-Schönberg, D.: and δ18O in a fast-growing Diploria strigosa coral: Evaluation of a new climate archive for the tropical Atlantic, Geochem. Geophy. Geosy., 7, Q10002, https://doi.org/10.1029/2006GC001347, 2006.
Hetzinger, S., Pfeiffer, M., Dullo, W.-C., Keenlyside, N., Latif, M., and Zinke, J.: Caribbean coral tracks Atlantic Multidecadal Oscillation and past hurricane activity, Geology, 36, 11–14, https://doi.org/10.1130/G24321A.1, 2008.
Hetzinger, S., Pfeiffer, M., Dullo, W.-C., Garbe-Schönberg, D., and Halfar, J.: Rapid 20th century warming in the Caribbean and impact of remote forcing on climate in the northern tropical Atlantic as recorded in a Guadeloupe coral, Palaeogeogr. Palaeocl., 296, 111–124, https://doi.org/10.1016/j.palaeo.2010.06.019, 2010.
Hickey, T. D., Reich, C. D., DeLong, K. L., Poore, R. Z., and Brock, J. C.: Holocene Core Logs and Site Methods for Modern Reef and Head-Coral Cores: Dry Tortugas National Park, Florida, U.S. Geological Survey, https://doi.org/10.3133/ofr20121095, 2013.
Huang, B., Thorne, P. W., Banzon, V. F., Boyer, T., Chepurin, G., Lawrimore, J. H., Menne, M. J., Smith, T. M., Vose, R. S., and Zhang, H.-M.: Extended Reconstructed Sea Surface Temperature, Version 5 (ERSSTv5): Upgrades, Validations, and Intercomparisons, J. Climate, 30, 8179–8205, https://doi.org/10.1175/JCLI-D-16-0836.1, 2017 (data available at: https://psl.noaa.gov/data/gridded/data.noaa.ersst.v5.html, last access: 19 May 2022).
Ionita, M., Felis, T., Lohmann, G., Rimbu, N., and Pätzold, J.: Distinct modes of East Asian Winter Monsoon documented by a southern Red Sea coral record, J. Geophys. Res.-Oceans, 119, 1517–1533, https://doi.org/10.1002/2013JC009203, 2014.
Jimenez, G., Cole, J. E., Thompson, D. M., and Tudhope, A. W.: Northern Galápagos Corals Reveal Twentieth Century Warming in the Eastern Tropical Pacific, Geophys. Res. Lett., 45, 1981–1988, https://doi.org/10.1002/2017GL075323, 2018.
Kawakubo, Y., Yokoyama, Y., Suzuki, A., Okai, T., Alibert, C., Kinsley, L., and Eggins, S.: Precise determination of by laser ablation ICP-MS compared to ICP-AES and application to multi-century temperate corals, Geochem. J., 48, 145–152, https://doi.org/10.2343/geochemj.2.0295, 2014.
Kawakubo, Y., Alibert, C., and Yokoyama, Y.: A Reconstruction of Subtropical Western North Pacific SST Variability Back to 1578, Based on a Porites Coral Record from the Northern Ryukyus, Japan, Paleoceanography, 32, 1352–1370, https://doi.org/10.1002/2017PA003203, 2017.
Kennedy, J. J., Rayner, N. A., Atkinson, C. P., and Killick, R. E.: An Ensemble Data Set of Sea Surface Temperature Change From 1850: The Met Office Hadley Centre HadSST.4.0.0.0 Data Set, J. Geophys. Res.-Atmos., 124, 7719–7763, https://doi.org/10.1029/2018JD029867, 2019.
Khider, D., Emile-Geay, J., McKay, N. P., Gil, Y., Garijo, D., Ratnakar, V., Alonso-Garcia, M., Bertrand, S., Bothe, O., Brewer, P., Bunn, A., Chevalier, M., Comas-Bru, L., Csank, A., Dassié, E., DeLong, K., Felis, T., Francus, P., Frappier, A., Gray, W., Goring, S., Jonkers, L., Kahle, M., Kaufman, D., Kehrwald, N. M., Martrat, B., McGregor, H., Richey, J., Schmittner, A., Scroxton, N., Sutherland, E., Thirumalai, K., Allen, K., Arnaud, F., Axford, Y., Barrows, T., Bazin, L., Pilaar Birch, S. E., Bradley, E., Bregy, J., Capron, E., Cartapanis, O., Chiang, H.-W., Cobb, K. M., Debret, M., Dommain, R., Du, J., Dyez, K., Emerick, S., Erb, M. P., Falster, G., Finsinger, W., Fortier, D., Gauthier, N., George, S., Grimm, E., Hertzberg, J., Hibbert, F., Hillman, A., Hobbs, W., Huber, M., Hughes, A. L. C., Jaccard, S., Ruan, J., Kienast, M., Konecky, B., Le Roux, G., Lyubchich, V., Novello, V. F., Olaka, L., Partin, J. W., Pearce, C., Phipps, S. J., Pignol, C., Piotrowska, N., Poli, M.-S., Prokopenko, A., Schwanck, F., Stepanek, C., Swann, G. E. A., Telford, R., Thomas, E., Thomas, Z., Truebe, S., von Gunten, L., Waite, A., Weitzel, N., Wilhelm, B., Williams, J., Williams, J. J., Winstrup, M., Zhao, N., and Zhou, Y.: PaCTS 1.0: A Crowdsourced Reporting Standard for Paleoclimate Data, Paleoceanography and Paleoclimatology, 34, 1570–1596, https://doi.org/10.1029/2019PA003632, 2019.
Kilbourne, K. H., Quinn, T. M., and Taylor, F. W.: A fossil coral perspective on western tropical Pacific climate ∼ 350 ka, Paleoceanography, 19, PA1019, https://doi.org/10.1029/2003PA000944, 2004a.
Kilbourne, K. H., Quinn, T. M., Taylor, F. W., Delcroix, T., and Gouriou, Y.: El Niño–Southern Oscillation–related salinity variations recorded in the skeletal geochemistry of a Porites coral from Espiritu Santo, Vanuatu, Paleoceanography, 19, PA4002, https://doi.org/10.1029/2004PA001033, 2004b.
