the Creative Commons Attribution 4.0 License.
the Creative Commons Attribution 4.0 License.
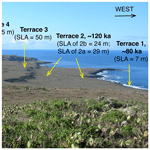
MIS 5e sea-level history along the Pacific coast of North America
Daniel R. Muhs
The primary last interglacial, marine isotope substage (MIS) 5e records on the Pacific coast of North America, from Washington (USA) to Baja California Sur (Mexico), are found in the deposits of erosional marine terraces. Warmer coasts along the southern Golfo de California host both erosional marine terraces and constructional coral reef terraces. Because the northern part of the region is tectonically active, MIS 5e terrace elevations vary considerably, from a few meters above sea level to as much as 70 m above sea level. The primary paleo-sea-level indicator is the shoreline angle, the junction of the wave-cut platform with the former sea cliff, which forms very close to mean sea level. Most areas on the Pacific coast of North America have experienced uplift since MIS 5e time, but the rate of uplift varies substantially as a function of tectonic setting. Chronology in most places is based on uranium-series ages of the solitary coral Balanophyllia elegans (erosional terraces) or the colonial corals Porites and Pocillopora (constructional reefs). In areas lacking corals, correlation to MIS 5e often can be accomplished using amino acid ratios of fossil mollusks, compared to similar ratios in mollusks that also host dated corals. Uranium-series (U-series) analyses of corals that have experienced largely closed-system histories range from ∼124 to ∼118 ka, in good agreement with ages from MIS 5e reef terraces elsewhere in the world. There is no geomorphic, stratigraphic, or geochronological evidence for more than one high-sea stand during MIS 5e on the Pacific coast of North America. However, in areas of low uplift rate, the outer parts of MIS 5e terraces apparently were re-occupied by the high-sea stand at ∼100 ka (MIS 5c), evident from mixes of coral ages and mixes of molluscan faunas with differing thermal aspects. This sequence of events took place because glacial isostatic adjustment processes acting on North America resulted in regional high-sea stands at ∼100 and ∼80 ka that were higher than is the case in far-field regions, distant from large continental ice sheets. During MIS 5e time, sea surface temperatures (SSTs) off the Pacific coast of North America were higher than is the case at present, evident from extralimital southern species of mollusks found in dated deposits. Apparently, no wholesale shifts in faunal provinces took place, but in MIS 5e time, some species of bivalves and gastropods lived hundreds of kilometers north of their present northern limits, in good agreement with SST estimates derived from foraminiferal records and alkenone-based reconstructions in deep-sea cores. Because many areas of the Pacific coast of North America have been active tectonically for much or all of the Quaternary, many earlier interglacial periods are recorded as uplifted, higher-elevation terraces. In addition, from southern Oregon to northern Baja California, there are U-series-dated corals from marine terraces that formed at ∼80 ka, during MIS 5a. In contrast to MIS 5e, these terrace deposits host molluscan faunas that contain extralimital northern species, indicating cooler SST at the end of MIS 5. Here I present a review and standardized database of MIS 5e sea-level indicators along the Pacific coast of North America and the corresponding dated samples. The database is available in Muhs et al. (2021b; https://doi.org/10.5281/zenodo.5903285).
- Article
(42114 KB) - Full-text XML
-
Supplement
(109 KB) - BibTeX
- EndNote
Because of the prospect of future sea-level rise, there has been an increasing interest in past but geologically recent times of higher than present sea level. One of the best studied of these is the last interglacial (LIG), recognized in terrestrial geologic records as the Sangamon (North America) or Eemian (Europe) periods. Within the deep-sea sediment core record, Arrhenius (1952) initiated the widely accepted practice of numbering Quaternary interglacial and glacial stages, which was encouraged with the pioneering work on oxygen isotopes in such cores by Emiliani (1955). Interglacial periods have odd numbers and glacial periods have even numbers. Thus, the last interglacial (sensu lato) in deep-sea cores is known as marine isotope stage (MIS) 5. Shackleton (1969) recognized five major substages of the MIS 5 complex (5e, 5d, 5c, 5b, 5a, from oldest to youngest), and those substages are now widely recognized by marine stratigraphers and paleoclimatologists. Another nomenclature suggested by Martinson et al. (1987) is followed by some investigators, with the peaks of these substages referred to as “events” MIS 5.5, 5.4, 5.3, 5.2, and 5.1, from oldest to youngest. MIS 5e or 5.5 is considered to be the period of peak global warmth and minimal global ice of the late Quaternary (see review in Murray-Wallace and Woodroffe, 2014). Some investigators also consider that MIS 5e alone is the last interglacial (sensu stricto).
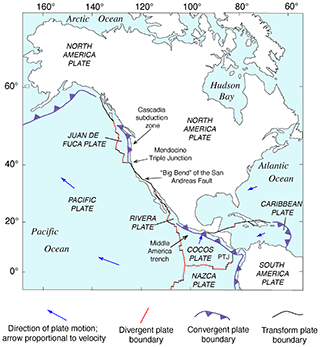
Figure 1Tectonic setting of North America showing lithospheric plates, plate boundaries, and features referred to in the text. Redrawn in simplified form from Simkin et al. (2006). PTJ: Panama Triple Junction.
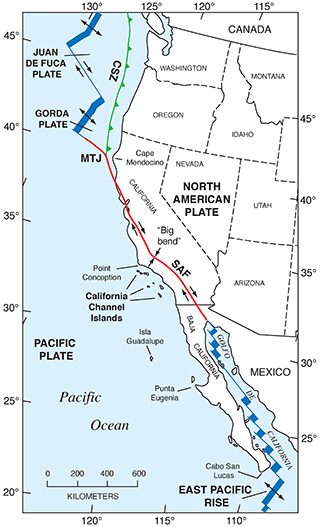
Figure 2Tectonic setting of the Pacific coast of North America, from southern Canada to southern Baja California Sur, Mexico, showing plates, plate boundaries, structures, and localities referred to in the text. Redrawn in simplified form from Drummond (1981) and Simkin et al. (2006). CSZ: Cascadia Subduction Zone; MTJ: Mendocino Triple Junction; SAF: San Andreas Fault.
The Pacific coast of North America contains a rich record of Quaternary sea-level history, particularly the peak of the LIG, MIS 5e, generally considered to date from ∼130 to ∼115 ka. Part of the richness of this sea-level record is due to the tectonic setting of North America (Fig. 1). Most of the continent is situated on the North America lithospheric plate. However, Baja California and part of westernmost California are both located on the Pacific plate, and the southern part of Central America is on the Caribbean plate. The boundaries between the two major (Pacific and North America) and smaller lithospheric plates are the tectonic controls on the crustal blocks that form the Pacific coast of North America. In southwestern Canada and the northwestern USA, the Cascadia Subduction Zone occurs where the southeast-moving Juan de Fuca and Gorda plates meet the North America plate (Fig. 2). Farther south, from northern California to the Golfo de California, the dominant structural control is the San Andreas Fault, a major right-lateral (dextral) strike-slip system, with many smaller, subparallel faults associated with it. Still farther south, at the head of the Golfo de California, the structural style changes again, with the boundary between the Pacific and North America plates taking the form of a spreading center, the northernmost part of the East Pacific Rise (Fig. 2). Finally, the structural style changes to the south once more, back to a subduction zone, in southern Mexico and Central America. Here the Cocos plate is being subducted under the North America plate (in the northern part) and under the Caribbean plate in the southern part (Fig. 1).
The importance of tectonic setting for studies of past shorelines, such as that of MIS 5e, is due to its influence on vertical movement of coastal crustal blocks. In collisional zones, such as the Cascadia Subduction Zone, it could be expected that some vertical movement might be found in the crust of the overriding plate. Indeed, a classical study by Uyeda and Kanamori (1979) proposed that where the dip of the subducting plate is shallow, rapid uplift should be seen in the overriding plate. However, detailed studies of marine terraces in northern California and Oregon by Harvey M. Kelsey and his colleagues (Kelsey, 1990; McInelly and Kelsey, 1990; Kelsey and Bockheim, 1994; Kelsey et al., 1994, 1996; Polenz and Kelsey, 1999) have shown convincingly that it is actually local structures (faults and folds) within the upper plate that control the rates of marine terrace uplift seen along much of the Cascadia Subduction Zone. Farther south, within the San Andreas Fault zone, rates of uplift are highly variable (see summary in Muhs et al., 2014c). Along much of the coast bordering this fault zone, uplift rates are modest, likely (though not yet proven) because movements along faults that have a predominantly strike-slip (horizontal) sense of movement have a small vertical component. Exceptions to this occur where there are restraining bends in these faults, the most famous of which is the “big bend” area of the San Andreas Fault zone (Fig. 2). Here, crustal compression results in extremely high rates of uplift. Away from the zone of maximum uplift south of the big bend in the San Andreas Fault, Shaw and Suppe (1994) proposed that uplift of the Santa Cruz Island and Anacapa Island shelf area of southern California is due to movement on an underlying, blind thrust fault. High rates of uplift can also be found on coastlines adjacent to triple junctions, such as the Mendocino Triple Junction (Fig. 2) and the Panama Triple Junction (“PTJ” in Fig. 1). Along coastlines bordering a spreading-center plate boundary, such as that in the Golfo de California, crustal blocks are moving away from each other, and accommodation space is increasing, so uplift rates are not expected to be particularly high. This simplified picture is to a great extent borne out by field studies (e.g., Ortlieb, 1991), although local structures can again play a role in generating uplift over limited parts of such a coastline. Uplift in Central America is rapid in places due to subduction of seamounts on the Cocos and Nazca plates.
Marine terraces along the Pacific coast of North America have been studied for more than a century. Lawson (1893) considered that emergent terraces formed by episodic (and presumably rapid) uplift, what would now be referred to as coseismic uplift. Smith (1900), studying terraces on the California islands, concurred with this hypothesis, reasoning that episodic uplift must alternate with periods of “comparative quiescence”. Interestingly, the concept of episodic rapid uplift is now known to have validity for some parts of the Pacific coast, in diverse tectonic settings (see discussion below on Holocene shorelines).
Grant and Gale (1931) also considered emergent terraces to have a tectonic origin but also pointed out the possibility of a eustatic component. It was Davis (1933), however, studying marine terraces in the Malibu, California, region, who was likely the first to point out explicitly that although uplift was obviously a factor in the formation of a flight of marine terraces, a eustatic component was important as well. Davis (1933) considered that uplift rates were likely to have geographic variability, but he noted that eustatic records ought to be the same everywhere. Despite the publication of this important paper, there was a return to the idea of terraces being dominantly of a tectonic origin in later studies by Putnam (1942), Woodring et al. (1946), and Upson (1951). Woodring et al. (1946) thought that eustatic effects were either obliterated or obscured in the geologic record of marine terraces. Although Upson (1951) considered that terraces were formed principally by episodic uplift, he recognized that there were problems with this explanation and thought that a eustatic component was present.
Interestingly, it was a master's degree thesis at the University of California at Berkeley that articulated our current concepts of marine terraces, uplift, and sea level clearly for the first time. Alexander (1953), working on both marine and stream terraces in the Capitola–Watsonville area of central California, measured the maximum elevations of marine terraces and the tops of stream-fill terraces, noting their similar elevations and reasoning that they must have a common, eustatic control. He also noted that in between times of stream terrace formation, there were episodes of valley cutting, which indicated periods of eustatically lowered sea level, during glacial periods. On the other hand, multiple marine terraces indicated long-term tectonic uplift. He (Alexander, 1953, p. 36) concluded that “Thus, the marine terraces of the Capitola–Watsonville area are regarded as having originated under conditions of a slowly and continuously rising coast against which occurred at least three complete cycles of eustatic changes in sea level”. This is a remarkable conclusion, reached before any modern methods of geochronology were in common use and based only on sound field mapping, elevation measurements, and geomorphic reasoning. It was this concept, along with uranium-series geochronology, that allowed Broecker et al. (1968), Mesolella et al. (1969), and Veeh and Chappell (1970) to infer that coral reef terraces on the uplifting coasts of Barbados and New Guinea recorded interglacial periods that supported the Milankovitch or orbital theory of climate change. In California, Alexander's (1953) concept was accepted explicitly or implicitly by subsequent workers in the following decades (e.g., Vedder and Norris, 1963; Birkeland, 1972; Bradley and Griggs, 1976; Wehmiller et al., 1977b), and his contribution is now recognized in one of the leading textbooks on geomorphology (Anderson and Anderson, 2010).
Dating of marine terraces on the Pacific coast of North America had a development similar to that for other coastlines. Early use of uranium-series (U-series) analyses of corals was reported by Veeh and Valentine (1967), Valentine and Veeh (1969), and Ku and Kern (1974). In these investigations and most subsequent studies, the taxon analyzed is the solitary coral Balanophyllia elegans (Gerrodette, 1979), which is by far the most common coral found in Oregon and California marine terrace deposits. These early studies permitted an interpretation that low-elevation terraces at Cayucos, San Nicolas Island, and Point Loma could all date to MIS 5e. Other studies attempted U-series analyses of fossil mollusks (e.g., Bradley and Addicott, 1968; Szabo and Rosholt, 1969; Szabo and Vedder, 1971), but a seminal study by Kaufman et al. (1971), with extensive data from California terraces, showed that mollusks are inappropriate materials for U-series geochronology.
A new development in geochronology, however, brought mollusks back to the forefront in dating marine terraces on the Pacific coast. Using the Cayucos, San Nicolas Island, and Point Loma U-series coral ages as calibration points, Wehmiller et al. (1977b), Wehmiller (1982), and Kennedy et al. (1982) showed that terraces from Baja California Sur to Oregon could be correlated to MIS 5e on the basis of amino acid ratios in fossil mollusks, a profound finding that demonstrated the extensive nature of the last interglacial record on the Pacific coast of North America. In addition, these studies also showed that uplift rates on the Pacific coast are variable, overturning a long-held concept that the lowest marine terrace is everywhere of the same age. Indeed, terraces estimated to be as young as ∼50 ka were found in areas of high uplift rate.
