the Creative Commons Attribution 4.0 License.
the Creative Commons Attribution 4.0 License.
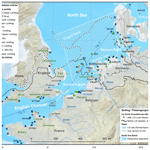
Last Interglacial sea-level data points from Northwest Europe
Víctor Cartelle
Robert Barnett
Freek S. Busschers
Natasha L. M. Barlow
Abundant numbers of sites and studies exist in NW Europe that document the geographically and geomorphologically diverse coastal record from the Last Interglacial (Eemian, Ipswichian, Marine Isotope Stage 5e). This paper summarises a database of 146 known Last Interglacial sea-level data points from in and around the North Sea (35 entries in the Netherlands, 10 Belgium, 23 in Germany, 17 in Denmark, 9 in Britain) and the English Channel (24 entries for the British and 25 for the French side, 3 on the Channel Isles) believed to be a representative and fairly complete inventory and assessment from ∼80 published sites. The geographic distribution (∼1500 km SW–NE) across the near field of the Scandinavian and British ice sheets and the attention paid to relative and numeric age control are assets of the NW European database. The research history of Last Interglacial coastal environments and sea level for this area is long, methodically diverse and spread through regional literature in several languages. Our review and database compilation effort drew from the original regional literature and paid particular attention to distinguishing between sea-level index points (SLIPs) and marine and terrestrial limiting points. We also incorporated an updated quantification of background rates of basin subsidence for the central and eastern North Sea region, utilising revised mapping of the base Quaternary, to correct for significant basin subsidence in this depocentre. As a result of subsidence, lagoonal and estuarine Last Interglacial shorelines of the Netherlands and the German Bight are preserved below the surface. In contrast, Last Interglacial shorelines along the English Channel are encountered above modern sea level.
This paper describes the dominant sea-level indicators from the region compliant with the WALIS database structure and referenced to original data sources (https://doi.org/10.5281/zenodo.6478094, Cohen et al., 2021). The sea-level proxies are mostly obtained from locations with good lithostratigraphic, morphostratigraphic and biostratigraphical constraints. Most continental European sites have chronostratigraphic age control, notably through regional pollen association zones with duration estimates. In all regions, many SLIPs and limiting points have further independent age control from luminescence, uranium series, amino acid racemisation and electron spin resonance dating techniques. Main foreseen usage of this database for the near-field region of the European ice sheets is in glacial isostatic adjustment modelling and fingerprinting Last Interglacial ice sheet melt.
- Article
(12790 KB) - Full-text XML
- BibTeX
- EndNote
Near-field records of Last Interglacial (LIG; ∼129–116 ka; within Marine Isotope Stage (MIS) 5e) sea level are critical for establishing improved reconstructions of past ice sheets, constraining models of solid Earth processes and fingerprinting the source of ice sheet melt (Dutton et al., 2015; Long et al., 2015). However, the near-field LIG sea level has received comparatively little attention compared to the far field due to the challenges of dating estuarine sequences prior to radiocarbon chronologies and the complications of regional glacial isostatic adjustment (GIA). The main aim of this paper is to describe a standardised database of geological sea-level proxies, compiled using the tools available through the World Atlas of Last Interglacial Shorelines (WALIS) project for Northwest (NW) Europe, in particular around the North Sea and English Channel region. This is a location that is near an extensive MIS-6 Eurasian ice sheet (e.g. Svendsen et al., 2004; Ehlers and Gibbard, 2004; Lambeck et al., 2006; Lang et al., 2018) and has an extensive history of palaeoenvironmental research (e.g. Dixon, 1850; Harting, 1874; Van Leeuwen et al., 2000).
The dataset documented in this paper comes from several countries and physiographically diverse lengths of LIG coastline, including tectonic depocentre areas with significant background basin subsidence (North Sea basin). The research history in this region is long, going back to the 19th century, when marine molluscan biostratigraphic evidence first identified the buried Last Interglacial equivalent of the North Sea (Harting, 1874; Lorié, 1906; Nordmann, 1928) and former shorelines along the south coast of England (Dixon, 1850; Reid, 1892, 1893, 1898). Many of the sites documented here were studied 50+ years ago by experts in microfossil and sedimentological analysis rather than with a focus on establishing sea-level index points (SLIPs) under now well-developed frameworks (Shennan, 1982; van de Plassche, 1986; Hijma et al., 2015; Rovere et al., 2016), meaning that data considered essential for modern sea-level databases (e.g. elevation surveys) are missing in some instances. Age constraints are also a challenge. In NW Europe, age control on LIG estuarine deposits typically relies on relative dating, based on micro- and macrofossil biostratigraphy (notably pollen; Zagwijn, 1961, 1996), and amino acid racemisation (AAR) methods. Although the biostratigraphic schemes allow us to resolve relative ages within the interglacial (i.e. early, middle and late), tying them to an absolute chronology has to rely on chronostratigraphical correlations to other parts of Europe and the North Atlantic. More recently studied or revisited sites may have luminescence-derived or U-series-derived numeric age constraints, which improves the dating independence.
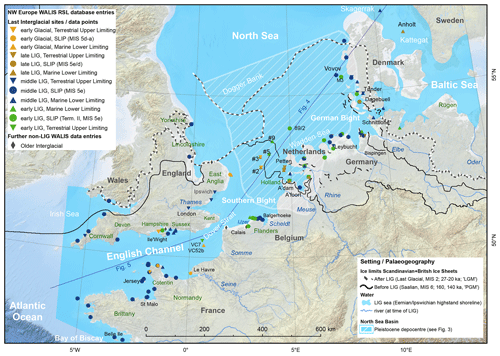
Figure 1Overview of study area (Northwest Europe) with data points (legend groups; cf. Sect. 2) showing location of all data points entered into WALIS as part of this work. Penultimate (Saalian, MIS 6, PGM) and Last Glacial maximum (LGM) ice limits compiled from regional studies (Ehlers et al., 2004; Busschers et al., 2008; Moreau et al., 2012; Lang et al., 2018; Gibbard et al., 2018; Cartelle et al., 2021) and adjoining super-regional overviews (Ehlers and Gibbard, 2004; Batchelor et al., 2019). Pleistocene depocentre in the North Sea further detailed in Sect. 3.3. Axis through North Sea and English Channel informs the presentation of data in Figs. 4 and 5. Selection of topographic names from text included, offshore sites with informal short IDs from source papers. Bathymetry and DEM backdrop: WMS service of EMODnet Bathymetry Consortium (2020), which incorporates land data © OpenStreetMap contributors 2020. Distributed under the Open Data Commons Open Database License (ODbL) v1.0.
The thickness and nature of the LIG coastal geomorphology and sedimentary sequences in NW Europe vary considerably. Differences in geological–geomorphological settings along and between the large stretches of coast have influenced the depth of preservation, surveying and mapping strategies and the taphonomy characteristics of typical sites. In the southern North Sea coastal region (e.g. the Netherlands, NW Germany and SW Denmark) and their offshore areas, key sites are many-metres-thick infills of topographic depressions in deglaciated terrain, between the maximum ice margins of the Saalian glaciation (MIS 6) and the Last Glacial (Fig. 1) (e.g. Zagwijn, 1983; Höfle et al., 1985; Streif, 2004; Beets et al., 2005; Konradi et al., 2005). Along the English Channel many LIG sites comprise flights of raised beaches, e.g. West Sussex coastal plain, southern England (Bates et al., 2010) and Cotentin, and northwestern France (Coutard et al., 2006). Along both the North Sea and the English Channel, the mouths of rivers record transgressed palaeovalleys and provide opportunities to constrain the regional LIG sea-level highstand (e.g. Antoine et al., 2007; Briant et al., 2012; Bogemans et al., 2016; Peeters et al., 2016). In the interest of brevity, a summary of the history of research in the study area is provided in Appendix A.
Table 1Global and regional time division schemes with details of the Eemian/Last Interglacial period.
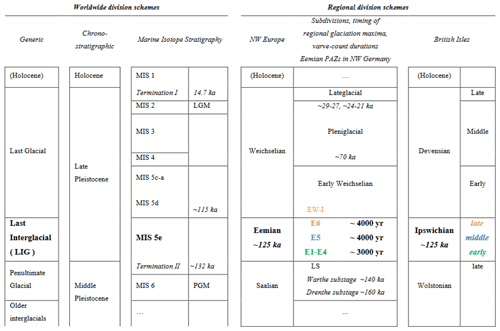
Table 2NW Europe WALIS data point totals, split by region.
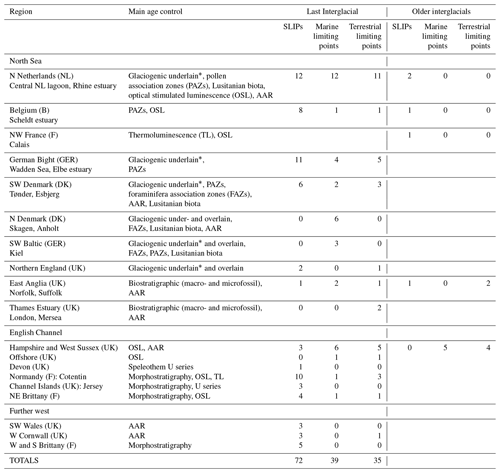
* One can commonly separate the Eemian from older interglacial deposits because they directly overlie MIS-6 glaciogenic sediments.
This paper sets out to describe the SLIPs and supporting marine limiting and terrestrial limiting data points (Table 2) extracted from the regional literature, evaluated and stored in the WALIS database according to the prescribed interface and formats. This paper first covers the North Sea, then the English Channel region. This regional division is also somewhat reflected in the descriptions of SLIP types, vertical land motion (VLM) and age assignments (various dating methods). At the end we provide some reflections on future research challenges in this region.
2.1 Regional context
Considerable spatial variation exists along the coastline of the present North Sea and English Channel (Fig. 1), as it did during the LIG (regionally known as Eemian and Ipswichian; Table 1). Extensive lowland coastal plains with back-barrier tidal environments, marshes and lagoons dominate the Belgian–Dutch–German–Danish stretch of North Sea coast. These are interrupted by headlands of Saalian-age ice-marginal morphology as well as by estuaries and deltas of the main rivers: Scheldt, Meuse, Rhine, Ems, Weser and Elbe. The northern English and East Anglian coasts of the North Sea have cliffs comprising Middle Pleistocene till accumulations over Cretaceous chalk bedrock. In SE England, the Thames Estuary is flanked by a coastal plain with smaller estuaries and Paleogene outcrops to the north and smaller-estuary-interrupted chalk cliffs to the south. The connection between the North Sea and English Channel is a transgressed gorge, eroded repeatedly since the Middle Pleistocene by lowstand periglacial and proglacial outwash rivers (Gibbard, 1995; Bridgland and D'Olier, 1995; Gupta et al., 2007; Toucanne et al., 2010; Mellett et al., 2013). The British and French coasts on either side of the English Channel alternate between estuaries (Somme, Seine, Sélune, Rance) and embayments rimmed with cliffs and gravelly and sand beaches, in a variety of substrates (Mesozoic, Palaeozoic, crystalline). Bedrock islands off the main coasts, such as the Isle of Wight and Channel Islands, were also islands in the LIG (Sect. 4).
There are also notable differences between LIG coastal geomorphology and that of today. The broad position of estuaries and cliff beach settings along the English Channel and SW North Sea can be regarded as essentially the same between the LIG and Holocene (Fig. 1). In contrast, the configuration of lagoons, river mouths and morainic islands in the Dutch and the German Bight sectors of the North Sea, critically in the area between the Saalian maximum and Last Glacial limits, is relatively different (Fig. 1). In the eastern North Sea region the Eemian transgression flooded a paraglacial landscape left after the Saalian deglaciation, whereas the MIS-6 glaciation did not extend as far south over the UK (Ehlers and Gibbard, 2004). Furthermore, long-term basin subsidence of the area means that in locations onshore and offshore the Netherlands and NW Germany (depocentre indicated in Fig. 1), even the shallowest preserved LIG sea-level archives are encountered several metres below modern mean sea level (MSL), below their elevation of deposition. By comparison, western North Sea and English Channel LIG features along the coast of the UK and France are generally preserved at elevations higher than present sea level. The combination of the “fresh” MIS-6 glaciated landscape and regional basin subsidence (Cohen et al., 2014, 2017) strongly affects the way deposits can be geologically and palaeogeographically mapped, reconstructed and studied (e.g. mainly from boreholes versus mainly from outcrops). This also impacts the extent over which deposits tend to be preserved (extensive basin-central patches versus small pockets along the rims of a system) and what relative age controls and dating opportunities apply, resulting in regional variability in the dating techniques applied (Table 2).
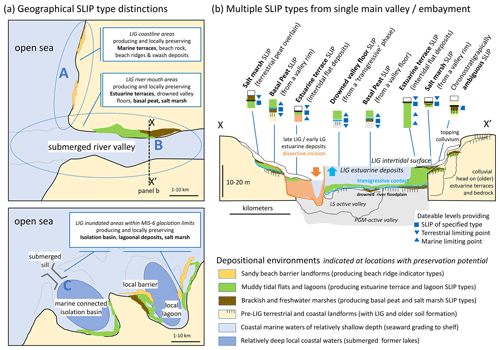
Figure 2Schematic map (a) and section (b) illustrating the geographical and sedimentary distinctions between the various SLIP types used for NW Europe in the WALIS database. In panel (a) three main groups of SLIPs are distinguished: A – coastline areas facing open sea, B – estuaries filling drowned river valleys, and C – glacially inherited marine-connected isolation basins and embayments. In panel (b) multiple SLIP types as well as terrestrial and marine limiting data points are summarised from local outcrops and boreholes. This includes SLIPs which record RSL rise, SLIPs which record stable sea level, and SLIPs which record RSL fall causing dissection and incision (orange arrow) within the estuarine cross-section. The symbology introduced for SLIP, terrestrial limiting and marine limiting points matches those in Fig. 1 and Figs. 4 and 5. Drowning sequences (e.g. submerged valley floors or basal peats, spanning a local or regional transgressive contact, common from early to middle interglacial phases in NW Europe) below the potential SLIP position often record a further terrestrial limiting data point. In contrast, estuarine terrace SLIPs typically have marine limiting indicators from facies underlying the SLIP and are common from middle–late and post-interglacial phases (Table 1).
2.2 Overview of indicator types
A wide range of sea-level indicators are present in NW European literature recording the LIG sea-level history in this region. Most of these studies constrain relative sea-level (RSL) elevations by combining sedimentary properties, fossil content and architectural evidence (Fig. 2). In the scope of the special issue and the WALIS database format we reviewed the literature and categorised the findings to WALIS-standardised sea-level indicator types (listed in Table 3a and further documented below), with particular attention to submerged valleys and further alluvial coastal plain settings from which series of SLIPs in lateral and vertical sequence can constrain sea level through multiple stages of the interglacial. In Table 3b we summarise the decision-making process of which geological observations become what SLIP types and the priority that is given in cases where a site could be classified as multiple SLIP types.
We employ 10 SLIP types, five of which were already part of the database set-up (“WALIS generic”) and five of which have been added by us during our literature review (“paper author added”). The SLIP types are split over three main settings (labelled A, B and C in Fig. 2 and Table 3b). Documentation of marine terraces and raised beaches (from setting A) is kept short as these SLIP types had been introduced and used in earlier WALIS publications (e.g. Rubio-Sandoval et al., 2021; Cerrone et al., 2021, in this special issue; and Rovere et al., 2016). SLIP types from estuarine river mouth, lagoonal and incised valley alluvial settings and the study-area-specific glaciogenic isolation basins (i.e. settings B and C) are documented in Sect. 2.2.1–2.2.6. All these SLIP types are widely used in Holocene RSL databases; their introduction in the LIG context and documentation here are mainly a formalisation for WALIS purposes.
The decision-making workflow in Table 3b shows that in addition to the specifically added types (salt marsh, basal peats, drowned valley floor, estuarine terrace, isolation basin; i.e. WALIS type IDs 17–20 and 37), we also occasionally use more generic, readily available “lagoonal deposits” and “shallow or intertidal fauna” classifications (WALIS type IDs 15 and 33), though as far less favoured options. Table 3b also outlines how to differentiate sites and samples as a marine or terrestrial limiting data point rather than a SLIP. For example, we classified so-called “regressive peat beds” from the late LIG that previous literature has used as sea-level constraints instead as late interglacial terrestrial limiting data points (Table 2).
2.2.1 Indicator type: drowned valley floor
This indicator type relates to a contact between terrestrial depositional facies (below) and subaqueous depositional facies (above) and provides constraints for marked sea-level-rise-created SLIPs (e.g. Fig. 2b). The terrestrial facies is typically a decimetre-thick organic river mud with immature palaeosol, if not a flood-basin peat bed, with further fluvial facies below. The subaquatic facies is typically a decimetre–metre-thick organic mud, rich in fine and coarse detrital organic matter; rich in silt admixture; bearing tidal indicators; and bearing microfossil indicators of occasional brackish, estuarine in-wash. It grades upward into established tidal, brackish to saline, full estuarine facies. See Hijma and Cohen (2011, 2019) for Holocene examples and Sier et al. (2015) and Peeters et al. (2016, 2019) for LIG examples.
The transgressive contact has a direct relationship to the point of estuarine inundation and is therefore a SLIP. The secondary contact within the terrestrial facies can be used as a terrestrial limiting point. Tides at the estuary mouth and their inland propagation, amplification and dissipation do affect estuarine-type SLIPs. Herein the estuary in a freshly drowned “underfilled” lowland valley differs (e.g. Martinius and Van den Berg, 2011) from that typically observed in later-stage filled estuaries. In wide valleys experiencing relatively rapid postglacial transgression, tidal amplification (owing to estuary funnelling) is not yet a major factor and may in fact be dampened inland. On the other hand, in inland parts of the estuary riverine discharge may impose a gradient and lift water levels to the same altitudes and above those of high tides in the estuary mouth (Van de Plassche, 1995; Vis et al., 2015). For these reasons, the “drowned valley floor” base-estuarine indicator type is kept separate from the “basal peat” and “estuary terrace” indicator types (Sect. 2.2.2 and 2.2.4; Table 3b).
The reference water level (RWL; Woodroffe and Barlow, 2015) definition for this indicator is the midpoint between the highest astronomical tide (HAT) and mean sea level (MSL, i.e. half-tide). The indicative range is the interval between HAT and MSL.
2.2.2 Indicator type: basal peats
Basal peats are terrestrial deposits encountered along the base of transgressive-to-highstand depositional systems and in submerged position on inner shelves (e.g. Jelgersma, 1979; Hanebuth et al., 2000). Using basal peats as RSL indicators became widely established in Holocene sea-level communities in the 1970s (Van de Plassche, 1986), both onshore and offshore. Jelgersma (1961) and Van de Plassche (1982, 1995) provide classic Holocene reference examples for the Netherlands. Likewise, it is useful as an RSL indicator in interglacial coastal settings (e.g. Zagwijn, 1983; Streif, 1990, 2004; Konradi et al., 2005), especially when combined with palynological investigations to provide time control on the position within the interglacial (see Sect. 2.3).
Basal peats are submerged terrestrial peats (swamp, fen, carr, marsh) overlain by subaqueous deposits (tidal, estuarine or lagoonal (organic) muds), allowing the identification of a regional transgressive surface at the drowning contact (e.g. Hijma and Cohen, 2011, 2019). Basal peats occur over multiple substrates and palaeosurfaces, such as those provided by valley floors and valley rims (e.g. Fig. 2b), but also over interfluve highs (regional relief) as well as on the flanks of small-scale isolated local relief within broader coastal deltaic plains. The encountered peaty terrestrial facies is typically a few decimetres thick (in a compacted state; cf. Greensmith and Tucker, 1986; Brain, 2015). Depending on lateral and vertical position (Fig. 2b) the basal peat may overlie a surface with just an immature floodplain palaeosol (see Sect. 2.2.1, “Indicator type: drowned valley floor”) or a surface with a more developed palaeosol (e.g. interfluve settings).
The very top of a basal peat bed indicates submergence of a swamp and marsh, and this level may be taken as a SLIP. The very base of basal peat bed indicates a palaeo-groundwater level (GWL), and in a sea-level reconstruction context that level becomes a terrestrial limiting point. In argued cases a limiting point may be upgraded to a SLIP if it formed along the inland rim of a transgressive lagoonal or lagoonal-deltaic environment (e.g. Van de Plassche, 1986; Nelson, 2015). To do so, one should assess the palaeogeographical situation of the basal peat data point or swarm of data points (Vis et al., 2015; Hijma and Cohen, 2019).
The RWL of this indicator for swamp basal peats is GWL − 0.1 m and for marsh basal peat is GWL − 0.5 m (GWL stood at 0.1 and 0.5 m, respectively, above the level where the peat formed). The indicative ranges for swamp and marsh peat GWL and derived sea-level indicators are 0–0.2 and 0.3–0.8 m, respectively, above the level where the peat formed. See Sect. 3.2 for additional compaction corrections.
2.2.3 Indicator type: isolation basin
Isolation basins are used extensively in Holocene sea-level studies in higher-latitude coastal environments, making use of the prominent occurrence of lakes in freshly deglaciated environments and the ecological sensitivity of such water bodies when connecting and disconnecting from the sea (e.g. Sundelin, 1917; Shennan et al., 2005; Long et al., 2011). In the North Sea area, substantial lakes formed when ice sheet cover from the penultimate glaciation disintegrated (during MIS 6). The period at which these lakes connected to the North Sea and transformed into highstand marine embayments has been a primary constraint on the relative timing of the Eemian transgression (e.g. Zagwijn, 1983). The reconstructed elevation of lake sills (the lowest point of the basin rim; Fig. 2b) provides the elevation of a SLIP of this type, whereas the contact between lacustrine environment (lower) and brackish marine environment (upper) established in the central part of a basin is where age control is obtained. Ideally the sill of an isolation basin is formed of unmodified bedrock (Long et al., 2011). In the North Sea case, it is formed by glacial diamicton and/or glaciotectonised ridges (Zagwijn, 1983; De Gans et al., 2000), which means additional uncertainty as to their elevation must be considered. Our WALIS database entries have registered the lake centre core location as coordinates, and note fields mention separately where the paired sill level is positioned. The RWL definition (midpoint between HAT and MSL) and indicative range are linked to the sill location and include the uncertainty in sill level elevation. Water depth of the lake is irrelevant in this application.
2.2.4 Indicator type: estuarine terrace
This indicator type is introduced as a variant of the marine terrace and raised beach entries (Table 3) as used elsewhere in the WALIS database. This allows one to assign a different indicative meaning to elevations sampled from estuarine terraces where the “flat” surfaces are usually formed in facies bearing intertidal sedimentological indications (alluvial terraces) than that assigned to marine terraces that are flattened due to abrasion processes (straths). A basic difference with the marine terraces is the orientation “across” and “along” that of the coastline (Fig. 2, Table 3b: setting A vs. setting B). A further difference is the opportunity to tie in estuarine terraces with fluvial and terrestrial units and this way (Zagwijn, 1983; Peeters et al., 2016) recognise dissective incision associated with sea-level fall (Fig. 2b). Paraphrasing the marine terrace description (Pirazzoli et al., 2005), it considers “any relatively flat surface of estuarine origin”. In the estuarine case, the flatness of the abandoned surface is not so much due to wave action and storm swash, but more due to intertidal and supratidal flooding just prior to terrace abandonment. For the seaward portions of estuarine terraces, the RWL definition is the midpoint between HAT and MSL, and the indicative range stretches from HAT to MSL. In the landward direction, estuarine terraces grade to riverine terraces and former floodplains that provide terrestrial limiting points rather than SLIPs. Examples include the Balgerhoeke (Heyse, 1979; IDs 339, 340; Scheldt: middle LIG, inland “high stand”) and Petten sites (Zagwijn, 1983; ID 146; Rhine: late LIG, seaward “regressive”).
2.2.5 Indicator types: salt marsh (various subtypes)
Salt marshes have been used extensively in Holocene sea-level research as their elevation and position are directly controlled by the tidal elevation and therefore can be directly related to a reference water level (Engelhart and Horton, 2012; Shennan et al., 2018; Barlow et al., 2013). The indicator type differs from “basal peat” and “estuarine tidal flat” types because the study of microfossils allowed it to be identified as a coastal salt marsh specifically. The identification of palaeo-salt marsh is usually through the identification of salt-marsh-specific taxa such as the pollen of Plantago maritima and Triglochin (Gehrels, 1994); the presence of salt marsh foraminifera such as Jadammina macrescens, Miliammina fusca and Trochammina inflata (Gehrels, 2000; Edwards and Horton, 2000); and brackish water diatoms and ostracods (Penney, 1987; Zong and Horton, 1998; Barlow et al., 2013). Such microfossils cover a limited elevation range from HAT to MSL and hence make palaeo-salt marshes an excellent SLIP. Where sedimentation could keep up with the rate of RSL change, salt marsh may be preserved at the transgressive or regressive boundaries often between freshwater peats and estuarine silts and clays. Microfossil sampling resolution is often coarse in LIG estuarine sediments (>5–10 cm intervals), and coastal salt marsh deposits may be missed between samples. Therefore, there are only two explicit occurrences in the LIG salt marsh SLIPs within the NW European database. A number of sites classified as “basal peat” SLIPs may well be overlain by fine-grained marine sediments that could be salt marsh. Also several “estuarine terrace tidal flat” sites are reported to have been overlain by salt marsh muds and peats (e.g. Balgerhoeke, Belgium: IDs 339 and 340; Land Hadeln, Germany: IDs 882 and 883). A default RWL for this indicator is the midpoint between HAT and MSL, and the interval between HAT and MSL is a default indicative range. Revisiting such sites may present opportunities to narrow down the RWL and the indicative range of some of these SLIPs.
2.2.6 Indicator type: shallow or intertidal marine fauna
This generic SLIP indicator type entry in WALIS considers palaeobiologically identified marine fauna that can be associated with very shallow water and/or intertidal environments, especially where fossilised “in viva”. It was used when sedimentary and morphological identifiers are not available and where sites did not convincingly fall into one of the categories listed above (Table 3b). In Danish contexts foraminifera and diatom assemblages have been used as water depth and current regime biotic indicators in Eemian shallow marine beds (e.g. Konradi, 1976; Konradi et al., 2005; Van Leeuwen et al., 2000; Beets et al., 2006). The otherwise most typical biotic indicators in the North Sea are shells of common intertidal mollusca; for example Cerastoderma edule (also known as Cardium) and Scrobicularia plana are very common intertidal species in the North Sea, in Holocene and LIG deposits alike. Macoma balthica and Spisula truncata are also frequently encountered (and used for AAR characterisation; Miller and Mangerud, 1985; Meijer and Cleveringa, 2009; Demarchi et al., 2011). In deeper waters of the offshore North Sea, Skagerrak and SW Baltic, the common species are Arctica islandica (also known as Cyprina) and Turritella communis. In the English Channel, foraminifera Elphidium sp. and Ammonia sp. are common intertidal indicators (e.g. Bates et al., 2010). Some of these species (“Lusitanian components”) in the LIG extended their common presence into the North Sea and the SW Baltic too (e.g. Madsen et al., 1908; Miller and Mangerud, 1985; Funder et al., 2002; Meng et al., 2022), whereas in the Holocene they did not (or to a far more limited extent). Examples are Venerupis senescens (Tapes aurea (var. eemensis), Paphia aurea, Amygdala), Bittium sp. and Cardium exiguum. Nolf (1973), Miller and Mangerud (1986: their part II), Meijer and Preece (1995), Wesselingh et al. (2010), and Meijer et al. (2021) are illustrative biota-oriented studies. This means that literature-reported molluscan faunas hold both “vertical” indicative meaning as well as “age” chronostratigraphic meaning.