Kilbourne, K. H., Quinn, T. M., Webb, R., Guilderson, T., Nyberg, J., and Winter, A.: Paleoclimate proxy perspective on Caribbean climate since the year 1751: Evidence of cooler temperatures and multidecadal variability, Paleoceanography, 23, PA3220, https://doi.org/10.1029/2008PA001598, 2008.
Kilbourne, K. H., Quinn, T. M., Webb, R., Guilderson, T., Nyberg, J., and Winter, A.: Coral windows onto seasonal climate variability in the northern Caribbean since 1479, Geochem. Geophy. Geosy., 11, Q10006, https://doi.org/10.1029/2010GC003171, 2010.
Kilbourne, K. H., Alexander, M. A., and Nye, J. A.: A low latitude paleoclimate perspective on Atlantic multidecadal variability, J. Marine Syst., 133, 4–13, https://doi.org/10.1016/j.jmarsys.2013.09.004, 2014.
Klein, R., Tudhope, A. W., Chilcott, C. P., Pätzold, J., Abdulkarim, Z., Fine, M., Fallick, A. E., and Loya, Y.: Evaluating southern Red Sea corals as a proxy record for the Asian monsoon, Earth Planet. Sc. Lett., 148, 381–394, https://doi.org/10.1016/S0012-821X(97)00021-6, 1997.
Konecky, B. L., McKay, N. P., Churakova (Sidorova), O. V., Comas-Bru, L., Dassié, E. P., DeLong, K. L., Falster, G. M., Fischer, M. J., Jones, M. D., Jonkers, L., Kaufman, D. S., Leduc, G., Managave, S. R., Martrat, B., Opel, T., Orsi, A. J., Partin, J. W., Sayani, H. R., Thomas, E. K., Thompson, D. M., Tyler, J. J., Abram, N. J., Atwood, A. R., Cartapanis, O., Conroy, J. L., Curran, M. A., Dee, S. G., Deininger, M., Divine, D. V., Kern, Z., Porter, T. J., Stevenson, S. L., von Gunten, L., and Iso2k Project Members: The Iso2k database: a global compilation of paleo-δ18O and δ2H records to aid understanding of Common Era climate, Earth Syst. Sci. Data, 12, 2261–2288, https://doi.org/10.5194/essd-12-2261-2020, 2020.
Konecky, B. L., McKay, N. P., Falster, G. M., Stevenson, S. L., Fischer, M. J., Atwood, A. R., Thompson, D. M., Jones, M. D., DeLong, K. L., Tyler, J. J., Martrat, B., Thomas, E. K., Conroy, J. L., Dee, S. G., Jonkers, L., Churakova (Sidorova), O. V., Kern, Z., Opel, T., Porter, T. J., Sayani, H. R., Skrzypek, G., and Iso2k Project Members: Temperature-driven changes in the global water cycle during the Common Era, in review, 2023.
Krawczyk, H., Zinke, J., Browne, N., Struck, U., McIlwain, J., O'Leary, M., and Garbe-Schönberg, D.: Corals reveal ENSO-driven synchrony of climate impacts on both terrestrial and marine ecosystems in northern Borneo, Sci. Rep.-UK, 10, 3678, https://doi.org/10.1038/s41598-020-60525-1, 2020.
Kuffner, I. B., Roberts, K. E., Flannery, J. A., Morrison, J. M., and Richey, J. N.: Fidelity of the proxy in recording ocean temperature in the western Atlantic coral Siderastrea siderea, Geochem. Geophy. Geosy., 18, 178–188, https://doi.org/10.1002/2016GC006640, 2017.
Kuhnert, H., Pätzold, J., Hatcher, B., Wyrwoll, K.-H., Eisenhauer, A., Collins, L. B., Zhu, Z. R., and Wefer, G.: A 200-year coral stable oxygen isotope record from a high-latitude reef off Western Australia, Coral Reefs, 18, 1–12, https://doi.org/10.1007/s003380050147, 1999.
Kuhnert, H., Pätzold, J., Wyrwoll, K.-H., and Wefer, G.: Monitoring climate variability over the past 116 years in coral oxygen isotopes from Ningaloo Reef, Western Australia, Int. J. Earth Sci., 88, 725–732, https://doi.org/10.1007/s005310050300, 2000.
Lawman, A. E., Quinn, T. M., Partin, J. W., Thirumalai, K., Taylor, F. W., Wu, C.-C., Yu, T.-L., Gorman, M. K., and Shen, C.-C.: A Century of Reduced ENSO Variability During the Medieval Climate Anomaly, Paleoceanography and Paleoclimatology, 35, e2019PA003742, https://doi.org/10.1029/2019PA003742, 2020a.
Lawman, A. E., Partin, J. W., Dee, S. G., Casadio, C. A., Di Nezio, P., and Quinn, T. M.: Developing a Coral Proxy System Model to Compare Coral and Climate Model Estimates of Changes in Paleo-ENSO Variability, Paleoceanography and Paleoclimatology, 35, e2019PA003836, https://doi.org/10.1029/2019PA003836, 2020b.
LeGrande, A. N. and Schmidt, G. A.: Global gridded data set of the oxygen isotopic composition in seawater, Geophys. Res. Lett., 33, L12604, https://doi.org/10.1029/2006GL026011, 2006.
LeGrande, A. N. and Schmidt, G. A.: Water isotopologues as a quantitative paleosalinity proxy, Paleoceanography, 26, PA3225, https://doi.org/10.1029/2010PA002043, 2011.
Linsley, B. K., Dunbar, R. B., Wellington, G. M., and Mucciarone, D. A.: A coral-based reconstruction of Intertropical Convergence Zone variability over Central America since 1707, J. Geophys. Res.-Oceans, 99, 9977–9994, https://doi.org/10.1029/94JC00360, 1994.
Linsley, B. K., Messier, R. G., and Dunbar, R. B.: Assessing between-colony oxygen isotope variability in the coral Porites lobata at Clipperton Atoll, Coral Reefs, 18, 13–27, https://doi.org/10.1007/s003380050148, 1999.