Concerted efforts to find corals yielded more U-series ages of marine terraces. Rockwell et al. (1989) mapped 14 terraces on Punta Banda, Baja California, the lowest 3 of which have shoreline angle elevations of 15–17, 22, and 27–43 m. The first or “Lighthouse” terrace has U-series ages (by alpha spectrometry) of corals and hydrocorals of ∼80 ka, the second terrace is undated, and the third or “Sea Cave” terrace has ages of ∼120 ka. This was the first study on the Pacific coast to provide definitive geochronologic evidence of both MIS 5e and MIS 5a (as well as a likely MIS 5c at ∼22 m) terraces. Muhs et al. (1990, 1992, 1994) reported additional U-series ages, again by alpha spectrometry, for MIS 5e terraces at Cayucos, Point San Luis, San Nicolas Island, San Clemente Island, and Point Loma (all in California), as well as Punta Banda, Isla Guadalupe, and Cabo Pulmo (in Baja California and Baja California Sur). Terraces dating to MIS 5a were reported from Coquille Point, near Bandon, Oregon; Point Arena, San Nicolas Island, and Point Loma (all in California); and Punta Banda (Baja California).
The development of U-series dating of corals by thermal ionization mass spectrometry (TIMS) led to a new level of complexity in the understanding of the Pacific coast marine terrace record. Stein et al. (1991) redated corals from the Cayucos and Point Loma areas, confirming that fossils dating to MIS 5e were present but also showing the possibility that some corals dated to MIS 5c (∼100 ka). Muhs et al. (2002a) confirmed these results for both Cayucos and Point Loma. Kennedy et al. (1982), in their amino acid study along the Pacific coast, reported that terraces correlated to MIS 5a had molluscan faunas with cool-water aspects, whereas those correlated to MIS 5e hosted molluscan faunas with warm-water aspects. Cool-water faunas were confirmed with corals dated to ∼80 ka using TIMS by Muhs et al. (2006) in a later study, in partial support of Kennedy et al. (1982). However, Muhs et al. (2002a) showed that the terraces at Cayucos and Point Loma, containing both MIS 5e and MIS 5c corals, hosted molluscan faunas with a mix of both warm-water taxa (thought to date from MIS 5e) and cool-water taxa (thought to date from MIS 5c). This idea was explored in more detail on San Nicolas Island, where the lowest three terraces (1, 2b, and 2a, in ascending elevation order) were mapped in detail, terrace elevations were measured precisely with differential GPS methods, corals from all three terraces were dated with TIMS, and the faunas were characterized (Muhs et al., 2012). Terrace 1 dates to ∼80 ka and hosts a cool-water fauna, terrace 2b has both 100 and 120 ka corals and hosts a mix of cool-water and warm-water taxa, and terrace 2a has only ∼120 ka corals, no cool-water taxa, but several warm-water taxa. This finding raised the possibility that the MIS 5c high-sea stand in this region had a paleo-sea-level elevation higher than what would have been inferred from the classic records on Barbados and New Guinea and that this high stand overtook at least the outer part of the MIS 5e terrace, reworking and mixing its fossils (with warm-water taxa) with shells dating to MIS 5c (with cool-water taxa). Subsequent studies have shown that other terraces dating to MIS 5e (by TIMS U-series on corals) or correlated to MIS 5e (by amino acids on mollusks) also contain mixes of warm-water and cool-water taxa (Muhs et al., 2014a, c; Muhs and Groves, 2018).
The main aim of this paper is to serve as a description to accompany a standardized database of MIS 5e sea-level indicators compiled following the WALIS template (Rovere et al., 2020). From the published papers in the area of interest, I extracted sea-level indicators and standardized the quantification of their elevation and indicative meaning (Shennan, 1982; Shennan et al., 2015; Rovere et al., 2016), along with appropriate metadata. Each sea-level indicator was then associated with one or more samples, which were dated with U-series or amino acid racemization (AAR) methods and were also added to the database. In some cases, U-series-dated samples were already present in the WALIS database from the compilation of Chutcharavan and Dutton (2021).
Table 1Different types of RSL indicators: reference water level (RWL) and indicative range (IR) on the Pacific coast of North America.
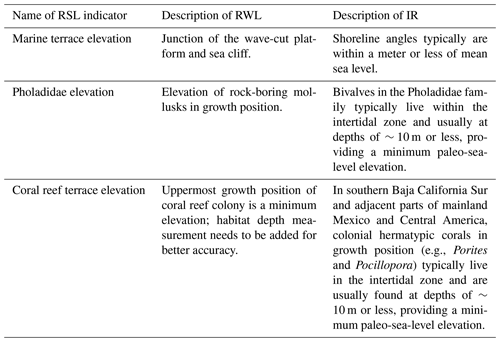
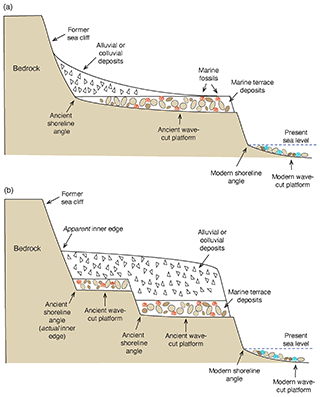
Figure 3Diagrams showing the terminology used for marine terraces: (a) simple case of a modern wave-cut bench or platform in the surf zone with marine gravels and modern shells (blue symbols), shoreline angle, and single emergent marine terrace above it, with a colluvial cover masking most of the marine terrace deposits with their fossils (red symbols). Such an emergent terrace could have formed from a higher-than-present sea level or from uplift since the time of initial terrace formation; (b) more complex case on an uplifting coast with the features described above but an additional (older) terrace above the lower one. Note that in (b), colluvial deposits cover both of the emergent terraces, making them appear as one landform, with a single inner edge that is at a higher elevation than the shoreline angles of both emergent terraces.
As pointed out by Rovere et al. (2016), critical to reconstructing past sea level during MIS 5e (or any past high-sea stand, for that matter) is an accurate assessment of paleo-sea-level indicators (Table 1). For the vast majority of MIS 5e geomorphic records along the Pacific coast of North America, the best relative sea-level (RSL) indicator is what is called the shoreline angle, a term that goes back to the classic study of terraces in the Malibu, California, area by Davis (1933). The shoreline angle is the junction of the marine platform (or “wave cut bench”), formed in the surf zone and the sea cliff, when viewed in cross section (Fig. 3a). Davis (1933) and virtually all investigators who have followed him have generally regarded the shoreline angle as the best overall RSL because it is considered to form at or near sea level. Kelsey (2015) points out that depending on bedrock type, structures within the local bedrock, orientation of the coast with respect to wave exposure, and other factors, shore-parallel variability in the shoreline angle elevation on modern coastlines can range by as much as 1–4 m. In the San Diego area, however, measurements made by Kern (1977) indicate that modern shoreline angles typically form within a meter of modern sea level. Whether shoreline angles on the Pacific coast form closest to mean sea level or high-tide level is probably not known with any certainty. In any case, however, the range of variability in shoreline angle elevations noted by Kelsey (2015) is typically greater than the mean tidal range. In southern and central California, from San Diego to San Francisco Bay, mean tidal range is typically only 1.1 to 1.2 m; in northern California, it increases to about 1.2 to 1.5 m; and in Oregon, it is 1.6 to 1.8 m (data from https://tidesandcurrents.noaa.gov/tide_predictions.html, last access: 4 February 2021). In most places that I have studied along the Pacific coast, marine platforms or wave-cut benches are typically only visible at low tide (Fig. 4) and are not visible at high tide. In most of California, therefore, with a mean tidal range (low to high tide) of only about a meter, these observations suggest that Kern's (1977) observations have general validity, and shoreline angles approximate mean sea level.
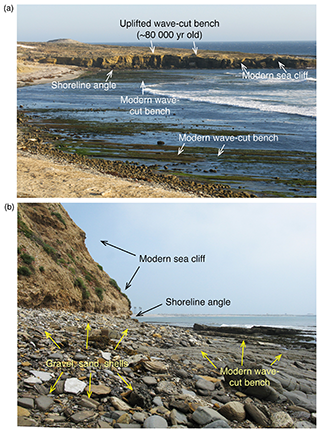
Figure 4(a) Modern wave-cut bench exposed at low tide, shoreline angle, sea cliff, and wave-cut bench of emergent, ∼80 ka marine terrace, Cormorant Rock, San Nicolas Island, California, USA. (b) Modern wave-cut bench exposed at low tide, overlying marine gravels, shoreline angle, and sea cliff, San Pedro, California, USA. Photographs by Daniel R. Muhs.
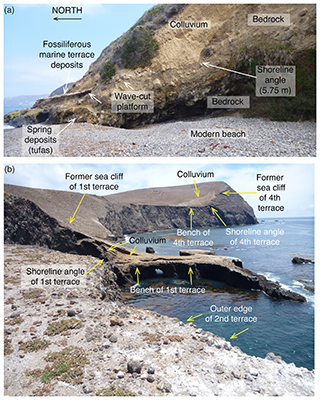
Figure 5Examples of exposures of ancient shoreline angles: (a) north coast of Santa Cruz Island, California, just east of Prisoners Harbor; (b) west side of Santa Barbara Island, California, showing benches and shoreline angles of three of the four lowest marine terraces. Photographs by Daniel R. Muhs.
For the field geomorphologist studying marine terraces, a much greater challenge lies in mapping shorelines accurately and finding good exposures of ancient shoreline angles. After terrace emergence, the wave-cut platform and the marine sediments covering it become the locus of deposition of terrestrial deposits, including alluvium, colluvium, and eolian sand (Fig. 5). Such deposits obscure the precise location of the inner edge of a marine terrace. The term “inner edge” is often used interchangeably with the term shoreline angle, but here it is meant to express the spatial extent of a shoreline, i.e., viewed planimetrically, in a shore-parallel sense. Put another way, it is the mapped expression of where the shoreline angle is situated, marking the former junction of land and sea. Terrestrial deposits that cover inner edges of marine terraces not only make mapping of a given terrace difficult, but they can also be extensive enough that they cover two or more discrete terraces. Alluvial and eolian deposition can sometimes generate a rather smooth surface that gives the impression of being an actual marine platform surface, which may in reality be many meters below (Fig. 3b). In the example shown in Fig. 3b, the unwary researcher might assume that there is only one terrace here and also could easily assume that the “apparent inner edge” is where the actual shoreline angle is situated, when in fact it is seaward of this and at a much lower elevation.
Even where shoreline angles are well exposed, an additional complication can arise, particularly in areas where uplift rates are low or no uplift at all is occurring. In such areas, successions of sea-level high stands that have similar paleo-sea levels may reoccupy a terrace. Examples of this with reoccupation of the MIS 5e terrace by the MIS 5c high-sea stand were noted earlier. Where this has occurred, it may sometimes be difficult or impossible to estimate paleo-sea level during MIS 5e time, even when a shoreline angle is well exposed as it would be unclear which high-sea stand produced that feature.
In the database, the upper and lower limits of the indicative range for a shoreline angle were set as the mean lower low water (MLLW) and the mean higher high water (MHHW) reported for the nearest NOAA tide station. In studies reviewed here, whenever the shoreline angle elevation was not reported by the original authors, the dated sample elevation was used, and readers are cautioned that such elevations are therefore minimum-limiting for an estimate of paleo-sea level.
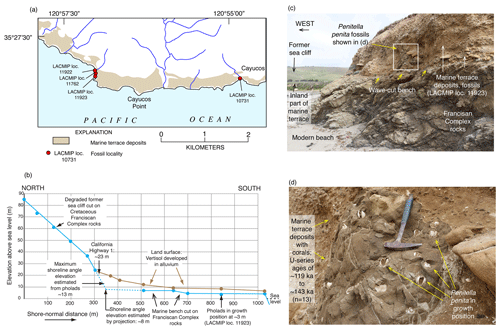
Figure 6Two methods of estimating paleo-sea level when shoreline angles are not exposed at Cayucos, California. (a) Map showing extent of marine terrace dated to ∼120 ka and fossil localities from Muhs et al. (2002a). (b) Topographic profile of a shore-normal transect in the vicinity of fossil localities LACMIP (Natural History Museum of Los Angeles County Invertebrate Paleontology) 11923, 11762, and 11922, showing measured bench elevations and paleo-sea cliff elevations, where they are exposed; intersection of extrapolated wave-cut bench slope landward and paleo-sea cliff slope downward yields an estimated shoreline angle elevation of ∼8 m. (c) Photograph of outer edge of terrace at fossil locality LACMIP 11923, showing wave-cut bench 3 m above sea level with Penitella penita fossils (rock-boring bivalves) in growth position (see enlargement in d). P. penita lives in waters 10 m deep or shallower, so bench elevation (3 m) plus maximum depth of growth (10 m) yields a maximum-limiting paleo-sea level of ∼13 m above present. Photographs by Daniel R. Muhs.