For this type, RWL and the indicative range are based on the upper and lower limits of living modern analogue faunas (the midpoint and the interval between, respectively).
Table 4Chronostratigraphical entries for North Sea, Skagerrak–Kattegat and SW Baltic Sea.
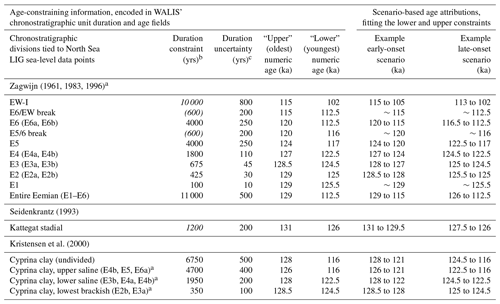
a WALIS entries use Zagwijn (1961, 1983, 1996) scheme. Descriptions for entries include correlations to schemes of Jessen and Milthers (1928; as used in Funder et al., 2002: Table 2), Selle (1962; as used in Konradi et al., 2005: Sect. 8), Behre (1962), Müller (1974), and Menke and Tynni (1984). Correlation for Cyprina clay complies with that in Kristensen et al. (2000) and Meng et al. (2022). b In regular font, for E1 to E6: based on NW German lowland varved lake site “Bispingen”, Muller (1974) and Zagwijn (1996). In italics: for E5/6 break and E6/EW break, arbitrarily defined as 300 years on either side of break. For EW-I: varve counts in Eifel mar lakes (Sirocko et al., 2005) and their dust-flux correlation with Greenland ice cores NGRIP and NEEM (Sirocko et al., 2005; NEEM community members, 2013; Sier et al., 2015). For the Kattegat stadial: assessment of Seidenkrantz (1993). These estimated durations are regarded as minimum durations in the early-onset scenario. c Authors' expert judgement: acknowledges varve miscount possibilities as well as PAZ correlation diachronicity issues over ca. 400 km distance between “coastal Netherlands” (Zagwijn, 1961; Amersfoort) and “NW German terrestrial” sites (Müller, 1974; Bispingen), Eifel mar lakes (Sirocko et al., 2005), NE Denmark (Kattegat stadial; Seidenkrantz, 1993) and/or the SW Baltic (Cyprina clay; Kristensen et al., 2002).
2.3 Eemian chronostratigraphy
Where U-series or OSL dates were reported, this age information was inserted into WALIS' database structure. The great majority of sites in continental NW Europe, however, have chronostratigraphic relative age constraints for which a separate registration scheme exists. We filled this with entries for the Eemian pollen association zones (PAZs; numbered E1–E6) of Zagwijn (1996) as established for the Netherlands. The information in this part of the WALIS database is summarised in Table 4. In the note field for each entry, we included the correlation to regarded equivalent zones in various NW German and Danish biostratigraphic schemes for the LIG (references in Table 4 footnote). In dedicated numeric fields, we register duration constraints provided by varve counts from the Bispingen site in NW Germany (Müller, 1974) as well as correlated minimum and maximum absolute ages (Table 4: left side). The presented ages aim to encompass all presently considered options for the age span represented by the floating PAZ scheme (see Long et al., 2015), which includes early-onset and late-onset options (Table 4: right side). Details on the age information (maximum and minimum spans, early- or late-onset options) are provided in Appendix B as well as in the note field for the main entry in the WALIS database itself.
Since the Tzedakis et al. (2018) publication on Italian speleothem U-series chronology and the Iberian margin marine isotopic record, the early-onset correlation option for PAZ E1–E6 stretches from ca. 129 to 116 ka (PAZ E5 commencing around 124 ka), and a late option covers ca. 126 to 112 ka (with PAZ E5 ending by 117–116 ka and durations closer to the original estimates of Müller, 1974). The respective WALIS minimum and maximum age entries for each PAZ cover both options. We note that the application of Eemian PAZ schemes within the interglacial is relatively straightforward in the LIG coastal zone because of the underlying glaciogenic deposits at most Dutch, German and Danish sites (Table 2). We stress that the PAZ correlation-attributed numeric ages (i.e. the onset scenarios in Table 4) should only be applied to localities around the eastern and southern North Sea area. Bispingen (location of varve counts of Müller, 1974) is at most 100 km from the German Bight and within 500 km of our Belgian sites (Fig. 1). If entries for RSL indicators with palynology-derived chronostratigraphic age control are needed for sites further east (e.g. Russian “Mikulinian” zonation) or to the southwest in the English Channel, we advise adding separate entries in WALIS, potentially adapting the advised oldest and youngest age bounds, and exploring possible W–E diachronicities.
The situation for UK sites is different as palynological indicators and settings are often ambiguous in revealing whether an interglacial deposit correlates to the LIG or an older counterpart. Due to significant advances in AAR methods (Penkman et al., 2013), combined with river terrace stratigraphy (Bridgland, 1994), vertebrate and mollusca biostratigraphy (Schreve, 2001; e.g. Preece, 2001), and independent geochronology, many British sites previously thought to relate to the LIG based on Ipswichian pollen stratigraphy (e.g. Hollin, 1977) are now argued to actually date from preceding interglacials (Penkman et al., 2008, 2011, 2013). Therefore, many correlated “Ipswichian” sites from the UK were not included in the database. Those sites with AAR-based LIG age associations were included in the WALIS-provided database substructure for this dating type.
3.1 Elevation measurements and datums
For any palaeoenvironmental record to provide a useful indictor of past sea level, the elevation of the deposit or landform must be recorded (Shennan et al., 2015). Following WALIS database structures for this, the measured elevations for the studied sites have been reported in various forms, detailed in Table 5. Our approach has been to express elevations for data points as much as possible to 19–20th-century reference mean sea level (details given in Table 6), applying conversions from local datums when needed (for further discussion see Woodroffe and Barlow, 2015).
3.2 Compaction corrections
Most SLIPs and limiting data points are from sites and indicator types that do not require compaction correction, e.g. marine terraces, beach deposits, drowned valley floor and isolation basin sills (Table 3). Basal peat, lagoonal and estuarine tidal flat type indicators do require estimates of compaction as the sediments are more compressible, and these are often sampled from buried positions with considerable overburden. The decompaction approach is a pragmatic one. Detailed compaction correction in general is not feasible, and our decompaction approach is based upon analogies and experience in Holocene settings. For the Holocene, geomechanical empirical modelling suggests similar decompaction factors for basal organic beds and clayey, tidal lagoonal sequences (e.g. Greensmith and Tucker, 1986; Brain, 2015; Keogh et al., 2021). The corrections have been primarily applied to records from the Netherlands, NW Germany and SW Denmark and then mainly to basal peat SLIPs and associated marine limiting indicators (Fig. 2b). All site-specific compaction corrections are documented in the “Notes on elevation and indicative range” field for each entry.
For basal peats we assume a full analogue with Holocene deposits from the area. Where Holocene peats are overlain by 10–15 m of coastal overburden, transgressed peats are compressed to 50 %–33 % of their original thickness, with the greatest majority of this compression happening in the first millennia after burial (Hijma et al., 2009; Hijma and Cohen, 2011), when the first 5 m of overburden accumulated (Van Asselen et al., 2011; Keogh and Törnqvist, 2019; Keogh et al., 2021). Decompaction of decimetre-thick basal peat beds is achieved by multiplying thickness by a factor of 2 to 3 (Berendsen et al., 2007; Hijma and Cohen, 2019). For Eemian basal peats, the overburden is similarly thick but exists for longer and can be relatively sandy (descriptions stored in note fields summarise overburden composition for those database entries where compaction assessments were incorporated). We hence considered a decompaction factor of 2.5 to 3.5 for dominantly organic beds and 2 to 3 for clay–peat alternating intervals.
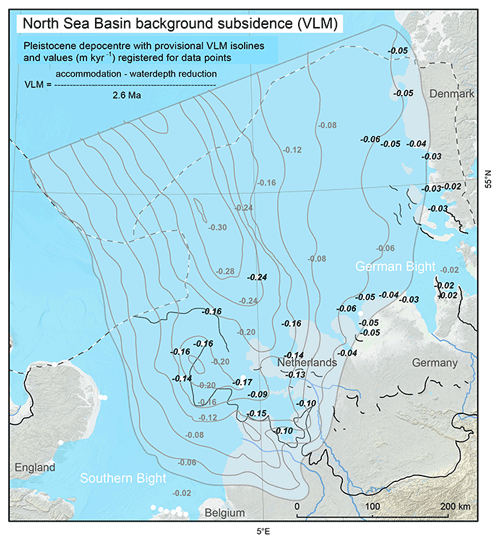
Figure 3VLM rates (m kyr−1) for North Sea basin LIG data points. Area of subsidence and VLM contours, based on depth of base Pleistocene in marine formations (onshore and offshore data from NL, GER and DK geological surveys), negotiated for water depth reduction during the Early Pleistocene (isoline increment: 0.04 m kyr−1, i.e. equivalent to 5 m RSL correction accumulated over 120 kyr). Values in black italics: WALIS VLM entries for LIG data points, reproducing Kooi et al. (1998) for the Netherlands. Offshore subsidence patterns deviate subtly from the 1998 publication, and for the German Bight coverage was extended based on improved mapping of base Pleistocene. Backdrop as in Fig. 1, containing land data © OpenStreetMap contributors 2020. Distributed under the Open Data Commons Open Database License (ODbL) v1.0.
For SLIPs from lagoonal deposits and estuarine tidal flat surfaces (Fig. 2, Table 3a), decompaction of clayey tidal deposits immediately underlying these levels is considered. As in the application to the top of basal peats, we mainly assess “post-depositional” compaction for estuarine sediments. The compressing of any underlying basal peats would have occurred rapidly, i.e. during the functioning of the estuary and eventually resulting in the estuary filled with a tidal flat surface (Brain, 2015). Subtidal clayey facies is regarded to be more prone to remaining compaction (decompaction factor 1.5 to 2.5) than intertidal and supratidal facies (decompaction factor 1 to 1.5) because the latter had been subjected to wet–dry cycles at the time of deposition (Paul et al., 2004). The thickness and composition of overburden affect whether to opt for the lower or higher side of the decompaction factor.
A few sites in the database are from particularly thick organo-clastic Eemian sequences, e.g. deglaciation-inherited deep channels onshore in the German Bight (e.g. Dagebüll, Schnittlohe), and from glacial-tongue basins in the Netherlands (e.g. Amsterdam, Amersfoort; Fig. 1). We avoided including heavily compaction-influenced sea-level markers and regression terrestrial limiting points from such localities, instead opting for sites along the rims of the basins (see Kasse et al., 2022, for the Amersfoort example). From the deep and thick German Bight sequences, SLIPs and limiting points are only recorded in the database from the deepest levels in the sequences, for which compaction corrections are relatively minor. The environments became subaqueous following the transgression, and it is the proxy water depth inaccuracy, rather than decompaction, that affects indicator elevation position and associated uncertainty. In the Amsterdam tongue basin, an isolation-basin-style sill of glaciogenic deposits controls the elevation of sea level, and therefore compaction could be ignored.
3.3 Vertical land motion (VLM) correction
Rates of vertical land motion (VLM), due to basin subsidence and sediment loading in the North Sea basin, are on the order of 0.1–0.2 m kyr−1 (Kooi et al., 1998), resulting in up to 10 m of apparent RSL fall simply due to VLM at locations around the LIG highstand shoreline and up to 20 m further offshore at locations closer to depocentres (Fig. 3). Such spatial variation in tectonic subsidence thus significantly affects the comparison elevation of inland and offshore RSL data points through the interglacial. Earlier studies that compiled LIG sea-level data from the North Sea region, as part of larger-scale analysis (notably Lambeck et al., 2006, and Kopp et al., 2009), implemented VLM corrections (mainly to the data points from Zagwijn, 1983; Fig. A1a) to account for this. Accordingly, we develop updated VLM corrections based on geological information independent of the LIG data points. To do this consistently for all onshore and offshore sites from the Netherlands, NW Germany and SW Denmark we incorporate up-to-date mapping of the base Quaternary (equivalent to base Pleistocene) sediments in these areas and expand and update the subsidence quantification analysis from Kooi et al. (1998). Applying VLM corrections allows us to remove uniform background non-GIA land-level change, which in turn allows the regional spatial and temporal pattern of GIA RSL due to the growth and melt of the Eurasian ice sheets to be explored.
The VLM rates for the southern North Sea in Fig. 3 echo those obtained for Dutch sectors in Kooi et al. (1998: their Fig. 4; all three components summed), who performed a tectono-sedimentary back-stripping analysis on thickness of Quaternary and Neogene sequence of the basin and presented mean rates estimated over the last 2.6 Myr. The starting point for that was the mapping of Quaternary thickness in the North Sea basin (“accommodation”), based on collated offshore seismo-stratigraphic and onshore lithostratigraphic mappings and relying on identification of Early Pleistocene marine strata therein (tens to hundreds of metres below the seabed and Middle Pleistocene glaciogenic unconformities; Ottesen et al., 2014). For reanalysis and assessment of the 1998 outcomes (1990s state of mapping offshore was rather coarse), we re-generated such source materials from current onshore–offshore geological survey digital mapping resources (2020 state of mapping, especially refined offshore and better aligned across national borders). For the onshore and nearshore the Netherlands, the VLM results broadly reproduced the accommodation patterns and mean subsidence rates over the last 2.6 Myr. For areas further offshore, the mapping of the base Quaternary had been considerably revised (e.g. Lamb et al., 2018) compared to the 1990s. This has shifted depocentre contours, which locally nudged VLM values from −0.03 to +0.03 m kyr−1 relative to the 1998 results. VLM rates for the German Bight lay outside the area covered in the 1998 analysis but are now otherwise produced in the same way. Figure 3 thus is an expansion of earlier basin subsidence component quantifying work.
The contour lines in Fig. 3 served as VLM subsidence isolines where values for newer data points had to be assessed. VLM used in WALIS for this region ranges from −0.02 m kyr−1 at marginal locations to −0.24 m kyr−1 at sites over Quaternary depocentres. VLM rate uncertainty is ±0.01 for sites along the basin margin (subsidence of −0.02 to −0.03 m kyr−1) and increases to ±0.04 in far offshore depocentres. The propagated effects of combined age uncertainty, palaeo-RSL uncertainty and VLM uncertainty on projecting North Sea data points in age–depth plots are considerable, shifting from decimetres to metres as reporting palaeo-RSL elevation with and without VLM correction in the next sections shows. Our vertical corrections and associated uncertainties for the Zagwijn (1983) subset were verified to reproduce such calculations reported in Lambeck et al. (2006). We also verified our rate uncertainties against those used in Kopp et al. (2009). The WALIS-registered uncertainty for North Sea offshore site “#2” is similar (−0.03 vs −0.0255 m kyr−1), but for inshore sites of the Zagwijn (1983) series our uncertainties are roughly twice those used in Kopp et al. (2009).
We do not provide VLM rates for the stable and modestly uplifting areas around the margin of the North Sea basin and along the English Channel. Independent estimation of the rates of VLM are not available as studies that provided uplift rates do so based upon the same marine terrace elevations that we document as SLIPs. Very modest long-term subsidence rates may apply to NE Belgium, Denmark and N Germany during the Pleistocene, or alternatively they can be viewed as fairly stable (Kiden et al., 2002). Whether the Dover–Calais area is neotectonically active, and owing to what cause, is debated (e.g. Van Vliet-Lanoë et al., 2000; Westaway et al., 2002; García-Moreno et al., 2015). The region is also known to have lost considerable volume of bedrock in Middle Pleistocene times (proglacial erosion of Dover Strait; Gibbard, 1995; Gupta et al., 2007, 2017) with some isostatic uplift in response. Regardless, the apparent uplift due to both GIA and non-GIA VLM based upon the flight of raised beaches is modest (+0.02 to +0.04 m kyr−1; Pedoja et al., 2018). This similarly applies to sites along the English and French sides of the English Channel. The flight of raised beaches in West Sussex (Bates et al., 2010; Briant et al., 2019) occurs in an area where net uplift is thought to be significant, with apparent rates between 0.06 and 0.12 m kyr−1, explaining the mean vertical separation of highstand beaches of the last few cycles (e.g. Westaway et al., 2006), although these rate calculations are sensitive to terrace age attributions (see Sect. 6.2). Moreover, the values for sections of French and English coastlines are total rates that do not independently allow for separating out non-GIA and GIA (including hydro-isostasy) components. Overall, the anticipated non-GIA VLM vertical corrections for uplifting sites along the English Channel coast would be significantly smaller than the ones specified for the North Sea depocentre (perhaps with West Sussex data points as a local exception). Developing such corrections remains an ongoing challenge for this area.
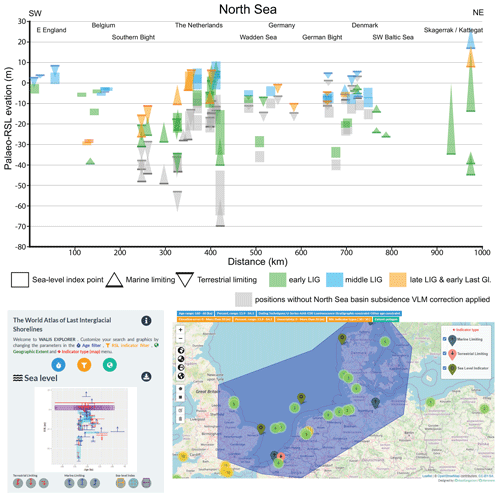
Figure 4SW–NE-organised plot of Last Interglacial WALIS data entries for the North Sea, split into type and relative position. Transect location in Fig. 1. Colour coding separates data points of middle-LIG relative age from those attributed to earlier and later phases within the LIG (see also Table 1). Bottom panels show sites and age–depth plot (without VLM corrections) as a screenshot of WALIS' visualisation interface (Garzon and Rovere, 2021): https://warmcoasts.eu/world-atlas.html (last access: 30 April 2022), which includes data as basemap © OpenStreetMap contributors 2020. Distributed under the Open Data Commons Open Database License (ODbL) v1.0. Only LIG data points are plotted.
Where we report VLM-corrected elevations for North Sea SLIPs and limiting data points in the next section, we calculated these with the “late onset” numeric ages as listed in Table 4. The vertical positions will further rise if the age model is shifted to the older onset scenario. In the transect plot of Fig. 4, such age attribution sensitivities will affect early- and late-LIG data points collected offshore more than the mid-interglacial data points that predominantly come from inland positions (Sect. 4.1 and 4.2).
This section summarises the database contents for NW Europe, as based upon the data input considerations outlined above. The overview is grouped by region and where age constraints allowed are broadly characterised as early, middle and late LIG. Figures 4 and 5 present the data points along broad SW–NE transects in the North Sea and the English Channel (see Fig. 1), i.e. at increasing distance from main MIS-6 ice cover over NW Europe.
4.1 North Sea: the Netherlands and Belgium
In the Netherlands and Belgium availability of national online geological datasets (e.g. Van der Meulen et al., 2013) means that all sites and boreholes in this region were looked up in web portals to verify coordinates, surface elevations, layer depths etc. For the Netherlands we used https://www.dinoloket.nl/en/subsurface-data (last access: 1 October 2021) and for Belgium (Flanders) https://www.dov.vlaanderen.be (last access: 1 October 2021), and we have included the original IDs from these portals. Coordinates of legacy sites based on outcrops were also checked using digital topographic and aerial photography resources.
Deposits along the rim of the Dutch Eemian coastal plain are typically lagoon- and estuary-fringe sites in the central Netherlands (Fig. 1; see also Fig. A1), most famously Amersfoort (Zagwijn, 1983; Cleveringa et al., 2000; Kasse et al., 2022: WALIS IDs 133, 137, 138), but also at Oosterwolde (Peeters et al., 2016: IDs 134–136), Rutten (Sier et al., 2015; Peeters et al., 2016: IDs 109, 111, 114, 115), Scharnegoutum (Zagwijn, 1983: IDs 124–127) and Annen (Bosch, 1990: IDs 4260, 4261). Each of these provided multiple SLIPS and limiting points, from PAZ E4 onwards (PAZ E4–E6; Oosterwolde site OSL-dated). The most inland, shallowest preserved coastal beds are encountered at −8 to −10 m m.s.l., notably at Amersfoort and Annen (both PAZ E5) as well as Scharnegoutum (PAZ E5/6 break). With VLM corrections applied, these relatively highest SLIPs plot at +3.5, +1.2 and +6.2 m m.s.l., respectively. From earlier in the interglacial (PAZ E4), additional SLIPs have palaeo-RSL depths between −21 and −13 m m.s.l., and with VLM correction these plot between −8.3 and −1.4 m (PAZ E4a) and −7.1 and +1.2 m (PAZ E4b). Overall, SLIP uncertainties range from ±0.35 to 1.3 m when decompaction is included and from ±3.6 to 4.5 m when also VLM is included (Fig. 4).
Seaward from the inner rim of the Eemian coastal plain, marine deposits are encountered down to −40 m m.s.l., and at −35 to −65 m m.s.l. offshore. This area provides SLIPs which record evidence of early interglacial transgression during PAZ E3 such as near Amsterdam (Zagwijn, 1983; Cleveringa et al., 2000; De Gans et al., 2000; Van Leeuwen et al., 2000; Beets and Beets, 2003: IDs 118–120) and offshore boreholes “BH89/2” (Sha et al., 1991; Beets et al., 2005) as well as during PAZs E6 and EW, such as at Petten (Zagwijn, 1983: IDs 146–148). Terrestrial limiting points help further constrain maximum elevations for sea-level rise at the time of regional onset of interglacial conditions (PAZ E1–E2), while marine limiting points (for PAZs E4 and E5) constrain minimum heights towards and during the middle interglacial.
The PAZ E3a SLIP from the Amsterdam glacial basin (ID 118) is characterised as the “isolation basin” type, recording the moment of marine connection. The sill height of this isolation basin occurs at m m.s.l. (tidal amplitude and sill elevation uncertainties considered), which with VLM accounted for rises to m m.s.l. Offshore site BH89/2 is also a glacial basin (Beets et al., 2005), where transgression of an isolation basin is recorded (ID 122, PAZ E3a) at 0 m m.s.l. The large uncertainty is due to the poorly defined sill location for this basin. This site has the greatest-estimated basin subsidence (Fig. 3); applying a VLM correction of m kyr−1 means the elevation is adjusted to m m.s.l. (Fig. 4). At Petten (ID 148, PAZ E6b) we combined coeval terrestrial and marine limiting evidence in a transect of cores (Zagwijn, 1983) to define a SLIP at m (VLM-corrected). North Sea boreholes “#2”, “#3”, “#5” and “#9” (Zagwijn, 1983) provide terrestrial and marine limiting data points only (IDs 309, 311, 317, 319 320, 380), as do onshore boreholes such as Noorderhoeve (Meijer, 2002; Peeters et al., 2016: ID 898).
The majority of Belgian RSL data points are from estuarine tidal deposits preserved west to east along the rim of the LIG Scheldt estuary (Fig. 1; see also Fig. A1). They occur at shallower subsurface positions than in the Netherlands, with three inland SLIPs coming from pit exposures – Meetkerke (De Moor and De Breuck, 1973; Nolf, 1973; ID 3259; see Fig. A1), Dhondt and Coppens pits (Heyse, 1979: IDs 341, 345) – and three more from boreholes at Waterpolder and Balgerhoeke (Heyse, 1979: IDs 338–340). The latter is the most eastward and shallowest site, preserving supratidal mud (ID 339, PAZ E5) and salt-marsh peat (ID 340, PAZ E5) through the absence of microfossil analysis to confirm this means this data point is characterised as of “estuarine terrace” type. The other SLIPs are from intertidal levels. For PAZ E5, the NW Belgian RSL positions occur between −3.1 and −2.9 m m.s.l. (Coppens pit, Waterpolder, Balgerhoeke), with a lower data point of −5.4 m m.s.l. (Dhondt pit) during PAZ E5 potentially recording the onset of falling RSL. These values include large modern-day outer-estuary tidal amplitudes for the Scheldt, with mesotidal palaeo-RSL uncertainties ranging from ±0.8 to 1.6 m. Reducing tidal range uncertainties and considering modest VLM subsidence (e.g. m kyr−1; Fig. 3) will raise the elevation of the Belgian points compared to the current plotting in Fig. 4 and warrants further investigation.
Westwards, further Belgian sites reveal truncated sequences lacking supratidal contacts, generating transgressive SLIPs from PAZ E4, ranging between m m.s.l. (De Clercq et al., 2018: Vlissegem, ID 353) and m m.s.l. (Meetkerke), whereas the terrestrial site Vossenhol (Heyse, 1979: ID 337) constrains RSL to below 2 m m.s.l. during the same period. Offshore, borehole GR1 (De Clercq et al., 2018) reveals active tidal scour during PAZ E4, suggesting a marine lower limit at −38.3 m m.s.l. Lastly, in southwestern Belgium, boreholes at Woumen and Kellen in the Yser palaeovalley (Bogemans et al., 2016) provide maximum SLIP altitudes of −5.7 m m.s.l. (ID 346, including some decompaction and assuming 2 to 3.5 m tidal amplitude) and −3.1 m m.s.l. for an older interglacial (ID 347, MIS 7).
4.2 North Sea: Germany and Denmark
In NW Germany and SW Denmark, the LIG highstand shoreline ran roughly parallel to that of the modern Wadden Sea (Fig. 1), locally preserving tidal flat and supratidal marsh deposits below −7.5 m m.s.l. Where the shoreline turns north in the German Bight, it shows more LIG estuarine indentations than it does in present times. These inshore settings, like in the Netherlands and Belgium, produced a series of tidal flat surfaces topped by terrestrial limiting points, suggesting falling RSL. These are the German sites Leybucht and Land Hadeln (Höfle et al., 1985; Streif, 1990, 2004: IDs 880–884, 4271–4273); the classic Danish site Tønder (Madsen et al., 1908; Nordmann, 1928; Friborg, 1996: IDs 865, 866); and further sites Ribe, Esbjerg, Ringkobing and Harbooere (Konradi et al., 2005: IDs 869–873). In NW Germany, the highest sea-level positions appear to have recorded for the entire length of PAZ E5. Where Danish–German sites have palynological chronostratigraphical control, the database entries encoded the established regional correlation schemes (e.g. Kristensen et al., 2000; Funder et al., 2002) towards the Zagwijn scheme (Table 4: upper rows). Where the relative dating was instead correlated to phases in the deposition established for the “Cyprina clay” (relatively deep water in the SW Baltic), this was encoded as such and subsequently in turn correlated to the Zagwijn PAZ scheme (Table 4, lower rows; following Funder et al., 2002).