Linsley, B. K., Wellington, G. M., and Schrag, D. P.: Decadal Sea Surface Temperature Variability in the Subtropical South Pacific from 1726 to 1997 A.D., Science, 290, 1145–1148, https://doi.org/10.1126/science.290.5494.1145, 2000.
Linsley, B. K., Wellington, G. M., Schrag, D. P., Ren, L., Salinger, M. J., and Tudhope, A. W.: Geochemical evidence from corals for changes in the amplitude and spatial pattern of South Pacific interdecadal climate variability over the last 300 years, Clim. Dynam., 22, 1–11, https://doi.org/10.1007/s00382-003-0364-y, 2004.
Linsley, B. K., Kaplan, A., Gouriou, Y., Salinger, J., deMenocal, P. B., Wellington, G. M., and Howe, S. S.: Tracking the extent of the South Pacific Convergence Zone since the early 1600s, Geochem. Geophy. Geosy., 7, Q05003, https://doi.org/10.1029/2005GC001115, 2006.
Linsley, B. K., Zhang, P., Kaplan, A., Howe, S. S., and Wellington, G. M.: Interdecadal-decadal climate variability from multicoral oxygen isotope records in the South Pacific Convergence Zone region since 1650 A.D., Paleoceanography, 23, PA2219, https://doi.org/10.1029/2007PA001539, 2008.
Loope, G., Thompson, D., Cole, J., and Overpeck, J.: Is there a low-frequency bias in multiproxy reconstructions of tropical pacific SST variability?, Quaternary Sci. Rev., 246, 106530, https://doi.org/10.1016/j.quascirev.2020.106530, 2020.
Lough, J. M.: Climate records from corals, WIREs Clin. Change, 1, 318–331, https://doi.org/10.1002/wcc.39, 2010.
Lough, J. M. and Barnes, D. J.: Several centuries of variation in skeletal extension, density and calcification in massive Porites colonies from the Great Barrier Reef: A proxy for seawater temperature and a background of variability against which to identify unnatural change, J. Exp. Mar. Biol. Ecol., 211, 29–67, https://doi.org/10.1016/S0022-0981(96)02710-4, 1997.
Madakumbura, G. D., Thackeray, C. W., Norris, J., Goldenson, N., and Hall, A.: Anthropogenic influence on extreme precipitation over global land areas seen in multiple observational datasets, Nat. Commun., 12, 3944, https://doi.org/10.1038/s41467-021-24262-x, 2021.
Maupin, C. R., Quinn, T. M., and Halley, R. B.: Extracting a climate signal from the skeletal geochemistry of the Caribbean coral Siderastrea siderea, Geochem. Geophy. Geosy., 9, Q12012, https://doi.org/10.1029/2008GC002106, 2008.
McConnaughey, T.: 13C and 18O isotopic disequilibrium in biological carbonates: I. Patterns, Geochim. Cosmochim. Ac., 53, 151–162, https://doi.org/10.1016/0016-7037(89)90282-2, 1989.
McGregor, H. V. and Gagan, M. K.: Western Pacific coral δ18O records of anomalous Holocene variability in the El Niño–Southern Oscillation, Geophys. Res. Lett., 31, L11204, https://doi.org/10.1029/2004GL019972, 2004.
McGregor, H. V., Gagan, M. K., McCulloch, M. T., Hodge, E., and Mortimer, G.: Mid-Holocene variability in the marine 14C reservoir age for northern coastal Papua New Guinea, Quat. Geochronol., 3, 213–225, https://doi.org/10.1016/j.quageo.2007.11.002, 2008.
McGregor, H. V., Fischer, M. J., Gagan, M. K., Fink, D., and Woodroffe, C. D.: Environmental control of the oxygen isotope composition of Porites coral microatolls, Geochim. Cosmochim. Ac., 75, 3930–3944, https://doi.org/10.1016/j.gca.2011.04.017, 2011.
McGregor, H. V., Evans, M. N., Goosse, H., Leduc, G., Martrat, B., Addison, J. A., Mortyn, P. G., Oppo, D. W., Seidenkrantz, M.-S., Sicre, M.-A., Phipps, S. J., Selvaraj, K., Thirumalai, K., Filipsson, H. L., and Ersek, V.: Robust global ocean cooling trend for the pre-industrial Common Era, Nat. Geosci., 8, 671–677, https://doi.org/10.1038/ngeo2510, 2015.
McKay, N. P. and Emile-Geay, J.: Technical note: The Linked Paleo Data framework – a common tongue for paleoclimatology, Clim. Past, 12, 1093–1100, https://doi.org/10.5194/cp-12-1093-2016, 2016.
McPhaden, M. J., Busalacchi, A. J., Cheney, R., Donguy, J.-R., Gage, K. S., Halpern, D., Ji, M., Julian, P., Meyers, G., Mitchum, G. T., Niiler, P. P., Picaut, J., Reynolds, R. W., Smith, N., and Takeuchi, K.: The Tropical Ocean-Global Atmosphere observing system: A decade of progress, J. Geophys. Res.-Oceans, 103, 14169–14240, https://doi.org/10.1029/97JC02906, 1998.
McPhaden, M. J., Busalacchi, A. J., and Anderson, D. L. T.: A TOGA Retrospective, Oceanography, 23, 86–103, 2010.
Mohtar, A. T., Hughen, K. A., Goodkin, N. F., Streanga, I.-M., Ramos, R. D., Samanta, D., Cervino, J., and Switzer, A. D.: Coral-based proxy calibrations constrain ENSO-driven sea surface temperature and salinity gradients in the Western Pacific Warm Pool, Palaeogeogr. Palaeocl., 561, 110037, https://doi.org/10.1016/j.palaeo.2020.110037, 2021.
Moses, C. S., Swart, P. K., and Rosenheim, B. E.: Evidence of multidecadal salinity variability in the eastern tropical North Atlantic, Paleoceanography, 21, PA3010, https://doi.org/10.1029/2005PA001257, 2006.