Because most Pacific coast marine terraces develop on a high-energy, erosive coastline, biological indicators of RSL are rare. Typically, marine fossils are found in a poorly sorted mix of sand and gravel. As a consequence, the fossils in marine terrace deposits, even those near the former shoreline, have been transported there by waves, sometimes from depths of 20 m or more. Exceptions to this, while uncommon, do occur and most often take the form of rock-boring mollusks in growth position, particularly bivalves in the Pholadidae family. A good example of this is the species Penitella penita. This taxon typically occurs in the mid-intertidal zone, based on modern specimen collections in the Santa Barbara Museum of Natural History (Paul Valentich-Scott, personal communication, March 2020). Only rarely is P. penita found below depths of ∼10 m (Coan et al., 2000). Thus, if fossil P. penita is found in growth position in bored holes of a wave-cut bench (Fig. 6), it is likely that one is within 10 m of paleo-sea level. While this criterion is not as specific an RSL indicator as a shoreline angle, it is often a complementary tool for paleo-sea level. Other species of bivalves can potentially serve as paleo-sea-level indicators if they are articulated, a characteristic not possible with gastropods. For example, the large bivalve Saxidomus nuttalli, which is presently found from northern California to Baja California Sur, typically lives in muddy sediments within the intertidal zone to ∼10 m depth (Coan et al., 2000), a range similar to Penitella penita. Thus, if an articulated fossil specimen of S. nuttalli is found, it is possible that it is close to where it was situated when it was living because wave transport commonly will disarticulate shells. However, S. nuttalli is not a rock-boring mollusk, so without occurrence in a hole that it has bored, one can never be certain, even with articulated shells, that one is near the position where the specimen lived.
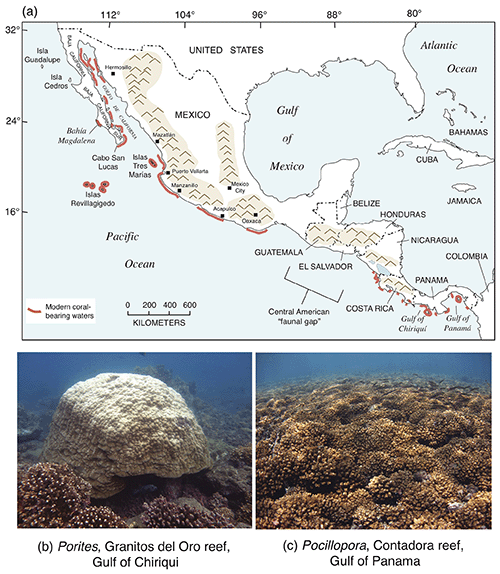
Figure 7(a) Map showing the distribution of living hermatypic corals and coral reefs along the Golfo de California coasts of Mexico, the Pacific coast of Mexico, and the Pacific coast of Central America (compiled from Reyes-Bonilla and López-Pérez, 2009; Alvarado et al., 2010; Glynn et al., 2017). (b, c) Examples of modern hermatypic corals along the Pacific coast of Central America (photographs courtesy of Lauren Toth, U.S. Geological Survey).
Farther south, along both shores of the Golfo de California and the Pacific coast of mainland Mexico and Central America, ocean water temperatures are higher than farther north, and hermatypic (reef-building) corals are found (Fig. 7). Although hermatypic corals can be found throughout much of this region, true coral reefs are far less common. For example, within the Golfo de California, although corals can be found along almost all of the Baja California coast and much of the Sonoran coast, true coral reefs have been documented only at a few localities. The region from the upper Golfo de California to Panama does, however, host a surprising diversity of coral species (Reyes-Bonilla and Lópéz-Pérez, 1998; Glynn and Ault, 2000; Glynn et al., 2017; Toth et al., 2017). Some of the most important genera are Porites (seven species), Pocillopora (six species), Psammocora (four species), and Pavona (five species). Porites panamensis (formerly P. californica in some studies) is found from the upper Golfo south to Panama but also has a disjunct distribution, with colonies of this taxon also found in Bahía Magdalena on the Pacific coast of Baja California Sur (Squires, 1959). According to Glynn and Ault (2000), maximum shelf depths where coral colonies or reefs have been observed, from the Golfo de California to Panama, are ∼10 m or less. This important observation provides a third relative sea-level indicator; where fossil hermatypic corals are found in growth position, sea level was likely no higher than ∼10 m above that elevation.
3.1 Elevation measurements
Virtually all of the studies cited herein provide measurements of the elevations of the RSL indicators. In most studies that were conducted before approximately 2010, measurements were typically made using contours on topographic maps, hand level and/or meter tape, transit and stadia rod, or barometric altimeter. For these studies, unless uncertainties are reported in the paper (or where the shoreline angle elevation range is given), elevation uncertainties are assumed to be 20 % of the original elevation. This procedure assumes that higher-elevation shoreline angles will have greater uncertainties and attribution to an appropriate sea-level datum. After approximately 2010, most studies provide elevation measurements done by either handheld or differential Global Positioning System (GPS) instruments (Table 2). Where elevation measurements were made with a handheld GPS instrument, uncertainties can be substantial, and here it is assumed that measurement errors are within ±3 m of the reported value. For measurements made with a differential GPS instrument, uncertainties are those given in the original study; if not reported, measurement errors are assumed to be within ±0.5 m.
3.2 Geochronology
All of the RSL indicators that represent MIS 5e on the Pacific coast of North America considered here have geochronological constraints based on either direct numerical dating using uranium-series (U-series) methods on corals or the correlated-age method of amino acid geochronology with ties to nearby U-series-dated (coral) localities. As a result, each RSL data point in the database is associated with one or more fossil samples dated with either U-series or amino acid geochronology. Luminescence methods have not been widely applied in this region, although the study by Grove et al. (1995) in the Tomales Bay area provides an important exception. U-series dating of mollusks was once considered a promising method for dating marine terrace fossils in California, but the study by Kaufman et al. (1971) has shown convincingly that mollusks do not take up U during growth, and frequently behave as open systems with respect to U and its daughter products. Thus, early studies that have attempted to date marine terraces by this method are not considered reliable. More recently, cosmogenic isotopes have been attempted in developing chronologies for marine terraces in California (Perg et al., 2001). This method, while promising in theory, requires careful discrimination of which sediments are sampled for analysis. In a study by Perg et al. (2001), ages derived for the terraces near Santa Cruz, California, do not agree with U-series ages on marine terrace corals from the same area (Muhs et al., 2006). The latter investigators speculated that the sediments analyzed by Perg et al. (2001) were likely taken from the terrestrial deposits overlying the marine terrace deposits, which explains the younger than expected cosmogenic ages. Finally, the unique altitudinal-spacing method of Bull (1985) has been applied to marine terraces on the Pacific coast of North America. Terraces correlated to MIS 5e using this method are not considered in the present review because Bull's (1985) method assumes that the sea-level history derived from the Huon Peninsula of New Guinea is a faithful representation of sea-level history on all coastlines around the world (this issue is reviewed in more detail below).
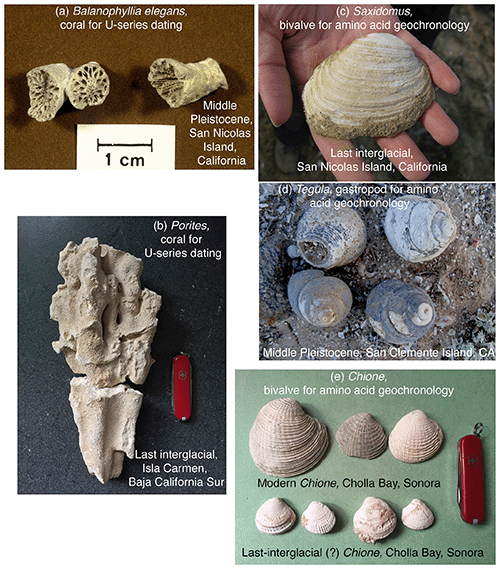
Figure 8Examples of fossil marine organisms used for geochronology of marine terrace deposits on the Pacific coast of North America: (a) solitary corals Balanophyllia elegans (fossil), San Nicolas Island, California (U-series dating); (b) Porites panamensis (fossil), Isla Carmen, Baja California Sur (U-series dating); (c) Saxidomus (fossil), San Nicolas Island, California (amino acid geochronology); (d) Tegula (fossil), San Clemente Island, California (amino acid geochronology); (e) Chione (modern: upper row; fossil: lower row), Cholla Bay, Sonora, Mexico (amino acid geochronology). All photographs by Daniel R. Muhs.
3.2.1 Uranium-series dating
Uranium-series dating is based on the fortunate characteristic of corals (Fig. 8) to take up small amounts of U (238U, 235U, 234U) from seawater into their aragonite skeletons during growth. The U assimilated by corals is in isotopic equilibrium with seawater. In contrast, Th and Pa are very insoluble elements, and therefore ocean water contains essentially no dissolved Th or Pa. Thus, 230Th and 231Pa atoms, absent in living corals, accumulate in a fossil due to decay of 234U and 235U, respectively. These two “daughter-deficient” methods utilize daughter/parent activity ratios ( and ) that begin with 0 in living corals and continue to increase in a fossil until equilibrium values of 1.0 are reached. In addition, 234U is present in seawater with an ∼14 %–16 % greater activity than 238U (i.e., the activity value in seawater is ∼1.15). In a fossil coral, the activity value decreases down to an equilibrium value of 1.0 over time, resulting in a third clock, a “daughter-excess” method.
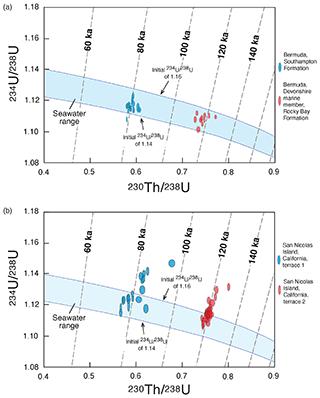
Figure 9Isotopic evolution diagrams for (a) colonial corals from the Southampton Formation (∼80 ka) and Devonshire marine member of the Rocky Bay Formation (∼120 ka) of Bermuda and (b) solitary corals from terrace 1 (∼80 ka) and terrace 2 (∼120 ka) of San Nicolas Island, California. Bermuda data are from Muhs et al. (2002b) but do not include two samples that have evidence of U loss. San Nicolas Island data are from Muhs et al. (2006). Blue bands show isotopic evolution pathways for corals having mostly closed-system history and initial activity values of 1.16 to 1.14, which bracket measured values in modern seawater (Chen et al., 1986; Delanghe et al., 2002) and modern corals (Muhs et al., 2002a).
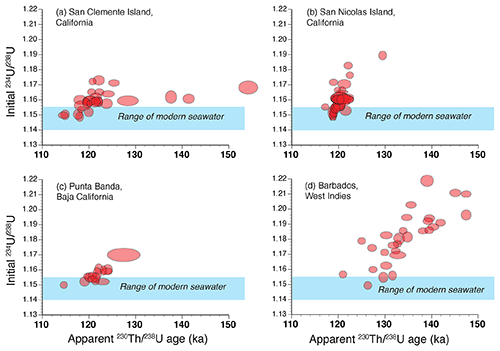
Figure 10Plots of apparent ages vs. back-calculated initial values in solitary corals from (a) Eel Point terrace, San Clemente Island, California, (b) Terrace 2, west end of San Nicolas Island, California (same as in Fig. 9b but with different scales), (c) Sea Cave terrace, Punta Banda, Baja California, Mexico, and (d) colonial corals (mostly Acropora palmata) from the Rendezvous Hill terrace, north end of Barbados, West Indies. San Clemente Island and Punta Banda data are from Muhs et al. (2002b), San Nicolas Island data are from Muhs et al. (2006), and Barbados data are from Muhs and Simmons (2017). Also shown (blue bands) is the range of activity values in modern seawater (Chen et al., 1986; Delanghe et al., 2002). Note that in both solitary corals and colonial corals, samples plotting above seawater values tend to be biased to older apparent ages, but the degree of bias varies from locality to locality.
Both solitary and colonial corals take up U from seawater during growth, usually in amounts ranging from 2 to 3 ppm, although some genera of corals (notably species of Acropora) take up U in amounts ranging from 3 to 4 ppm. Along the northern part of the Pacific coast of North America, from Oregon to Baja California, the most common species used for U-series dating is the solitary coral Balanophyllia elegans (Fig. 8). Based on studies of living and dead-collected modern specimens, B. elegans takes up some additional U after death but apparently does so from seawater while still submerged and in isotopic equilibrium with U in the ocean (Muhs et al., 2002a, 2006). Farther south, where colonial, hermatypic corals are found, species of the genera Pocillopora and Porites (Fig. 8) are the taxa most commonly used for U-series dating. In practice, the two clocks used most commonly in U-series dating are and . Because of the laboratory challenges in using a Pa spike, few laboratories measure . It is a common practice to assess ages by plotting measured values against measured values, along with the expected isotopic evolution pathways, assuming initial values in seawater. In Fig. 9a, such an array is shown for corals from Bermuda and the pathways expected from initial seawater values (for 1.140–1.155; shown here from 1.140 to 1.160; for , the initial value is 0.0). Corals that follow these expected isotopic evolution pathways yield ages that likely have minimal bias and can be considered to have had mostly closed-system histories with respect to U-series nuclides. In Fig. 9b, what is shown is a much more common situation with corals from the Pacific coast of North America, with examples from the first and second terraces on San Nicolas Island, California. While some corals indicate a closed-system history, similar to Bermuda, others plot above the closed-system evolution pathways. This indicates an open-system history with respect to U-series isotopes in these corals, likely due to recoil-derived additions of 230Th and 234U from dissolved U in water passing through the host sediment. An alternative method of assessing degree of closed-system history of fossil corals is to plot the apparent age as a function of its back-calculated initial value using the measured value and the apparent age. Examples of this approach are given in Fig. 10, where samples, if they have experienced a closed-system history, should fall within the blue-shaded bands that define the range of variability in modern seawater. As is evident from the plots shown in Fig. 10, both solitary corals from the Pacific coast of North America and colonial corals from Barbados are prone to open-system histories, but some corals show good evidence of a likely closed-system history. In the examples shown here, it would appear that those corals with closed-system histories on the Pacific coast have an age range of ∼124 to ∼114 ka.