Palaeo-RSL from the shallowest SLIPs between Ems and Weser estuaries (Fig. 1) plot at −10 m m.s.l. (Leybucht, Land Hadeln), which is ∼5 m deeper than the data points north of the Elbe estuary in Danish–German borderlands (Tønder, Ribe; marine limiting data only). Northwards into Denmark, SLIP elevations fall to between −15 and −10 m m.s.l. (Esbjerg, Ringkobing, Harbooere). A particularity is borehole site Vovov Bakkeøer (Konradi et al., 2005: ID 874) in the western Danish offshore area that revealed a patch of Saalian till and outwash deposits with Eemian marine cover, bearing foraminiferal evidence for later shallower local submergence than that of the deeper waters surrounding it (e.g. Horn Reef M3, ID 864). Hence, despite being positioned offshore, this “drowned island” location produces a middle interglacial SLIP (E4b–E6a) at m m.s.l. Terrestrial LIG exposures are obtained from around modern MSL (−4 to +2 m) from shallow boreholes (Esbjerg, ID 871) and classic exposures in low cliffs along the Danish Waddensea (Nordmann, 1928: Emmerlev Klev, ID 867). They place a terrestrial limit at −3.6 m m.s.l. and at modern sea level (0 m m.s.l.), to which inland shallow lacustrine beds at Tønder add a third such point at +2 m (Friborg, 1996: ID 866). Deploying VLM corrections to the German and Danish sites (with generally lower subsidence rates than applied to data points in the Netherlands; Fig. 3) adjusts the maximum RSL elevations to between −5.2 and +2 m m.s.l. (and −7.1 m for Vovov Bakkeøer) while projecting the terrestrial limits to 2 to +4.4 m m.s.l. The vertical uncertainty in these SLIPs ranges between ±0.5 and ±1.0 m and expands to typically ±2.5 m when VLM is included. Importantly a good part of the middle interglacial SLIPs after VLM correction in this region have a position just below present MSL, suggesting significant near-field GIA fingerprint effects to be in play in this area (as in the Holocene; e.g. Vink et al., 2007).
A cluster of early and late Eemian rather than middle interglacial data points comes from relatively deep and/or offshore boreholes in the Ems Estuary and western-German Wadden islands Borkum, Norderney and Spiekeroog (Höfle et al., 1985; Streif, 1990, 1991, 2004; Schaumann et al., 2021: IDs 885–888, 4269–4270, 4274–4275). These include a transgression SLIP (Borkum, ID 886, PAZ E3b) at −36 m m.s.l. and a coeval terrestrial limiting at −16.5 m m.s.l. (Ems Estuary, ID 885, PAZ E3) as well as terrestrial limiting points at −8.5 m m.s.l. (Norderney, ID 887, PAZ E6) and −16.5 m m.s.l. (Spierkeroog, ID 888, PAZ E6b/EW-Ia), coincident with the end-of-interglacial conditions. With differential VLM correction, the points plot at −28.6 and −9.1 m m.s.l. (early interglacial, PAZ E3) and −8.5 and −16.5 m m.s.l. (late interglacial–early glacial), respectively. Uncertainties are ±0.5–1.0 m without and ±2.5 m with VLM correction (Fig. 4).
A further major cluster of such early and late Eemian sites comes from the German–Danish borderlands from deeper buried estuarine and marine deposits filling outwash valleys inherited from the MIS-6 deglaciation. The lower parts of these valley fills (typically below −17 m m.s.l.) survived erosion by glacio-fluvial outwash systems of Last Glacial age. The sites recorded regional transgressive sequences, which yield early Eemian SLIPs and marine limiting points. Higher up the sequences also recorded local regression, and those levels have provided late Eemian data points. The cluster comprises sites Schnittlohe (Kosack and Lange, 1985; Lambeck et al., 2006; ID 889), Dagebüll (Winn and Erlenkeuser, 1995; Winn et al., 2000; IDs 875–877), Højer (Madsen et al., 1909; Nordmann, 1928; Konradi et al., 2005; ID 868) and Horns Reef M3 (Konradi et al., 2005; ID 864) in the German Bight and sites Krummland (Winn and Erlenkeuser, 1995; Winn et al., 2000: IDs 878, 879) and Tuschenbeck (Winn and Erlenkeuser, 1998; ID 890) along the German Baltic Sea coastline (Fig. 1). These types of sites are especially important along the SW Baltic as there it represents rare preservation of inland-most coastal and estuarine beds as the Last Glacial ice advance overran areas that otherwise eroded superficial littoral LIG deposits (making the area unsuitable to provide LIG SLIPs). In the SW Baltic, the sequences typically hold lacustrine deposits in the lowest part, which due to imprecise water depth control do not offer valuable terrestrial limiting points. However, the timing of marine inundation is well established, occurring in PAZ E3a at Baltic-facing sites Tuschenbeck and Krummland and later at the inland site of Schnittlohe (PAZ E4). North-Sea-facing sites Horns Reef M3 (basal peat), Dagebüll (beach facies at base) and Højer (shallow channel fill peat) all come from the valley floor rather than lake settings, which provides a better opportunity to define RSLs.
Early interglacial SLIPs from local transgressive contexts are present at −40.0 m m.s.l. (ID 864, PAZ E2a), at −24 m m.s.l. (ID 875, PAZ E3a–E4b) and at −23 m m.s.l. (ID 868, PAZ E4) and plot at m, m and m m.s.l., respectively, with differential VLM correction (Fig. 4). In the sequences at Dagebüll, Schnittlohe, Krummland and Tuschenbeck this is followed by marine limiting data from PAZ 4b–E6a, the highest at −12.5 m (Krummland, ID 879) as well as −6.8 m (Dagebüll, ID 876) and −4 m (Schnittlohe, ID 889). The top of the Eemian marine sequence at Dagebüll is intertidal and records a regressive SLIP at −9.7 m m.s.l. (ID 878, PAZ E6, m m.s.l. with VLM correction), which is rare from N Germany (Fig. 4). Abundant palynological, foraminiferal, molluscan and high-density subsurface mapping provides control on the setting, palaeoenvironment and relative ages of the above set of sites. At Dagebüll additional the above set of sites. At Dagebüll additional U series (base), oxygen isotopic investigations (middle), and an electron spin resonance date (top) confirm a MIS-5e age (Winn et al., 2000).
Lastly, two deep-water marine limiting sites are available from the northeast of Denmark, Skaerumhede I (Jessen et al., 1910; Houmark-Nielsen, 1987; Larsen et al., 2009; ID 856) and Flakket, Anholt (Seidenkranz, 1993; IDs 857–861). The former is a deep research borehole recovering some 80 m of deep-water facies (lower Skaerumhede Clay Formation) deposited in the LIG equivalent of the Skagerrak channel (Fig. 1), with foraminifera and mollusca biostratigraphy (Bahnson et al., 1973; Knudsen and Lykke-Andersen, 1982; Knudsen et al., 2009) which tie the water depths to 100–200 m in the facies encountered at −35 m m.s.l. This translates to a single marine limiting point (ID 856) at m m.s.l. (Fig. 4). Marine palynological and biostratigraphically data from Flakket also (Seidenkrantz, 1993) suggested that waters deepen from 35±5 to 100±10 m between the onset of the Eemian (ID 857; Kattegat stadial; ID 858, PAZ E2a–E3a) and through the middle and later stages of the interglacial (ID 859, PAZ E3a–E4b; ID 860, PAZ E4b–E6a). This deepening correlates with the Cyprina clay phases in the SW Baltic (Table 4) and with the boreo-Lusitanian (see Sect. 2.2.6) molluscan and foraminifera in the Skaerumhede boreholes. With species water depth tolerances accounted for, the marine limiting depths rise from −40 to −36.5 m m.s.l. in the early Eemian, then to m m.s.l. during PAZ E3a–E3b, and a maximum of m m.s.l. (PAZ E4b–E6a). The top of the Flakket sequence shows a decreasing water depth, but the site remains marine during PAZ E6, defining a marine limiting point (ID 861) of m m.s.l. The apparent super-elevation of the LIG RSL data from these northwesternmost sites (Fig. 4) reflects the strong gradient in GIA from this repeatedly ice-covered part of the study area to the increasingly GIA-peripheral North Sea and increasingly GIA-peripheral North Sea and English Channel regions to the southwest (as known from Holocene sea-level studies for NE Denmark; Clemmensen et al., 2018).
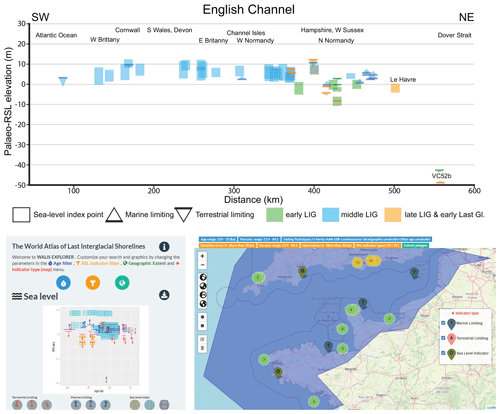
Figure 5SW–NE-organised plot of Last Interglacial WALIS data entries for the English Channel, split into type and relative position. Transect location in Fig. 1. Colour coding separates data points of middle-LIG relative age from those attributed to earlier and later phases within the LIG (see also Table 1). Bottom panel shows sites and age–depth plot as a screenshot of WALIS' interactive viewer (Garzon and Rovere, 2021): https://warmcoasts.eu/world-atlas.html (last access: 30 April 2022), which includes data as basemap © OpenStreetMap contributors 2020. Distributed under the Open Data Commons Open Database License (ODbL) v1.0. Only LIG data points plotted.
4.3 North Sea: Thames Estuary, East Anglia, northern England
Sea-level data points from the western North Sea coast primarily comprise estuarine deposits from in or just above tidal frame. In the Thames, Preece (1999) describes a terrestrial limiting point (ID 3713) based upon the presence of freshwater mollusca throughout a sequence of organic silts from −2.4 to +3 m OD, that overlie a basal gravel (similar to the stratigraphy found at other locations in Trafalgar Square). The presence of the brackish water ostracod Cyprideis torosa and a few tests of the foraminifera Elphidium articulatum, which were likely carried upstream by tidal action, suggest this site was deposited just beyond the limit of HAT. Similarly macro- (hippopotamus, hyaena and elephant) and microfossils (freshwater ostracods and mollusca) found in a channel sequence at East Mersea in Essex constrain a terrestrial limiting point (ID 3715) within the modern foreshore at ∼3.2 m OD (Bridgland et al., 1995a; Briant et al., 2012). A further terrestrial limiting point (ID 4064) is present at Bobbitshole, Suffolk, which is the type of site for the Ipswichian interglacial, with the youngest LIG freshwater deposits as indicated by pollen and mollusca at approximately +1 m OD (West and Godwin, 1957; Sparks, 1957). The age of each of these terrestrial limiting sites is constrained by AAR dating of Bithynia tentaculata opercula, with ascription of a MIS-5e interglacial supported by the biostratigraphy (Penkman et al., 2013).
The same AAR dating method (Penkman et al., 2013) is applied to the sequence from Tattershall Castle, Lincolnshire, where a salt marsh pollen sequence of detrital mud and organic silts contains grains of Chenopodiaceae, Plantago maritima and Artemisia, alongside the brackish water ostracods and mollusca Pseudamnicola confuse and Hydrobia ventrosa (Holyoak and Preece, 1985). This allows us to ascribe this site to the salt marsh indicator type and a SLIP of m OD (ID 3736). Further north, on the modern East Yorkshire coast is the only non-estuarine MIS-5e deposit from this region: a chalk boulder beach (Lamplugh, 1881, 1887; Catt and Penny, 1966), overlain by windblown sands from which OSL samples were collected and dated to 120.9±11.8 ka (Bateman and Catt, 1996). Due to the presence of landslide material covering the LIG boulder beach (visited by Natasha L. M. Barlow in 2020) there is some uncertainty as to the exact elevation of the beach (ID 1380, 2.3±2.8 m OD), which had to be estimated from the field diagrams (Bateman and Catt, 1996; Catt and Penny, 1966). Offshore of Norfolk two vibrocores record a transgressive sedimentary succession of sands and silts sat over a unit of glaciofluvial gravel and gravely sand (Paddenberg et al., 2008; Russell and Tizzard, 2011). Silty deposits at ca. −29.54 m OD date to MIS 5 and display a microfossil assemblage dominated by the ostracod Cyprideis torosa and foraminifera Ammonia beccarii, Haynesina germanica and Elphidium oceanis, indicative of intertidal environments (IDs 3432–3434).
4.4 English Channel: southern England
Sea-level data from southern and southwestern Britain include a mix of marine and terrestrial limiting data as well as precise SLIPs. Several important sites along the Hampshire (Briant et al., 2006, 2019) and West Sussex (Bates et al., 2010) coastlines have been dated using OSL, providing an absolute chronology for the terraced sequences of raised littoral deposits along this stretch of the southern British coastline. In West Sussex, the Aldingbourne Raised Beach sequence of marine sands and gravels found at elevations between +17.5 and 27.4 m OD (at Norton Farm and Pear Tree Knap; IDs 3716–3722) dates to MIS 7 based on replicate OSL sampling at multiple sites (Bates et al., 2010). The lower-positioned Pagham Raised Beach sequence of marine sands and gravels has been dated at multiple sites to MIS 5 and in places to MIS 5e (Bates et al., 2010). Its SLIP elevations are around +5 m OD (sites Selsey West Street, Pagham Water Treatment Plant, Chalcroft Nurseries, Warblington and Woodhorn Farm; IDs 3723–3733).
Fluvial deposits found at intermediate elevations between the Aldingbourne and Pagham raised beaches (Solent Breezes, IDs 3734, 3735) and dated to MIS 6 provide important terrestrial limiting data points (Bates et al., 2010). In Hampshire, several sequences have been dated that contain interglacial estuarine organic deposits located between lower and upper marine sand and gravel units (Allen et al., 1996; Briant et al., 2006, 2019). At Lepe, lower gravel units are OSL-dated to MIS 6 and provide terrestrial limiting points (ID 3755, 3757, 3758). The upper gravel units date to MIS 4 (IDs 3753, 3754, 3756), placing the interbedded estuarine deposits in MIS 5 (SLIP IDs 3759, 3760), although a paired date for the estuarine deposits is unavailable (Briant et al., 2006, 2019). OSL dates from overlying gravels in comparable sequences at Pennington Quarry (IDs 3761–3764) place buried estuarine deposits at −4.6 m OD in late MIS 5 (Allen et al., 1996; Briant et al., 2006) and provide terrestrial limiting points. Buried interglacial estuarine deposits associated with the Solent River estuary system are further found on the Isle of Wight in association with the Bembridge Raised Beach (ID 4004, +4 m OD), which is dated with thermoluminescence to MIS 5e (Preece et al., 1990).
Raised interglacial beaches in both Devon and Cornwall (Scourse, 1996; Campbell et al., 1998) are commonly associated within sequences of pebble conglomerate beach facies sitting on top of raised wave-cut platforms and overlain by backshore sand facies that grade into aeolian dune sands. Four sites are included, of which only one (Fistral Beach, Newquay; ID 729) has a geochronology supported by thermoluminescence dating, independent from AAR (Southgate, 1985). That site dates overlying dune deposits (+9 m OD), providing a terrestrial limiting data point. The further three sites (Trebetherick, St Ives Bay, Saunton; IDs 528, 730, 735) each have AAR and stratigraphic information that indicate MIS-5 ages (Andrews et al., 1979; Bowen et al., 1985; Davies, 1983, 1984; James, 1968, 1975, 1995, 2008; Gilbert, 1996; Scourse, 1996; Campbell et al., 1998), yet with low confidence in age quality. These plot as SLIPs at +4.2 to +7.2 m OD (Fig. 5).
The remaining MIS-5-associated data points included for southern and southwestern Britain derive from raised littoral sediments found within cave systems in South Devon (Proctor and Smart, 1991; ID 4008) and South Wales (Sutcliffe and Currant, 1984; Stringer et al., 1986; Sutcliffe et al., 1987; IDs 4005–4007) and on Jersey (Keen et al., 1981; ID 4003). Each site contains speleothem deposits in association with the raised littoral sediments providing robust chronologies based on U-series dating. The Minchin Hole Cave (IDs 4005, 4006) site in South Wales is further supported with luminescence dates (Southgate, 1985). Lastly, offshore in the English Channel, vibrocores dated by luminescence record a fluvial palaeosol (ID 3679) of late MIS-6 age at −42.9 m OD and laminated sand (ID 3678) rich in shell fragments and shallow marine foraminifera (Elphidium sp. and Ammonia sp.) of MIS-5 age at −48.8 m OD (Mellett et al., 2012, 2013).
4.5 English Channel: northwestern France
The most common sea-level indicators on the French side of the English Channel are marine terraces and raised beaches. These features have been described from western Brittany to eastern Normandy as part of a staircase of coastal platforms with each step attributed to different interglacials (Coutard et al., 2005, 2006; Pedoja et al., 2011, 2014, 2018). The lower level is generally ascribed to the LIG, and its age has been constrained by dating the deposits sat on top of the platforms (mainly heads, loess and beach sediments) or by the identification of Palaeolithic artefacts (Mousterian industry, Cliquet et al., 2003, 2009; Cliquet, 2013; Regnauld et al., 2003; Lautridou and Cliquet, 2006). Absolute ages are available at a limited number of locations, and the rest of the sites are regionally correlated based on the developed stratigraphic frameworks (van Vliet-Lanoë et al., 2000; Bates et al., 2003; Regnauld et al., 2003; Monnier et al., 2011; Pedoja et al., 2018).
Marine terraces are generally found between 0 and +8 m NGF in mainland France (e.g. Trez Rouz, ID 3677; Cap La Hague, ID 3674; Asnelles, ID 3672) and in different islands distributed along Brittany's west coast (e.g. Belle Île, ID 3667; Ouessant, ID 3658; Chausey, ID 3668). In Brittany, dune sands from deposits overlying an abrasion platform at Du Guesclin (ID 3659) were dated by Regnauld et al. (2003) providing a minimum age of 90 ka, and artefacts attributed to early Palaeolithic age were found overlying an equivalent platform at Le Verger (Regnauld et al., 2003; ID 3660). Absolute ages pointing to MIS 5 were also obtained from raised beach deposits at +8 m NGF at Piégu (ID 3657) by electron spin resonance on quartz grains (Bahain et al., 2012). Normandy shows the highest density of LIG sites, particularly around the Cotentin peninsula, including a suite of sea-level index points and marine and terrestrial limiting data. Sandy beach deposits that are found between 4 and 7.9 m NGF (IDs 3550, 3551, 3646, 3651, 3671) have been dated by OSL and TL to MIS 5, probably MIS 5e (Folz, 2000; van Vliet-Lanoë et al., 2006; Coutard et al., 2006; Cliquet et al., 2009), while aeolian sands (mainly dunes; IDs 3536, 3549, 3648) and marine deposits (ID 3552) provide ages for marine and terrestrial limiting points. Further east, in Le Havre, beach sediments found at −1 m NGF (ID 3676) have been interpreted as MIS-5e regressive deposits based on its stratigraphic position (Breton et al., 1991; Lautridou et al., 2003), but numeric ages are not available.
The NW Europe database (Cohen et al., 2021; V2: 2022: https://doi.org/10.5281/zenodo.6478094), as a scientific product, is open-access. The data points used in this study were compiled and contributed to WALIS by the authors, and in various ways the entries cross-refer to (i) governmental databases (with public portals, but their contents are not open-data or open-access in the academic output sense) and (ii) tabulated and graphed data contained in recent and legacy literature (a great majority of which is web-disclosed, not in all cases open-access, and DOI-referenced where appropriate). The files at this link were re-exported from the WALIS database interface on 19 April 2022. A description of each field in the database is contained at https://doi.org/10.5281/zenodo.3961544 (Rovere et al., 2020), readily accessible and searchable at https://walis-help.readthedocs.io/en/latest/ (last access: 30 April 2022). More information on the World Atlas of Last Interglacial Shorelines can be found at https://warmcoasts.eu/world-atlas.html (last access: 30 April 2022). Users of the database are encouraged to cite the original sources along with our database and this article.
This database presents 146 LIG data points from NW Europe, standardised to the format and structure of WALIS and taking into consideration VLM, compaction and elevational uncertainties where possible to provide a comprehensive overview of LIG sea level in the region. The history of research in the region presents a wealth of data and opportunities but also presents some interesting challenges that users of this database should take into consideration and provides stimulus for future research.
6.1 Chronostratigraphical challenges
Near-field sites often include temperate and high-latitude estuarine sequences (e.g. salt marsh), which due to their close relationship with tidal levels have the potential to provide accurate and precise vertical constraints on RSL (Shennan et al., 2015). The main challenge with such data points is not vertical, but temporal imprecision and independent dating (amongst others because the timeframe is outside the reach of radiocarbon dating). This is a challenge of any LIG sea-level database. Even in low-latitude regions where absolute U-series dating can be applied to palaeo-corals, debate on the age constraints remains (e.g. Chutcharavan and Dutton, 2021). In our study area, the MIS-6 glaciation limit (Fig. 1) divides the study area and the dataset quite evenly (N=74 within the glaciation limit, N=73 outside). For locations within the Saalian ice limit, the lower stratigraphic units left by the ice provide a strong maximum chronological constraint. Therefore the main chronological challenge in this area is determining the absolute ages for the successive Eemian pollen zones (Table 4, Appendix B). This is especially relevant if rates of sea-level rise and fall during parts of the interglacial are to be resolved and analysed and if one tries to investigate the extent to which LIG developments (climatological, sea-level history, coastal depositional) are analogous to Holocene situations and anticipated futures. This high-resolution chronostratigraphical issue remains intertwined with other challenges, discussed in sections below.
Outside of the ice margin, the limited lithostratigraphic controls means that assigning a pollen biozone to one specific interglacial is difficult. Although recent advances in AAR dating has led to some progress to resolve this problem, it has still resulted in several ambiguous sites not being included in our compilation (Sect. 4.3). The chronological particularities and uncertainties in the North Sea region affect the basin subsidence corrections and graphical comparison of WALIS data against earlier work and syntheses. Users of this database must be mindful of these regionally specific chronological challenges, which are discussed further in the Appendix B. There also remains considerable opportunities to revisit many interglacial sites and to apply now established sea-level research protocols (Shennan et al., 2015) and spatially intensive geochronology sampling strategies that, if combined with statistical (e.g. Bayesian) frameworks, could greatly advance knowledge and data precision across this region.
6.2 Vertical land motion over- and underestimation
In the North Sea area we provide estimates of the rates of VLM based upon independent geological mapping (Sect. 3.3), presented as an average rate calculated over the last 2.6 Myr (mapping of base Quaternary). There is a strong indication (analysis in Barthes et al., 1999; Kuhlman et al., 2006a, b; Arfai et al., 2018) that the 2.6–0 Ma averaged subsidence rate that Kooi et al. (1998) reported is the combined result of a phase of very strong sediment-loading subsidence induced between 2.6 and 1.8 Ma (rates considerably higher than average), followed by much reduced subsidence after 1.8 Ma (rates below average, estimated at 80 %). Additional changes in the rate of basin subsidence before and after 1 Ma may also be speculated on but for that timeframe are hard to spatially quantify and calculate independently of GIA. For the 2.0–1.5 Ma timeframe, it may be possible in the future to map “base Olduvai” consistently across the depocentre and use that to quantify VLM rates for the youngest 1.8 Ma. Awaiting such analysis, one could decide to regard presently included VLM rates (Fig. 3) as maximum subsidence rates and opt to lower them by 80 % as an advised minimum rate. Doing so would result in a lowering of the reported VLM-corrected palaeo-sea-level elevation (Fig. 4, Sect. 4.1) by some 5 m in offshore depocentre locations and by some 2 m for coastal sites.
For the English Channel coasts and the English side of the North Sea, our database provides no VLM rates and assumes these as generally smaller than +0.04 m kyr−1, with the local West Sussex exception of possibly up to +0.12 m kyr−1. These are clear maximum values, and it is important to compare these to regional GIA modelling outputs to assess the amount of vertical motion that glacio- and hydro-isostatic processes could explain. So far, the studies addressing long-term land-level change and those addressing GIA during interglacials have mostly been separate work, and resolving this was beyond the scope of a database-filling project. A future dedicated study should seek to separate out non-GIA and GIA VLM in the region and then add non-GIA correction to the WALIS entries along the English Channel.
6.3 Comparison to older interglacials
The current version of the NW Europe database contains a modest number of older sea-level data points (Fig. 1 and Table 2): five SLIP sites along the North Sea (Morton, Norfolk UK; Sangatte, NW France; Kellen, Belgium; Noorderhoeve and Ameland in the Netherlands; IDs 4063, 3665, 347, 895, 429) and along the Channel the OSL-dated Aldingbourne Raised Beach (West Sussex, UK; see Sect. 4.4). All of these are attributed to MIS 7 (Gale et al., 1988; Hoare et al., 2009; Balescu et al., 1991; Bogemans et al., 2016; Meijer et al., 2021) and likely represent RSL highstand positions. The indicators occur in a raised position along the English Channel, East Anglia and also in the Dover Strait (Sangatte) and in a subsided position in the Netherlands (VLM rates; Sect. 3.3). The Belgian site is from the Yser valley and a setting similar to the LIG counterpart Woumen (Bogemans et al., 2016; ID 346). The Morton site is a beach deposit with no clear nearby counterpart in East Anglia. Sangatte is also without a preserved LIG counterpart, likely due to Holocene highstand erosion (Sect. 6.5). The Dutch sites (Meijer, 2002; Meijer et al., 2021; IDs 429, 895) are from estuaries overridden by the MIS-6 glaciation. In terms of depositional environments, they are similar to LIG and Holocene settings, but in terms of geographic position and orientation they are dissimilar. Geological survey investigations in the northern Netherlands (Bosch, 1990, and later and ongoing work) reveal a complex of glaciogenic (older than MIS 6), estuarine and fluvial deposits, including regionally traceable peat and shallow intertidal levels, similar in degree of preservation to those from the LIG. This will certainly provide opportunity to add older-interglacial RSL data points from this region in the near future, contributing to insights in both RSL rise and maximum positions during the Middle Pleistocene. The series of MIS-7 and older attributed sites along the Thames Estuary (see Appendix B) has not been entered due to limited chronological constraints. Our compilation efforts focused on the LIG; however we do recommend that WALIS is expanded with entries for older interglacials.
6.4 Comparison to Holocene sea-level indicators
There is a long history of research into Holocene RSL sea-level indicators from this region, in particular in the UK and the Netherlands (Shennan, 1989; Flemming, 1982; van de Plassche, 1982), the databases of which provide the foundation for the design of modern RSL databases (Hijma et al., 2015). For recent datasets, we refer to Vink et al. (2007) and to HOLSEA regional publications (Shennan et al., 2018; Hijma and Cohen, 2019; Rosentau et al., 2021; Bungenstock et al., 2021). This wealth of information has provided important insights into our data review for the LIG, in particular when defining the indicative meaning (Shennan, 1986; van de Plassche, 1986) where we have documented the SLIP types in use for LIG sea-level reconstruction in estuaries, barrier-lagoon systems and glacially inherited embayment systems, particularly along the North Sea.