Murty, S. A., Goodkin, N. F., Halide, H., Natawidjaja, D., Suwargadi, B., Suprihanto, I., Prayudi, D., Switzer, A. D., and Gordon, A. L.: Climatic Influences on Southern Makassar Strait Salinity Over the Past Century, Geophys. Res. Lett., 44, 11967–11975, https://doi.org/10.1002/2017GL075504, 2017.
Murty, S. A., Bernstein, W. N., Ossolinski, J. E., Davis, R. S., Goodkin, N. F., and Hughen, K. A.: Spatial and Temporal Robustness of -SST Calibrations in Red Sea Corals: Evidence for Influence of Mean Annual Temperature on Calibration Slopes, Paleoceanography and Paleoclimatology, 33, 443–456, https://doi.org/10.1029/2017PA003276, 2018a.
Murty, S. A., Goodkin, N. F., Wiguna, A. A., and Gordon, A. L.: Variability in Coral-Reconstructed Sea Surface Salinity Between the Northern and Southern Lombok Strait Linked to East Asian Winter Monsoon Mean State Reversals, Paleoceanography and Paleoclimatology, 33, 1116–1133, https://doi.org/10.1029/2018PA003387, 2018b.
Nakamura, N., Kayanne, H., Iijima, H., McClanahan, T. R., Behera, S. K., and Yamagata, T.: Mode shift in the Indian Ocean climate under global warming stress, Geophys. Res. Lett., 36, L23708, https://doi.org/10.1029/2009GL040590, 2009.
Neukom, R., Steiger, N., Gómez-Navarro, J. J., Wang, J., and Werner, J. P.: No evidence for globally coherent warm and cold periods over the preindustrial Common Era, Nature, 571, 550–554, https://doi.org/10.1038/s41586-019-1401-2, 2019.
Nurhati, I. S., Cobb, K. M., Charles, C. D., and Dunbar, R. B.: Late 20th century warming and freshening in the central tropical Pacific, Geophys. Res. Lett., 36, L21606, https://doi.org/10.1029/2009GL040270, 2009.
Nurhati, I. S., Cobb, K. M., and Lorenzo, E. D.: Decadal-Scale SST and Salinity Variations in the Central Tropical Pacific: Signatures of Natural and Anthropogenic Climate Change, J. Climate, 24, 3294–3308, https://doi.org/10.1175/2011JCLI3852.1, 2011.
Okai, T., Suzuki, A., Kawahata, H., Terashima, S., and Imai, N.: Preparation of a New Geological Survey of Japan Geochemical Reference Material: Coral JCp-1, Geostandard Newslett., 26, 95–99, https://doi.org/10.1111/j.1751-908X.2002.tb00627.x, 2002.
Osborne, M. C., Dunbar, R. B., Mucciarone, D. A., Sanchez-Cabeza, J.-A., and Druffel, E.: Regional calibration of coral-based climate reconstructions from Palau, West Pacific Warm Pool (WPWP), Palaeogeogr. Palaeocl., 386, 308–320, https://doi.org/10.1016/j.palaeo.2013.06.001, 2013.
Osborne, M. C., Dunbar, R. B., Mucciarone, D. A., Druffel, E., and Sanchez-Cabeza, J.-A.: A 215-yr coral δ18O time series from Palau records dynamics of the West Pacific Warm Pool following the end of the Little Ice Age, Coral Reefs, 33, 719–731, https://doi.org/10.1007/s00338-014-1146-1, 2014.
PAGES 2k Consortium: Continental-scale temperature variability during the past two millennia, Nat. Geosci., 6, 339–346, https://doi.org/10.1038/ngeo1797, 2013.
PAGES2k Consortium: A global multiproxy database for temperature reconstructions of the Common Era, Scientific Data, 4, 170088, https://doi.org/10.1038/sdata.2017.88, 2017.
PAGES 2k Consortium: Consistent multidecadal variability in global temperature reconstructions and simulations over the Common Era, Nat. Geosci., 12, 643–649, https://doi.org/10.1038/s41561-019-0400-0, 2019.
PAGES 2k Network Coordinators: Understanding the climate of the past 2000 years: Phase 3 of the PAGES 2k Network, PAGES Magazine, 25, 110, https://doi.org/10.22498/pages.25.2.110, 2017.
PAGES Hydro2k Consortium: Comparing proxy and model estimates of hydroclimate variability and change over the Common Era, Clim. Past, 13, 1851–1900, https://doi.org/10.5194/cp-13-1851-2017, 2017.
Pawlowicz, R.: M_Map: A mapping package for MATLAB, version 1.4m, EOAS [software], https://www.eoas.ubc.ca/~rich/map.html (last access: 19 May 2022), 2020.
Pfeiffer, M., Timm, O., Dullo, W.-C., and Podlech, S.: Oceanic forcing of interannual and multidecadal climate variability in the southwestern Indian Ocean: Evidence from a 160 year coral isotopic record (La Réunion, 55∘ E, 21∘ S), Paleoceanography, 19, PA4006, https://doi.org/10.1029/2003PA000964, 2004a.
Pfeiffer, M., Dullo, W.-C., and Eisenhauer, A.: Variability of the Intertropical Convergence Zone recorded in coral isotopic records from the central indian Ocean (Chagos Archipelago), Quaternary Res., 61, 245–255, https://doi.org/10.1016/j.yqres.2004.02.009, 2004b.
Pfeiffer, M., Reuning, L., Zinke, J., Garbe-Schönberg, D., Leupold, M., and Dullo, W.-C.: 20th Century δ18O Seawater and Salinity Variations Reconstructed From Paired δ18O and Measurements of a La Reunion Coral, Paleoceanography and Paleoclimatology, 34, 2183–2200, https://doi.org/10.1029/2019PA003770, 2019.
Power, S., Delage, F., Chung, C., Kociuba, G., and Keay, K.: Robust twenty-first-century projections of El Niño and related precipitation variability, Nature, 502, 541–545, https://doi.org/10.1038/nature12580, 2013.
Qu, T., Song, Y. T., and Maes, C.: Sea surface salinity and barrier layer variability in the equatorial Pacific as seen from Aquarius and Argo, J. Geophys. Res.-Oceans, 119, 15–29, https://doi.org/10.1002/2013JC009375, 2014.