In examining U-series data from corals of reef terraces on Barbados, Gallup et al. (1994) noted that even with open-system histories on isotope evolution plots, a roughly linear trend was observed, with corals that plotted farther above the closed-system pathway showing a bias to older apparent ages. On the Pacific coast of North America, the same kind of trend is seen as that on Barbados (see Fig. 9b), indicating that this may be a general condition in the near-surface environment where fossil corals are found despite substantial differences in climate, soil and groundwater hydrology, and composition of surrounding terrains. Nevertheless, noting this typically linear trend on Barbados, Gallup et al. (1994) suggested that extrapolation of linear trends back to a closed-system composition could yield an approximate age for a given terrace. This is also part of the basis of the open-system method of U-series age correction devised by Thompson et al. (2003).
Because of the analytical challenges in determining ages, it has become a common practice within the U-series geochronology community to assess the reliability of ages with the use of the back-calculated values and a comparison to modern seawater. Although in principle this is an appropriate cross-check, it is not completely reliable. Studies by Gallup et al. (2002), Edwards et al. (1997), and Cutler et al. (2003) on corals from Barbados and New Guinea showed that some corals that demonstrated concordant and ages did not show back-calculated values within the range of modern seawater. Conversely, some corals that did show back-calculated values within the range of modern seawater did not have concordant and ages.
Marine terrace corals dated by U-series methods are found within the WALIS database and/or within the compilation of Chutcharavan and Dutton (2021). Generalized information about each U-series-dated locality can be found in Table S1 in the Supplement.
3.2.2 Amino acid geochronology
In the absence of corals in a marine terrace deposit or emergent reef, mollusks, both bivalves and gastropods, can be used for amino acid geochronology. For marine terraces on the Pacific coast of North America, amino acid geochronology was pioneered by John F. Wehmiller and his colleagues (Wehmiller et al., 1977b; Lajoie et al., 1980; Kennedy et al., 1982; Wehmiller, 1982, 1992, 2013a, b). The method is based on the observation that living organisms contain only amino acids with the “L” (levo, or left-handed) configuration. Upon death of an organism, amino acids of the L configuration convert to amino acids of the “D” (dextro, or right-handed) configuration, a reaction called racemization. Racemization is a reversible process that results in increased D L ratios in a fossil until an equilibrium ratio of 1.0 is reached. A related process, called epimerization, is conversion of the amino acid L-isoleucine (found in living organisms) to D-alloisoleucine (not found in living organisms). Epimerization, like racemization, begins with D-alloisoleucine L-isoleucine values of 0.0 in a fossil, but this ratio increases over time until an equilibrium value of 1.25–1.30 is reached (Miller and Mangerud, 1985). Some of the fossils that have been most commonly used on the Pacific coast of North America are the bivalves Saxidomus and Chione (in protected, sandy or muddy bay environments) and Tegula (in high-energy, rocky-shore environments), shown in Fig. 8.
Amino acid values in fossil mollusks can be used for lateral correlation of marine terrace deposits, exploiting the fact that both racemization and epimerization rates increase with higher diagenetic temperature histories. This means that D L values in shells reach equilibrium values more quickly in warmer climates than they do in cooler climates. Thus, on north–south-trending coastlines in the Northern Hemisphere, such as the Pacific coast of North America, shells in terrace deposits at more southerly localities are expected to have higher D L values than shells of the same genus but of similar age in cooler, northerly localities. When D L values are arrayed on a latitudinal plot or a plot of mean annual air temperatures, there should be a south-to-north decrease in D L values in shells of the same age. In practice, some localities along such an array have independent age control from U-series dating of corals. If so, then a shore-parallel correlation of locality to locality, from south to north, can be accomplished, yielding an “aminozone” corresponding to the age of the independently dated localities. Shells from younger terraces would define an aminozone below such a zone, and older terraces would define an aminozone above it.
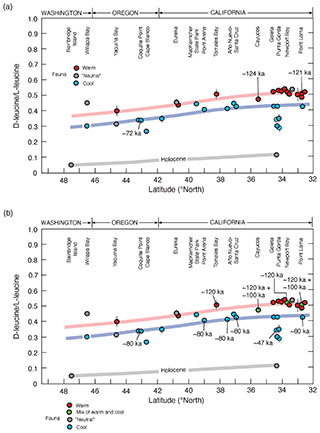
Figure 11(a) Plot showing D-leucine L-leucine in fossil Saxidomus shells (or equivalent values converted from Leukoma staminea shells; see Lajoie et al., 1980) from marine terrace deposits of the Pacific coast of the USA, from Kennedy et al. (1982). Localities are arranged from north (left) to south (right), parallel to latitudinal trend of mean annual air temperatures increasing to the south. Samples plotting along the pink line are correlated with MIS 5e (∼120 ka) based on calibration to U-series-dated corals from Cayucos and Point Loma; samples plotting along the blue line are correlated to MIS 5a (∼80 ka), based on U-series-dated corals from Coquille Point, Oregon. Calibration points used are the only ones that were available at the time of the original study. Samples plotting below these lines are correlated with MIS 3 or to Holocene-dated deposits (gray line). Not included from the original study are data points from Whidbey Island, Washington, which are interpreted to be from glaciomarine deposits (Polenz et al., 2009). Colors of circles indicate molluscan fauna thermal aspects (see discussion of Fig. 13). (b) Plot of same data as in (a), except new U-series ages of corals, generated since 1982, have been added and thermal aspects of some faunas have been modified (Muhs et al., 2002a, 2006, 2014c).
The first major attempts at aminostratigraphic correlation along the Pacific coast using the approach just described were those by Wehmiller et al. (1977b), Kennedy et al. (1982), and Wehmiller (1982). The north-to-south correlation of terraces from Kennedy et al. (1982) is shown in Fig. 11a, along with three U-series-dated localities that serve as calibration points. Kennedy et al. (1982) also noted that most localities correlated to either MIS 5a or MIS 3 hosted terrace faunas with cool-water aspects, whereas those correlated to MIS 5e had warm-water faunas, or at least faunas that were “neutral”, lacking cool- or warm-water taxa. In the time since the Kennedy et al. (1982) study was conducted, more U-series ages on coral have been reported (∼120, ∼80, and ∼47 ka), many of which support the original aminostratigraphic correlations (Fig. 11b). Nevertheless, some localities are now known to host mixes of warm and cool faunas, and at least two of these have mixes of ∼120 ka (MIS 5e) and ∼100 ka (MIS 5c) corals (Fig. 11b). This issue is discussed in more detail below.
Despite some concerns, amino acid geochronology has been shown to be a very powerful coast-parallel correlation tool. Even within the limited geographic range of central California to northern Baja California, there is enough of an air temperature gradient that aminostratigraphic correlation can be accomplished. At a given locality where two terraces are found (one at a low elevation, one at a higher elevation), MIS 5a and MIS 5e terrace deposits can usually be distinguished from one another (Fig. 12). Furthermore, lateral correlation of MIS 5e and MIS 5a deposits from central California to northern Baja California can be made, anchored by localities with U-series ages on corals.
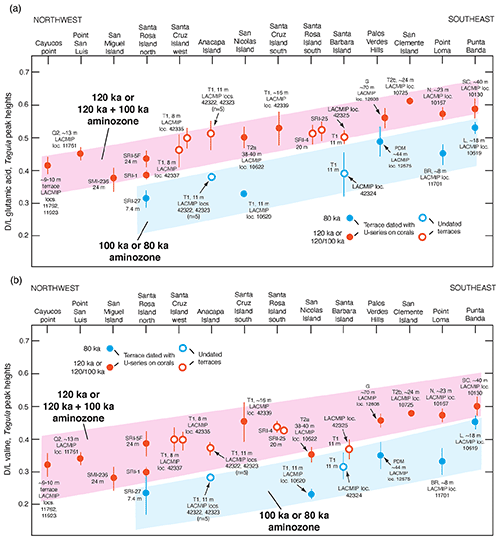
Figure 12(a) Plot of mean D L values in glutamic acid (vertical axis) in fossil Tegula from dated (filled circles) and undated (open circles) marine terraces on the California and Baja California coast, shown as a function of latitude (horizontal axis) as a proxy for long-term temperature history, which is cooler in the northwest and warmer in the southeast. Error bars are ±1 standard deviation, based on D L values in three to six individual shells from the same deposit. Colored bands (“aminozones”) indicate correlation between fossil localities of the same age, anchored by U-series dating of corals. Terrace name abbreviations: SMI: San Miguel Island, SRI: Santa Rosa Island; SCRZI: Santa Cruz Island; N: Nestor; BR: Bird Rock; PDM: Paseo del Mar; G: Gaffey; SC: Sea Cave; L: Lighthouse; see Muhs et al. (1994, 2002a, 2006, 2014c, 2015) for terrace stratigraphic names and U-series ages. Data from Santa Cruz Island-west, Santa Cruz Island south, Santa Barbara Island, and Anacapa Island are from Muhs and Groves (2018); all other data are from Muhs et al. (2014c). (b) Same as in (a) but for mean D L values in valine. All data obtained by reverse-phrase liquid chromatography (Kaufman and Manley, 1998).
Similar to U-series-dated marine terrace corals, those terrace localities correlated to MIS 5e with amino acid racemization or epimerization methods are found within the WALIS database, along with linkage to the U-series-dated localities that served as calibration. Generalized information about each locality correlated to MIS 5e with amino acid geochronology can be found in Table S2.
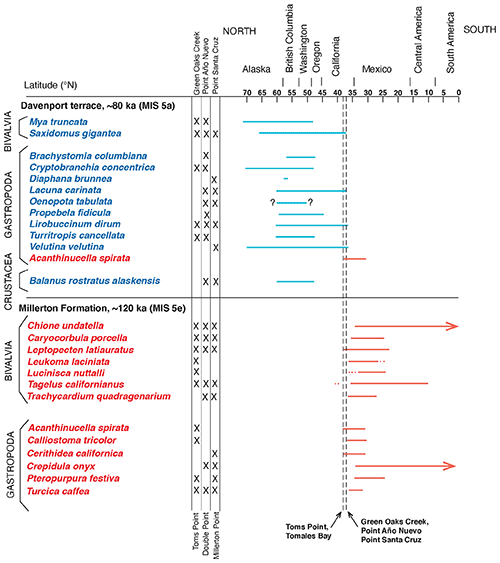
Figure 13Modern geographic ranges of extralimital and northward- or southward-ranging fossil mollusks found in ∼80 000 yr BP marine terrace deposits at Green Oaks Creek, Point Año Nuevo, and Santa Cruz, California, and the ∼130 000 yr BP Millerton Formation at Toms Point, Tomales Bay, California. Ages are from Grove et al. (1995) for the Millerton Formation at Toms Point and Muhs et al. (2006) for the other localities. Fossil data for the Millerton Formation are from Johnson (1962); Davenport terrace fossil data are from Addicott (1966) and Muhs et al. (2006). Modern species names and geographic ranges updated by the author from Abbott and Haderlie (1980), O'Clair and O'Clair (1998), and Coan et al. (2000).
3.2.3 Zoogeographic aspects of terrace faunas
In a pioneering study of marine terraces on the Pacific coast of North America, Kennedy et al. (1982) used the aminostratigraphic approach described above to extend earlier work by Wehmiller et al. (1977b). Both studies established that the lowest marine terrace along the Pacific coast of North America is not the same age at all localities due to varying rates of uplift from one reach of coast to another. In addition, Kennedy et al. (1982) noted that localities dated (by U-series on coral) to or correlated with MIS 5e host either zoogeographically “neutral” molluscan fossil faunas or faunas that contain extralimital southern species. In contrast, localities that were either dated or correlated to the ∼80 ka MIS 5a host molluscan fossil faunas with several extralimital northern species (Fig. 11). Extralimital species (or northward- or southward-ranging species) are those that, while extant, do not live at a particular locality at present but are found either entirely or mostly to the north (cool waters in this region) or to the south (warmer waters in this region). An example of a locality, dated to ∼130 ka by thermoluminescence (Grove et al., 1995), is the marine deposit in Tomales Bay, north of San Francisco, California. This deposit contains many “neutral” species, i.e., those that still live in the area at present, but also hosts a large number of extralimital southern and southward-ranging species (Fig. 13). In contrast, the Davenport terrace in the Santa Cruz, California, area, dated to ∼80 ka by U-series methods on corals (Muhs et al., 2006), hosts only one southward-ranging species but several extralimital northern and northward-ranging species. Warmer waters off California during MIS 5e and cooler waters during MIS 5a are consistent with the zoogeographic aspects of planktonic foraminiferal faunas found in deep-sea cores (Kennett and Venz, 1995) and with sea surface temperatures (SST) derived from alkenones (Herbert et al., 2001; Yamamoto et al., 2007).
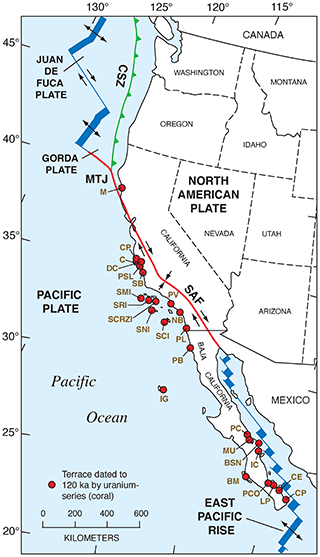
Figure 14Map of the Pacific coast of North America with structural features as shown in Fig. 2, but also plotted are localities (filled red circles) where U-series ages of corals dating to MIS 5e (∼120 ka) have been reported. Structural features are redrawn in simplified form from Drummond (1981) and Simkin et al. (2006). Abbreviations are keyed to Table S1 and are as follows: M: MacKerricher State Park; CP: Cayucos Point; C: Cayucos; DC: Diablo Canyon; PSL: Point San Luis; SB: Shell Beach; SMI: San Miguel Island; SRI: Santa Rosa Island; SCRZI: Santa Cruz Island; SNI: San Nicolas Island; PV: Palos Verdes Hills; NB: Newport Beach; SCI: San Clemente Island; PL: Point Loma; PB: Punta Banda; IG: Isla Guadalupe; BM: Bahía Magdalena; CP: Cabo Pulmo; CE: Isla Cerralvo; LP: La Paz; PCO: Punta Coyote; IC: Isla Coronado; BSN: Bahía San Nicolas; MU: Mulegé; PC: Punta Chivato; CSZ: Cascadia Subduction Zone; MTJ: Mendocino Triple Junction; SAF: San Andreas Fault.