It is a different matter, however, to consider the LIG RSL change directly analogous to Holocene counterparts. Comparison requires differential correction of VLM (Sects. 3.3 and 5.4), which in turn is dependent on age attribution (Sects. 2.3, 5.1 and Appendix B) and the degree of analogy drawn between Holocene and LIG. The spatio-temporal pattern of deglaciation of the NW European ice sheets over Termination II vs. Termination I is a key driver of differences in RSL between the Holocene and LIG (e.g. Lambeck et al., 2006; Long et al., 2015) and may even help constrain the chronologies (Appendix B). Our data entry in WALIS and this paper prepare for and recommend (Sect. 6.5) but do not execute LIG-to-Holocene intercomparisons.
6.5 Near-field importance in resolving global Last Interglacial sea levels
The main foreseen use of this database from the near-field region of the European ice sheets is in GIA modelling and analysis of ice melt fingerprints. The pattern and magnitude of RSL change at near-field sites, proximal to existing or former ice sheets, are strongly dependent on the magnitude and timing of the change in ice load and the relaxation characteristics of the underlying mantle (Farrell and Clark, 1976; Yokoyama and Purcell, 2021). Therefore, near-field sea-level observations have the power to resolve ice sheet histories and changes in the solid Earth that far-field data do not, as has been extensively demonstrated with near- and intermediate-field Holocene RSL data (e.g. Lambeck, 1995; Peltier, 2002; Bradley et al., 2011; Engelhart et al., 2011; Long et al., 2011) and has also been explored before in the LIG (Lambeck et al., 2006). The relative density of data in NW Europe, over a transect away from the Saalian ice sheet margin (Figs. 4 and 5), means RSL data from this region have the potential to reconstruct the magnitude and deglaciation of the MIS-6 Eurasian ice sheet, which is currently poorly constrained and important for both near- and far-field LIG sea level (Rohling et al., 2017; Dendy et al., 2017). Near-field sites can also take advantage of the sea-level fingerprint of ice sheet mass balance changes to constrain the source of ice sheet melt (Tamisiea et al., 2001; Mitrovica et al., 2009). Ongoing debate suggests asynchronicity between the timing of the contribution of the Greenland and Antarctic ice mass loss to LIG barystatic sea level (Rohling et al., 2019; Turney et al., 2020), a hypothesis which near-field RSL data can test (Kopp et al., 2009; Hay et al., 2014; Long et al., 2015).
One of the challenges of near-field RSL data is that regional GIA is a dominant component of the overall sea-level signal. This does in turn present advantages as alongside long-term VLM, GIA can provide the accommodation space for the accumulation and preservation of (near-)continuous late Quaternary sedimentary packages (e.g. Eaton et al., 2020). Interglacial RSL highstands will occur earlier in the far field than the near field due to solid Earth processes, with the initial transgressive phase being relatively slow and prolonged and therefore having the potential to capture fluctuations in RSL (Cohen et al., 2012; Long et al., 2015). At the end of an interglacial, RSL fall in the near field is relatively marked as changes in ocean volume due to growth of ice sheets of the commencing glacial phase outpace regional GIA. Improving the quality of LIG SLIPs needs to be an area of intensive research as near-field RSL constraints from pervious warm periods are essential to identify the sources and forcing mechanisms responsible for sea-level change (Dutton et al., 2015).
6.6 Future data collection directions
The records in the NW Europe database typically cover the middle of the Eemian interglacial as the latter phases of RSL rise resulted in regional highstands with evidence preserved relatively inland, mapped onshore and in shallow marine boreholes. By comparison records of RSL change during the early interglacial are more limited and widely spatially distributed, with the logistic difficulties of collecting LIG RSL data offshore, compounding uncertainties in VLM corrections (Sect. 6.2). To improve RSL data coverage for the early Eemian, targeted collection of a series of transgressive SLIPS from along submerged Saalian palaeorelief off the Dutch coast (see geomorphological context in Cartelle et al., 2021) has been carried out (ERC RISeR project; Barlow and affiliates) and is anticipated to append the current dataset. For similar accessibility issues, evidence of falling sea level and RSL oscillations in the remainder of MIS 5 (Table 1) is much more limited than that for the middle Eemian. Nevertheless, areas with offshore borehole records testify for marine conditions up to and after the end of the LIG and offer the potential to provided SLIPs and limiting data points from the MIS-5c and MIS-5a sub-stages (Table 1) and potentially even from MIS 3. Increasing the number of data points from these periods requires a targeted data collection effort to resolve the RSL signals towards the anticipated minima of MIS 4 and eventually MIS 2 (LGM; Table 1).
The potential offshore record from the Late Pleistocene is not restricted to terrestrial limiting data from fluvial settings alone but also includes marshy deltaic and shallow marine strata. The Southern Bight, where new generations of seismic surveying instruments and analysis capacity are used for targeted vibrocoring of submerged landscape features (e.g. Missiaen et al., 2020), offers SLIP potential. In this region, falling-stage sea-level research links up with interests in Neanderthal and Mesolithic archaeology (e.g. Hijma et al., 2012) and with intensifying human activity in the nearshore for wind farm construction and dredging for coastal nourishment and seaward harbour extensions (e.g. Cartelle et al., 2021), adding to the potential wider research. OSL dating also in such offshore settings has been demonstrated to offer a viable dating technique (e.g. Mellett et al., 2012, 2013; De Clercq et al., 2018). The potential for post-Eemian MIS-5 RSL data points also extends to parts of the North Sea coastal zone, owing to river valley palaeogeography and dissection (Figs. 1 and 2) and VLM subsidence (Fig. 3). Along the western rim of Holland, for example, basal parts of post-Eemian estuarine and fluvial–tidal channels appear preserved below MIS-4–MIS-2 Rhine–Meuse deposits (Törnqvist et al., 2003; Wallinga et al., 2004; Busschers et al., 2005, 2007; Hijma et al., 2012; Peeters et al., 2016), with MIS-5 SLIP and limiting data point potential.
On land, new data collection need not be constrained to finding new sites. Many sites from the older literature are still possible to revisit, and it is possible to collect new data from them to upgrade the quality of the chronologies and elevation constraints. Such work has been carried out with a focus on older late Quaternary interglacials in southern and eastern England (iGlass project NE/I008675/1; Long et al., 2015; Barlow et al., 2017); re-coring and resampling at Amersfoort in the Netherlands (e.g. Kasse et al., 2022); and re-investigations at Tønder (Friborg, 1996), Dagebüll (Winn et al., 2000) and Norderney (Schaumann et al., 2021) in Danish–German borderlands and the Wadden Sea. Choices of which legacy sites to revisit should be guided by outstanding research questions, e.g. constraining important spatial gaps for GIA modelling, instead of returning to classic sites that have been well studied.
There is a long history of palaeoenvironmental and sedimentological research in NW Europe, dating back to the 19th century. However, much of this work predates modern standards for sea-level databases and therefore is of variable quality and application for the WALIS database.
North Sea: the Netherlands onshore and offshore
A key paper on LIG sea-level data points in the southern North Sea and the onshore Netherlands is Zagwijn (1983), which importantly also informs relative age control within the interglacial by regional pollen assemblages (“pollen association zones”, PAZs) of know durations (PAZs E1–E6; Table 1 in main text and discussed below), which is applied extensively in NW European settings. The non-GIA-corrected sea-level record encompasses a period of rising RSL (>20 m within 1000 years early in the Eemian), a slowdown and highstand (over ca. 6000 years, in the middle of the Eemian), and a sea-level drop during the late Eemian (ca. 4000 years, MIS-5e–MIS-5d transition) and beginning of the Weichselian (MIS 5d). These classic data points (highlighted in Fig. A1a) have been included in many later global compilations (e.g. Kopp et al., 2009). Because the absolute numeric age of the Eemian pollen zone chronology remains debated (see Appendix B), different authors have used and placed the start of the RSL curve at different points, from ∼130 to ∼120 ka (discussed further in Long et al., 2015).
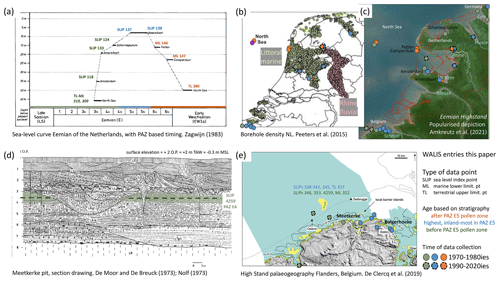
Figure A1Selected figures illustrating research history and database contents for the Netherlands (a–c) and Belgium (d–e), with annotations (database IDs, grouping early-, middle- and late-LIG points). (a) Sea-level curve of Zagwijn (1983). (b) Borehole density for the Netherlands (Peeters et al., 2015) overlain with legacy and more recent RSL data points. (c) Popularised depiction of Eemian highstand (Doggerland exhibition, Rijksmuseum voor Oudheden, Leiden; Amkreutz et al., 2021). (d) Exposure drawing of pit Meetkerke, Belgium (De Moor and De Breuck, 1973); elevation of SLIP highlighted. (e) Palaeogeographical map for Eemian highstand in Flanders for a series in De Clercq et al. (2018). Panels reproduced with permission (a: Stichting NJG; b: Elsevier; c: RMO/Odé; d: present holder unknown, archived by https://www.vliz.be, last access: 1 November 2021; e: Wiley).
In the late 1980s, offshore investigations by geological surveys from all North Sea countries revealed the location of the initial Eemian RSL rise offshore the northern Netherlands (Sha et al., 1991; Beets et al., 2005; site BH89/2). New data points also became available from the onshore NE Netherlands, from geological survey mapping campaigns (e.g. Bosch, 1990). In the 1990s, the infill and setting of the Amsterdam and Amersfoort basins were subject of detailed multi-proxy studies, aiming to resolve the history of the Saalian deglaciation and the Eemian interglacial optimum (Van Leeuwen et al., 2000; Cleveringa et al., 2000), though the focus was more on chronostratigraphy, palaeoclimatology and general depositional history than improving reconstruction of past sea level. A cross-section from the centre of the Amsterdam basin to the southeastern rim (De Gans et al., 2000) confirmed the presence of intertidal deposits from the Eemian highstand, echoing Zagwijn (1983) observations. At this time, amino acid racemisation (AAR) dating of marine mollusca shells (Miller and Mangerud, 1985) confirmed the Eemian age of the offshore and onshore mollusca-bearing beds recorded in Zagwijn (1983).
Since the late 1990s, investigations concentrated on the sedimentary development and chronostratigraphy of the river Rhine. Initially, this focused on areas south of the Saalian maximum ice limit (Törnqvist et al., 2003; Wallinga et al., 2004; Busschers et al., 2005, 2007; Hijma et al., 2012), where falling RSL erosion and MIS-4–MIS-2 lowstand fluvial reworking dominated, and hence no Eemian sea-level constraints are preserved (Fig. 1 and Table 2 in the main text). Coastal deposits onlap Saalian glaciogenic landforms and river valleys or the floors and rims of valleys that dissected such landforms. OSL dates from within the Late Saalian and Eemian units confirm their deposition occurred between 140–110 ka (Busschers et al., 2007; Peeters et al., 2016). The river Rhine significantly shifted course during the Late Pleistocene, from its northerly route occupied during the Saalian deglaciation and throughout the Eemian to the southerly course that it shares with the rivers Meuse and Scheldt. This shift explains the absence of preserved Eemian coastal sites in the subsurface of today's Rhine–Meuse delta immediately south of the Saalian glacial limit. In the last 10 years, the northerly Rhine course and Eemian estuary have received significant research attention (Sier et al., 2015; Peeters et al., 2016, 2019), yielding new sites at Rutten and Oosterwolde with sea-level indicators from relative inland lagoon rims (Peeters et al., 2016; Fig. A1b–c).
North Sea: Belgium, Southern Bight
LIG coastal intertidal deposits in Belgium are encountered at shallow depths along the inland rim of the modern coastal plain, typically separated from Holocene equivalents by periglacial deposits of Last Glacial age. From the NW of Belgium, the monograph of Heyse (1979) provides a series of pit exposures, describing the sedimentology and palynology (using the PAZ E1–E6 scheme, as in the Netherlands). Further LIG deposits from estuarine environments are associated with valley fills underlying the Flanders coastal plain, the main one cut by the river Scheldt in the Middle Pleistocene (Flemish Valley; Gibbard, 1988; De Moor and Pissart, 1992) and in the southwest a second valley of the same broad age cut by the Yser river (Bogemans et al., 2016). De Moor and De Breuck (1973) and Nolf (1973) describe the tidal sedimentology and marine mollusca of pit site Meetkerke (Fig. A1d), west of Bruges. Mathys (2009) and De Clercq et al. (2018) mapped the onshore–offshore continuation of these valley systems, the former with morphostratigraphic attention (intersection of Last Glacial dissected phenomena of the Dover Strait; Bridgland and D'Olier, 1995; Gupta et al., 2007) and the latter with particular attention to palaeogeography during phases of RSL rise and fall during the LIG (Fig. A1e).
Compared to the relatively straight present-day coastline, the Eemian coastline is considered to be more irregular, with Paleogene clay hilly outcrops forming capes and islands. The cluster of sites documented by Heyse (1979) occupies a position just north of a clay outcrop. River valleys functioned in the valleys before and after the LIG. As in many lower-reach valley settings, the falling and lowstand RSL of the Last Glacial induced considerable erosion of the Belgian LIG valley fills. Onshore, highstand deposits are only preserved locally along valley rims. Near the modern coastline, early transgressive units are preserved locally below erosive contacts. During the Last Glacial, the Scheldt river established new northwestward to northward valleys into the SW Netherlands (Vandenberghe, 1985), further explaining the degree of preservation of LIG deposits in the NW of Belgium and spatial distribution of WALIS entries.
North Sea: German Bight, NW Germany, SW Denmark
LIG coastal deposition in and along the German Bight accumulated and preserved in very similar settings to those in the central and northern Netherlands. The research history starts with boreholes at Tønder (Madsen et al., 1908) and Højer (Nordman, 1928) in the Vidå valley. The area lay outside Scandinavian ice sheet coverage of the Last Glacial, though outwash produced along that margin buried and eroded the Eemian coastal record. Eemian sediments overlay and infill landforms generated during the Saalian glaciation, deglaciation, readvance (Drenthe-2 and Warthe morainic lines; Fig. 1 and Table 1) and (glacio-)fluvial dissection (conduits of Vidå, Eider, Elbe, Weser and Ems). Miller and Mangerud (1985) resampled sites from along the Danish Eemian North Sea. Höfle et al. (1985) and later Streif (2004) summarised evidence around the German Bight, linking up to Dutch, Belgian and English North Sea coastal sectors and providing comparison with older interglacials and the Holocene. Konradi et al. (2005), in their paper entitled Marine Eemian in the Danish eastern North Sea, provide palaeogeographical details for an inventory of sea-level indicator sites. They aptly characterise the Danish Eemian shoreline as “more irregular than present”. Some of the sea-level data points are reported from environments resembling those of the modern Wadden Sea, whereas others come from what would better be called “fjords” and “straits” cut in the Quaternary glacial and river-lain substrate of the freshly exposed glacial landscape at the onset of Eemian transgression. A palaeogeographical particularity is a W–E marine highstand passage between the German Bight and the SW Baltic Sea across German–Danish borderlands (Fig. 1), unlike the peninsular present situation (Winn and Erlenkeuser, 1995; Konradi et al., 2005). Such a connection is suspected from geological mapping of the German Bight Eemian highstand (e.g. at Schnittlohe, ID 889, N Germany; Menke, 1985; Knudsen, 1985) and from biotic changes in the SW Baltic regionally extensive Cyprina clay marine beds (Kubisch and Schönfeld, 1985; Funder et al., 2002), although the supposed connection would have been shallow and is not fully traced and hence not evident (Miller and Mangerud, 1985; Kosack and Lange, 1985; Schulz et al., 2001 – op cit. Konradi et al., 2005).
RSL rise inundated extensive areas of the German Bight Saalian glacially derived push moraine and till plateau islands (dubbed “Bakkeøer”), which were subsequently hidden by LGM and deglacial sediments and subsidence. Between the Bakkeøer island series and the Danish mainland a retreat-stage meltwater plain is present which was inundated by RSL rise (research background in Konradi et al., 2005, p. 24). Southwest of the Bakkeøer islands and Warthe limit lay the Elbe valley, an ice-marginal river during the Warthe ice limit and the LGM, which formed an Eemian estuary preserving LIG sea-level indicators recorded by Menke and Tynni (1984), Menke (1985), and Streif (1990, 1991). West of the Elbe valley and estuary, a secondary morainic limit exists (Fig. 1; e.g. Ehlers et al., 2004), and the associated lows host Eemian and Early Weichselian terrestrial deposits (Selle and Schneekloth, 1965; Behre et al., 2005; key sites Oerel and Glinde). The northernmost, deepest sites also preserve evidence of marine incursions (Streif, 1990, 2004). Further west, the till plateau of East Frisia and minor valleys set into it host Eemian coastal deposits (Dechend and Sindowski, 1956; Höfle et al., 1985; Schaumann et al., 2021), similar to sites further west along the Wadden Sea in the Netherlands.
Age control of the NW German and SW Danish sites is largely based upon pollen investigations on terrestrial beds immediately below and above tidal coastal deposits and contained in the brackish and saline deposits, resolving broad-scale vegetation succession (i.e. in Danish and German origin PAZ schemes, tightly correlated to the Zagwijn E1–E6 scheme; e.g. Funder et al., 2002; see Table 4 and Sect. 2.3). Supporting luminescence age control exists (e.g. Murray and Funder, 2003; Buylaert et al., 2011). Like in the Dutch sector, the basal Saalian deglacial geomorphological means identifying LIG from older interglacial deposits is relatively simple. Importantly, no inland fluvial-estuarine sites are known for the German Bight: falling RSL and lowstand erosional activity during the last glacial cycle appears to have mostly removed expected Eemian morphostratigraphic records from along the valleys of the Elbe (which also routed the aforementioned LGM glacio-fluvial outwash) and Weser (Höfle et al., 1985). This is in contrast to the records in the Rhine and Scheldt in the Dutch and Belgian LIG North Sea coastal segments where valley rerouting promoted preservation and may be regarded as similar to the situation in the Meuse (the Netherlands) and Thames (England) North Sea LIG estuaries (Fig. 1).
North Sea: Thames Estuary, East Anglia, northern England
The stratigraphy of the Thames region of eastern England has been extensively studied and documented from the 19th century as the development of London and the surrounding area exposed multiple phases of cold-riverine and warm organic interglacial sediments, many with marine and freshwater micro- and macrofossils. The discovery of Eemian Hippopotamus remains during excavations of Trafalgar Square in the 1950s (Franks, 1960) garners continued wider attention, but numerous less publicised investigations have identified previous interglacial brackish, coastal and shallow marine sediments (e.g. Abbott, 1892; Hinton and Kennard, 1901; Sparks and West, 1963; Gibbard, 1985; Preece, 1999). Similarly in East Anglia, local site investigations document the presence of brackish or marine mollusca at several sites corresponding to the “Ipswichian” (Eemian) interglacial based upon pollen stratigraphy, for example at March (Whitaker et al., 1893) and Wretton (Sparks and West, 1970). Further north, evidence of LIG sea level is sparser, with much of it removed by the subsequent Last Glacial (Devensian) ice advance along the coast of northeastern England and Yorkshire (Catt, 2007; Sutherland et al., 2020), and is largely restricted to the Sewerby Raised Beach, East Yorkshire (Lamplugh, 1887; Catt and Penny, 1966; Bateman and Catt, 1996), which is now buried under a landslide.
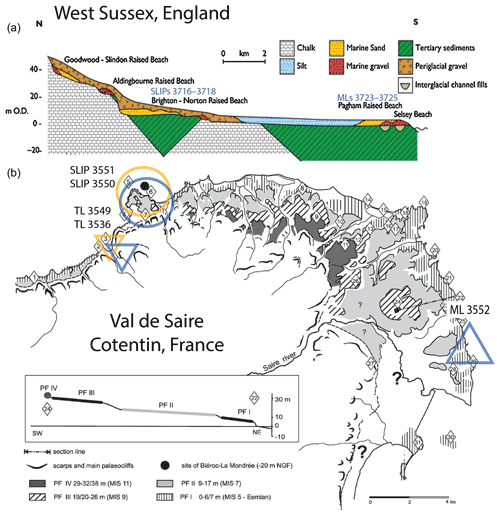
Figure A2Selected figures illustrating research history and database contents for southern England (a) and northwestern France (b), with annotations (database IDs, SLIPs, marine limiting ML or terrestrial limiting TL). (a) Schematic section of raised beach series (Bates et al., 2010), with SLIP-producing and ML-producing sites annotated. (b) Wave-cut platforms (PFs) as mapped for NE Cotentin (Coutard et al., 2006), associated with the PF I level. Panels reproduced with permission (a, b: Elsevier).
A major challenge is that interglacial sites from England, unlike in the Dutch–German–Danish contexts, where Saalian glaciogenic landforms and substrates make it possible to separate LIG from older interglacial sites, often appear in ambiguous stratigraphic positions. Where palynology and mollusca were identified to be from warm periods and near-coastal environments, the sites lack independent dating, and pollen sub-stages of the “Ipswichian” and the preceding “Hoxnian” interglacials have very similar vegetation profiles (Thomas, 2001; Turner and West, 1968). Attempts to correlate seven – then termed “Ipswichian” – estuarine sequences from the Thames, alongside those from East Anglia, based upon pollen sub-stages and their relative elevations, resulted in a paper by Hollin (1977) identifying the potential for a double LIG sea-level highstand. Though in keeping with more recent hypotheses (e.g. O'Leary et al., 2013), significant advances in AAR methods (Penkman et al., 2013), combined with Thames terrace stratigraphy (Bridgland, 1994), vertebrate and mollusca biostratigraphy (Schreve, 2001; e.g. Preece, 2001), and independent geochronology (e.g. OSL), have since shown that many British “Ipswichian” sites actually date from the preceding three marine isotope stages (Penkman et al., 2008, 2011, 2013). Trafalgar Square is the only site in the analysis of Hollin (1977) analysis which is still regarded to be of LIG age.
English Channel: southern England
LIG deposits are found along the length of the southern coastline of Britain, typically as part of expansive sequences of raised littoral terraces from the Pleistocene. Occurrences of Pleistocene deposits that formed during interglacials are well documented across all southern coastal counties (Prestwich, 1892) in early literature, from Kent in the southeast (Mackie, 1851), through Sussex (Godwin-Austen, 1857; Prestwich, 1859; Reid, 1892), Hampshire (Reid, 1893) and Dorset (Weston, 1852; Prestwich, 1875), to Devon and Cornwall (De La Beche, 1839; Ussher, 1879) in the southwest. Pleistocene littoral deposits occur around Lands End (the southwesternmost point of mainland Britain) and can be found on the northern coastlines of Cornwall and Devon, the east coast of Somerset (Woodward, 1876), and from there across the southern coastline of South Wales (Murchison, 1868; Prestwich, 1892). Unsurprisingly, the early literature associated with these widespread deposits extends to include raised littoral deposits on the main island groups in the English Channel: the Isle of Wight (Godwin-Austen, 1855; Prestwich, 1859), the Isles of Scilly (Barrow, 1906) and the Channel Islands (Zeuner, 1946).
Early literature typically relied on the identification of mammalian and molluscan faunas as well as pollen assemblages and successions to attribute deposits to successive interglacials. The elevations and relative positions of littoral terraces (typically, raised beach deposits) are also used. The relative elevations of deposits also became a useful tool for tracking contemporaneous raised littoral terraces across wider geographical areas (Palmer and Cooke, 1923; Arkell, 1943; Mitchell, 1960; Orme, 1960; West and Sparks, 1960; Hodgson, 1964). A commonality that emerged was that the Ipswichian (Eemian/MIS 5e) interglacial was likely associated with the lowest terrace of deposits, often reported as a “15 ft raised beach” (ca. 4.5 m O.D.) (Fig. A2a). However, association with the preceding interglacial (MIS 7) was hard to rule out, especially given the presence of multiple, distinguishable low-level raised beach deposits at several locations (Davies and Keen, 1985; Mottershead et al., 1987; Bates et al., 1997). Dating attempts often remained inconclusive and debated (Mitchell, 1972; Bowen, 1973). Unfortunately, despite the development and application of novel quantitative dating approaches during and since the 1980s, many low-level raised littoral deposits in southern and southwestern Britain still lack confident and precise age determinations (see Appendix B).
English Channel: northwestern France
The northwestern coast of France, from Brittany to the Straits of Dover, is characterised by the occurrence of a staircase of polygenic coastal platforms that were correlated with Pleistocene interglacial periods. These platforms are intermittently covered by a variety of coastal (mainly raised beaches) and periglacial deposits (heads and loess) that have been studied for more than a century (Barrois, 1877, 1882; Bigot, 1885, 1930; Pellerin and Dupeuble, 1979; Lautridou et al., 1999; Regnauld et al., 2003; Coutard et al., 2005, 2006; Cliquet et al., 2009; Pedoja et al., 2018). The absence of well-preserved fossiliferous remains in most of these sites gave rise to an extensive discussion about their chronostratigraphy, and the age was generally established based on the altitude of the beach deposits relative to other sites. In the Armorican Massif, two levels of marine deposits, between 0–5 and 15–20 m above sea level, were attributed to the LIG (Elhaï, 1963), while Morzadec-Kerfourn and Monnier (1982) attributed the three lower levels, found between 0–15 m in Brittany, to three distinct marine transgressions, probably MIS 9 to MIS 5.
The discovery and excavation of Palaeolithic sites along the coast and the progressive application of thermoluminescence (TL), optically stimulated luminescence (OSL) and electron spin resonance (ESR) geochronology (e.g. Balescu et al., 1991; Balescu and Lamothe, 1992; Loyer et al., 1995; Folz, 2000; Coutard et al., 2006; Cliquet et al., 2003, 2009; Monnier et al., 2011) boosted the understanding of the coastal sequences and helped to better constrain the distribution of the LIG deposits. Loyer et al. (1995) suggested that up to four different interglacials were preserved in the same coastal platform in Saint-Brieuc Bay (Brittany) based on palaeoenvironmental data and TL dating of loess deposits, later further supported by ESR dating of Palaeolithic sites (Bahain et al., 2012). TL dating of burnt flints in Port-Racine (Normandy) attributes beach deposits at 3 m to the LIG (Cliquet, 1994, Lautridou et al., 1999). Coutard et al. (2006) OSL-dated several marine, beach and dune deposits in Val de Saire (Normandy; Fig. A2b) pointing to the MIS 5e to MIS 5d. Despite the fragmentary nature of the deposits and the relatively small number of absolute age determinations, there were also some attempts to reconstruct the LIG coastline in Normandy (Regnauld et al., 2003) and to establish regional stratigraphic correlations across the English Channel (van Vliet-Lanoë et al., 2000; Bates et al., 2003). Deposits corresponding to the LIG appear better preserved in Brittany and western Normandy (Fig. A2b), where the local geomorphology protected these sediments from later erosion; however, further east Pleistocene beach deposits were eroded in younger interglacials and the Holocene (van Vliet-Lanoë et al., 2000; Bates et al., 2003; Regnauld et al., 2003). As an exception, at Sangatte in NW France a cliff foot beach (De Heinzelin, 1966) that predates the LIG (Balescu and Haesaerts, 1984; Haesaerts and Dupuis, 1986; Balescu et al., 1991) is preserved under colluvium, re-exposed by Holocene erosion.