Quinn, T. M., Taylor, F. W., and Crowley, T. J.: A 173 year stable isotope record from a tropical south pacific coral, Quaternary Sci. Rev., 12, 407–418, https://doi.org/10.1016/S0277-3791(05)80005-8, 1993.
Quinn, T. M., Crowley, T. J., and Taylor, F. W.: New stable isotope results from a 173-year coral from Espiritu Santo, Vanuatu, Geophys. Res. Lett., 23, 3413–3416, https://doi.org/10.1029/96GL03169, 1996.
Quinn, T. M., Taylor, F. W., and Crowley, T. J.: Coral-based climate variability in the Western Pacific Warm Pool since 1867, J. Geophys. Res.-Oceans, 111, C11006, https://doi.org/10.1029/2005JC003243, 2006.
Ramos, R. D., Goodkin, N. F., Siringan, F. P., and Hughen, K. A.: Coral Records of Temperature and Salinity in the Tropical Western Pacific Reveal Influence of the Pacific Decadal Oscillation Since the Late Nineteenth Century, Paleoceanography and Paleoclimatology, 34, 1344–1358, https://doi.org/10.1029/2019PA003684, 2019.
Ramos, R. D., Goodkin, N. F., and Fan, T.-Y.: Coral Records at the Northern Edge of the Western Pacific Warm Pool Reveal Multiple Drivers of Sea Surface Temperature, Salinity, and Rainfall Variability Since the End of the Little Ice Age, Paleoceanography and Paleoclimatology, 35, e2019PA003826, https://doi.org/10.1029/2019PA003826, 2020.
Rayner, N. A., Parker, D. E., Horton, E. B., Folland, C. K., Alexander, L. V., Rowell, D. P., Kent, E. C., and Kaplan, A.: Global analyses of sea surface temperature, sea ice, and night marine air temperature since the late nineteenth century, J. Geophys. Res.-Atmos., 108, 4407, https://doi.org/10.1029/2002JD002670, 2003.
Reed, E. V., Cole, J. E., Lough, J. M., Thompson, D., and Cantin, N. E.: Linking climate variability and growth in coral skeletal records from the Great Barrier Reef, Coral Reefs, 38, 29–43, https://doi.org/10.1007/s00338-018-01755-8, 2019.
Reed, E. V., Thompson, D. M., Cole, J. E., Lough, J. M., Cantin, N. E., Cheung, A. H., Tudhope, A., Vetter, L., Jimenez, G., and Edwards, R. L.: Impacts of Coral Growth on Geochemistry: Lessons From the Galápagos Islands, Paleoceanography and Paleoclimatology, 36, e2020PA004051, https://doi.org/10.1029/2020PA004051, 2021.
Ren, L., Linsley, B. K., Wellington, G. M., Schrag, D. P., and Hoegh-guldberg, O.: Deconvolving the δ18O seawater component from subseasonal coral δ18O and at Rarotonga in the southwestern subtropical Pacific for the period 1726 to 1997, Geochim. Cosmochim. Ac., 67, 1609–1621, https://doi.org/10.1016/S0016-7037(02)00917-1, 2003.
Reynolds, R. W., Rayner, N. A., Smith, T. M., Stokes, D. C., and Wang, W.: An Improved In Situ and Satellite SST Analysis for Climate, J. Climate, 15, 1609–1625, https://doi.org/10.1175/1520-0442(2002)015<1609:AIISAS>2.0.CO;2, 2002.
Rixen, T., Ramachandran, P., Lehnhoff, L., Dasbach, D., Gaye, B., Urban, B., Ramachandran, R., and Ittekkot, V.: Impact of monsoon-driven surface ocean processes on a coral off Port Blair on the Andaman Islands and their link to North Atlantic climate variations, Global Planet. Change, 75, 1–13, https://doi.org/10.1016/j.gloplacha.2010.09.005, 2011.
Rodriguez, L. G., Cohen, A. L., Ramirez, W., Oppo, D. W., Pourmand, A., Edwards, R. L., Alpert, A. E., and Mollica, N.: Mid-Holocene, Coral-Based Sea Surface Temperatures in the Western Tropical Atlantic, Paleoceanography and Paleoclimatology, 34, 1234–1245, https://doi.org/10.1029/2019PA003571, 2019.
Ropelewski, C. F. and Halpert, M. S.: Global and Regional Scale Precipitation Patterns Associated with the El Niño/Southern Oscillation, Mon. Weather Rev., 115, 1606–1626, https://doi.org/10.1175/1520-0493(1987)115<1606:GARSPP>2.0.CO;2, 1987.
Saha, N., Rodriguez-Ramirez, A., Nguyen, A. D., Clark, T. R., Zhao, J., and Webb, G. E.: Seasonal to decadal scale influence of environmental drivers on Ba Ca and Y Ca in coral aragonite from the southern Great Barrier Reef, Sci. Total Environ., 639, 1099–1109, https://doi.org/10.1016/j.scitotenv.2018.05.156, 2018.
Saha, N., Webb, G. E., Zhao, J.-X., Lewis, S. E., Duc Nguyen, A., and Feng, Y.: Spatiotemporal variation of rare earth elements from river to reef continuum aids monitoring of terrigenous sources in the Great Barrier Reef, Geochim. Cosmochim. Ac., 299, 85–112, https://doi.org/10.1016/j.gca.2021.02.014, 2021.
Saji, N. H., Goswami, B. N., Vinayachandran, P. N., and Yamagata, T.: A dipole mode in the tropical Indian Ocean, Nature, 401, 360–363, https://doi.org/10.1038/43854, 1999.
Sanchez, S. C., Charles, C. D., Carriquiry, J. D., and Villaescusa, J. A.: Two centuries of coherent decadal climate variability across the Pacific North American region, Geophys. Res. Lett., 43, 9208–9216, https://doi.org/10.1002/2016GL069037, 2016.