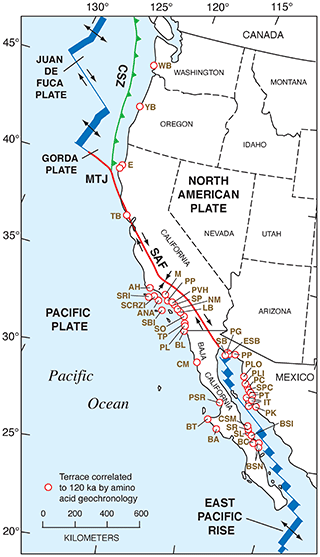
Figure 15Map of the Pacific coast of North America with structural features as shown in Fig. 2, but also plotted are localities (open red circles) where amino acid geochronology has permitted correlation of marine deposits to MIS 5e (∼120 ka). Structural features are redrawn in simplified form from Drummond (1981) and Simkin et al. (2006). Abbreviations are keyed to Table S2 and are as follows: WB: Willapa Bay; YB: Yaquina Bay; E: Eureka; TB: Tomales Bay; AH: Arroyo Hondo; SRI: Santa Rosa Island; SCRZI: Santa Cruz Island; ANA: Anacapa Island; SBI: Santa Barbara Island; M: Malibu; PP: Pacific Palisades; PVH: Palos Verdes Hills; SP: San Pedro; NM: Newport Mesa; LB: Laguna Beach; SO: San Onofre; TP: Torrey Pines; PL: Point Loma; BL: border locality; CM: Camalú; PSR: Punta Santa Rosalíllíta; BT: Bahía Tortuga; BA: Bahía Asunción; BSN: Bahía San Nicolas North; BC: Bahía Concepción; BSI: Bahía Santa Inés; SL: San Lucas; SR: Santa Rosalia; CSM: Caleta Santa Maria; SB: Salina la Borrascosa; PG: Punta Gorda; ESB: east of Salina la Borrascosa; PLO: Puerto Lobos; PLI: Puerto Libertad; PC: Punta Cuevas; SPC: southeast of Punta Cuevas; PT: Punta Tepopa; IT: Isla Tiburón; PK: Punta Kino; CSZ: Cascadia Subduction Zone; MTJ: Mendocino Triple Junction; SAF: San Andreas Fault.
Relative sea-level indicators from the Pacific coast of North America for MIS 5e and all pertinent data related to them are given in Table S1 and Fig. 14 (U-series-dated coral-bearing localities) and Table S2 and Fig. 15 (localities correlated to MIS 5e using aminostratigraphy). In the sections that follow, the regions these localities are from are discussed with respect to the nature of the sea-level record as this differs from region to region. Within the course of these discussions, previous studies are examined, and the basis for the age assignments is discussed critically. For simplicity, the review of the regions is taken from north to south. In the text that follows, there is an indication near each site discussed of what the unique RSL identification is, corresponding to the WALIS database.
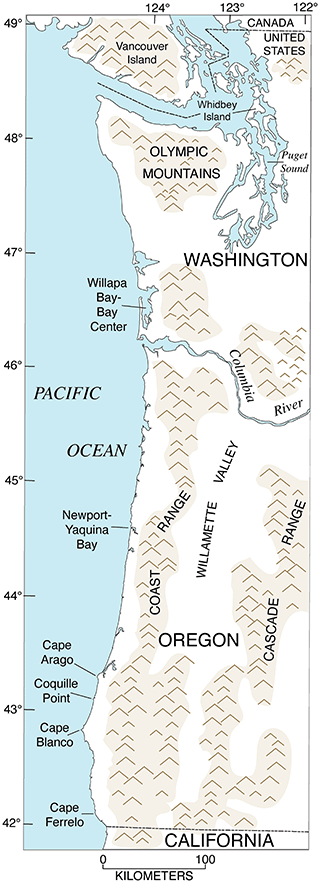
Figure 16Map of southwestern Canada, Washington, and Oregon, showing physical geography (shaded areas are mountain ranges) and localities referred to in the text. Redrawn in simplified form from U.S. Geological Survey (public domain) base maps of Washington (1982), scale 1:500 000, and Oregon (1982), scale 1:500 000.
4.1 Southwestern Canada, British Columbia
Records of marine deposits dating to MIS 5e are difficult to find on the coast of British Columbia. Erosion by repeated advances of the Cordilleran ice sheet has likely removed much of the potential record. Furthermore, the sedimentary record that does exist is highly complex due to rapid sedimentation rates, active tectonics, and glacial isostatic adjustment (GIA) effects. Mollusk-bearing glaciomarine sediments were deposited in lowland areas adjacent to coastal British Columbia or in Puget Sound when isostatic depression of these areas allowed inflow of ocean waters. Thus, at least some of the marine record that is now emergent is not strictly “interglacial” but likely occurred at the transition between a glacial period and the following interglacial period.
In southwestern Canada, most investigators have hypothesized, from the stratigraphic sections that have been studied, that the main record of MIS 5e is the Muir Point Formation (Hicock and Armstrong, 1983; Alley and Hickock, 1986; Hicock, 1990; Clague et al., 1992). On Vancouver Island in British Columbia (Fig. 16), the Muir Point Formation consists of gravel, sand, and silt, with abundant peat and wood layers, suggesting a mostly terrestrial origin, but Hicock and Armstrong (1983) hypothesize an alluvial sequence of fan to floodplain to coastal plain to delta, based on the sediment facies. Indeed, Alley and Hicock (1986) and Hicock (1990) report minor amounts of marine dinoflagellate cysts in a part of the Muir Point Formation, implying tidal or estuarine conditions, and these investigators infer a paleo-sea level of at least +10 m, relative to present. A last interglacial origin for the Muir Point Formation was hypothesized early in the study of this formation by its stratigraphic position: it has organic materials that date to >40 ka and has normal polarity but is underlain by older till and overlain by mid-Wisconsin (MIS 3) Cowichan Head Formation sediments, in turn overlain by Vashon Till dating to the Fraser Glaciation (= late Wisconsin, or MIS 2) (Alley and Hicock, 1986; Hicock, 1990). Vegetation evidence also suggests a climate at least as warm as today's, based primarily on the abundance of thermophyllous Pseudotsuga (Douglas fir) pollen, implying interglacial conditions (Hicock and Armstrong, 1983; Alley and Hicock, 1986; Hicock, 1990). An MIS 5e age is permitted by optically stimulated luminescence (OSL) ages of 119±9 and 112±11 ka from the Muir Point Formation at and near its type section (Lian et al., 1995). Because more study is needed for assessment of the age of the Muir Point Formation, no specific entry in the WALIS database was attempted here.
4.2 Washington, USA
Only two fossil-bearing localities are candidates for MIS 5e deposits in the State of Washington: one in Puget Sound and the other on the outer coast, at Willapa Bay (Fig. 16). Both have had a confusing and/or controversial history of study.
4.2.1 Whidbey Island, Puget Sound
As is the case with British Columbia, the southern Puget Sound area, within the boundaries of Washington State, has been subjected to rapid sedimentation rates, active tectonics, and GIA effects, as well as removal of much of the geologic record, due to advances and retreats of the Cordilleran ice sheet. Also similar to British Columbia, the main geologic unit that most investigators agree records the last interglacial period (MIS 5e) is not primarily a marine deposit at all but a terrestrial deposit called the Whidbey Formation. Hansen and Mackin (1949) were among the first to study the formation, noting that it occurred stratigraphically below deposits dating to the last glacial period (i.e., MIS 4 through MIS 2) and that it hosted pollen indicating an interglacial vegetation similar to that of the present. Easterbrook et al. (1967) were the first investigators to apply the formal name Whidbey Formation to the pollen-bearing unit studied by Hansen and Mackin (1949) and designate the type locality on coastal bluffs of southwestern Whidbey Island (Fig. 16). At the type section, Easterbrook et al. (1967) and Easterbrook (1968, 1969) noted that the Whidbey Formation is underlain by what is called Double Bluff Drift, consisting of till and glaciomarine sediments. At this locality, the Whidbey Formation is overlain by glacial deposits of Possession (MIS 4?) and Vashon (MIS 2) age. Easterbrook et al. (1967) conducted pollen analyses of Whidbey Formation sediments and concluded that the vegetation implied an interglacial climate similar to the present. They also reported ages that showed the unit was beyond the range of radiocarbon dating. More detailed pollen work was conducted by Heusser and Heusser (1981), who reached the same conclusions about past climate conditions. Karrow et al. (1995) reported on nonmarine fossils in the Whidbey Formation, including mollusks, ostracodes, insects, fish, vertebrates, and plant macrofossils. Their interpretations are similar to those of Hansen and Mackin (1949), Easterbrook et al. (1967), and Heusser and Heusser (1981), which is that the deposit likely represents an interglacial period with a degree of warmth similar to that of the present. It is important to note that in all of the studies just cited, the Whidbey Formation is described as a terrestrial deposit, likely formed as floodplain sediments. None of the studies cited here mention the presence of marine fossils within the deposit. Later studies have all confirmed a likely MIS 5 age for the Whidbey Formation, based on thermoluminescence (TL) dating (151±43 to 102±38 ka; Berger and Easterbrook, 1993), optically stimulated luminescence (OSL) dating (107±8 ka; Lian et al., 1995), and dating of plagioclase from pumice within the formation (128±9 ka; Dethier et al., 2008).
With the advent of amino acid geochronology, several studies presented data on some of the marine-shell-bearing deposits of Whidbey Island. Most of these studies focus on a shell-bearing deposit along Admiralty Bay, on the west coast of Whidbey Island. This deposit is visible in an ∼17 m thick coastal exposure (∼2 m above sea level) of diamicton and/or glaciomarine sediment composed of gravel, sand, and silt, with a layer of marine fossils, dominated by Saxidomus gigantea, in its uppermost part (Polenz et al., 2009). The upper contact of this complex deposit is obscured by a recent landslide, but a short distance inland and at higher (∼40 to ∼60 m) elevations, glaciomarine deposits of the Everson Interstade, outwash of the Fraser Glaciation, and till of the Vashon Stade (all of MIS 2 age) are mapped (Polenz et al., 2009) and likely overlie the shell-bearing deposits exposed at lower elevation. Kvenvolden et al. (1980) reported that this fossiliferous deposit lies stratigraphically between the Whidbey Formation and “middle Wisconsin sediments” and used amino acid ratios in Saxidomus gigantea to estimate an age of ∼80 ka. Their study, however, presents no stratigraphic evidence of the Whidbey Formation being exposed at Admiralty Bay. Blunt (1982) analyzed shells from the same locality as Kvenvolden et al. (1980) and another locality ∼150 m to the north. He used kinetic modeling to derive an age range of 77 to 99 ka for the locality studied by Kvenvolden et al. (1980) and 75–110 ka for the newer locality. In a later study derived primarily from data in Blunt (1982), Blunt et al. (1987, p. 331–332) described the Admiralty Bay deposit as belonging to the Possession Glaciation (which postdates the Whidbey Formation) but later in the same paper (p. 340 and p. 346) said that the deposits are correlated with the Whidbey Formation. These investigators also used Saxidomus gigantea and kinetic modeling to estimate ages of ∼96 and ∼107 ka for the deposit, apparently pooling the two localities. Using these data, Easterbrook (1994) correlated the deposit at Admiralty Bay with the Whidbey Formation. Kennedy et al. (1982) used the Saxidomus gigantea single-shell analysis in Kvenvolden et al. (1980) to estimate an aminozone-derived, correlated age of 80 ka and apparently used the pooled Saxidomus gigantea data in Blunt (1982) to estimate an aminozone-derived, correlated age of 120 ka. In addition, Kennedy et al. (1982) reported that the 80 ka locality hosts a cool-water fauna, and the ∼120 ka locality hosts a warm-water fauna. This is puzzling because no faunal data are given in Kvenvolden et al. (1980) or Kennedy et al. (1982), although Blunt (1982) reports a single extralimital northern or at least northward-ranging species, Mya truncata, in the fossil deposit at Admiralty Bay. Furthermore, the two localities are only ∼150 m apart and occur at roughly the same elevation according to Blunt (1982). Finally, Polenz et al. (2009) presented sedimentological data indicating that the deposits at Admiralty Bay have a glaciomarine origin. These investigators correlated the deposit either to the pre-last-interglacial Double Bluff Glaciation (their favored option) or the post-last-interglacial Possession Glaciation. In my own examinations of the deposits at Admiralty Bay, I have seen no evidence for more than one stratigraphic unit. I also agree with Polenz et al. (2009) that the shell-bearing deposit exposed there is likely glaciomarine drift, dating to the transition between the penultimate glacial period (MIS 6), represented by the Double Bluff unit, and MIS 5e. It is likely an older equivalent of the shell-bearing glaciomarine drift of the late, last-glacial Fraser glaciation, a unit called the Everson glaciomarine deposits. For this reason, this locality has not been entered into the WALIS database.
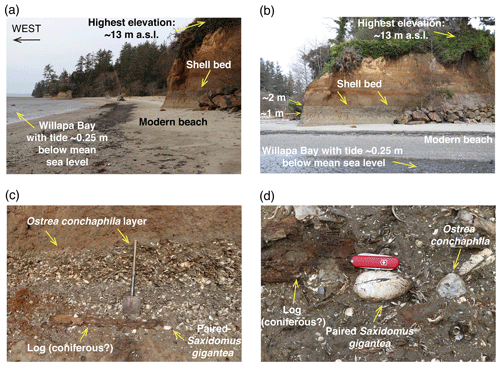
Figure 17Photographs of possible MIS 5e marine deposits in the Willapa Bay area, near Bay Center, Washington: (a, b) location of shell-bearing layer relative to modern sea level; (c, d) closeup views showing shell bed with Ostrea conchaphila, Saxidomus gigantea, and coniferous wood fragments. All photographs by Daniel R. Muhs.