One of the challenges of any LIG sea-level database is the chronostratigraphic constraints as developing chronologies for Pleistocene timeframes is outside the reach of radiocarbon dating. In the structure of the WALIS database we have included entries for the Eemian pollen association zones (PAZs) under “chronostratigraphy” (Table 4 in main text) and have used this to encode the relative dating of LIG deposits at Dutch, Belgian, German and Danish sites. Appendix B provides background on how the particular table stores the correlative numeric age constraints for these entries, on where the upper and lower ages communicated in Table 4 come from, and on time control on LIG data points for the English and French part of the study area.
How WALIS stores correlated numeric ages with chronostratigraphical entries
To make WALIS database usage not too dependent on positions regarding timing and duration of the NW European Eemian (see main text and next section), the design of this section of the database (Rovere et al., 2020: “Strat” table) allows for and requires the filling of four duration and timing database fields. Table 4 (main text) lists these four. Two of these deal with the locally established, varve-counting- and palynological-correlation-based durations of the respective chronozones (Müller, 1974; Zagwijn, 1996) and their uncertainty. These durations are regarded minimum durations in some numeric age attribution schemes (see main text and below) and have been used as more strict constraints in others. The two other fields then store an upper- and lower-limiting numeric age, of which the values have to be based on correlations to numerically dated sequences elsewhere, such as in SW Europe (Tzedakis et al., 2018: U-series-speleothem-based). The “upper” age bounds the oldest moment the chronozone in question may have begun (called the upper age limit in WALIS, although vertical-geologically it is a “lower” bound); the “lower” age bounds the latest moment possibly represented by the chronozone in question. The upper and lower bounds mean that the floating chronozones should be assigned numeric ages within these bounds. It does not mean that the floating units stretch the full span of time between the upper (oldest possible onset age) and lower bound (youngest possible ending age).
Entries were made for 23 LIG chronostratigraphic divisions, 18 of them dealing with terrestrial palynological subdivisions (PAZs), 5 dealing with SW Baltic and Kattegat–Skagerrak marine environmental phases that in turn were correlated and connected to the PAZs (see Table 4 footnote). Comprehensive explanatory text, referencing positions taken in the literature, fills the note field for the parent entries and provides a rationale for the lower and upper numeric ages provided. To avoid redundancy that note field for child entries just contains a cross-reference to the main note in the parent field. The entries also made use of a meta-field in the WALIS table structure that stores parent–child relationships between chronozones. The entries refer to the six main pollen zones of the Zagwijn (1961, 1983, 1996) floating scheme, and the descriptions include the further correlated schemes of Behre (1962), Selle (1962), Müller (1974), and Menke and Tynne (1984) for NW Germany; Jessen and Milthers (1928) in Denmark and NW Germany; and the outcomes of discussions thereof in Benda and Schneekloth (1965), Grüger (1989), Zagwijn (1996), and Funder et al. (2002).
The WALIS database itself and the Zenodo dataset extraction associated with this paper only store age bounds and provide information regarding durations for chronostratigraphic entries, and thus not directly the older and younger onset ages considered in Table 4 (and its background section below). A database user may want to calculate age midpoints between both bounds and formulate some rules that make sure that PAZ order and (relative) durations remain honoured while shifting or stretching the PAZ series (e.g. as in Kopp et al., 2009: Supplement) to reproduce the earlier- and later-onset options in Table 4 (main text) or a more optimised solution of one's own making. The latter could involve Bayesian age-modelling approaches (e.g. Bronk-Ramsey, 2008) to explore what amount and direction of shift best minimises differences with sites that have numeric age control (e.g. against rare U-series ages or against OSL ages and sequences thereof). This road of optimisation has pitfalls, however, because the numeric dating techniques may themselves be subtly biased (inaccurate) to the older or younger site (U-series assumptions) or imprecise (OSL limitations). As demonstrated in the Holocene (e.g. Hijma and Cohen, 2019), ideally a regional Bayesian calibration approach combines optimisations in both the vertical (RSL; see Sect. 2) and temporal (age) planes. The WALIS dataset of the paper may be a starting point for such work for the LIG (Düsterhus et al., 2016), incorporating near-field LIG data from NW Europe. An alternative to Bayesian optimisation against local independent numeric ages is to integrate the floating-age shifting assessment with the regional sea-level predictions of GIA models. Lambeck et al. (2006) provides an early example where GIA modelling was iteratively used to assess an optimal shifting of the timing of the North Sea regional highstand, with an outcome within the lower and upper age bounds in Table 4. The caveat here is that the age solution is dependent on the ice history and rheologies implemented in such GIA modelling (with every simulation, there is a new optimum). The WALIS database chronostratigraphic entries at least provide absolute numeric bounds that any numerically optimised shifting of the floating PAZ-correlated scheme should stay within.
Background to lower and upper ages specified in Table 4
The North Sea PAZ entries (Sect. 3.3, Table 4 in the main text) bracket the full set of PAZs (E1–E6) of Zagwijn (1996) to between 129 ka (acclaimed earliest possible start of PAZ E1) and 112.5 ka (latest possible ending of PAZ E6). This totals 16 500 years, which broadly equals estimates of the length of the LIG globally (Lisiecki and Raymo, 2005: 130–115 ka for MIS 5e) but is longer than the varve-counted 11 000 years traditionally associated with the scheme since Müller (1974). This means that a user requiring absolute time control must decide where on the absolute timescale the Eemian starts (PAZ E1) and ends (PAZ E6).
In the literature, different positions and some debate exist regarding the absolute timing (i.e. numeric ages) of onset of what is palaeo-environmentally regarded the LIG in NW Europe. The debate and options regard a degree of anticipated diachronicity within and between the regional pollen schemes of the various countries, their correlation with southern Europe counterparts, and (non)analogy with the Late Glacial–Holocene (e.g. Van Leeuwen et al., 2000; Cleveringa et al., 2000; Turner, 2002; Kukla et al., 2002; Beets and Beets, 2003; Beets et al., 2006; Lambeck et al., 2006; Sier et al., 2015; Long et al., 2015). The spread in quoted ages in that literature has been considered in filling the upper- and lower-bounding age for the PAZ E1–E6 entries (Table 4 in main text), but with some filtering and actualisation to current (i.e. 2021) insight.
The youngest E1–E6 time span we considered is 125.5 to 112.5 ka. In that late-onset option, the PAZ E5/6 break is put at ∼116 ka (Table 1 in main text), which would mean that the regionally observed climatic cooling correlates (Sirocko et al., 2005) with global signals seen in Greenland ice cores, deep-sea oxygen isotopes, and coral-data-derived LIG climate and sea-level history compilations (e.g. NEEM community members, 2013). Starting the NW European Eemian onset late implies that the Blake palaeomagnetic event occurred early in the interglacial (Sier et al., 2015). Opting for even later onsets (Sier et al., 2015, coined an onset as late as 121 ka) is controversial as that implies that North Sea temperate conditions started >5000 years later than the onset of the interglacial conditions in southwestern Europe at ∼129 ka (Italian speleothems and Portuguese shelf: Tzedakis et al., 2018). With an onset at 125.5 ka the time lag between the southwestern and northwestern unit is ca. 3500 years. Such a lag time implies that early in MIS 5e, greater temperature gradients existed north to south across Europe than those in the Holocene. Such scenarios can be considered (Kukla et al., 2002) because the timing of deglaciation of Scandinavia is not independently dated either and can be suspected to have been slower over Termination II than over Termination I as the MIS-6 ice mass was bigger than in MIS 2. Assuming that Scandinavian deglaciation was slower and the onset of the temperate conditions in NW Europe hence later has repercussions for GIA and RSL both regionally and globally (Long et al., 2015). Adopting the younger onset options hence can be advisable when one wishes to assess scenarios where the Eemian course of Scandinavian deglaciation differed greatly from that in the Holocene.
Placing the start of the NW European Eemian between 129 (the upper bound) and 125.5 ka (the lower bound) provides in-between options. The lag time between interglacial environmental conditions establishing in SW and NW Europe then is less than ∼3 kyr. Such scenarios, e.g. entertaining an onset of 127 ka (∼2 kyr), would be more analogous with the Holocene in terms of vegetation development, as regards the duration of the Scandinavian deglaciation (and the timing of regional GIA response to unloading that drives a good part of the local sea-level signal in the region; Lambeck et al., 2006; Kopp et al., 2009; Long et al., 2015). Investigating this further is an important foreseen application of the WALIS data and may also contribute to resolving the course of events of LIG regional environmental and climatic change from southern to northern Europe.
An even earlier onset of the Eemian, and hence the onset of sea-level rise in the North Sea, has been proposed by some authors, notably Funder et al. (2002) at 132.5 ka and Beets et al. (2006) at 131 ka. We reject these options because they were falsified by the Portuguese shelf studies that put the onset at ∼129 ka (Tzedakis et al., 2018), but not as far as 131 or 132.5 ka. In the case of Funder et al. (2002), the 132.5 ka age was based on a preliminary U-series chronology for midpoint Termination II at ∼135 ka (which Tzedakis et al., 2018, have U-series-dated at ca. 132 ka, i.e. a 3 kyr shift), which their interglacial onset lags by 2.5 kyr. Starting from the presently considered midpoint of Termination II, the onset would be at ∼129 ka, coinciding with Tzedakis et al. (2018) and our “oldest option”. The Lambeck et al. (2006) GIA modelling study focused on the MIS-6 to MIS-4 time period in Europe. It used an ice history with the Warthe sub-stage maximum set at 143–140 ka and midpoint Termination II and onset Eemian age as in Funder et al. (2002). It reproduced the RSL curve of Zagwijn (1983) and from the cessation of global RSL rise (i.e. the midpoint of Termination II onwards) deduces that 1.5–2.0 kyr of extra shifting of the PAZs to the younger side is appropriate (i.e 4.0–4.5 kyr onset lag). The Lambeck et al. (2006) results are in line with a 128–127.5 ka Eemian onset if they are updated with the 3 kyr shift in the midpoint of Termination II.
Lastly, an age of ∼126 ka has long been considered for the onset of interglacial conditions in SW Europe (Iberian Shelf: Sanchez-Goñi et al., 1999; Shackleton et al., 2002, 2003) and used in some of the NW Europe onset age discussion too (without and with additional lag times), before it was falsified and revised to ∼129 ka (Tzedakis et al., 2018). This revision affected onset age positions taken in Sier et al. (2015) and the swath of options considered in Long et al. (2015). It has triggered us to consider 125.5 ka (4500 years lag time to the onset in SW Europe) as the youngest onset option provided in Table 4.
What surfaces from the above summary is that the different advocated ages for onset of the Eemian pollen zones all include dependencies to one or more ages from isotopic records, which in turn have been subject to revision and choice (e.g. Tzedakis, 2003). This is the nature of correlative means of establishing numeric ages and the effect of slowly increasing overall resolution and discovery of new records and techniques (also, for example, improvement in U-series dating). The sources of tie-point ages are (i) stacked marine records (e.g. the Termination II midpoint of Table 1; cf. Lisiecki and Raymo, 2005, derived from Shackleton et al., 2002, 2003); (ii) events in the Greenland ice cores (e.g. Sirocko et al., 2005, for the ending of the interglacial); and (iii) ages established with U-series dating from higher-resolution speleothems and transferred to single marine isotopic records, most recently Tzedakis et al. (2018). The latter paper revised not only the interglacial onset age for southern Europe (to ∼129 ka), but also that of the midpoint Termination II as recorded in the same speleothem record and its correlation to the Iberian margin marine signals. The latter age was put at ∼132 ka, pushed back 2 kyr compared to the Lisiecki and Raymo (2005) age of ∼130 ka. The WALIS database may consider updating this numeric age in its dedicated part for MIS ages (“form_MIS_ages”; Rovere et al., 2020) to have the North Sea numeric age constraint entries fully compatible with the overall WALIS set-up.
Time control and data filtering in England and France
In the British Isles, biostratigraphy has also been typically used to characterise the sites into the pollen sub-stages of the “Ipswichian” and the preceding “Hoxnian” interglacials (West, 1977), but without lithostratigraphic constraints as in continental Europe or the presence of a full Ipswichian pollen profile at a single site. It has since been shown that these “pollen interglacials” have very similar vegetation profiles and in fact represent more than one interglacial (Thomas, 2001; Turner and West, 1968), with many early “Ipswichian” sites conflated with those from MIS 7 (Lewis et al., 2011; Bridgland, 1994), and the “Hoxnian” pollen spectra have been shown to be very similar in both MIS 9 and MIS 11 (Roe et al., 2009; Thomas, 2001). Furthermore, due to the lack of a continuous pollen profile (Lewis et al., 2011; Thomas, 2001) and the difference in the marine climate of the UK versus the relatively continental Northwest European plain, it is not possible to simply correlate the “Ipswichian” pollen zones with the Eemian zones from NW Europe (Turner, 2000).
Though dating approaches have significantly improved since many of the UK “Ipswichian” sites were first studied, not all sites are available to be revisited with modern methods, and therefore several sites which some may consider to be evidence for LIG sea level have been discounted from our database. These include, for example, Somersham, Cambridgeshire (West et al., 1999), Kirmington, Lincolnshire (Straw, 2018), Burtle Beds, Somerset (Kidson and Heyworth, 1976) and six of the seven Thames sites in the analysis of Hollin (1977). Furthermore, questions around microfossil and sediment reworking and preservation, e.g. Tattershall Thorp, Lincolnshire (Holyoak and Preece, 1985) and Somersham (West et al., 1999), and debate around the presence and absence of fauna and flora during specific interglacials (e.g. Meijer and Preece, 2000) mean that sites that cannot conclusively be ascribed to a single interglacial period or have an indicative meaning (i.e. ambiguous sites; cf. Fig. 2b) are not included in the database.
To address some of the issues in biostratigraphic chronology across Britain, relative dating of interglacial coastal sites using amino acid racemisation (AAR) began in 1979 (Andrews et al., 1979; Miller et al., 1979) and continued apace throughout the 1980s (Keen et al., 1981; Campbell et al., 1982; Davies, 1983; Andrews et al., 1984; Bowen et al., 1985; Davies and Keen, 1985). Such investigation was also performed on material from multiple marine interglacial sites collected from across Europe (Miller and Mangerud, 1985). A landmark review of these early works is given in Penkman (2010). Early AAR results often indicated potential associations with two or more interglacial periods within individual sites or even multiple ages within single interglacials. Major challenges were the evolving preparation procedures and inconsistent choices of species for sampling, which both hindered comparability of AAR values across different studies. Around the same time, results from the application of novel dating techniques to raised interglacial deposits in the region were being used to inform the AAR debate. U-series dates from the raised beaches at Belle Hogue Cave on Jersey (Keen et al., 1981) and at Minchin Hole (Sutcliffe and Currant, 1984) and Bacon Hole (Stringer et al., 1986) in South Wales were often cited to correlate AAR-dated deposits to MIS 5e (Davies, 1983; Bowen et al., 1985; Davies and Keen, 1985; Bridgland et al., 1995b), although single U-series dates were insufficient for describing the full complexity of single sites given the multiple (age) groupings that were often present within AAR analyses. Early thermoluminescence techniques were also being used at this time (Southgate, 1985) but were still unable to distinguish interglacial associations as results from fine-grained and large-grained sand fractions provided dates of MIS 5e or MIS 7, respectively.
Improved certainty in the AAR debate was reached by 2013 when an aminostratigraphy for the British Quaternary was developed using the opercula or the gastropod species Bithynia tentaculata (Penkman et al., 2013). Unfortunately, none of the existing AAR results from interglacial coastal sites in southern and southwestern Britain were based on this species, and material from only limited sites in eastern England is available, and therefore definitive age determinations for many of these sites remain elusive, resulting in some sites being excluded from the database. Parallel to the AAR investigation efforts, development and application of luminescence and U-series dating techniques have gone some way to confirming interglacial associations at several sites (Preece et al., 1990; Proctor and Smart, 1991; Bates et al., 2010; Briant et al., 2019), though there are numerous sites in both the UK and France that lack independent dating control (e.g. Tastet, 1999; Haslett and Curr, 2001).
With a few exceptions, most of the sites in northern France have been attributed to MIS 5 based on ages that were obtained from deposits overlying or underlying the LIG features, and in most cases these results are not enough to discard age attributions older or younger than MIS 5e.
There remains considerable opportunity to revisit many (known and as yet undescribed) interglacial raised beach sites across southern and southwestern Britain and France and to apply now-established sea-level research protocols (Shennan et al., 2015) and spatially intensive geochronology sampling strategies that could combine with statistical (e.g. Bayesian) frameworks to greatly advance knowledge and data precision across this region.
KMC, RB, VC and NLMB each reviewed regional bodies of literature, compiled the data, assigned indicative meanings and documented WALIS database entries that underpin this paper (with the Netherlands, Belgium, the German Bight, Denmark and the Danish–German borderlands southeast of the Baltic Sea compiled by KMC; the British North Sea coast compiled by NLMB; the Sussex coastal plain, southeastern England and France, and offshore records in the British North Sea complied by VC; and southern and southwestern Britain compiled by RB). KMC and VC prepared figures and tables. FSB and KMC assessed North Sea basin subsidence VLM. All authors edited the manuscript (in the template of the WALIS database special issue), designed figure and table legends, and addressed referee comments. KMC, FSB and NLMB executed the revision.
The contact author has declared that neither they nor their co-authors have any competing interests.
Publisher's note: Copernicus Publications remains neutral with regard to jurisdictional claims in published maps and institutional affiliations.
This article is part of the special issue “WALIS – the World Atlas of Last Interglacial Shorelines”. It is not associated with a conference.
This paper forms a contribution to the RISeR project, which has received funding from the European Research Council (ERC) under the European Union's Horizon 2020 research and innovation programme (grant agreement no. 802281; Natasha L. M. Barlow as PI). The authors acknowledge PALSEA, a working group of the International Union for Quaternary Sciences (INQUA) and Past Global Changes (PAGES), which in turn received support from the Swiss Academy of Sciences and the Chinese Academy of Sciences. We are grateful to Deirdre Ryan (special issue handling editor) and Alessio Rovere (WALIS lead and special issue lead editor) for guidance and assistance in the preparation of this paper, Kirsty Penkman (University of York) for discussion of the AAR data compilation, Sebastian Garzon (internship student from University of Münster) for developing the WALIS visualisation interface, and Amy McGuire (University of Leeds) and Chronis Tzedakis (University College London) for discussion around UK interglacial pollen. Preprint reviews and comments by Torbjörn Törnqvist and an anonymous reviewer greatly helped in preparation of the final paper. The data used in this study were compiled in WALIS, a sea-level database interface developed by the ERC Starting Grant WARMCOASTS (ERC-StG-802414), in collaboration with the PALSEA (PAGES–INQUA) working group. The database structure was designed by Alessio Rovere, Deirdre Ryan, Thomas Lorscheid, Andrea Dutton, Peter Chutcharavan, Dominik Brill, Nathan Jankowski, Daniela Mueller, Melanie Bartz, Kim Cohen and Evan Gowan.
This research has been supported by the European Research Council's H2020 research and innovation programme (grant nos. RISeR (ERC-StG-802281) and WARMCOASTS (ERC-StG-802414)).
This paper was edited by Deirdre Ryan and reviewed by Torbjörn Törnqvist and one anonymous referee.
Abbott, L.: The section exposed in the foundations of the new Admiralty Offices, P. Geologist. Assoc., 12, 346–356, 1892.
Allen, L. G., Gibbard, P. L., Pettit, M. E., Preece, R. C., and Robinson, J. E.: Late Pleistocene interglacial deposits at Pennington Marshes, Lymington, Hampshire, southern England, P. Geologist. Assoc., 107, 39–50, 1996.
Amkreutz, L., Cohen, K., Hijma, M., and Odé, O.: Verdrinkend land in kaart, in: Doggerland, verdwenen wereld in de Noordzee, edited by: Amkreutz, L. and van der Vaart-Verschoof, S., Rijksmuseum van Oudheden/Sidestone press, e-book ISBN 9789464260083, 33–37, 2021.
Andrews, J. T., Bowen, D. Q., and Kidson, C.: Amino acid ratios and the correlation of raised beach deposits in south-west England and Wales, Nature, 281, 256–258, 1979.
Andrews, J. T., Gilberston, D. D., and Hawkins, A. B.: The Pleistocene succession of the Severn Estuary: a revised model based upon amino acid racemisation studies, J. Geol. Soc. London, 141, 967–974, 1984.
Antoine, P., Lozouet, N. L., Chaussé, C., Lautridou, J. P., Pastre, J. F., Auguste, P., Bahain, J.-J., Falguères, C., and Galehb, B.: Pleistocene fluvial terraces from northern France (Seine, Yonne, Somme): synthesis, and new results from interglacial deposits, Quaternary Sci. Rev., 26, 2701–2723, https://doi.org/10.1016/j.quascirev.2006.01.036, 2007.
Arfai, J., Franke, D., Lutz, R., Reinhardt, L., Kley, J., and Gaedicke, C.: Rapid quaternary subsidence in the northwestern German North Sea, Sci. Rep.-UK, 8, 1–12, https://doi.org/10.1038/s41598-018-29638-6, 2018.
Arkell, W. J.: The Pleistocene Rocks at Trebetherick Point, North Cornwall: Their interpretation and correlation, P. Geologist. Assoc., 54, 141–170, https://doi.org/10.1016/S0016-7878(43)80001-8, 1943.
Bahain, J. J., Falguères, C., Laurent, M., Shao, Q., Dolo, J. M., Garcia, T., Douville, E., Frank, N., Monnier, J. L., Hallégouët, B., Laforge, M., Huet, B., Auguste, P., Liouville, M., Serre, F., and Gagnepain, J.: ESR and ESR/U-series dating study of several middle Palaeolithic sites of Pléneuf-Val-André (Brittany, France): Piégu, Les Vallées and Nantois, Quat. Geochronol., 10, 424–429, https://doi.org/10.1016/j.quageo.2012.02.013, 2012.
Bahnson, H., Petersen, K. S., Konradi, P., and Knudsen, K. L.: Stratigraphy of Quaternary deposits in the Skaerumhede II boring: Lithology, molluscs and foraminifera, Danmarks Geologiske Undersøgelse, Årbog, 1973, 27–62, 1974.
Balescu, S. and Haesaerts, P.: The Sangatte raised beach and the age of the opening of the Strait of Dover, Geol. Mijnbouw, 63, 355–362, 1984.
Balescu, S. and Lamothe, M.: The blue emission of K-feldspar coarse grains and its potential for overcoming TL age underestimation, Quaternary Sci. Rev., 11, 45–51, https://doi.org/10.1016/0277-3791(92)90041-6, 1992.
Balescu, S., Packman, S. C., and Wintle, A. G.: Chronological Separation of Interglacial Raised Beaches from Northwestern Europe Using Thermoluminescence, Quaternary Res., 35, 91–102, https://doi.org/10.1016/0033-5894(91)90097-O, 1991.
Barlow, N. L. M., Shennan, I., Long, A. J., Gehrels, W. R., Saher, M. H., Woodroffe, S. A., and Hillier, C.: Salt marshes as geological tide gauges, Global Planet. Change, 106, 90–110, 2013.
Barlow, N. L. M., Long, A. J., Gehrels, W. R., Saher, M. H., Scaife, R. G., Davies, H. J., Penkman, K. E. H., Bridgland, D. R., Sparkes, A., Smart, C. W., and Taylor, S.: Relative sea-level variability during the late Middle Pleistocene: new evidence from eastern England, Quaternary Sci. Rev., 173, 20–39, 2017.
Barrois, C.: Note sur les traces de l'époque glaciaire en quelques points des côtes de Bretagne, B. Soc. Geol. Fr., 5, 535–537, 1877.
Barrois, C.: Sur les plages soulevées de la côte ouest du Finistère, Annales de la Société Géologique du Nord, 9, 239–268, 1882.
Barrow, G.: The Geology of the Isles of Scilly, Memoirs of the Geological Survey, England and Wales, ISBN 9781330203675, 1906.
Barthes, V., Pozzi, J. P., Vibert-Charbonnel, P., Thibal, J., and Melieres, M. A.: High-resolution chronostratigraphy from downhole susceptibility logging tuned by palaeoclimatic orbital frequencies, Earth Planet. Sc. Lett., 165, 97–116, https://doi.org/10.1016/S0012-821X(98)00214-3, 1999.
Batchelor, C. L., Margold, M., Krapp, M., Murton, D. K., Dalton, A. S., Gibbard, P. L., Stokes, C. R., Murton, J. B., and Manica, A.: The configuration of Northern Hemisphere ice sheets through the Quaternary, Nat. Commun., 10, 3713–3713, https://doi.org/10.1038/s41467-019-11601-2, 2019.
Bateman, M. and Catt, J.: An absolute chronology for the raised beach and associated deposits at Sewerby, East Yorkshire, England, J. Quaternary Sci., 11, 389–395, 1996.
Bates, M. R., Parfitt, S. A., and Roberts, M. A.: The chronology, palaeogeography and archaeological significance of the marine Quaternary record of the West Sussex coastal plain, southern England, U.K., Quaternary Sci. Rev., 16, 1227–1252, https://doi.org/10.1016/S0277-3791(96)00119-9, 1997.
Bates, M. R., Keen, D. H., and Lautridou, J.-P.: Pleistocene marine and periglacial deposits of the English Channel, J. Quaternary Sci., 18, 319–337, https://doi.org/10.1002/JQS.747, 2003.
Bates, M. R., Briant, R. M., Rhodes, E. J., Schwenninger, J.-L., and Whittaker, J. E.: A new chronological framework for Middle and Upper Pleistocene landscape evolution in the Sussex/Hampshire coastal corridor, UK, P. Geologist. Assoc., 121, 369–392, https://doi.org/10.1016/j.pgeola.2010.02.004, 2010.
Beets, C. J. and Beets, D. J.: A high resolution stable isotope record of the penultimate deglaciation in lake sediments below the city of Amsterdam, The Netherlands, Quaternary Sci. Rev., 22, 195–207, https://doi.org/10.1016/S0277-3791(02)00089-6, 2003.
Beets, D. J., Meijer, T., Beets, C. J., Cleveringa, P., Laban, C., and Van der Spek, A. J. F.: Evidence for a Middle Pleistocene glaciation of MIS 8 age in the southern North Sea, Quatern. Int., 133, 7–19, https://doi.org/10.1016/j.quaint.2004.10.002, 2005.