Sanchez, S. C., Westphal, N., Haug, G. H., Cheng, H., Edwards, R. L., Schneider, T., Cobb, K. M., and Charles, C. D.: A Continuous Record of Central Tropical Pacific Climate Since the Midnineteenth Century Reconstructed From Fanning and Palmyra Island Corals: A Case Study in Coral Data Reanalysis, Paleoceanography and Paleoclimatology, 35, e2020PA003848, https://doi.org/10.1029/2020PA003848, 2020.
Sanchez, S. C., Hakim, G. J., and Saenger, C. P.: Climate Model Teleconnection Patterns Govern the Niño-3.4 Response to Early Nineteenth-Century Volcanism in Coral-Based Data Assimilation Reconstructions, J. Climate, 34, 1863–1880, https://doi.org/10.1175/JCLI-D-20-0549.1, 2021.
Sayani, H. R., Cobb, K. M., DeLong, K., Hitt, N. T., and Druffel, E. R. M.: Intercolony δ18O and variability among Porites spp. corals at Palmyra Atoll: Toward more robust coral-based estimates of climate, Geochem. Geophy. Geosy., 20, 5270–5284, https://doi.org/10.1029/2019GC008420, 2019.
Sayani, H. R., Thompson, D. M., Carilli, J. E., Marchitto, T. M., Chapman, A. U., and Cobb, K. M.: Reproducibility of Coral Mn/Ca-Based Wind Reconstructions at Kiritimati Island and Butaritari Atoll, Geochem. Geophy. Geosy., 22, e2020GC009398, https://doi.org/10.1029/2020GC009398, 2021.
Schmid, C., Molinari, R. L., Sabina, R., Daneshzadeh, Y.-H., Xia, X., Forteza, E., and Yang, H.: The Real-Time Data Management System for Argo Profiling Float Observations, J. Atmos. Ocean. Tech., 24, 1608–1628, https://doi.org/10.1175/JTECH2070.1, 2007.
Schrag, D. P.: Rapid analysis of high-precision ratios in corals and other marine carbonates, Paleoceanography, 14, 97–102, https://doi.org/10.1029/1998PA900025, 1999.
Shen, G. T., Cole, J. E., Lea, D. W., Linn, L. J., McConnaughey, T. A., and Fairbanks, R. G.: Surface ocean variability at Galapagos from 1936–1982: Calibration of geochemical tracers in corals, Paleoceanography, 7, 563–588, https://doi.org/10.1029/92PA01825, 1992.
Smith, J. M., Quinn, T. M., Helmle, K. P., and Halley, R. B.: Reproducibility of geochemical and climatic signals in the Atlantic coral Montastraea faveolata, Paleoceanography, 21, PA1010, https://doi.org/10.1029/2005PA001187, 2006.
Smith, S. V., Buddemeier, R. W., Redalje, R. C., and Houck, J. E.: Strontium-Calcium Thermometry in Coral Skeletons, Science, 204, 404–407, https://doi.org/10.1126/science.204.4391.404, 1979.
Song, F., Leung, L. R., Lu, J., Dong, L., Zhou, W., Harrop, B., and Qian, Y.: Emergence of seasonal delay of tropical rainfall during 1979–2019, Nat. Clim. Change, 11, 605–612, https://doi.org/10.1038/s41558-021-01066-x, 2021.
Steiger, N. J., Smerdon, J. E., Cook, E. R., and Cook, B. I.: A reconstruction of global hydroclimate and dynamical variables over the Common Era, Scientific Data, 5, 180086, https://doi.org/10.1038/sdata.2018.86, 2018.
Storz, D., Gischler, E., Fiebig, J., Eisenhauer, A., and Garbe-Schönberg, D.: Evaluation of oxygen isotope and ratios from a Maldivian scleractinian coral for reconstruction of climate variability in the northwestern Indian Ocean, PALAIOS, 28, 42–55, https://doi.org/10.2110/palo.2012.p12-034r, 2013.
Swart, P. K., Healy, G. F., Dodge, R. E., Kramer, P., Hudson, J. H., Halley, R. B., and Robblee, M. B.: The stable oxygen and carbon isotopic record from a coral growing in Florida Bay: a 160 year record of climatic and anthropogenic influence, Palaeogeogr. Palaeocl., 123, 219–237, https://doi.org/10.1016/0031-0182(95)00078-X, 1996.
Swart, P. K., White, K. S., Enfield, D., Dodge, R. E., and Milne, P.: Stable oxygen isotopic composition of corals from the Gulf of Guinea as indicators of periods of extreme precipitation conditions in the sub-Sahara, J. Geophys. Res.-Oceans, 103, 27885–27891, https://doi.org/10.1029/98JC02404, 1998.
Swart, P. K., Healy, G., Greer, L., Lutz, M., Saied, A., Anderegg, D., Dodge, R. E., and Rudnick, D.: The use of proxy chemical records in Coral skeletons to ascertain past environmental conditions in Florida Bay, Estuaries, 22, 384–397, https://doi.org/10.2307/1353206, 1999.
Tangri, N., Dunbar, R. B., Linsley, B. K., and Mucciarone, D. M.: ENSO's Shrinking Twentieth-Century Footprint Revealed in a Half-Millennium Coral Core From the South Pacific Convergence Zone, Paleoceanography and Paleoclimatology, 33, 1136–1150, https://doi.org/10.1029/2017PA003310, 2018.
Tardif, R., Hakim, G. J., Perkins, W. A., Horlick, K. A., Erb, M. P., Emile-Geay, J., Anderson, D. M., Steig, E. J., and Noone, D.: Last Millennium Reanalysis with an expanded proxy database and seasonal proxy modeling, Clim. Past, 15, 1251–1273, https://doi.org/10.5194/cp-15-1251-2019, 2019.
Thompson, D. M., Ault, T. R., Evans, M. N., Cole, J. E., and Emile-Geay, J.: Comparison of observed and simulated tropical climate trends using a forward model of coral δ18O, Geophys. Res. Lett., 38, L14706, https://doi.org/10.1029/2011GL048224, 2011.