4.2.2 Willapa Bay
The only other emergent, fossil-bearing locality that is a candidate for an MIS 5e deposit in Washington State is along the inner shores of Willapa Bay (RSL ID 3684) (Fig. 16). Near Bay Center, sea cliffs expose marine sediments that are richly fossiliferous (Fig. 17). Addicott (1966) reported the fossil fauna from this locality, which consists mostly of bivalves, and no taxa are extralimital or even northward or southward ranging. Kvenvolden et al. (1979) provided the first published amino acid data from this area. They recognized four stratigraphic units (I, intertidal; II, subtidal; III, subaerial; and IV, subtidal, from oldest to youngest). Their unit IV is the thickest and apparently the most extensive deposit, interpreted to have an estuarine origin; the top of this unit defines a marine terrace surface, at an elevation of ∼13 m. Almost all of the Saxidomus gigantea specimens they analyzed are from this youngest deposit. Using assumed calibration ages of ∼68 and ∼100 ka for the lowest terrace at Santa Cruz, California (Bradley and Addicott, 1968), which also hosts fossil Saxidomus gigantea, Kvenvolden et al. (1979) used linear kinetic modeling (taking temperature differences into account) to generate age estimates of 190±40 ka for units I and II and 120±40 ka for unit IV, which they correlated to MIS 7 (I and II) and MIS 5 (IV). Their terrestrial unit III was interpreted to have formed when sea level lowered during MIS 6. It is now known that U-series ages on mollusks, including the ∼68 and ∼100 ka ages for Santa Cruz reported by Bradley and Addicott (1968), are not reliable (Kaufman et al., 1971). Nevertheless, reliable U-series ages on corals from the same terrace yielded ages in between these, averaging about 80 ka (Muhs et al., 2006). Thus, the newer ages, if used as calibration, would not change the original kinetic model ages for the Willapa Bay deposits. In any case, Wehmiller (1981) challenged Kvenvolden et al.'s (1979) age estimates, arguing that nonlinear kinetic modeling is more appropriate for numerical ages using amino acid data. Using nonlinear kinetic modeling, Wehmiller (1981) recalculated the ages of units I and II and IV at Willapa Bay to be 300±50 and 70±15 ka, respectively, suggesting correlation with MIS 9 and MIS 5a. Kvenvolden et al. (1981) countered that linear kinetics could be applied to amino acid values within the ranges of what their samples yielded, and also noted that Wehmiller's (1981) age estimates would require a much more complex geologic history than their age estimates. Kennedy et al. (1982) reported new amino acid values in Saxidomus from unit IV and, using a lateral correlation (aminozone) approach, considered that the unit IV deposits at Willapa Bay were of MIS 5a age, in agreement with Wehmiller (1981). They also reported that the fauna at the Bay Center locality of unit IV hosted cool-water forms, although Addicott (1966) reported no extralimital species or northward-ranging species. The cool-water aspect of the fauna at Bay Center is apparently based on the identification of Mya japonica in these deposits, reported by Kennedy (1978). Although M. japonica was once considered to range only in the Arctic seas, from Japan to Nome, Alaska (Abbott, 1974), Coan et al. (2000) consider that M. japonica does not have differences with M. arenaria that are sufficient to merit specific status. If so, then there are no extralimital northern species in the fauna of unit IV at Willapa Bay, and the assemblage as a whole can be considered zoogeographically “neutral”. Given all the uncertainties in what has been reported thus far for Willapa Bay, it seems likely that unit IV of Kvenvolden et al. (1979) could date to MIS 5e, but more geochronological information is needed to be certain of this.
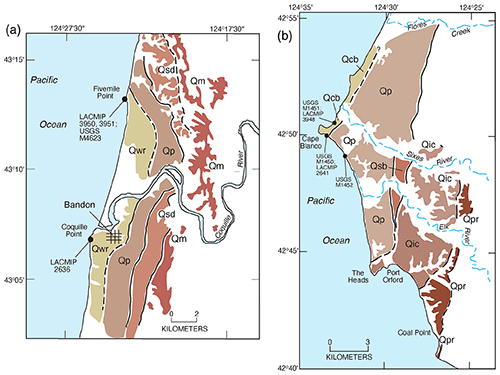
Figure 18Maps of marine terraces in the Coquille Point (a) and Cape Blanco (b) areas of southwestern Oregon. (a) Qwr, Whisky Run terrace deposits; Qp: Pioneer terrace deposits; Qsd: Seven Devils terrace deposits (correlated to MIS 5e); Qm: Metcalf terrace deposits. (b) Qcb: Cape Blanco terrace deposits; Qp: Pioneer terrace deposits; Qsb: Silver Butte terrace deposits (correlated to MIS 5e); Qic: Indian Creek terrace deposits; Qpr: Poverty Ridge terrace deposits. Redrawn from terrace maps in McInelly and Kelsey (1990) for (a) and Kelsey (1990) for (b).
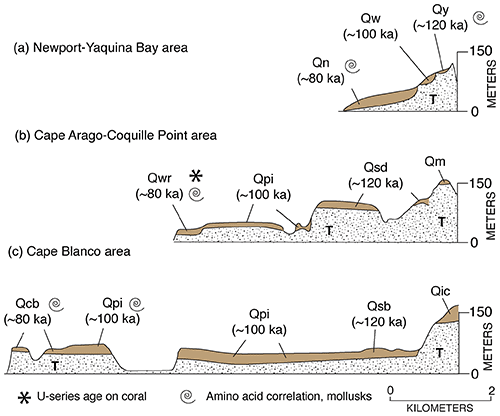
Figure 19Cross sections of marine terrace deposits in coastal Oregon in the Newport-Yaquina Bay area (a), Cape Arago–Coquille Point area (b), and Cape Blanco area (c), as well as estimated ages based on U-series dating of corals, amino acid geochronology of mollusks, and degree of soil development. Cross sections from Kelsey et al. (1996) for (a), McInelly and Kelsey (1990) for (b), and Kelsey (1990) for (c); geochronological data from Kennedy et al. (1982), Muhs et al. (1990, 2006), and Kelsey et al. (1996). Deposit abbreviations as defined in Fig. 18 caption.
4.3 Oregon, USA
Moving south from Washington, coastal Oregon is where the dominant geomorphic expression of MIS 5e shorelines as erosional marine terraces begins. The coast of Oregon is within the Cascadia Subduction Zone (Fig. 2), and most of it can be characterized as a high-wave-energy environment. Thus, erosional marine terraces are common landforms along a substantial amount of the coast, particularly in the central and southern parts of Oregon (Fig. 16). A pioneering study by Griggs (1945) involved the mapping and naming of the lowest three marine terraces in southern Oregon, and the terrace names are still in use today. More recently, detailed mapping of marine terraces along the Oregon coast has been conducted primarily by Harvey M. Kelsey and his colleagues and students (Kelsey, 1990; McInelly and Kelsey, 1990; Bockheim et al., 1992; Kelsey and Bockheim, 1994; Kelsey et al., 1996). Candidate landforms for some or all substages of MIS 5 are the lowest three terraces found along much of the central and southern Oregon coast. Kennedy et al. (1982) inferred that the lowest of these, the Whisky Run terrace near Coquille Point (Figs. 18, 19), likely correlated to MIS 5a because of a U-series age of ∼72 ka on a coral from its deposits, as well as relatively low D L leucine values in Saxidomus gigantea and a cool-water aspect to the terrace fauna (Zullo, 1969; Kennedy, 1978). Later, both alpha-spectrometry and TIMS U-series ages of corals from the Whisky Run terrace confirmed an age of ∼80 ka, and a more extensive cool-water fauna was reported (Muhs et al., 1990, 2006). Higher terraces are present in this area and north to Cape Arago, named the Pioneer, Seven Devils, and Metcalf terraces (lowest to highest), mapped by McInelly and Kelsey (1990). Based on the ∼80 ka age of the Whisky Run terrace, McInelly and Kelsey (1990) inferred that MIS 5e is represented by the Seven Devils terrace, with the Pioneer terrace correlated to MIS 5c.
Farther south, the lowest terrace at Cape Blanco also hosts a cool-water fauna (Addicott, 1964a), but based on amino acid values, Kennedy et al. (1982) interpreted this terrace to be of post-MIS 5 age, possibly as young as MIS 3. In a later study, Kelsey (1990) remapped the terraces in this area and named this the Cape Blanco terrace (Fig. 20). He correlated this with the Whisky Run terrace at Coquille Point, supported by new amino acid and oxygen isotope values in Saxidomus gigantea from Cape Blanco (Muhs et al., 1990). Kelsey also mapped and named higher landforms above the Cape Blanco terrace, the Pioneer, Silver Butte, and Indian Creek, from lowest to highest (Figs. 18, 19). He considered the Pioneer terrace to represent the MIS 5c high-sea stand and the Silver Butte terrace to represent the MIS 5e high stand. Amino acid data given by Muhs et al. (1990) support the correlation of the Pioneer terrace to MIS 5c, but no fossils have yet been found on the Silver Butte terrace.
North of Coquille Point, near Newport, Oregon, Kennedy et al. (1982) reported amino acid values in Saxidomus gigantea from a low marine terrace near Newport jetty and a higher terrace at Yaquina Bay. On the basis of these amino acid ratios and a cool-water fauna (lower terrace) and a warm-water fauna (higher terrace), Kennedy et al. (1982) correlated these terraces with MIS 5a and MIS 5e, respectively. Later mapping by Kelsey et al. (1996) identified these as the Newport (lower) and Yachats (higher) terraces, respectively, with an intermediate-elevation landform they named the Wakonda terrace. They correlated the Newport, Wakonda, and Yachats terraces with MIS 5a, 5c, and 5e, respectively.
Summarizing all these studies, U-series, amino acid, oxygen isotope, and faunal data all support a correlation of the lowest marine terrace at Newport, Coquille Point, and Cape Blanco to MIS 5a (Fig. 19). At Cape Blanco, similar amino acid ratios and oxygen isotope data correlate the Pioneer terrace with MIS 5c. At Newport–Yaquina Bay, the Yachats terrace (RSL ID 3685) is correlated to MIS 5e by amino acids and faunal data. Lack of fossils precludes correlation of intermediate and higher terraces at all these localities. To address this problem, Kelsey and Bockheim (1994) used degree of soil development to correlate undated terraces in all three areas, plus a fourth area in southernmost Oregon, near Cape Ferrelo (Fig. 16), where all terraces lack fossils. With the generation of a soil development index that utilizes time-dependent soil properties (e.g., Bt horizon thickness, color, texture, clay content), they identified, from north to south, the Yachats, Seven Devils, Silver Butte, and Gowman terraces as the likely candidates for records of the MIS 5e high-sea stand.
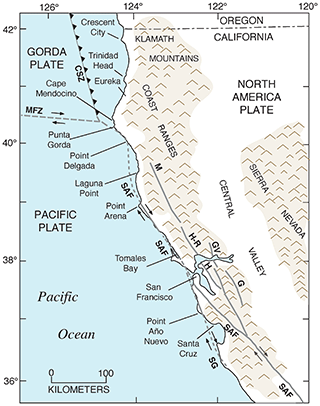
Figure 21Map of coastal northwestern California showing geographic and structural features and locations discussed in the text. CSZ: Cascadia Subduction Zone; MFZ: Mendocino Fault Zone; SAF: San Andreas Fault; SG: San Gregorio fault zone; H: Hayward Fault; M: Maacama Fault Zone; GV: Green Valley Fault; G: Greenville Fault; H-R: Healdsburg–Rodgers Creek Fault. Faults and tectonic features redrawn from Merritts (1996) and Wakabayashi (1999).
4.4 Northern California, USA
4.4.1 Crescent City coastal plain
Surprisingly few studies of marine terraces have been undertaken in northern California in part because fossil-bearing occurrences that would permit dating are rare. Northernmost California is within the Cascadia Subduction Zone, similar to coastal Oregon (Fig. 21). About 25 km south of the Oregon border, marine terraces have been studied for decades on the Crescent City coastal plain. Maxson (1933) named all the marine terrace deposits in this area collectively the Battery Formation, and he also noted the presence of fossil invertebrates in the deposits. Similarly, Delattre and Rosinski (2012) mapped deposits of the entire Crescent City coastal plain as the Battery Formation. The first attempt at dating these deposits was by Kennedy et al. (1982), who presented amino acid data from Saxidomus gigantea from low-elevation (∼7 m) sea cliff exposures in southern Crescent City. These investigators also reported a cool-water fauna from this low-elevation terrace and, on the basis of D L leucine values, correlated the terrace with MIS 5a.
In contrast to Maxson (1933) and Delattre and Rosinski (2012), Polenz and Kelsey (1999) recognized three marine terraces in this area (Qpm3, Qpm2, and Qpm1, from youngest to oldest), differentiated by subtle elevation changes and differing degrees of soil development, following the approach used by Kelsey and Bockheim (1994) in southern Oregon. Polenz and Kelsey (1999) correlated the three terraces they mapped (Qpm3, Qpm2, Qpm1) with MIS 5a, 5c, and 5e, respectively, although they noted that Qpm1 could correlate with MIS 7. The localities studied by Kennedy et al. (1982) are situated on what Polenz and Kelsey (1999) mapped as Qpm2, the terrace they correlated with MIS 5c. It should be noted, however, that it is questionable whether amino acid ratios can distinguish ∼80 ka deposits from ∼100 ka deposits, and cool-water faunas are expected from terraces of either age, based on alkenone paleotemperature data from a nearby deep-sea core (ODP 1020) studied by Herbert et al. (2001). Thus, the best evidence for a possible MIS 5e shoreline in this area is the Qpm1 terrace mapped by Polenz and Kelsey (1999), found mostly inland of the younger terraces. This terrace has maximum platform elevations of ∼29 to ∼15 m.