Beets, D. J., Beets, C. J., and Cleveringa, P.: Age and climate of the late Saalian and early Eemian in the type-area, Amsterdam basin, The Netherlands, Quaternary Sci. Rev., 25, 876–885, https://doi.org/10.1016/j.quascirev.2005.10.001, 2006.
Behre, K. E.: Pollen- und diatomeenanalytische Untersuchungen an letzinterglazialen Kieselgurlagen der Lueneburger Heide (Schwindebeck und Grevenhof im oberen Luhetal), Flora, 152, 325–370, 1962.
Behre, K. E., Hölzer, A., and Lemdahl, G.: Botanical macro-remains and insects from the Eemian and Weichselian site of Oerel (northwest Germany) and their evidence for the history of climate, Veg. Hist. Archaeobot., 14, 31–53, 2005.
Benda, L. and Schneekloth, H.: Das Eem-Interglazial von Köhlen, Krs. Wesermünde, Geologisches Jahrbuch, 83, 699–716, 1965.
Berendsen, H. J. A., Makaske, B., Van de Plassche, O., Van Ree, M. H. M., Das, S., Dongen, M. V., Ploumen, S., and Schoenmakers, W.: New groundwater-level rise data from the Rhine-Meuse delta–implications for the reconstruction of Holocene relative mean sea-level rise and differential land-level movements, Neth. J. Geosci., 86, 333–354, 2007.
Bigot, A.: Sur l'existence d'une station préhistorique à la Hougue (Manche), Mémoires de la Société nationale des sciences naturelles de Cherbourg XXV, 277–280, 1885.
Bigot, A.: Les terrasses pléistocènes du littoral du Cotentin, Livre jubilaire de la Société géologique de France I, 133–148, 1930.
Bogemans, F., Roe, H. M., and Baeteman, C.: Incised Pleistocene valleys in the Western Belgium coastal plain: Age, origins and implications for the evolution of the Southern North Sea Basin, Palaeogeogr. Palaeocl., 456, 46–59, 2016.
Bosch, J. H. A.: Toelichtingen bij de Geologische Kaart van Nederland 1:50000, Blad Assen (12 W), Blad Assen (12 O), 1990.
Bowen, D. Q.: The Pleistocene succession of the Irish Sea, P. Geologist. Assoc., 84, 249–272, 1973.
Bowen, D. Q., Sykes, G. A., Reeves, A., Miller, G. H., Andrews, J. T., Brew, J. S., and Hare, P. E.: Amino acid geochronology of raised beaches in south west Britain, Quaternary Sci. Rev., 4, 279–318, 1985.
Bradley, S. L., Milne, G. A., Shennan, I., and Edwards, R.: An improved glacial isostatic adjustment model for the British Isles, J. Quaternary Sci., 26, 541–552, https://doi.org/10.1002/jqs.1481, 2011.
Brain, M. J.: Compaction, in: Handbook of sea-level research, edited by: Shennan, I., Long, A. J., and Horton, B. P., John Wiley & Sons, Ltd, Chichester, UK, 452–469, https://doi.org/10.1002/9781118452547.ch30, 2015.
Breton, G., Cousin, R., Huault, M.-F., Lechevallier, C., and Lefebvre, D.: Les sédiments quaternaires du quartier de l'Hotel de Ville, au Havre: séquences marines pré-éemienne, éemienne et holocène de l'estuaire de la Seine, Bulletin de la Société Géologique de Normandie, 4, 15–63, 1991.
Briant, R. M., Bates, M. R., Schwenninger, J.-L., and Wenban-Smith, F.: An optically stimulated luminescence date Middle to Late Pleistocene fluvial sequence from the western Solent Basin, southern England, J. Quaternary Sci., 21, 507–523, https://doi.org/10.1002/jqs.1035, 2006.
Briant, R. M., Kilfeather, A., Parfitt, S., Penkman, K., Preece, R., Roe, H., Schwenninger, J.-L., Wenban-Smith, F., and Whittaker, J.: Integrated chronological control on an archaeologically significant Pleistocene river terrace sequence: the Thames-Medway, eastern Essex, England, P. Geologist. Assoc., 123, 87–108, https://doi.org/10.1016/j.pgeola.2011.07.008, 2012.
Briant, R. M., Bates, M. R., Boreham, S., Cameron, N. G., Coope, G. R., Field, M. H., Hatch, B. M., Holmes, J. A., Keen, D. H., Kilfeather, A. A., Penkman, K. E. H., Simons, R. M. J., Schwenninger, J.-L., Wenban-Smith, F. F., Whitehouse N. J., and Whittaker, J. E.: Early Ipswichian (last interglacial) sea level rise in the channel region: Stone Point Site of Special Scientific Interest, Hampshire, England, P. Geologist. Assoc., 130, 1–26, https://doi.org/10.1016/j.pgeola.2018.03.002, 2019.
Bridgland, D. R.: Quaternary of the Thames, Joint Nature Conservation Committee, Chapman & Hall, London, ISBN 0412488302, 1994.
Bridgland, D. R. and d'Olier, B.: The Pleistocene evolution of the Thames and Rhine drainage systems in the southern North Sea Basin, Geological Society, London, Special Publications, 96, 27–45, https://doi.org/10.1144/GSL.SP.1995.096.01.04, 1995.
Bridgland, D., Currant, A., and Preece, R.: East Mersea restaurant site (TM 053136), in: The Quaternary of the Lower Reaches of the Thames: Field Guide, edited by: Bridgland, D. R., Allen, P., and Haggart, B. A., Quaternary Research Association, London, 271–274, ISBN 9780907780229, 1995a.
Bridgland, D. R., Keen, D. H., Green, G. P., Bowen, D. Q., and Sykes, G. A.: Last Interglacial deposits at Folkestone, Kent, P. Geologist. Assoc., 106, 183–193, 1995b.
Bronk Ramsey, C.: Deposition models for chronological records, Quaternary Sci. Rev., 27, 42–60, 2008.
Bungenstock, F., Freund, H., and Bartholomä, A.: Holocene relative sea-level data for the East Frisian barrier coast, NW Germany, southern North Sea, Neth. J. Geosci., 100, E16, https://doi.org/10.1017/njg.2021.11, 2021.
Busschers, F. S., Weerts, H. J. T., Wallinga, J., Cleveringa, P., Kasse, C., De Wolf, H., and Cohen, K. M.: Sedimentary architecture and optical dating of Middle and Late Pleistocene Rhine-Meuse deposits-fluvial response to climate change, sea-level fluctuation and glaciation, Neth. J. Geosci., 84, 25–41, 2005.
Busschers, F. S., Kasse, C., Van Balen, R. T., Vandenberghe, J., Cohen, K. M., Weerts, H. J. T., Wallinga, J., Johns, C., Cleveringa, P., and Bunnik, F. P. M.: Late Pleistocene evolution of the Rhine-Meuse system in the southern North Sea basin: imprints of climate change, sea-level oscillation and glacio-isostacy, Quaternary Sci. Rev., 26, 3216–3248, 2007.
Busschers, F. S., Van Balen, R. T., Cohen, K. M., Kasse, C., Weerts, H. J., Wallinga, J., and Bunnik, F. P.: Response of the Rhine–Meuse fluvial system to Saalian ice-sheet dynamics, Boreas, 37, 377–398, https://doi.org/10.1111/j.1502-3885.2008.00025.x, 2008.
Buylaert, J. P., Huot, S., Murray, A. S., and Van Den Haute, P.: Infrared stimulated luminescence dating of an Eemian (MIS 5e) site in Denmark using K-feldspar, Boreas, 40, 46–56, https://doi.org/10.1111/j.1502-3885.2010.00156.x, 2011.
Campbell, S., Andrews, J. T., and Shakesby, R. A.: Amino acid evidence for Devensian ice, west Gower, South Wales, Nature, 300, 249–251, 1982.
Campbell, S., Hunt, C. O., Scourse, J. D., and Keen, D. H.: Quaternary of South-west England, Chapman & Hall, London, https://doi.org/10.1007/978-94-011-4920-4, 1998.
Cartelle, V., Barlow, N. L. M., Hodgson, D. M., Busschers, F. S., Cohen, K. M., Meijninger, B. M. L., and van Kesteren, W. P.: Sedimentary architecture and landforms of the late Saalian (MIS 6) ice sheet margin offshore of the Netherlands, Earth Surf. Dynam., 9, 1399–1421, https://doi.org/10.5194/esurf-9-1399-2021, 2021.
Catt, J.: The Pleistocene glaciations of eastern Yorkshire: a review, P. Yorks. Geol. Soc., 56, 177–207, 2007.
Catt, J. A. and Penny, L. F.: The Pleistocene Deposits of Holderness, East Yorkshire, P. Yorks. Geol. Soc., 35, 375–409, https://doi.org/10.1144/pygs.35.3.375, 1966.
Cerrone, C., Vacchi, M., Fontana, A., and Rovere, A.: Last Interglacial sea-level proxies in the western Mediterranean, Earth Syst. Sci. Data, 13, 4485–4527, https://doi.org/10.5194/essd-13-4485-2021, 2021.
Chutcharavan, P. M. and Dutton, A.: A global compilation of U-series-dated fossil coral sea-level indicators for the Last Interglacial period (Marine Isotope Stage 5e), Earth Syst. Sci. Data, 13, 3155–3178, https://doi.org/10.5194/essd-13-3155-2021, 2021.
Clemmensen, L. B., Hougaard, I. W., Murray, A. S., and Pedersen, S. S.: A high-resolution sea-level proxy dated using quartz OSL from the Holocene Skagen Odde spit system, Denmark, Boreas, 47, 1184–1198, 2018.
Cleveringa, P., Meijer, T., Van Leeuwen, R. J. W., Wolf, H. D., Pouwer, R., Lissenberg, T., and Burger, A. W.: The Eemian stratotype locality at Amersfoort in the central Netherlands: a re-evaluation of old and new data, Neth. J. Geosci., 79, 197–216, https://doi.org/10.1017/S0016774600023659, 2000.
Cliquet, D.: Le gisement paléolithique moyen de Saint-Germain des Vaux/Port-Racine (Manche) dans son cadre régional, Editions ERAUL, Liège, ISSN 0777-2173, 1994.
Cliquet, D.: Palaeolithic settlements of Normandy in their chronostratigraphic context: “archaeological scraps”, Quaternaire, 24, 315–358, 2013.
Cliquet, D., Mercier, N., Valladas, H., Froget, L., Michel, D., van Vliet-Lanoë, B., and Vilgrain, G.: Apport de la thermoluminescence sur silex chauffes à la chronologie de site paléolithiques de Normandie: nouvelles données et interprétations, Quaternaire, 14, 51–64, https://doi.org/10.3406/quate.2003.1729, 2003.
Cliquet, D., Lautridou, J.-P., Lamothe, M., Mercier, N., Schwenninger, J.-L., Alix, P., and Vilgrain, G.: Nouvelles données sur le site majeur d'Écalgrain: datations radiométriques et occupations humaines de la Pointe de la Hague (Cotentin, Normandie), Quaternaire, 20, 345–359, https://doi.org/10.4000/quaternaire.5244, 2009.
Cohen, K. M., MacDonald, K., Joordens, J. C. A., Roebroeks, W., and Gibbard, P. L.: The earliest occupation of north-west Europe: a coastal perspective, Quatern. Int., 271, 70–83, https://doi.org/10.1016/j.quaint.2011.11.003, 2012.
Cohen, K. M., Gibbard, P. L., and Weerts, H. J. T.: North Sea palaeogeographical reconstructions for the last 1 Ma, Neth. J. Geosci., 93, 7–29, https://doi.org/10.1017/njg.2014.12, 2014.
Cohen, K. M., Westley, K., Erkens, G., Hijma, M. P., and Weerts, H. J. T.: The North Sea, in: Submerged landscapes of the European continental shelf: quaternary paleoenvironments, Wiley, Chichester, 147–186, https://doi.org/10.1002/9781118927823.ch7, 2017.
Cohen, K. M., Cartelle, V., Barnett, R., Busschers, F. S., and Barlow, N. L. M.: Last Interglacial sea-level data points from Northwest Europe, V1: https://doi.org/10.5281/zenodo.5608459, V2: https://doi.org/10.5281/zenodo.6478094, Zenodo [data set], 2021.
Coutard, S., Lautridou, J.-P., and Rhodes, E.: Discontinuités dans l'enregistrement des cycles interglaciaire-glaciaire sur un littoral en contexte intraplaque. Exemple du Val de Saire (Normandie, France), Quaternaire, 16, 217–227, https://doi.org/10.4000/quaternaire.434, 2005.
Coutard, S., Lautridou, J. P., Rhodes, E., and Clet, M.: Tectonic, eustatic and climatic significance of raised beaches of Val de Saire, Cotentin, Normandy, France, Quaternary Sci. Rev., 25, 595–611, https://doi.org/10.1016/j.quascirev.2005.02.003, 2006.
Davies, K. H.: Amino acid analysis of Pleistocene marine molluscs from the Gower Peninsula, Nature, 32, 137–139, 1983.
Davies, K. H.: The aminostratigraphy of British Pleistocene beach deposits, unpublished PhD Thesis, U.C.W. Aberystwyth, 1984.
Davies, K. H. and Keen, D. H.: The age of Pleistocene marine deposits at Portland, Dorset, P. Geologist. Assoc., 96, 217–225, 1985.
De Clercq, M., Missiaen, T., Wallinga, J., Zurita Hurtado, O., Versendaal, A., Mathys, M., and De Batist, M.: A well-preserved Eemian incised-valley fill in the southern North Sea Basin, Belgian Continental Shelf-Coastal Plain: Implications for northwest European landscape evolution, Earth Surf. Proc. Land., 43, 1913–1942, https://doi.org/10.1002/esp.4365, 2018.
De Gans, W., Beets, D. J., and Centineo, M. C.: Late Saalian and Eemian deposits in the Amsterdam glacial basin, Neth. J. Geosci., 79, 147–160, https://doi.org/10.1017/S0016774600021685, 2000.
De Heinzelin, J.: Falaise et plage suspendue de Sangatte, Bulletin de la Société Belge de Géologie 75, 292–296, 1966.
De La Beche, H. T.: Report on the geology of Cornwall and Devon and West Somerset, Memoirs of the Geological Survey, London, 1839.
De Moor, G. and De Breuck, W.: Sedimentologie en stratigrafie van enkele pleistocene afzettingen in de Belgische kustvlakte, Natuurwetenschappelijk Tijdschrift, 55, 3–96, 1973.
De Moor, G. and Pissart, A.: Het reliëf, in: Geografie van België, edited by: Denis, J., Gemeentekrediet, Brussels, 128–215, http://hdl.handle.net/1854/LU-222633, 1992.
Dechend, W. and Sindowski, K.-H.: Die Gliederung der Quartärs im Raum Krummhörn-Dollart (Ostfriesland) und die geologische Entwicklung der unteren Ems, Geologisches Jahrbuch, 71, 461–490, 1956.
Dendy, S., Austermann, J., Creveling, J. R., and Mitrovica, J. X.: Sensitivity of Last Interglacial sea-level high stands to ice sheet configuration during Marine Isotope Stage 6, Quaternary Sci. Rev., 171, 234–244, 2017.
Demarchi, B., Williams, M. G., Milner, N., Russell, N., Bailey, G., and Penkman, K.: Amino acid racemization dating of marine shells: A mound of possibilities, Quatern. Int., 239, 114–124, https://doi.org/10.1016/j.quaint.2010.05.029, 2011.
Dixon, F.: The Geology and Fossils of the Tertiary and Cretaceous Formations of Sussex, Longman, Brown, Green and Longmans, https://doi.org/10.5962/bhl.title.14790, 1850.
Düsterhus, A., Tamisiea, M. E., and Jevrejeva, S.: Estimating the sea level highstand during the last interglacial: a probabilistic massive ensemble approach, Geophys. J. Int., 206, 900–920, https://doi.org/10.1093/gji/ggw174, 2016.
Dutton, A., Carlson, A. E., Long, A. J., Milne, G. A., Clark, P. U., DeConto, R., Horton, B. P., Rahmstorf, S., and Raymo, M. E.: Sea-level rise due to polar ice-sheet mass loss during past warm periods, Science, 349, 6244, https://doi.org/10.1126/science.aaa4019, 2015.
Eaton, S. J., Hodgson, D. M., Barlow, N. L., Mortimer, E. E., and Mellett, C. L.: Palaeogeographical changes in response to glacial–interglacial cycles, as recorded in Middle and Late Pleistocene seismic stratigraphy, southern North Sea, J. Quaternary Sci., 35, 760–775, 2020.
Edwards, R. J. and Horton, B. P.: Reconstructing relative sea-level change using UK salt-marsh foraminifera, Mar. Geol., 169, 41–56, https://doi.org/10.1016/s0025-3227(00)00078-5, 2000.
Ehlers, J. and Gibbard, P. L.: Quaternary glaciations-extent and chronology: part I: Europe, Elsevier, ISBN 978-0-444-51462-2, 2004.
Ehlers, J., Eissman, L., Lippstreu, L., Stephan, H. J., and Wansa, S.: Pleistocene glaciations of north Germany, in: Quaternary Glaciations-Extent and Chronology: Part I: Europe, 135–146, edited by: Ehlers, J. and Gibbard, P. L., https://doi.org/10.1016/S1571-0866(04)80064-2, 2004.
Elhaï, H.: La Normandie occidentale entre la Seine et le Golfe normand-breton, Etude morphologique, Bière, Bodeaux, http://www.persee.fr/doc/ingeo_0020-0093_1963_num_27_4_5567 (last access: 19 June 2022), 1963.
EMODnet Bathymetry Consortium: EMODnet Digital Bathymetry (DTM), https://doi.org/10.12770/bb6a87dd-e579-4036-abe1-e649cea9881a, 2020.
Engelhart, S. E. and Horton, B. P.: Holocene sea level database for the Atlantic coast of the United States, Quaternary Sci. Rev., 54, 12–25, https://doi.org/10.1016/j.quascirev.2011.09.013, 2012.
Engelhart, S. E., Horton, B. P., and Kemp, A. C.: Holocene Sea Level Changes Along the United States' Atlantic Coast, Oceanography, 24, 70–79, https://doi.org/10.1016/j.quascirev.2011.09.013, 2011.
Farrell, W. E. and Clark, J. A.: On postglacial sea level, Geophys. J. Roy. Astr. S., 46, 647–667, 1976.
Flemming, N. C.: Multiple regression analysis of earth movements and eustatic sea-level change in the United Kingdom in the past 9000 years, P. Geologist. Assoc., 93, 113–125, https://doi.org/10.1016/S0016-7878(82)80035-7, 1982.
Folz, E.: La luminescence stimulée optiquement du quartz: développements méthodologiques et applications à la datation de séquences du pléistocène supérieur du nord-ouest de la France, https://www.theses.fr/2000PA077082 (last access: 19 June 2022), 2000.
Franks, J. W.: Interglacial deposits at Trafalgar Square, London, New Phytol., 59, 145–152, https://doi.org/10.1111/j.1469-8137.1960.tb06212.x, 1960.
Friborg, R.: The landscape below the Tinglev outwash plain: a reconstruction, B. Geol. S. Denmark, 43, 34–40, 1996.
Funder, S., Demidov, I., and Yelovicheva, Y.: Hydrography and mollusc faunas of the Baltic and the White Sea–North Sea seaway in the Eemian, Palaeogeogr. Palaeocl., 184, 275–304, https://doi.org/10.1016/S0031-0182(02)00256-0, 2002.
García-Moreno, D., Verbeeck, K., Camelbeeck, T., De Batist, M., Oggioni, F., Zurita Hurtado, O., Versteeg, W., Jomard, H., Collier, J. S., Gupta, S., Trentesaux, A., and Vanneste, K.: Fault activity in the epicentral area of the 1580 Dover Strait (Pas-de-Calais) earthquake (northwestern Europe), Geophys. J. Int., 201, 528–542, https://doi.org/10.1093/gji/ggv041, 2015.
Gale, S. J., Hoare, P. G., Hunt, C. O., and Pye, K.: The middle and upper Quaternary deposits at Morton, North Norfolk, UK Geological Magazine, 125, 521–533, https://doi.org/10.1017/S001675680001325X, 1988.
Garzon, S. and Rovere, A.: WALIS visualization interface (Version 1.0), Zenodo [code], https://doi.org/10.5281/zenodo.4943541, 2021.
Gehrels, W. R.: Determining relative sea-level change from salt-marsh foraminifera and plant zones on the coast of Maine, USA, J. Coastal Res., 10, 990–1009, 1994.
Gehrels, W. R.: Using foraminiferal transfer functions to produce high-resloution sea-level records from salt-marsh deposits, Maine, USA, Holocene, 10, 367–376, 2000.
Gibbard, P. L.: The Pleistocene history of the Middle Thames valley, Cambridge University Press, ISBN 0 312 26578 9, 1985.
Gibbard, P. L.: The history of the great northwest European rivers during the past three million years, Philos. T. Roy. Soc. B, 318, 559–602, 1988.
Gibbard, P. L.: The formation of the Strait of Dover, Geological Society, London, Special Publications, 96, 15–26, 1995.
Gibbard, P. L., West, R. G., and Hughes, P. D.: Pleistocene glaciation of Fenland, England, and its implications for evolution of the region, Roy. Soc. Open Sci., 5, 170736, https://doi.org/10.1098/rsos.170736, 2018.
Gilbert, A.: The raised shoreline sequence at Saunton, in: Devon and East Cornwall, edited by: Charman, D. J., Newnham, R. M., and Croot, D., Field Guide, Quaternary Research Association, London, 40–47, ISBN 9780907780274, 1996.
Godwin-Austen, R.: On the land-surfaces beneath the Drift-Gravel, J. Geol. Soc. London, 11, 112–119, 1855.
Godwin-Austen, R.: On the Newer Tertiary Deposits of the Sussex Coast, Quarterly J. Geol. Soc. London, 13, 40–72, 1857.
Greensmith, J. T. and Tucker, E. V.: Compaction and consolidation, in: Sea-level research: a manual for the collection and evaluation of data, edited by: Van de Plassche, O., 591–603, https://doi.org/10.1007/978-94-009-4215-8_22, 1986.
Grüger, E.: Palynostratigraphy of the last interglacial/glacial cycle in Germany, Quatern. Int., 3, 69–79, 1989.
Gupta, S., Collier, J. S., Palmer-Felgate, A., and Potter, G.: Catastrophic flooding origin of shelf valley systems in the English Channel, Nature, 448, 342–345, https://doi.org/10.1038/nature06018, 2007.
Gupta, S., Collier, J. S., Garcia-Moreno, D., Oggioni, F., Trentesaux, A., Vanneste, K., De Batist, M., Camelbeeck, T., Potter, G., Vliet-Lanoë, V., and Arthur, J. C.: Two-stage opening of the Dover Strait and the origin of island Britain, Nat. Commun., 8, 15101, https://doi.org/10.1038/ncomms15101, 2017.
Haesaerts, P. and Dupuis, C.: Contribution à la stratigraphie des nappes alluviales de la Somme et de l'Avre dans la région d'Amiens. Supplément au Bulletin de l'Association Française pour l'Etude du Quaternaire, 26, 171–186, 1986.
Hanebuth, T., Stattegger, K., and Grootes, P. M.: Rapid flooding of the Sunda Shelf: a late-glacial sea-level record, Science, 288, 1033–1035, 2000.
Harting, P.: De bodem van het Eemdal, Verslagen en Verhandelingen Koninklijke Academie van Wetenschappen, 2e Reeks 8, 282–290, 1874.
Haslett, S. K. and Curr, R. H. F.: Stratigraphy and palaeoenvironmental development of Quaternary coarse clastic beach deposits at Plage de Mezpeurleuch, Brittany (France), Geol. J., 36, 171–182, https://doi.org/10.1002/GJ.888, 2001.
Hay, C., Mitrovica, J. X., Gomez, N., Creveling, J. R., Austermann, J., and E. Kopp, R.: The sea-level fingerprints of ice-sheet collapse during interglacial periods, Quaternary Sci. Rev., 87, 60–69, https://doi.org/10.1016/j.quascirev.2013.12.022, 2014.
Heyse, I.: Bijdrage tot de Geomorfologische Kennis van het Noordwesten van Oost-Vlaanderen (Belgie), Verhandelingen van de Koninklijke Academie voor Wetenschappen, Letteren en Schone Kunsten van Belgie, Klasse der Wetenschappen, Jaargang XLI, Vol. 155, 1979.
Hijma, M. P. and Cohen, K. M.: Holocene transgression of the Rhine river mouth area, The Netherlands/Southern North Sea: palaeogeography and sequence stratigraphy, Sedimentology, 58, 1453–1485, https://doi.org/10.1111/j.1365-3091.2010.01222.x, 2011.
Hijma, M. P. and Cohen, K. M.: Holocene sea-level database for the Rhine-Meuse Delta, The Netherlands: Implications for the pre-8.2 ka sea-level jump, Quaternary Sci. Rev., 214, 68–86, https://doi.org/10.1016/j.quascirev.2019.05.001, 2019.
Hijma, M. P., Cohen, K. M., Hoffmann, G., Van der Spek, A. J., and Stouthamer, E.: From river valley to estuary: the evolution of the Rhine mouth in the early to middle Holocene (western Netherlands, Rhine-Meuse delta), Neth. J. Geosci., 88, 13–53, https://doi.org/10.1017/S0016774600000986, 2009.
Hijma, M. P., Cohen, K. M., Roebroeks, W., Westerhoff, W. E., and Busschers, F. S.: Pleistocene Rhine–Thames landscapes: geological background for hominin occupation of the southern North Sea region, J. Quaternary Sci., 27, 17–39, https://doi.org/10.1002/jqs.1549, 2012.
Hijma, M., Engelhart, S. E., Törnqvist, T. E., Horton, B. P., Hu, P., and Hill, D. F.: A protocol for a geological sea-level database, in: Handbook of sea-level research, edited by: Shennan, I., Long, A. J., and Horton, B. P., John Wiley & Sons, Ltd, Chichester, UK, 536–553, https://doi.org/10.1002/9781118452547.ch34, 2015.
Hinton, M. A. C. and Kennard, A. S.: Contributions to the Pleistocene geology of the Thames Valley. 1. The Grays–Thurrock area, part 1, Essex Naturalist, 11, 226–270, 1901.
Hoare, P. G., Gale, S. J., Robinson, R. A. J., Connell, E. R., and Larkin, N. R.: Marine Isotope Stage 7–6 transition age for beach sediments at Morston, north Norfolk, UK: implications for Pleistocene chronology, stratigraphy and tectonics, J. Quaternary Sci., 24, 311–316, 2009.
Höfle, H. G., Merkt, J., and Müller, H.: Die Ausbreitung des Eem-Meeres in Nordwestdeutschland, Eiszeitalter und Gegenwart, 35, 49–59, 1985.
Hodgson, J. M.: The low-level Pleistocene marine sands and gravels of the West Sussex Coastal Plain, P. Geologist. Assoc., 75, 547–562, 1964.