Tierney, J. E., Abram, N. J., Anchukaitis, K. J., Evans, M. N., Giry, C., Kilbourne, K. H., Saenger, C. P., Wu, H. C., and Zinke, J.: Tropical sea surface temperatures for the past four centuries reconstructed from coral archives, Paleoceanography, 30, 226–252, https://doi.org/10.1002/2014PA002717, 2015.
Tudhope, A. W., Shimmield, G. B., Chilcott, C. P., Jebb, M., Fallick, A. E., and Dalgleish, A. N.: Recent changes in climate in the far western equatorial Pacific and their relationship to the Southern Oscillation; oxygen isotope records from massive corals, Papua New Guinea, Earth Planet. Sc. Lett., 136, 575–590, https://doi.org/10.1016/0012-821X(95)00156-7, 1995.
Tudhope, A. W., Chilcott, C. P., McCulloch, M. T., Cook, E. R., Chappell, J., Ellam, R. M., Lea, D. W., Lough, J. M., and Shimmield, G. B.: Variability in the El Niño-Southern Oscillation Through a Glacial-Interglacial Cycle, Science, 291, 1511–1517, https://doi.org/10.1126/science.1057969, 2001.
Ummenhofer, C. C., Murty, S. A., Sprintall, J., Lee, T., and Abram, N. J.: Heat and freshwater changes in the Indian Ocean region, Nat. Rev. Earth Environ., 2, 525–541, https://doi.org/10.1038/s43017-021-00192-6, 2021.
Urban, F. E., Cole, J. E., and Overpeck, J. T.: Influence of mean climate change on climate variability from a 155-year tropical Pacific coral record, Nature, 407, 989–993, https://doi.org/10.1038/35039597, 2000.
Vásquez-Bedoya, L. F., Cohen, A. L., Oppo, D. W., and Blanchon, P.: Corals record persistent multidecadal SST variability in the Atlantic Warm Pool since 1775 AD, Paleoceanography, 27, PA3231, https://doi.org/10.1029/2012PA002313, 2012.
Vazquez-Cuervo, J. and Gomez-Valdes, J.: SMAP and CalCOFI Observe Freshening during the 2014–2016 Northeast Pacific Warm Anomaly, Remote Sens., 10, 1716, https://doi.org/10.3390/rs10111716, 2018.
von Reumont, J., Hetzinger, S., Garbe-Schönberg, D., Manfrino, C., and Dullo, W.-Chr.: Impact of warming events on reef-scale temperature variability as captured in two Little Cayman coral records, Geochem. Geophy. Geosy., 17, 846–857, https://doi.org/10.1002/2015GC006194, 2016.
von Reumont, J., Hetzinger, S., Garbe-Schönberg, D., Manfrino, C., and Dullo, C.: Tracking Interannual- to Multidecadal-Scale Climate Variability in the Atlantic Warm Pool Using Central Caribbean Coral Data, Paleoceanography and Paleoclimatology, 33, 395–411, https://doi.org/10.1002/2018PA003321, 2018.
Walter, R. M., Sayani, H. R., Felis, T., Cobb, K. M., Abram, N. J., Arzey, A. K., Atwood, A., Brenner, L. D., Dassié, E. P., DeLong, K. L., Ellis, B., Fischer, M. J., Goodkin, N. F., Hargreaves, J. A., Kilbourne, K. H., Krawczyk, H. A., McKay, N. P., Murty, S. A., Ramos, R. D., Reed, E. V., Samanta, D., Sanchez, S. C., Zinke, J., and PAGES CoralHydro2k Project Members: NOAA/WDS Paleoclimatology – CoralHydro2k Database (Common Era coral δ18O and data compilation), NOAA National Centers for Environmental Information [data set], https://doi.org/10.25921/yp94-v135, 2022.
Watanabe, T., Winter, A., and Oba, T.: Seasonal changes in sea surface temperature and salinity during the Little Ice Age in the Caribbean Sea deduced from Mg Ca and 18O 16O ratios in corals, Mar. Geol., 173, 21–35, https://doi.org/10.1016/S0025-3227(00)00166-3, 2001.
Watanabe, T. K., Watanabe, T., Yamazaki, A., Pfeiffer, M., Garbe-Schönberg, D., and Claereboudt, M. R.: Past summer upwelling events in the Gulf of Oman derived from a coral geochemical record, Sci. Rep.-UK, 7, 4568, https://doi.org/10.1038/s41598-017-04865-5, 2017.
Weber, J. N.: Incorporation of strontium into reef coral skeletal carbonate, Geochim. Cosmochim. Ac., 37, 2173–2190, https://doi.org/10.1016/0016-7037(73)90015-X, 1973.
Weber, J. N. and Woodhead, P. M. J.: Temperature dependence of oxygen-18 concentration in reef coral carbonates, J. Geophys. Res., 77, 463–473, https://doi.org/10.1029/JC077i003p00463, 1972.
Webster, P. J., Moore, A. M., Loschnigg, J. P., and Leben, R. R.: Coupled ocean–atmosphere dynamics in the Indian Ocean during 1997–98, Nature, 401, 356–360, https://doi.org/10.1038/43848, 1999.
Weerabaddana, M. M., DeLong, K. L., Wagner, A. J., Loke, D. W. Y., Kilbourne, K. H., Slowey, N., Hu, H.-M., and Shen, C.-C.: Insights from barium variability in a Siderastrea siderea coral in the northwestern Gulf of Mexico, Mar. Pollut. Bull., 173, 112930, https://doi.org/10.1016/j.marpolbul.2021.112930, 2021.
Wei, G., McCulloch, M. T., Mortimer, G., Deng, W., and Xie, L.: Evidence for ocean acidification in the Great Barrier Reef of Australia, Geochim. Cosmochim. Ac., 73, 2332–2346, https://doi.org/10.1016/j.gca.2009.02.009, 2009.
Weinzierl, M. S., Reich, C. D., Hickey, T. D., Bartlett, L. A., and Kuffner, I. B.: Collection methods and descriptions of coral cores extracted from massive corals in Dry Tortugas National Park, Florida, U.S.A., Open-File Report, U.S. Geological Survey, https://doi.org/10.3133/ofr20161182, 2016.