4.4.2 Trinidad Head area
Marine terraces are scarce between Crescent City and along a coastal reach ∼60 km to the south. However, in the Trinidad Head area (Fig. 21), there are multiple marine terraces, well expressed geomorphically. This area, like the Crescent City coastal plain, is also within the Cascadia Subduction Zone. Carver (1992) mapped seven terraces in this area, with additional undifferentiated higher-elevation terraces. Based on ages assigned from the oxygen isotope record and an untested assumption of a constant uplift rate, Carver (1992) gave estimated terrace ages that were also followed by Delattre and Rosinski (2012). The rationale for these age assignments is reported to be from degree of soil development and thermoluminescence (TL) ages reported by Berger et al. (1991). However, the method by which ages from degree of soil development are derived is not described, and all of the TL ages reported by Berger et al. (1991) are either not consistent with Carver's (1992) mapping or date younger deposits that overlie the marine terrace deposits. Although not mapped, McCrory (2000) also presented shore-parallel terrace profiles for seven marine terraces in this area, with shoreline angle elevations ranging from ∼15 to ∼255 m. No numerical ages are available, but McCrory (2000) used a graphical method of estimating terrace ages, as described by Lajoie (1986). Finally, Padgett et al. (2019) remapped the terraces in this area and assigned ages based on degree of soil development and an assumption that the terrace with the most prominent inner edge (their “Surface 3”) dates to MIS 5e. They further assumed that the lower-elevation “Surface 1” and “Surface 2” terraces date to MIS 5a and 5c, respectively. Thus, the mapping and age assignments of Carver (1992) and Delattre and Rosinski (2012) disagree with those of Padgett et al. (2019), but it is important to emphasize that none of these studies have any supporting numerical ages. Interestingly, pre-MIS 5e ages are given by Kennedy et al. (1982) for marine deposits in this area, based on D L values in Saxidomus gigantea, but because no geomorphic or stratigraphic data are given, it is not known how these aminostratigraphic data can be linked to the other studies. Much more work needs to be done on dating the terraces in this area, and although it seems likely that an MIS 5e record is present, it cannot be determined at this time which shoreline is representing it.
4.4.3 Eureka–Cape Mendocino area
The Eureka area is situated within the southernmost part of the Cascadia Subduction Zone, but Cape Mendocino is close to the Mendocino Triple Junction, where the North America, Gorda, and Pacific plates intersect (Fig. 21). McLaughlin et al. (2000) mapped the geology of the Eureka, California, area, as well as the Cape Mendocino area to the south of Eureka. Within the Eureka area itself, these investigators mapped a unit simply called “Qt”, which is primarily nonmarine, fluvial terrace deposits but which also includes shallow marine deposits, including an informally named deposit called the “Hookton marine” unit (Ogle, 1953). Wehmiller et al. (1977a) and Kennedy et al. (1982) reported leucine D L values in Saxidomus gigantea from two localities in the Eureka area (one of which is at an elevation of 15–17 m, RSL ID 3686). Both localities are within the “Qt” unit of McLaughlin et al., 2000) that would permit correlation of the host deposits with MIS 5e. One of these localities is reported to host a warm-water fauna, and the other is reported to host a zoogeographically “neutral” fauna, but stratigraphic and faunal details are not given. It seems likely that the Eureka area hosts marine deposits that correlate to MIS 5e, but more detailed work is required to confirm this.
Between Cape Mendocino and Point Delgada, the region is within the influence of the tectonically active Mendocino Triple Junction (Fig. 21). Along this rugged part of the coast, numerous terraces have been identified, including some dating to the Holocene and even historic time, as described later. For the Pleistocene, terrace elevation transects were reported by Merritts and Bull (1989). No numerical ages are given, but terraces correlated to MIS 5e were reported at elevations of ∼150 m (a short distance north of Point Delgada) and at ∼250 m (near Punta Gorda). These correlations were made using a graphical correlation method described by Bull (1985). Bull's (1985) method was developed before much was known about the importance of GIA effects, which have been shown to affect the California coast, and this issue is discussed later. Thus, whether the correlations of Merritts and Bull (1989) of the terraces in the Punta Gorda and Point Delgada areas to MIS 5e are valid or not will have to await independent dating.
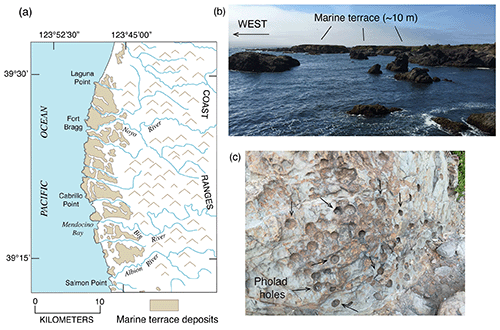
Figure 22(a) Map showing the distribution of marine terraces in the Laguna Point–Fort-Bragg–Cabrillo Point area (redrawn from Jennings and Strand, 1960); (b) photograph of 10 m high marine terrace near Laguna Point, correlated to MIS 5e (Merritts and Bull, 1989); (c) pholad holes in outer edge of 10 m high, MIS 5e terrace. Photographs by Daniel R. Muhs.
4.4.4 Laguna Point to Point Arena
Between Laguna Point and Point Arena, marine terraces form the coastal plain area of this part of northern California. This area is south of the Mendocino Triple Junction and is within the strike-slip tectonic region of the San Andreas Fault zone (Fig. 21). Although detailed mapping has not been conducted in this area, general terrace maps are available, and a portion of terraced coastline is shown here (Fig. 22). Merritts and Bull (1989) have reported elevations of terrace inner edges on shore-normal transects in this area as well. These investigators report as many as six marine terraces in the Cabrillo Point area, from ∼10 to ∼130 m above sea level. Unfortunately, only one locality, thus far, has yielded fossils in this reach of coastline. On the lowest terrace (shoreline angle of ∼10 m) at Laguna Point, within MacKerricher State Park (Fig. 14), Kennedy et al. (1982) reported D L leucine ratios on Saxidomus gigantea fragments, as well as a fauna with extralimital northern species. The amino acid ratios on these shells plotted above their ∼80 ka aminozone, creating a dilemma: the amino acid data suggested correlation with MIS 5e, but the fauna was typical of MIS 5a deposits. Dorothy J. Merritts of Franklin and Marshall College returned to this locality and recovered two solitary corals (Balanophyllia elegans) that she submitted to laboratories at the U.S. Geological Survey. For this review, she kindly allowed use of these previously unpublished data. One coral has a low U content and an apparent age of ∼156 ka, too old to be considered to be of MIS 5e age but likely biased old because of U loss. However, the other coral has a U content of 4.82±0.10 ppm, a 232Th content of 0.06 ppm, a value of 180, a value of 1.0976±0.0016, a value of 0.7771±0.0024, and an age of 130.4±0.9 ka. The back-calculated initial value of 1.1411±0.0022 is within the range of modern seawater, giving the age of ∼130 ka a high degree of confidence. This age is consistent with the age implied by the amino acid data reported by Kennedy et al. (1982). The issue of an MIS 5e terrace such as this, containing a cool-water fauna, is contrary to the general model proposed by Kennedy et al. (1982). These apparently contradictory observations can be reconciled, however, in areas of low uplift rate by the formation of an MIS 5e terrace, followed by reoccupation (and fossil reworking) during the MIS 5c high-sea stand, a topic that is explored in more detail later.
Marine terraces at Point Arena and south of it have been mapped by Muhs et al. (2003, 2006), who report three terraces in this area. Corals from their “Qt1”, the lowest terrace (∼20–25 m), were dated by alpha-spectrometric methods to ∼80 ka, or MIS 5a (Muhs et al., 1990, 1994). Later dating of coral from this terrace by TIMS also gave an age of ∼80 ka, and paleontological studies yielded a fauna with extralimital northern forms (Muhs et al., 2006). All these results are in good agreement with those of Kennedy et al. (1982), who correlated the terrace to MIS 5a on the basis of D L leucine values in Saxidomus gigantea. The two higher terraces mapped by Muhs et al. (2003) have elevations of ∼40–45 m (Qt2) and ∼60–65 m (Qt3). A reasonable working hypothesis is that Qt3 represents the last interglacial peak (MIS 5e) high-sea stand and that the intermediate Qt2 terrace formed during the MIS 5c high-sea stand. Unfortunately, fossils on both of these terraces have yet to be found, so testing of this hypothesis is not yet possible.
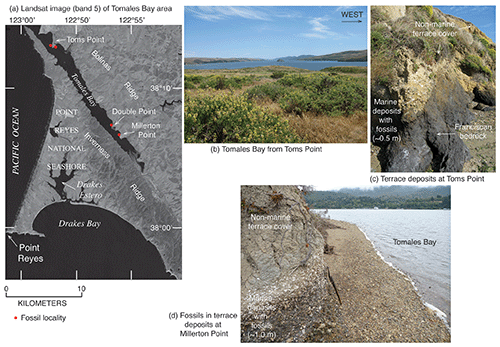
Figure 23(a) Landsat band 5 image (from U.S. Geological Survey) of the Tomales Bay, California, area with fossil localities of the Millerton Formation (filled red circles) from Johnson (1962); the formation is correlated to MIS 5e by thermoluminescence (Grove et al., 1995) and amino acid geochronology (Muhs and Groves, 2018). (b) Ground photograph from Toms Point, looking south to Tomales Bay. (c) Marine terrace deposits with fossils exposed on wave-cut bench at Toms Point. (d) Marine deposits with fossils exposed at Millerton Point. Photographs by Daniel R. Muhs.
4.4.5 Tomales Bay
Tomales Bay (RSL ID 3794) has been of interest to geologists because of its unusual configuration, conditioned largely by the fact that the San Andreas Fault zone is situated within the bay, parallel to the bay's long axis (Fig. 23). At a few points on the eastern side of Tomales Bay, there are exposures of a marine deposit called the Millerton Formation, long considered to be of Pleistocene age. On the most recent geologic map of the area, the formation is simply included within what is mapped as “marine terrace deposits” (Graymer et al., 2006). At Toms Point, it is a fossil-rich bed (with abundant Saxidomus and Chione shells), ∼0.5 m thick, overlying a bench cut on Franciscan rocks and overlain by nonmarine terrestrial deposits. The shell-rich bed is ∼8–9 m above sea level at Toms Point. At Millerton Point, the beds are gravelly, ∼1.0 m thick, and rich in Ostrea and Leukoma (formerly Protothaca) shells, all exposed just above modern beach level. Johnson (1962), who conducted the most thorough study of the fossils from the Millerton Formation, noted that several extralimital southern and southward-ranging species are present (Fig. 13) with no northern species, implying water temperatures much warmer than those at Tomales Bay today. Kennedy et al. (1982) noted the warm-water aspect of the fauna in the Millerton Formation and presented D L leucine data in Saxidomus that fall slightly above their ∼120 ka, MIS 5e aminozone. These investigators did not specifically accept or reject a possible MIS 5e age for the formation. Grove et al. (1995) studying the tectonics of the area, reported a TL age of 134±12 ka for the Millerton Formation (analyzed by Glenn W. Berger). Unfortunately, no analytical data are given for further consideration of this TL age. However, Muhs and Groves (2018) presented D-alloisoleucine / L-isoleucine data for Chione from the Millerton Formation, collected at Toms Point. Their data fall into a last interglacial (MIS 5e) aminozone when compared with a south-to-north transect of similar data from Chione in Baja California and California. More dating of this important formation would be highly desirable, but the available information indicates that the Millerton Formation along the northeast shores of Tomales Bay represents deposits that can be correlated to MIS 5e.
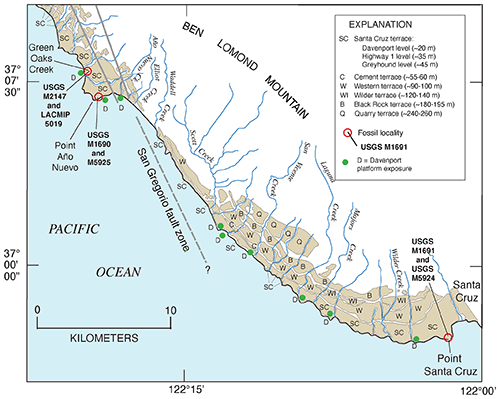
Figure 24Map of a portion of the central coast of California, from Santa Cruz to just north of Point Año Nuevo, showing marine terraces, fossil localities, and location of the San Gregorio fault zone (solid gray lines; dashed where uncertain). Marine terrace inner edges redrawn from Bradley and Griggs (1976) and Weber et al. (1979); location of the San Gregorio fault zone from Weber et al. (1979) and Weber (1990).