Hollin, J. T.: Thames interglacial sites, Ipswichian sea levels and Antarctic ice surges, Boreas, 6, 33–52, https://doi.org/10.1002/jqs.3390080407, 1977.
Holyoak, D. T. and Preece, R. C.: Late Pleistocene Interglacial Deposits at Tattershall, Lincolnshire, Philos. T. Roy. Soc. B, 311, 193–236, 1985.
Houmark-Nielsen, M.: Pleistocene stratigraphy and glacial history of the central part of Denmark, B. Geol. Soc. Denmark, https://doi.org/10.37570/bgsd-1988-36-01, 1987.
James, H. C. L.: Aspects of the raised beaches of South Cornwall, Proc. Ussher, 2, 55–56, 1968.
James, H. C. L.: An examination of recently exposed Pleistocene sections at Godrevy, Proc. Ussher, 3, 299–301, 1975.
James, H. C. L.: Raised beaches of West Cornwall and their evolving geochronology, Proc. Ussher, 8, 437–440, 1995.
James, H. C. L.: An extension of the well-known Quaternary section at Godrevy, St Ives Bay, West Cornwall: Analysis and review, Proc. Ussher, 12, 55–57, 2008.
Jelgersma, S.: Post-glacial rise of sea-level in the Netherlands (a preliminary report), Geol. Mijnbouw, 39, 201–207, 1960.
Jelgersma, S.: Holocene sea-level changes in the Netherlands, PhD thesis, Leiden Univ. Med. Geol. St., Serie C 6, 1–100, 1961.
Jelgersma, S.: Sea-level changes in the North Sea basin, in: The Quaternary history of the North Sea. Symposia Universitatis Upsaliensis annum quingen-tesimum celebrantis, 2, 238–248, 1979.
Jessen, A., Milthers, V., Nordmann, V., Hartz, N., and Hesselboe, A.: En Boring gennem de Kvartaere Lag ved Skaerumhede, Danmarks Geologiske Undersøgelse, Series II, 25, 175 pp., 1910.
Jessen, K. and Milthers, V.: Stratigraphical and palaeontological studies of interglacial fresh-water deposits in Jutland and northwest Germany, Danmarks Geologiske Undersøgelse, Series II, 48, 1–379, 1928.
Kasse, C., van der Woude, J. D., Woolderink, H. A., and Schokker, J.: Eemian to Early Weichselian regional and local vegetation development and sedimentary and geomorphological controls, Amersfoort Basin, The Netherlands, Neth. J. Geosci., 101, E7, https://doi.org/10.1017/njg.2022.4, 2022.
Keen, D. H., Harmon, R. S., and Andrews, J. T.: U-series and amino acid dates from Jersey, Nature, 289, 162–164, https://doi.org/10.1038/289162a0, 1981.
Keogh, M. E. and Törnqvist, T. E.: Measuring rates of present-day relative sea-level rise in low-elevation coastal zones: a critical evaluation, Ocean Sci., 15, 61–73, https://doi.org/10.5194/os-15-61-2019, 2019.
Keogh, M. E., Törnqvist, T. E., Kolker, A. S., Erkens, G., and Bridgeman, J. G.: Organic Matter Accretion, Shallow Subsidence, and River Delta Sustainability, J. Geophys. Res.-Earth., 126, e2021JF006231, https://doi.org/10.1029/2021JF006231, 2021.
Kiden, P., Denys, L., and Johnston, P.: Late Quaternary sea-level change and isostatic and tectonic land movements along the Belgian–Dutch North Sea coast: geological data and model results, J. Quaternary Sci., 17, 535–546, https://doi.org/10.1002/jqs.709, 2002.
Kidson, C. and Heyworth, A.: The Quaternary deposits of the Somerset Levels, Q. J. Eng. Geol. Hydroge., 9, 217–235, https://doi.org/10.1144/GSL.QJEG.1976.009.03.05, 1976.
Knudsen, K.-L.: Foraminiferal faunas in Eemian deposits of the Oldenbüttel area near the Kiel Canal, Germany, Geol. Jb., A86, 27–47, 1985.
Knudsen, K. L. and Lykke-Andersen, A.-L.: Foraminifera in Late Saalian, Eemian, Early and Middle Weichselian of the Skaerumhede I boring, B. Geol. Soc. Denmark, 30, 97–109, 1982.
Knudsen, K. L., Kristensen, P., and Larsen, N. K.: Marine glacial and interglacial stratigraphy in Vendsyssel, northern Denmark: foraminifera and stable isotopes, Boreas, 38, 787–810, 2009.
Konradi, P. B.: Foraminifera in Eemian deposits at Stensigmose, southern Jutland, Danmarks Geologiske Undersøkelse, Series II, 105, 57 pp., 1976.
Konradi, P. B., Larsen, B., and Sørensen, A. B.: Marine Eemian in the Danish eastern North Sea, Quatern. Int., 133, 21–31, https://doi.org/10.1016/j.quaint.2004.10.003, 2005.
Kooi, H., Johnston, P., Lambeck, K., Smither, C., and Molendijk, R.: Geological causes of recent (∼ 100 yr) vertical land movement in the Netherlands, Tectonophysics, 299, 297–316, https://doi.org/10.1016/S0040-1951(98)00209-1, 1998.
Kopp, R. E., Simons, F. J., Mitrovica, J. X., Maloof, A. C., and Oppenheimer, M.: Probabilistic assessment of sea level during the last interglacial stage, Nature, 462, 863–867, https://doi.org/10.1038/nature08686, 2009.
Kosack, B. and Lange, W.: Das Eem-Vorkommen von Offenbüttel/Schnittlohe und die Ausbreitung des Eem-Meeres zwischen Nord-und Ostsee. Geologisches Jahrbuch. Reihe A, Allgemeine und regionale Geologie BR Deutschland und Nachbargebiete, Tektonik, Stratigraphie, Paläontologie, 86, 3–17, 1985.
Kristensen, P., Gibbard, P., Knudsen, K. L., and Ehlers, J.: Last interglacial stratigraphy at Ristinge Klint, south Denmark, Boreas, 29, 103–116, https://doi.org/10.1111/j.1502-3885.2000.tb01204.x, 2000.
Kubisch, M. and Schönfeld, J.: Eine neue “Cyprinen-Ton”-Scholle bei Stohl (Schleswig-Holstein): Mikrofauna und Grobfraktionsanalyse von Sedimenten der Eemzeitlichen Ostsee, Meyniana, 37, 89–95, 1985.
Kuhlmann, G., Langereis, C., Munsterman, D., van Leeuwen, R. J., Verreussel, R., Meulenkamp, J., and Wong, T.: Chronostratigraphy of Late Neogene sediments in the southern North Sea Basin and paleoenvironmental interpretations, Palaeogeogr. Palaeocl., 239, 426–455, https://doi.org/10.1016/j.palaeo.2006.02.004, 2006a.
Kuhlmann, G., Langereis, C. G., Munsterman, D., Van Leeuwen, R. J., Verreussel, R., Meulenkamp, J. E., and Wong, T. E.: Integrated chronostratigraphy of the Pliocene-Pleistocene interval and its relation to the regional stratigraphical stages in the southern North Sea region, Neth. J. Geosci., 85, 19–35, https://doi.org/10.1017/S0016774600021405, 2006b.
Kukla, G. J., Bender, M. L., de Beaulieu, J. L., Bond, G., Broecker, W. S., Cleveringa, P., Gavin, J. E., Herbert, T. D., Imbrie, J., Jouzel, J., Keigwin, L. D., Knudsen, K.-L., McManus, J. F., Merkt, J., Muhs, D. R., Müller, Poore, R. Z., Porter, S. C., Seret, G., Shackleton, N. J., Turner, C., and Tzedakis, P. C.: Last Interglacial Climates, Quaternary Res., 58, 2–13, https://doi.org/10.1006/qres.2001.2316, 2002.
Lamb, R. M., Harding, R., Huuse, M., Stewart, M., and Brocklehurst, S. H.: The early Quaternary North Sea Basin, J. Geol. Soc., 175, 275–290, https://doi.org/10.1144/jgs2017-057, 2018.
Lambeck, K.: Late Devensian And Holocene Shorelines Of The British-Isles And North-Sea From Models Of Glacio-Hydro-Isostatic Rebound, J. Geol. Soc., 152, 437–448, 1995.
Lambeck, K., Purcell, A., Funder, S., KjæR, K. H., Larsen, E., and Moller, P. E. R.: Constraints on the Late Saalian to early Middle Weichselian ice sheet of Eurasia from field data and rebound modelling, Boreas, 35, 539–575, https://doi.org/10.1080/03009480600781875, 2006.
Lamplugh, G. W.: On the Bridlington and Dimlington Glacial Shell-beds, Geol. Mag., 8, 535–546, https://doi.org/10.1017/S0016756800159229, 1881.
Lamplugh, G. W.: Report on the buried cliff at Sewerby, near Bridlington, P. Yorks. Geol. Polytech. Soc., 9, 381–392, https://doi.org/10.1144/pygs.9.3.381, 1887.
Lang, J., Lauer, T., and Winsemann, J.: New age constraints for the Saalian glaciation in northern central Europe: Implications for the extent of ice sheets and related proglacial lake systems, Quaternary Sci. Rev., 180, 240–259, https://doi.org/10.1016/j.quascirev.2017.11.029, 2018.
Larsen, N. K., Krohn, C. F., Kronborg, C., Nielsen, O. B., and Knudsen, K. L.: Lithostratigraphy of the late Saalian to middle Weichselian Skaerumhede Group in Vendsyssel, northern Denmark, Boreas, 38, 762–786, https://doi.org/10.1111/j.1502-3885.2009.00102.x, 2009.
Lautridou, J.-P. and Cliquet, D.: Le Pléistocène supérieur de Normandie et peuplements préhistoriques, Quaternaire, 17, 187–206, https://doi.org/10.4000/quaternaire.815, 2006.
Lautridou, J.-P., Baize, S., Clet, M., Coutard, J.-P., and Ozouf, J.-C.: Les séquences plio-pléistocènes littorales et estuariennes de Normandie, Quaternaire, 10, 161–169, 1999.
Lautridou, J.-P., Auguste, P., Carpentier, G., Cordy, J.-M., Lebret, P., Lechevalier, C., and Lefebvre, D.: L'Eemien et le Pléistocène moyen récent fluvio-marin et continental de la vallée de la Seine de Cléon au Havre (Normandie), Quaternaire, 14, 25–30, https://doi.org/10.3406/quate.2003.1726, 2003.
Lewis, S., G., Ashton, N., and Jacobi, R.: 9 – Testing Human Presence During the Last Interglacial (MIS 5e): A Review of the British Evidence, in: Developments in Quaternary Sciences, edited by: Ashton, N., Lewis, S. G., and Stringer, C., Elsevier, 125–164, https://doi.org/10.1016/B978-0-444-53597-9.00009-1, 2011.
Lisiecki, L. E. and Raymo, M. E.: A Pliocene-Pleistocene stack of 57 globally distributed benthic δ18O records, Paleoceanogr., 20, PA1003, https://doi.org/10.1029/2004PA001071, 2005.
Long, A. J., Woodroffe, S. A., Roberts, D. H., and Dawson, S.: Isolation basins, sea-level changes and the Holocene history of the Greenland Ice Sheet, Quaternary Sci. Rev., 30, 3748–3768, https://doi.org/10.1016/j.quascirev.2011.10.013, 2011.
Long, A. J., Barlow, N. L. M., Busschers, F. S., Cohen, K. M., Gehrels, W. R., and Wake, L. M.: Near-field sea-level variability in northwest Europe and ice sheet stability during the last interglacial, Quaternary Sci. Rev., 126, 26–40, https://doi.org/10.1016/j.quascirev.2015.08.021, 2015.
Lorié, J.: De Geologische Bouw der Gelderse Vallei, benevens Beschrijving van eenige nieuwe grondboringen, VII – Verh. Kon. Akad. Wetensch., 2-12-1, 1–100, 1906.
Loyer, S., van Vliet-Lanoë, B., Monnier, J.L., Hallegouet, B., and Mercier, N.: La coupe de Nantois (Baie de Saint-Brieuc, France): Datations par thermoluminescence (TL) et données paléoenvironnementales nouvelles pour le Pléistocène de Bretagne, Quaternaire, 6, 21–33, https://doi.org/10.3406/quate.1995.2034, 1995.
Mackie, S. J.: On a deposit at Folkestone containing bones of Mammalia, Quarterly J. Geol. Soc. London, VII, 257–262, 1851.
Madsen, V. C., Nordmann, V. J. H., and Hartz, N. E. K.: Eem-zonerne: Studier over Cyprinaleret og andre Eem-aflejringer i Danmark, Nord-Tyskland og Holland, Danmarks Geologiske Undersøgelse, Series II, 17–19, 1908.
Martinius, A. W. and Van den Berg, J. H.: Atlas of sedimentary structures in estuarine and tidally-influenced river deposits of the Rhine-Meuse-Scheldt system, Houten, EAGE, 298 pp., ISBN 9789073834118, 2011.
Mathys, M.: The Quaternary geological evolution of the Belgian Continental Shelf, southern North Sea, PhD thesis, Ghent University, 382 pp., http://hdl.handle.net/1854/LU-716421, 2009.
Mauz, B., Vacchi, M., Green, A., Hoffmann, G., and Cooper, A.: Beachrock: a tool for reconstructing relative sea level in the far-field, Mar. Geol., 362, 1–16, https://doi.org/10.1016/j.margeo.2015.01.009, 2015.
Meijer, T.: The late Middle Pleistocene non-marine molluscan fauna of borehole Noorderhoeve-19E117 (province of Noord-Holland, the Netherlands), Cainozoic Research, 2, 129–134, 2002.
Meijer, T. and Preece, R. C.: Malacological evidence relating to the insularity of the British Isles during the Quaternary, Geol. Soc. London, Spec. Publ. 96, 89–110, 1995.
Meijer, T. and Preece, R.C.: A review of the occurrence of Corbicula in the Pleistocene of North-West Europe, Neth. J. Geosc., 79, 241–255, https://doi.org/10.1017/S0016774600021739, 2000.
Meijer, T. and Cleveringa, P.: Aminostratigraphy of Middle and Late Pleistocene deposits in The Netherlands and the southern part of the North Sea Basin, Global Planet. Change, 68, 326–345, 2009.
Meijer, T., Pouwer, R., Cleveringa, P., de Wolf, H., Busschers, F. S., and Wesselingh, F. P.: Fossil molluscs from borehole Hollum (Ameland, the Netherlands) constrain three successive Quaternary interglacial marine intervals in the southern North Sea Basin, Neth. J. Geosci., 100, e13, 2021.
Mellett, C. L., Mauz, B., Plater, A. J., Hodgson, D. M., and Lang, A.: Optical dating of drowned landscapes: A case study from the English Channel, Quat. Geochronol., 10, 201–208, https://doi.org/10.1016/j.quageo.2012.03.012, 2012.
Mellett, C. L., Hodgson, D. M., Plater, A. J., Mauz, B., Selby, I., and Lang, A.: Denudation of the continental shelf between Britain and France at the glacial–interglacial timescale, Geomorphology, 203, 79–96, https://doi.org/10.1016/j.geomorph.2013.03.030, 2013.
Meng, S., Börner, A., Menzel-Harloff, H., Strahl, J., and Müller, U.: Palaeo-ecological development and interpretation of the macrofauna inventory (Bivalvia and Gastropoda) in marine Eemian deposits at Warnow Bay (NE Germany), Quatern. Int., 630, 84–96, https://doi.org/10.1016/j.quaint.2021.05.008, 2022.
Menke, B.: Palynologische Untersuchungen zur Transgression des Eem-Meeres im Raum Offenbüttel/Nord-Ostsee-Kanal, Geologisches Jahrbuch A, 86, 19–26, 1985.
Menke, B. and Tynni, R.: Das Eeminterglazial und das Weichselfruh-glazial von Rederstall/Dithmarschen und ihre Bedeutung für die mitteleuropaische Jungpleistozan-Gliederung, Geologisches Jahrbuch A, 76, 1–109, 1984.
Miller, G. H. and Mangerud, J.: Aminostratigraphy of European marine interglacial deposits, Quaternary Sci. Rev., 4, 215–278, https://doi.org/10.1016/0277-3791(85)90002-2, 1985.
Miller, G. H., Hollin, J. T., and Andrews, J. T.: Aminostratigraphy of UK Pleistocene deposits, Nature, 281, 539–543, 1979.
Missiaen, T., Fitch, S., Muru, M., Harding, R., Fraser, A., De Clercq, M., Garcia Moreno, D. G., Versteeg, W., Busschers, F., van Heteren, S., Hijma, M., Reichart, G.-J., and Gaffney, V.: Targeting the mesolithic: Interdisciplinary approaches to archaeological prospection in the Brown Bank area, southern North Sea, Quatern. Int., 584, 141–151, 2020.
Mitchell, G. F.: The Pleistocene history of the Irish Sea, BAAS Adv. Sci., 17, 313–325, 1960.
Mitchell, G. F.: The Pleistocene history of the Irish Sea: Second approximation, Sci. P. R. Dublin Soc., 4, 181–199, 1972.
Mitrovica, J. X., Gomez, N., and Clark, P. U.: The Sea-Level Fingerprint of West Antarctic Collapse, Science, 323, 753, https://doi.org/10.1126/science.1166510, 2009.
Monnier, J.-L., Huet, B., and Laforge, M.: Application of sedimentological analysis to correlation of eroded layers under beaches with local and regional Pleistocene stratigraphy: A contribution to geological dating of Palaeolithic sites, northern coast of Brittany, France, Quatern. Int., 231, 78–94, https://doi.org/10.1016/j.quaint.2010.06.033, 2011.
Moreau, J., Huuse, M., Janszen, A., van der Vegt, P., Gibbard, P. L., and Moscariello, A.: The glaciogenic unconformity of the southern North Sea, Geol. Soc. London Spec. Publ., 368, 99–110, 2012.
Morzadec-Kerfourn, M. T. and Monnier, J. L.: Chronologie relative des cordons littoraux pléistocènes de Bretagne, B. Assoc. Fr. Etud. Quaternaire, 4, 195–203, 1982.
Mottershead, D. N., Gilbertson, D. D., and Keen, D. H.: The raised beaches and shore platforms of Tor Bay: a re-evaluation, P. Geologist. Assoc., 98, 241–257, 1987.
Müller, H.: Pollenanalytische Untersuchungen und Jahresschichtenzahlung an der eem-zeitlichen Kieselgur von Bispingen/Luhe, Geologisches Jahrbuch, A-21, 149–169, 1974.
Murchison, C.: Mastodon, elephant, rhinoceros, ossiferous caves, primeval man and his contemporaries. Palaeontological Memoirs and Notes of the Late High Falconer, Vol. II. London, 1868.
Murray, A. S. and Funder, S.: Optically stimulated luminescence dating of a Danish Eemian coastal marine deposit: a test of accuracy, Quaternary Sci. Rev., 22, 1177–1183, 2003.
NEEM community members: Eemian interglacial reconstructed from a Greenland folded ice core, Nature, 493, 489–494, https://doi.org/10.1038/nature11789, 2013.
Nelson, A. R.: Coastal sediments, in: Handbook of Sea-Level Research, edited by: Shennan, I., Long, A. J., and Horton, B. P., 47–65, https://doi.org/10.1002/9781118452547.ch4, 2015.
Nolf, D.: Mollusken uit het marien Kwartair te Meetkerke (West-Vlaanderen, België), Natuurwetenschappelijk Tijdschrift, 55, 97–120, 1973.
Nordmann, V.: La Position stratigraphique des Depots d'Eem. Danmarks Geologiske Undersøgelse, Series II, 47, 81 pp., 1928.
O'Leary, M. J., Hearty, P. J., Thompson, W. G., Raymo, M. E., Mitrovica, J. X., and Webster, J. M.: Ice sheet collapse following a prolonged period of stable sea level during the last interglacial, Nat. Geosci., 6, 796–800, 2013.
Orme, A. R.: The Raised Beaches and Strandlines of South Devon, Field Studies Journal, 1, 109–130, https://fsj.field-studies-council.org/media/344669/vol1.2_15.pdf (last access: 19 June 2022), 1960.
Ottesen, D., Dowdeswell, J. A., and Bugge, T.: Morphology, sedimentary infill and depositional environments of the Early Quaternary North Sea Basin (56–62 N), Mar. Petrol. Geol., 56, 123–146, 2014.
Otvos, E. G.: Beach ridges–definitions and significance, Geomorphology, 32, 83–108, 2000.
Paddenberg, D., Russel, J., and Tizzard, L.: Seabed Prehistory: Gauging the Effects of Marine Aggregate Dredgin – Final Report: Volume IV – Great Yarmouth, London, https://doi.org/10.5284/1009322, 2008.
Palmer, L. S. and Cooke, J. H.: The Pleistocene deposits of the Portsmouth district and their relation to man, P. Geologist. Assoc., 34, 253–292, 1923.
Paul, M. A., Barras, B. F., and Mein, J. E.: Geotechnical properties of British estuarine clays: towards a geological framework, in: Advances in geotechnical engineering: The Skempton conference: Proceedings of a three day conference on advances in geotechnical engineering, Th. Telford, 568–579, https://doi.org/10.1680/aigev1.32644.0032, 2004.
Pedoja, K., Husson, L., Regard, V., Cobbold, P.R., Ostanciaux, E., Johnson, M. E., Kershaw, S., Saillard, M., Martinod, J., Furgerot, L., Weill, P., and Delcaillau, B.: Relative sea-level fall since the last interglacial stage: Are coasts uplifting worldwide?, Earth-Sci. Rev., 108, 1–15, https://doi.org/10.1016/j.earscirev.2011.05.002, 2011.
Pedoja, K., Husson, L., Johnson, M.E., Melnick, D., Witt, C., Pochat, S., Nexer, M., Delcaillau, B., Pinegina, T., Poprawski, Y., Authemayou, C., Elliot, M., Regard, V., and Garestier, F.: Coastal staircase sequences reflecting sea-level oscillations and tectonic uplift during the Quaternary and Neogene, Earth-Sci. Rev., 132, 13–38, https://doi.org/10.1016/j.earscirev.2014.01.007, 2014.
Pedoja, K., Jara-Muñoz, J., de Gelder, G., Robertson, J., Meschis, M., Fernandez-Blanco, D., Nexer, M., Poprawski, Y., Dugué, O., Delcaillau, B., Bessin, P., Benabdelouahed, M., Authemayou, C., Husson, L., Regard, V., Menier, D., and Pinel, B.: Neogene-Quaternary slow coastal uplift of Western Europe through the perspective of sequences of strandlines from the Cotentin Peninsula (Normandy, France), Geomorphology, 303, 338–356, https://doi.org/10.1016/j.geomorph.2017.11.021, 2018.
Peeters, J., Busschers, F. S., and Stouthamer, E.: Fluvial evolution of the Rhine during the last interglacial-glacial cycle in the southern North Sea basin: a review and look forward, Quatern. Int., 357, 176–188, 2015.
Peeters, J., Busschers, F. S., Stouthamer, E., Bosch, J. H. A., Van den Berg, M. W., Wallinga, J., Versendaal, A. J., Bunnik, F. P. M., and Middelkoop, H.: Sedimentary architecture and chronostratigraphy of a late Quaternary incised-valley fill: a case study of the late Middle and Late Pleistocene Rhine system in the Netherlands, Quaternary Sci. Rev., 131, 211–236, https://doi.org/10.1016/j.quascirev.2015.10.015, 2016.
Peeters, J., Cohen, K. M., Thrana, C., Busschers, F. S., Martinius, A. W., Stouthamer, E., and Middelkoop, H.: Preservation of Last Interglacial and Holocene transgressive systems tracts in the Netherlands and its applicability as a North Sea Basin reservoir analogue, Earth-Sci. Rev., 188, 482–497, https://doi.org/10.1016/j.earscirev.2018.10.010, 2019.
Pellerin, J. and Dupeuble, P. A.: Le bas niveau marin éémien de Graye-sur-Mer (Calvados), Bulletin de la Société linnéenne de Normandie, 107, 21–26, 1979.
Peltier, W. R.: Global glacial isostatic adjustment: palaeogeodetic and space geodetic test of the ICE-4G (VM2) model, J. Quaternary Sci., 17, 491–510, 2002.
Penkman, K.: Aminoacid geochronology: its impact on our understanding of the Quaternary stratigraphy of the British Isles, J. Quaternary Sci., 25, 501–514, 2010.
Penkman, K. E. H., Kaufman, D. S., Maddy, D., and Collins, M. J.: Closed-system behaviour of the intra-crystalline fraction of amino acids in mollusc shells, Quat. Geochronol., 3, 2–25, https://doi.org/10.1016/j.quageo.2007.07.001, 2008.
Penkman, K. E., Preece, R. C., Bridgland, D. R., Keen, D. H., Meijer, T., Parfitt, S. A., White, T. S., and Collins, M. J.: A chronological framework for the British Quaternary based on Bithynia opercula, Nature, 476, 446–449, 2011.
Penkman, K. E., Preece, R. C., Bridgland, D. R., Keen, D. H., Meijer, T., Parfitt, S. A., White, T. S., and Collins, M. J.: An aminostratigraphy for the British Quaternary based on Bithynia opercula, Quaternary Sci. Rev., 61, 111–134, 2013.
Penney, D. N.: Application of Ostracoda to sea-level studies, Boreas, 16, 237–247, 1987.
Pirazzoli, P. A.: Marine terraces, Encyclopedia of Coastal Science, Springer Netherlands, Dordrecht, https://doi.org/10.1007/978-3-319-48657-4_209-2, 632–633, 2005.
Preece, R. C.: Mollusca from Last Interglacial fluvial deposits of the River Thames at Trafalgar Square, London, J. Quaternary Sci., 14, 77–89, 1999.
Preece, R. C.: Molluscan evidence for differentiation of interglacials within the “Cromerian Complex”, Quaternary Sci. Rev., 20, 1643–1656, 2001.
Preece, R. C., Scourse, J. D., Houghton, S. D., Knudsen, K. L., and Penney, D. N.: The Pleistocene sea level and neotectonic history of the Eastern Solent, Southern England, Philos. T. R. Soc. B, 328, 425–477, https://doi.org/10.1016/j.quaint.2010.06.033, 1990.
Prestwich, J.: On the westward extension of the old raised beach of Brighton and on the extent of the sea-bed of the same period, Quarterly J. Geol. Soc. London, 15, 215–221, 1859.
Prestwich, J.: Notes on the phenomenon of the Quaternary period in the Isle of Portland and around Weymouth, Quarterly J. Geol. Soc. London, 31, 29–54, 1875.
Prestwich, J.: The raised beaches and “head” or rubble-drift of the South of England: their relation to the Valley Drifts and to the Glacial Period; and on a late post-Glacial submergence, Quarterly J. Geol. Soc. London, 48, 263–343, 1892.
Proctor, C. J. and Smart, P. L.: A dated cave sediment record of Pleistocene transgressions on Berry Head, Southwest England, J. Quaternary Sci., 6, 233–244, https://doi.org/10.1002/jqs.3390060306, 1991.