Wilkinson, M. D., Dumontier, M., Aalbersberg, I. J., Appleton, G., Axton, M., Baak, A., Blomberg, N., Boiten, J.-W., da Silva Santos, L. B., Bourne, P. E., Bouwman, J., Brookes, A. J., Clark, T., Crosas, M., Dillo, I., Dumon, O., Edmunds, S., Evelo, C. T., Finkers, R., Gonzalez-Beltran, A., Gray, A. J. G., Groth, P., Goble, C., Grethe, J. S., Heringa, J., 't Hoen, P. A. C., Hooft, R., Kuhn, T., Kok, R., Kok, J., Lusher, S. J., Martone, M. E., Mons, A., Packer, A. L., Persson, B., Rocca-Serra, P., Roos, M., van Schaik, R., Sansone, S.-A., Schultes, E., Sengstag, T., Slater, T., Strawn, G., Swertz, M. A., Thompson, M., van der Lei, J., van Mulligen, E., Velterop, J., Waagmeester, A., Wittenburg, P., Wolstencroft, K., Zhao, J., and Mons, B.: The FAIR Guiding Principles for scientific data management and stewardship, Scientific Data, 3, 160018, https://doi.org/10.1038/sdata.2016.18, 2016.
Wu, H. C., Linsley, B. K., Dassié, E. P., Schiraldi, B., and deMenocal, P. B.: Oceanographic variability in the South Pacific Convergence Zone region over the last 210 years from multi-site coral records, Geochem. Geophy. Geosy., 14, 1435–1453, https://doi.org/10.1029/2012GC004293, 2013.
Wu, H. C., Moreau, M., Linsley, B. K., Schrag, D. P., and Corrège, T.: Investigation of sea surface temperature changes from replicated coral variations in the eastern equatorial Pacific (Clipperton Atoll) since 1874, Palaeogeogr. Palaeocl., 412, 208–222, https://doi.org/10.1016/j.palaeo.2014.07.039, 2014.
Wu, H. C., Felis, T., Scholz, D., Giry, C., Kölling, M., Jochum, K. P., and Scheffers, S. R.: Changes to Yucatán Peninsula precipitation associated with salinity and temperature extremes of the Caribbean Sea during the Maya civilization collapse, Sci. Rep.-UK, 7, 15825, https://doi.org/10.1038/s41598-017-15942-0, 2017.
Xiao, H., Deng, W., Chen, X., Wei, G., Zeng, T., and Zhao, J.: Wet and cold climate conditions recorded by coral geochemical proxies during the beginning of the first millennium CE in the northern South China Sea, J. Asian Earth Sci., 135, 25–34, https://doi.org/10.1016/j.jseaes.2016.12.012, 2017.
Xu, Y.-Y., Pearson, S., and Halimeda Kilbourne, K.: Assessing coral –SST calibration techniques using the species Diploria strigosa, Palaeogeogr. Palaeocl., 440, 353–362, https://doi.org/10.1016/j.palaeo.2015.09.016, 2015.
Zhang, R., Sutton, R., Danabasoglu, G., Kwon, Y.-O., Marsh, R., Yeager, S. G., Amrhein, D. E., and Little, C. M.: A Review of the Role of the Atlantic Meridional Overturning Circulation in Atlantic Multidecadal Variability and Associated Climate Impacts, Rev. Geophys., 57, 316–375, https://doi.org/10.1029/2019RG000644, 2019.
Zinke, J., Dullo, W.-Chr., Heiss, G. A., and Eisenhauer, A.: ENSO and Indian Ocean subtropical dipole variability is recorded in a coral record off southwest Madagascar for the period 1659 to 1995, Earth Planet. Sc. Lett., 228, 177–194, https://doi.org/10.1016/j.epsl.2004.09.028, 2004.
Zinke, J., Pfeiffer, M., Timm, O., Dullo, W.-C., Kroon, D., and Thomassin, B. A.: Mayotte coral reveals hydrological changes in the western Indian Ocean between 1881 and 1994, Geophys. Res. Lett., 35, L23707, https://doi.org/10.1029/2008GL035634, 2008.
Zinke, J., Rountrey, A., Feng, M., Xie, S.-P., Dissard, D., Rankenburg, K., Lough, J. M., and McCulloch, M. T.: Corals record long-term Leeuwin current variability including Ningaloo Niño/Niña since 1795, Nat. Commun., 5, 3607, https://doi.org/10.1038/ncomms4607, 2014a.
Zinke, J., Loveday, B. R., Reason, C. J. C., Dullo, W.-C., and Kroon, D.: Madagascar corals track sea surface temperature variability in the Agulhas Current core region over the past 334 years, Sci. Rep.-UK, 4, 4393, https://doi.org/10.1038/srep04393, 2014b.
Zinke, J., Hoell, A., Lough, J. M., Feng, M., Kuret, A. J., Clarke, H., Ricca, V., Rankenburg, K., and McCulloch, M. T.: Coral record of southeast Indian Ocean marine heatwaves with intensified Western Pacific temperature gradient, Nat. Commun., 6, 8562, https://doi.org/10.1038/ncomms9562, 2015.
Zinke, J., Reuning, L., Pfeiffer, M., Wassenburg, J. A., Hardman, E., Jhangeer-Khan, R., Davies, G. R., Ng, C. K. C., and Kroon, D.: A sea surface temperature reconstruction for the southern Indian Ocean trade wind belt from corals in Rodrigues Island (19∘ S, 63∘ E), Biogeosciences, 13, 5827–5847, https://doi.org/10.5194/bg-13-5827-2016, 2016.
Zweng, M. M., Reagan, J. R., Seidov, D., Boyer, T. P., Locarnini, R. A., Garcia, H. E., Mishonov, A. V., Baranova, O. K., Weathers, K. W., Paver, C. R., and Smolyar, I. V.: World Ocean Atlas 2018, Volume 2: Salinity, edited by: Mishonov, A. V., NOAA Atlas NESDIS 82, https://www.ncei.noaa.gov/sites/default/files/2020-04/woa18_vol2.pdf (last access: 19 May 2022), 2019.