Regnauld, H., Mauz, B., and Morzadec-Kerfourn, M. T.: The last interglacial shoreline in northern Brittany, western France, Mar. Geol., 194, 65–77, https://doi.org/10.1016/S0025-3227(02)00699-0, 2003.
Reid, C.: The Pleistocene deposits of the Sussex Coast, and their equivalents in other districts, Quarterly J. Geol. Soc. London, 48, 344–346, https://doi.org/10.1144/GSL.JGS.1892.048.01-04.20, 1892.
Reid, C.: A fossiliferous Pleistocene deposit at Stone, on the Hampshire coast, Quarterly J. Geol. Soc. London, 49, 325–329, https://doi.org/10.1144/GSL.JGS.1893.049.01-04.51, 1893.
Reid, C.: Geology of the Country Around Bournemouth, Memoirs of the Geological Survey, England and Wales, New Series, No. 329, 1898.
Roe, H. M., Coope, G. R., Devoy, R. J. N., Harrison, C. J. O., Penkman, K. E. H., Preece, R. C., and Schreve, D. C.: Differentiation of MIS 9 and MIS 11 in the continental record: vegetational, faunal, aminostratigraphic and sea-level evidence from coastal sites in Essex, UK, Quaternary Sci. Rev., 28, 2342–2373, https://doi.org/10.1016/j.quascirev.2009.04.017, 2009.
Rohling, E. J., Hibbert, F. D., Williams, F. H., Grant, K. M., Marino, G., Foster, G. L., Hennekam, R., de Lange, G. J., Roberts, A. P., Yu, J., Webster, J. M., and Yokoyama, Y.: Differences between the last two glacial maxima and implications for ice-sheet, δ18O, and sea-level reconstructions, Quaternary Sci. Rev., 176, 1–28, https://doi.org/10.1016/j.quascirev.2017.09.009, 2017.
Rohling, E. J., Hibbert, F. D., Grant, K. M., Galaasen, E. V., Irvalı, N., Kleiven, H. F., Marino, G., Ninnemann, U., Roberts, A. P., Rosenthal, Y., Schulz, H., Williams, F. H., and Yu, J.: Asynchronous Antarctic and Greenland ice-volume contributions to the last interglacial sea-level highstand, Nat. Commun., 10, 5040, https://doi.org/10.1038/s41467-019-12874-3, 2019.
Rosentau, A., Klemann, V., Bennike, O., Steffen, H., Wehr, J., Latinović, M., Bagge, M., Ojala, A., Berglund, M., Peterson Becher, G., Schoning, K., Hansson, A., Nielsen, L., Clemmensen, L.B., Hede, M.U., Kroon, A., Pejrup, M., Sander, L., Stattegger, K., Schwarzer, K., Lampe, R., Lampe, M., Uścinowicz, S., Bitinas, A., Grudzinska, I., Vassiljev, J., Nirgi, T., Kublitskiy, Y., and Subetto, D. A: Holocene relative sea-level database for the Baltic Sea, Quaternary Sci. Rev., 266, 107071, https://doi.org/10.1016/j.quascirev.2021.107071, 2021.
Rovere, A., Raymo, M. E., Vacchi, M., Lorscheid, T., Stocchi, P., Gómez-Pujol, L., Harris, D. L., Casella, E., O'Leary, M. J., and Hearty, P. J.: The analysis of Last Interglacial (MIS 5e) relative sea-level indicators: Reconstructing sea-level in a warmer world, Earth-Sci. Rev., 159, 404–427, https://doi.org/10.1016/j.earscirev.2016.06.006, 2016.
Rovere, A., Ryan, D., Murray-Wallace, C., Simms, A., Vacchi, M., Dutton, A., Lorscheid, T., Chutcharavan, P., Brill, D., Bartz, M., Jankowski, N., Mueller, D., Cohen, K., and Gowan, E.: Descriptions of database fields for the World Atlas of Last Interglacial Shorelines (WALIS) (Version 1.0), Zenodo [code], https://doi.org/10.5281/zenodo.3961544, 2020.
Rubio-Sandoval, K., Rovere, A., Cerrone, C., Stocchi, P., Lorscheid, T., Felis, T., Petersen, A.-K., and Ryan, D. D.: A review of last interglacial sea-level proxies in the western Atlantic and southwestern Caribbean, from Brazil to Honduras, Earth Syst. Sci. Data, 13, 4819–4845, https://doi.org/10.5194/essd-13-4819-2021, 2021.
Russell, J. and Tizzard, L.: Seabed Prehistory: Site Evaluation Techniques (Area 240) Synthesis, Wessex Archaeology, https://doi.org/10.5284/1008287, 2011.
Sanchez-Goñi, M. F., Eynaud, F., Turon, J., and Shackleton, N. J.: High resolution palynological record off the Iberian margin: direct land-sea correlation for the Last Interglacial complex, Earth Planet. Sc. Lett., 171, 123–137, https://doi.org/10.1016/S0012-821X(99)00141-7, 1999.
Schaumann, R. M., Capperucci, R. M., Bungenstock, F., McCann, T., Enters, D., Wehrmann, A., and Bartholomä, A.: The Middle Pleistocene to early Holocene subsurface geology of the Norderney tidal basin: new insights from core data and high-resolution sub-bottom profiling (Central Wadden Sea, southern North Sea), Neth. J. Geosci., 100, e15, https://doi.org/10.1017/njg.2021.3, 2021.
Schreve, D. C.: Differentiation of the British late Middle Pleistocene interglacials: the evidence from mammalian biostratigraphy, Quaternary Sci. Rev., 20, 1693–1705, 2001.
Schulz, H., Emeis, K.-C., Winn, K., and Erlenkeuser, H.: Oberflächentemperaturen des Eem-Meeres in Schlesvig-Holstein–die UK'37-Indizien, Meyniana, 53, 163–181, 2001.
Scourse, J. D.: Late Pleistocene stratigraphy of North and West Cornwall, Transactions of the Royal Geological Society Cornwall, 22, 2–56, 1996.
Seidenkrantz, M. S.: Benthic foraminiferal and stable isotope evidence for a “Younger Dryas-style” cold spell at the Saalian-Eemian transition, Denmark, Palaeogeogr. Palaeocl., 102, 103–120, https://doi.org/10.1016/0031-0182(93)90008-7, 1993.
Selle, W.: Geologische und vegetationskundliche Untersuchungen an einigen wichtigen Vorkommen des letzen Interglazials in Nordwestdeutschland, Geologische Jahrbuch, 79, 295–352, 1962.
Selle, W. and Schneekloth, H.: Ergebnisse einer Kernbohrung in Oerel, Krs. Bremervörde; drei Interstadiale über Ablagerungen des Eem-Interglazials, Zeitschrift der Deutschen Geologischen Gesellschaft, 115, 109–117, 1965.
Sha, L. P. (Ed.), Schwarz, C., Maenhout van Lemberge, V, Cameron, T. D. J., Zollmer, V., Konradi, P., Laban, C., Streif, H., and Schuttenhelm, R. T. E.: Quaternary sedimentary sequences in the southern North Sea Basin. Sedimentological Working Group of the Southern North Sea Project, Commission of the European Communities (contract no. SCI* -128-C 9EDB), 1991.
Shackleton, N. J., Chapman, M., Sanchez-Goñi, M. F., Pailler, D., and Lancelot, Y.: The classic marine isotope substage 5e, Quaternary Res., 58, 14–16, https://doi.org/10.1006/qres.2001.2312, 2002.
Shackleton, N. J., Sanchez-Goñi, M. F., Pailler, D., and Lancelot, Y.: Marine isotope substage 5e and the Eemian interglacial, Global Planet. Change, 36, 151–155, https://doi.org/10.1016/S0921-8181(02)00181-9, 2003.
Shennan, I.: Interpretation of Flandrian sea-level data from the Fenland, England, P. Geologist. Assoc., 93, 53–63, 1982.
Shennan, I.: Flandrian sea-level changes in the Fenland I. The geographical setting and evidence of relative sea-level changes, J. Quaternary Sci., 1, 119–154, 1986.
Shennan, I.: Holocene crustal movements and sea-level changes in Great Britain, J. Quaternary Sci., 4, 77–89, 1989.
Shennan, I., Hamilton, S., Hillier, C., and Woodroffe, S.: A 16,000-year record of near-field relative sea-level changes, northwest Scotland, United Kingdom, Quatern. Int., 133–34, 95–106, 2005.
Shennan, I., Long, A. J., and Horton, B. P.: Handbook of Sea-Level Research, John Wiley & Sons, Ltd, Chichester, UK, https://doi.org/10.1002/9781118452547, 2015.
Shennan, I., Bradley, S. L., and Edwards, R.: Relative sea-level changes and crustal movements in Britain and Ireland since the Last Glacial Maximum, Quaternary Sci. Rev., 188, 143–159, https://doi.org/10.1016/j.quascirev.2018.03.031, 2018.
Sier, M. J., Peeters, J., Dekkers, M. J., Pares, J. M., Chang, L., Busschers, F. S., Cohen, K. M., Wallinga, J., Bunnik, F. P. M., and Roebroeks, W.: The Blake Event recorded near the Eemian type locality? a diachronic onset of the Eemian in Europe, Quat. Geochronol., 28, 12–28, 2015.
Sirocko, F., Seelos, K., Schaber, K., Rein, B., Dreher, F., Diehl, M., Lehne, R., Jäger, K., Krbetschek, M., and Degering, D.: A late Eemian aridity pulse in central Europe during the last glacial inception, Nature, 436, 833–836, https://doi.org/10.1038/nature03905, 2005.
Southgate, G. A.: Thermoluminescence dating of beach and dune sands: potential of single-grain measurements, Nuclear Tracks, 10, 743–747, https://doi.org/10.1016/0735-245X(85)90084-5, 1985.
Sparks, B. W.: The non-marine Mollusca of the interglacial deposits at Bobbitshole, Ipswich, Philos. T. Roy. Soc. B, 241, 33–44, https://doi.org/10.1098/rstb.1957.0007, 1957.
Sparks, B. W. and West, R. G.: The Interglacial Deposits at Stutton, Suffolk, P. Geologist. Assoc., 74, 419–432, 1963.
Sparks, B. W. and West, R. G.: Late Pleistocene deposits at Wretton, Norfolk. I. Ipswichian interglacial deposits, Philos. T. Roy. Soc. B, 258, 1–30, https://doi.org/10.1098/rstb.1970.0030, 1970.
Straw, A.: Geomorphology of the Kirmington interglacial deposits and the Immingham channel, north Lincolnshire, Mercian Geologist, 19, 134–140, 2018.
Streif, H.: Quaternary Sea-Level Changes in the North Sea, an Analysis of Amplitudes and Velocities, in: Earth's Rotation from Eons to Days, edited by: Brosche, P. and Sünderman, J., 201–214, https://doi.org/10.1007/978-3-642-75587-3_21, 1990.
Streif, H.: Zum Ausmass und Ablauf eustatischer Meeresspiegelschwankungen im südlichen Nordseegebiet seit Beginn des Letzten Interglazials, in: Klimageschichtliche Probleme der letzten 130 000 Jahre, edited by: Frenzel, B., Fisher, New York, Stuttgart, 231–249, ISBN 9783437304590, 1991.
Streif, H.: Sedimentary record of Pleistocene and Holocene marine inundations along the North Sea coast of Lower Saxony, Germany, Quatern. Int., 112, 3–28, 2004.
Stringer, C. B., Currant, A. P., Schwarcz, H. P., and Collcutt, S. N.: Age of Pleistocene faunas from Bacon Hole, Wales, Nature, 320, 59–62, 1986.
Sundelin, U.: Fornsjöstudier inom Stångåns och Svartåns vattenområden med särskild hänsyn till den sen-och postglaciala klimatutvecklingen, Sveriges geologiska undersökning, 16, 1917.
Sutcliffe, A. J. and Currant, A. P.: Minchin Hole Cave, in: Wales: Gower, Preseli and Forest Fawr, edited by: Bowen, D. Q. and Henry, A., Quaternary Research Association Field Guide, Durham, 33–37, 1984.
Sutcliffe, A. J., Currant, A. P., and Stringer, C. B.: Evidence of sea-level change from coastal caves with raised beach deposits, terrestrial faunas and dated stalagmites, Prog. Oceanogr., 18, 243–271, 1987.
Sutherland, J. L., Davies, B. J., and Lee, J. R.: A litho-tectonic event stratigraphy from dynamic Late Devensian ice flow of the North Sea Lobe, Tunstall, east Yorkshire, UK, P. Geologist. Assoc., 131, 168–186, https://doi.org/10.1016/j.pgeola.2020.03.001, 2020.
Svendsen, J. I., Alexanderson, H., Astakhov, V. I., Demidov, I., Dowdeswell, J. A., Funder, S., Gataullin, V., Henriksen, M., Hjort, C., Houmark-Nielsen, M., Hubberten, H. W., Ingólfsson, Ó., Jakobsson, M., Kjær, K. H., Larsen, E., Lokrantz, H., Lunkka, J. P., Lyså, A., Mangerud, J., Matiouchkov, A., Murray, A., Möller, P., Niessen, F., Nikolskaya, O., Polyak, L., Saarnisto, M., Siegert, C., Siegert, M. J., Spielhagen, R. F., and Stein, R.: Late Quaternary ice sheet history of northern Eurasia, Quaternary Sci. Rev., 23, 1229–1271, https://doi.org/10.1016/j.quascirev.2003.12.008, 2004.
Tamisiea, M. E., Mitrovica, J. X., Milne, G. A., and Davis, J. L.: Global geoid and sea level changes due to present-day ice mass fluctuations, J. Geophys. Res.-Sol. Ea., 106, 30849–30863, https://doi.org/10.1029/2000jb000011, 2001.
Tastet, J. P.: Le Pléistocène de la façade atlantique du Nord-Médoc (France): état des connaissances sur la lithologie et la chronostratigraphie des “Argiles du Gurp”, Quaternaire, 10, 199–212, https://doi.org/10.3406/quate.1999.1643, 1999.
Thomas, G.: Late Middle Pleistocene pollen biostratigraphy in Britain: Pitfalls and possibilities in the separation of interglacial sequences, Quaternary Sci. Rev., 20, 1621–1630, https://doi.org/10.1016/S0277-3791(01)00026-9, 2001.
Törnqvist, T. E., Wallinga, J., and Busschers, F. S.: Timing of the last sequence boundary in a fluvial setting near the highstand shoreline — Insights from optical dating, Geology, 31, 279–282, 2003.
Toucanne, S., Zaragosi, S., Bourillet, J. F., Marieu, V., Cremer, M., Kageyama, M., Van Vliet-Lanoë, B., Eynaud, F., Turon, J.-L., and Gibbard, P. L.: The first estimation of Fleuve Manche palaeoriver discharge during the last deglaciation: Evidence for Fennoscandian ice sheet meltwater flow in the English Channel ca 20–18 ka ago, Earth Planet. Sc. Lett., 290, 459–473, 2010.
Turner, C.: The Eemian interglacial in the North European plain and adjacent areas, Geol. Mijnbouw/Neth. J. Geosci., 79, 217–231, https://doi.org/10.1017/S0016774600023660, 2000.
Turner, C.: Problems of the duration of the Eemian interglacial in Europe North of the Alps, Quaternary Res., 58, 45–48, https://doi.org/10.1006/qres.2002.2366, 2002.
Turner, C. and West, R.: The subdivision and zonation of interglacial periods, Eiszeitalter und Gegenwart, 19, 93–101, 1968.
Turney, C. S. M., Fogwill, C. J., Golledge, N. R., McKay, N. P., van Sebille, E., Jones, R. T., Etheridge, D., Rubino, M., Thornton, D. P., Davies, S. M., Ramsey, C. B., Thomas, Z. A., Bird, M. I., Munksgaard, N. C., Kohno, M., Woodward, J., Winter, K., Weyrich, L. S., Rootes, C. M., Millman, H., Albert, P. G., Rivera, A., van Ommen, T., Curran, M., Moy, A., Rahmstorf, S., Kawamura, K., Hillenbrand, C.-D., Weber, M. E., Manning, C. J., Young, J., and Cooper, A.: Early Last Interglacial ocean warming drove substantial ice mass loss from Antarctica, P. Natl. A. Sci. USA, 117, 3996–4006, https://doi.org/10.1073/pnas.1902469117, 2020.
Tzedakis, C.: Timing and duration of Last Interglacial conditions in Europe: a chronicle of a changing chronology, Quaternary Sci. Rev., 22, 763–768, https://doi.org/10.1016/S0277-3791(03)00004-0, 2003.
Tzedakis, P. C., Drysdale, R. N., Margari, V., Skinner, L. C., Menviel, L., Rhodes, R. H., Taschetto, S., Hodell, D. A., Crowhurst, S. J., Hellstrom, J. C., Fallick, A. E., Grimalt, J. O., McManus, J. F., Martrat, B., Mokeddem, Z., Parrenin, F., Regattieri, E., Roe, K., and Zanchetta, G.: Enhanced climate instability in the North Atlantic and southern Europe during the Last Interglacial, Nat. Commun., 9, 1–14, https://doi.org/10.1038/s41467-018-06683-3, 2018.
Ussher, W. A. E.: The post-tertiary geology of Cornwall, Stephen Austin and Sons, Hertford, 1879.
Van Asselen, S., Karssenberg, D., and Stouthamer, E.: Contribution of peat compaction to relative sea-level rise within Holocene deltas, Geophys. Res. Lett., 38, L24401, https://doi.org/10.1029/2011gl049835, 2011.
Van de Plassche, O.: Sea-level change and water movements in the Netherlands during the Holocene, Mededelingen Rijks Geologische Dienst, 36, 1–93, https://puc.overheid.nl/doc/PUC_147237_31 (last access: 19 June 2022), 1982.
Van de Plassche, O.: Sea-Level Research: a manual for the collection and evaluation of data, GeoBooks, Norwich, 618 pp., https://doi.org/10.1007/978-94-009-4215-8, 1986.
Van de Plassche, O.: Evolution of the intra-coastal tidal range in the Rhine-Meuse delta and Flevo Lagoon, 5700–3000 yrs cal BC, Mar. Geol., 124, 113–128, 1995.
Van der Meulen, M. J., Doornenbal, J. C., Gunnink, J. L., Stafleu, J., Schokker, J., Vernes, R. W., Van Geer, F. C., Van Gessel, S. F., Van Heteren, S., Van Leeuwen, R. J. W., and Bakker, M. A.: 3D geology in a 2D country: perspectives for geological surveying in the Netherlands, Neth. J. Geosci., 92, 217–241, https://doi.org/10.1017/S0016774600000184, 2013.
Van Leeuwen, R. J., Beets, D. J., Bosch, J. H. A., Burger, A. W., Cleveringa, P., Harten, D. V., Herngreen, G. F. W., Kruk, R. W., Langereis, C. G., Meijer, T., and Pouwer, R.: Stratigraphy and integrated facies analysis of the Saalian and Eemian sediments in the Amsterdam-Terminal borehole, the Netherlands, Neth. J. Geosci., 79, 161–196, https://doi.org/10.1017/S0016774600023647, 2000.
Van Vliet-Lanoë, B., Laurent, M., Bahain, J. L., Balescu, S., Falguères, C., Field, M., Hallégouët, B., and Keen, D. H.: Middle Pleistocene raised beach anomalies in the English Channel: regional and global stratigraphic implications, J. Geodyn., 29, 15–41, https://doi.org/10.1016/S0264-3707(99)00063-0, 2000.
Van Vliet-Lanoë, B., Cliquet, D., Auguste, P., Folz, E., Keen, D., Schwenninger, J.-L., Mercier, N., Alix, P., Roupin, Y., Meurisse, M., and Seignac, H.: L'abri sous-roche du Rozel (France, Manche): un habitat de la phase récente du Paléolithique moyen dans son contexte géomorphologique, Quaternaire, 17, 207–258, https://doi.org/10.4000/quaternaire.826, 2006.
Vandenberghe, J.: Paleoenvironment and stratigraphy during the Last Glacial in the Belgian-Dutch border region, Quaternary Res., 24, 23–38, 1985.
Vink, A., Steffen, H., Reinhardt, L., and Kaufmann, G.: Holocene relative sea-level change, isostatic subsidence and the radial viscosity structure of the mantle of northwest Europe (Belgium, the Netherlands, Germany, southern North Sea), Quaternary Sci. Rev., 26, 3249–3275, 2007.
Vis, G. J., Cohen, K. M., Westerhoff, W. E., Veen, J. H. T., Hijma, M. P., van der Spek, A. J., and Vos, P. C.: Paleogeography, in: Handbook of sea-level research, edited by: Shennan, I., Long, A. J., and Horton, B. P., John Wiley & Sons, Ltd, Chichester, UK, 514–535, https://doi.org/10.1002/9781118452547.ch33, 2015.
Wallinga, J., Törnqvist, T. E., Busschers, F. S., and Weerts, H. J.: Allogenic forcing of the late Quaternary Rhine–Meuse fluvial record: the interplay of sea-level change, climate change and crustal movements, Basin Res., 16, 535–547, https://doi.org/10.1111/j.1365-2117.2003.00248.x, 2004.
Wesselingh, F., Visser, P., and Meijer, T.: Het Eemien in zuidelijk Flevoland: een blik in de bodem van het bekken van Amersfoort, Afzettingen WTKG, 31, https://natuurtijdschriften.nl/pub/567575 (last access: 19 June 2022), 2010.
West, R. G.: Pleistocene Geology and Biology, Longman, ISBN 9780582446205, 1977.
West, R. G. and Godwin, H.: Interglacial deposits at Bobbitshole, Ipswich, Philos. T. Roy. Soc. B, 241, 1–31, https://doi.org/10.1098/rstb.1957.0006, 1957.
West, R. G. and Sparks, B. W.: Coastal interglacial deposits of the English Channel, Philos. T. Roy Soc. B, 243, 95–133, 1960.
West, R. G., Andrew, R., Catt, J. A., Hart, C. P., Hollin, J. T., Knudsen, K. L., Miller, G., Penney, D. N., Pettit, M., Preece, R. C., Switsur, V. R., Whiteman, C. A., and Zhou, L. P.: Late and Middle Pleistocene deposits at Somersham, Cambridgeshire, U.K.: a model for reconstructing fluvial/estuarine depositional environments, Quaternary Sci. Rev., 18, 1247–1314, 1999.
Westaway, R., Maddy, D., and Bridgland, D.: Flow in the lower continental crust as a mechanism for the Quaternary uplift of south-east England: constraints from the Thames terrace record, Quaternary Sci. Rev., 21, 559–603, 2002.
Westaway, R., Bridgland, D., and White, M.: The Quaternary uplift history of central southern England: evidence from the terraces of the Solent River system and nearby raised beaches, Quaternary Sci. Rev., 25, 2212–2250, 2006.
Weston, C. H.: On the sub-escarpments of the Ridgeway Range and their contemporary deposits in the Isle of Portland, Quarterly J. Geol. Soc. London, 8, 110–120, 1852.
Whitaker, W., Skertchly, S. B. J., and Jukes-Browne, A. J.: The geology of south-western Norfolk and of northern Cambridgeshire, (Explanation of sheet 65), H.M. Stationary Off, London, https://pubs.bgs.ac.uk/publications.html?pubID=B01415 (last access: 19 June 2022), 1893.
Winn, K. and Erlenkeuser, H.: Das Eem Meer in Norddeutschland: Die Kernbohrungen bei Dagebüll–Erstergebnisse, Meyniana, 47, 101–113, https://doi.org/10.2312/meyniana.1995.47.101, 1995.
Winn, K. and Erlenkeuser, H. (Eds.): Das Eem-Meer in Norddeutschland und angrenzenden Gebieten: Aufbau einer stratigraphischen Feingliederung und Untersuchung der palaeohydrographischen Entwicklung nach isotopischen, sedimentologischen, geochemischen und faunistischen Kriterien Abschlussbericht zum DFG-Forschungsvorhaben Wi 1322/1-1,-2, Christian-Albrechts-Universitaet, Kiel, Germany, http://hdl.handle.net/10013/epic.46007.d001, 1998.
Winn, K., Glos, R., Averdieck, F.-R., and Erlenkeuser, H.: On the age of the marine Eem in northwestern Germany, Geologos, 5, 41–56, 2000.
Woodroffe, S. A. and Barlow, N. L.: Reference water level and tidal datum, in: Handbook of sea-level research, edited by: Shennan, I., Long, A. J., and Horton, B. P., John Wiley & Sons, Ltd, Chichester, UK, 171–180, https://doi.org/10.1002/9781118452547.ch11, 2015.
Woodward, H. B., Blake, J. H., Bristow, H. W., Rutley, F., and Ussher, W. A. E.: Geology of the east Somerset and the Bristol Coalfields, Memoirs of the Geological Survey, London, https://pubs.bgs.ac.uk/publications.html?pubID=B01840 (last access: 19 June 2022), 1876.
Yokoyama, Y. and Purcell, A.: On the geophysical processes impacting palaeo-sea-level observations, Geosci. Lett., 8, 13, https://doi.org/10.1186/s40562-021-00184-w, 2021.
Zagwijn, W. H.: Vegetation, climate and radiocarbon datings in the late Pleistocene of the Netherlands: I. Eemian and early Weichselian, Mededelingen van de Geologische Stichting Nieuwe Serie 14, 15–45, 1961.
Zagwijn, W. H.: Sea-level changes in the Netherlands during the Eemian, Geol. Mijnbouw, 62, 437–450, 1983.
Zagwijn, W. H.: An analysis of Eemian climate in western and central Europe, Quaternary Sci. Rev., 15, 451–469, https://doi.org/10.1016/0277-3791(96)00011-X, 1996.
Zecchin, M., Nalin, R., and Roda, C.: Raised Pleistocene marine terraces of the Crotone peninsula (Calabria, southern Italy): facies analysis and organization of their deposits, Sediment. Geol., 172, 165–185, 2004.
Zeuner, F. E.: Cervus elaphus jerseyensis and other fauna in the 25 ft beach of Belle Hougue Cave, Bulletin de la Société Jerseiaise, 14, 238–254, 1946.
Zong, Y. and Horton, B. P.: Diatom zones across intertidal flats and coastal saltmarshes in Britain, Diatom Res., 13, 375–394, 1998.
- Abstract
- Introduction
- Sea-level indicators and chronostratigraphy
- Elevation measurements and corrections
- Overview of data points
- Data availability
- Closing remarks: challenges and recommendations
- Appendix A: An overview of NW European research history on LIG coastal landscapes
- Appendix B: Regional chronostratigraphical database challenges
- Author contributions
- Competing interests
- Disclaimer
- Special issue statement
- Acknowledgements
- Financial support
- Review statement
- References
- Abstract
- Introduction
- Sea-level indicators and chronostratigraphy
- Elevation measurements and corrections
- Overview of data points
- Data availability
- Closing remarks: challenges and recommendations
- Appendix A: An overview of NW European research history on LIG coastal landscapes
- Appendix B: Regional chronostratigraphical database challenges
- Author contributions
- Competing interests
- Disclaimer
- Special issue statement
- Acknowledgements
- Financial support
- Review statement
- References