4.5 Central California, USA
4.5.1 Point Año Nuevo–Santa Cruz area
South of San Francisco, marine terraces dominate many parts of the coast of central California. As noted earlier, it was in this area, east of Santa Cruz (Fig. 21), that Alexander (1953) formulated the modern concept of how marine terraces form on tectonically active coastlines, specifically as landforms cut during interglacial sea-level high stands superimposed on crustal blocks experiencing steady uplift. Just west of where Alexander (1953) worked, Bradley and Griggs (1976) mapped six prominent terraces in the Santa Cruz–Point Año Nuevo area (Fig. 24). The lowest of these six marine terraces, between Santa Cruz and extending to at least just north of Point Año Nuevo, is called the Santa Cruz terrace. Detailed seismic profiling and examination of outcrops carried out by Bradley and Griggs (1976) show that the Santa Cruz terrace actually consists of three distinct platforms cut on bedrock. However, the three wave-cut platforms are covered with marine and, importantly, nonmarine deposits that have smoothed over the subaerial surface topographically into a single, broad landform. Bradley and Griggs (1976) referred to the three buried platforms as the Greyhound level (∼45 m), Highway 1 level (∼35 m), and Davenport level (∼20 m). These elevations are rough averages as the shoreline angle elevations vary as a function of where they are situated with respect to active geologic structures. Too few exposures allow for these terraces to be mapped separately, but isolated outcrops where the Davenport platform can be identified are present along the coast between Santa Cruz and north of Point Año Nuevo (Fig. 24). Above the Santa Cruz terrace complex, higher terraces are found at ∼55–60 m (Cement terrace), ∼90–100 m (Western terrace), ∼120–140 m (Wilder terrace), ∼180–195 m (Black Rock terrace), and ∼240–260 m (Quarry terrace).
For years, there has probably been no greater speculation about the age of a coastal landform in California than that of the Davenport platform between Point Año Nuevo and Santa Cruz. Anderson and Menking (1994) reviewed many of the previous age estimates made for this terrace. Bradley and Addicott (1968) reported U-series ages of mollusks from this terrace that ranged from ∼100 to ∼60 ka, but it is now well known that U-series analyses of mollusks are not reliable (Kaufman et al., 1971). In a later study, Bradley and Griggs (1976) recognized this problem and suggested instead that the Highway 1 platform, present just above the Davenport platform, was cut during the ∼120 ka (MIS 5e) high-sea stand. They interpreted the Davenport platform, although at a lower elevation, to have been cut during a hypothesized lower, ∼140 ka sea stand, such as that seen at reef VIIa on New Guinea (Bloom et al., 1974). Based on amino acid ratios in fossil mollusks and the faunal zoogeographic aspect, Kennedy et al. (1982) concluded that the fossils on the Davenport platform dated to the ∼80 ka high stand of sea during MIS 5a. Assuming an age of ∼120 000 years for the Highway 1 terrace, Lajoie et al. (1991) estimated an age of ∼100 ka (MIS 5c) for the Davenport terrace. Perg et al. (2001) used cosmogenic isotopes to estimate an age of 65 ka for the Highway 1 terrace where it is found just northwest of Santa Cruz, which would correlate the terrace with MIS 3. These workers offered cosmogenic isotope ages for the higher terraces as well, including ∼92 ka (Western terrace), correlated to MIS 5a; ∼137 ka (Wilder terrace), correlated to MIS 5c; ∼139 ka (Black Rock terrace), correlated to MIS 5e; and ∼226 ka (Quarry terrace), correlated to MIS 7. The age estimates of Perg et al. (2001) combined with the elevations given above would characterize this reach of coastline as having one of the highest rates of uplift along the California coast, exceeded only by the coast near the Mendocino Triple Junction and south of the “big bend” of the San Andreas Fault, as discussed later.
Muhs et al. (2006) reported U-series ages on corals from Green Oaks Creek, Point Año Nuevo, and Point Santa Cruz, all from deposits of the Davenport level of the Santa Cruz terrace (Fig. 24). A total of 12 corals gave ages ranging from ∼84 to ∼76 ka, and all 11 corals collected near Green Oaks Creek have back-calculated initial values ranging from 1.154 to 1.1460. These values fall well within the range of modern seawater, giving a high degree of confidence that the corals have experienced closed-system histories with respect to U-series isotopes. In addition, deposits of the Davenport terrace have faunas containing a large number of extralimital northern or northward-ranging species of mollusks, consistent with dated, ∼80 ka terraces elsewhere in Oregon and California (Addicott, 1966; Kennedy, 1978; Muhs et al., 2006). The U-series ages of ∼80 ka agree with the amino acid age estimate reported earlier by Kennedy et al. (1982).
Although Perg et al. (2001) did not analyze sediments from the Davenport platform directly, their ages for the higher terraces imply that the Davenport terrace should correlate with one of the interstadial high stands of sea, recorded as uplifted, coral reef terraces on New Guinea. These terraces date to ∼50 000, ∼40 000, or ∼30 000 yr BP (Chappell et al., 1996; Cutler et al., 2003). However, based on the U-series ages for corals from the Davenport terrace, it is very likely that the cosmogenic ages for the older Santa Cruz terraces are underestimates. A reasonable explanation is that the ages reported by Perg et al. (2001) reflect the ages of alluvium that overlies the marine deposits. The terrestrial sedimentary cover in this area is typically much thicker than the marine cover, and marine sediments are rarely, if ever, exposed at the ground surface. For example, at Point Año Nuevo, the sea cliff exposes the Davenport platform at ∼7.8 m above sea level, overlain by ∼0.5 to ∼0.2 m of marine deposits with fossils. However, above the marine deposits are ∼9.8 m of alluvial sands and gravels, interbedded with silts and clays. A well-developed soil, with an A/E/Bt [Bts]/C profile developed in these nonmarine deposits, indicates a substantial age for this alluvium.
In light of the ∼80 ka (MIS 5a) age for the Davenport terrace, a reasonable working hypothesis is that the other platforms in the Santa Cruz terrace complex date to earlier high-sea stands of MIS 5. Thus, the Highway 1 platform could date to MIS 5c, and the Greyhound platform could date to MIS 5e. If this correlation is correct, then much of the MIS 5e shoreline (represented hypothetically by the Greyhound platform) has been eroded away. Based on the shore-parallel elevation profiles of Bradley and Griggs (1976), less than ∼4 km of the shore-parallel extent of this terrace still exists along ∼32 km of coastline that they mapped. In contrast, the Highway 1 platform occurs nearly continuously from the city of Santa Cruz northwest to Point Año Nuevo. If this platform was cut during MIS 5c, then much of the MIS 5e (Greyhound) terrace must have been removed before much uplift could take place, contrary to the high uplift rates implied by Perg et al. (2001). The challenge here, as in many places, is to devise a method of dating terraces that lack fossils.
4.5.2 San Luis Obispo County, California
In northern San Luis Obispo County, five marine terraces have been mapped by Hanson et al. (1994) in the area around San Simeon (Fig. 25). These terraces are, in ascending order, the Point (7–8 m), San Simeon (4–23 m), Tripod (23–38 m), Oso (29–47 m), and La Cruz (53–79 m) terraces. Terrace elevations vary as a function of proximity to the northwest-trending San Simeon Fault zone. Unfortunately, fossils are apparently lacking in this area, so there is little age control for any of these terraces. Hanson et al. (1994) correlated the Tripod terrace to MIS 5e, based on a simple lateral correlation to the low-elevation marine terrace exposed near Cayucos to the south (see discussion below). However, this correlation is currently only a working hypothesis as the Cayucos terrace is ∼35 km distant, and it is not certain that such a long, shore-parallel correlation can be justified.
West of the town of Cayucos (RSL ID 3688) a broad marine terrace extends along the coast for several kilometers, as discussed earlier (Fig. 6a). This terrace and its deposits are well known in part because the terrace sediments host a rich molluscan fauna (Valentine, 1958) but also because it is the first terrace in California where a coral was dated by U-series (Veeh and Valentine, 1967). The latter workers reported an age of ∼130 ka for this coral, which is recalculated here to ∼122 ka using more recent estimates of the half-lives of U-series nuclides. With this correlation to MIS 5e, the Cayucos terrace has been an important calibration point for many aminostratigraphic studies (Wehmiller et al., 1977b; Kennedy et al., 1982; Wehmiller, 1982; Muhs et al., 2014c). The terrace is broad, with a shore-normal extent of up to ∼600 m. Although it has been reported that the shoreline angle of the terrace, at around 7–8 m above sea level, is exposed near the town of Cayucos itself (Stein et al., 1991), this measurement is actually of the wave-cut bench behind a paleo-sea stack. However, the measurement is not greatly different from that made by extending a shore-normal topographic profile of the wave-cut bench landward and finding its possible intersection with an extension of the paleo-sea cliff topographic profile downward (Fig. 6b), which yields a possible shoreline angle elevation of ∼8 m. In this area (RSL IDs 3776, 3801), apparent U-series ages of corals range from 143 ka to 119 ka with high initial 234U/238U values (Muhs et al., 2002a).
Using TIMS U-series methods, Stein et al. (1991) analyzed 12 corals from near LACMIP (Natural History Museum of Los Angeles County Invertebrate Paleontology) loc. 10731 (RSL ID 3688), near the town of Cayucos (Figs. 6a, 25). A total of 11 corals from this locality gave ages ranging from ∼125 to ∼113 ka, with somewhat elevated initial values, indicating that the ages are probably biased old to some degree but still likely correlative to MIS 5e. However, one coral gave an apparent age of ∼101 ka, with an initial value that was only slightly elevated, indicating minimal age bias. Muhs et al. (2002a) revisited the same locality and analyzed seven corals, with four yielding ages of ∼123 to ∼116 ka, in broad agreement with Stein et al. (1991), and with three giving ages of ∼110 to ∼108 ka. Muhs et al. (2002a) interpreted these data to indicate the possibility that during MIS 5c at ∼100 ka, the high-sea stand overtook at least the outer part of the terrace created during MIS 5e at ∼120 ka, with the result that fossils of two ages were mixed together. This interpretation is supported by a reexamination of the fossil fauna reported by Valentine (1958) using updated modern zoogeography. Although the fauna contains several extralimital southern species of mollusks, as well as some southward-ranging mollusks, it also contains some extralimital northern and northward-ranging species. This mix of warm-water (∼120 ka?) and cool-water (∼100 ka?) molluscan forms was interpreted by Muhs et al. (2002a) to be consistent with the apparent mix of ∼120 and ∼100 ka corals.
South of Cayucos, in the area of Diablo Canyon (RSL ID 3808), Point San Luis (RSL ID 3777), and Shell Beach (RSL ID 3807) (Fig. 25), Hanson et al. (1994) mapped a sequence of at least 12 marine terraces up to an elevation of at least ∼200 m. The lowest two terraces, Q1 (12–4 m) and Q2 (34–12 m) were correlated with MIS 5a and 5e, respectively, using a variety of dating methods. A U-series age, recalculated here using updated half-lives, on corals from the Q2 at Point San Luis (done by alpha spectrometry) is ∼118 ka and supports this correlation (Muhs et al., 1994), as do ages (by TIMS) on corals from Shell Beach that range from ∼127 to ∼122 ka (Stein et al., 1991). However, at other localities of the Q2 terrace, Hanson et al. (1994) noted inconsistencies between amino acid age estimates of the terrace mollusks and zoogeographic aspects of the faunas. For example, at some localities, amino acid ratios would imply correlation to MIS 5a, but faunas have a warm-water aspect, implying a correlation to MIS 5e. At other localities, such as Shell Beach, both amino acid data and faunal data would indicate an MIS 5a age (Hanson et al., 1994), but U-series data by Stein et al. (1991) imply an MIS 5e age. Some of these issues were also discussed by Wehmiller (1992) and Kennedy (2000), but a lack of details of the faunas and amino acid data do not allow for complete resolution of the problem. At one locality on the Q2 terrace, near Diablo Canyon, Muhs et al. (1994) reported a U-series age on coral (by alpha spectrometry) of ∼108 ka, implying correlation with MIS 5c. If that age is correct, then it is possible that, like Cayucos, the Q2 terrace in this area contains fossils of both MIS 5c and 5e age, which would explain the occurrence of both cool-water (∼100 ka) and warm-water (∼120 ka) forms on the same terrace. More work is needed at localities on both the Q1 and Q2 terraces to resolve this problem.
4.6 Southern California, USA
Coastal southern California is defined here as that part of the coast that is east or south of Point Conception (Fig. 25). This point is a major geographic feature because here the California coast changes from a north–south orientation to an east–west one. The change in coastal orientation is structurally controlled by the orientation of the San Andreas Fault, which has a major restraining bend (informally referred to as the “Big Bend”) inland of and to the northeast of Point Conception. Point Conception also marks a major faunal boundary in the marine invertebrate communities of the Pacific coast of North America, long recognized by marine zoogeographers (Valentine, 1966). This has relevance to the interpretation of marine terrace faunas, as discussed in more detail below.
4.6.1 Point Conception to Arroyo Hondo
Marine terraces form the coastal plain between Point Conception and Arroyo Hondo (Fig. 25). Upson (1951) was the first to study these terraces and map them. He also noted the presence of marine fossils in some of the deposits and reported identifications of the taxa, based on an examination of his collections by Wendell P. Woodring. Preliminary age assignments for low-elevation terraces in this area, based on amino acid ratios, were made by Kennedy et al. (1982). Later, however, Rockwell et al. (1992) mapped five terraces (I, II, III, IV, and V, from lowest to highest) in the area, and Kennedy et al. (1992) provided new amino acid data and faunal lists from terraces I, II, and III. Terrace I, also called the Cojo terrace, has shoreline angle elevations varying from ∼17 to ∼10 m, depending on proximity to local structures. Two bone specimens from deposits overlying this terrace gave concordant and ages of ∼70 and ∼87 ka, leading Rockwell et al. (1992) to conclude that these were close minimum-limiting ages and permitted correlation with MIS 5a. Two localities on terrace I have D L leucine and valine values in Saxidomus that are significantly lower than those of this genus from deposits from terrace III (∼40–30 m), which Rockwell et al. (1992) correlated with MIS 5e (RSL ID 3687). The intermediate-elevation terrace II is correlated with MIS 5c. Faunas in deposits of both terraces I and II have a cool-water aspect, whereas the fauna of terrace III has a warm-water aspect, supporting these age assignments (Kennedy et al., 1992). Thus far, no corals have been reported in terrace deposits in this area.
4.6.2 Malibu
Marine terrace deposits along the Malibu coast of Los Angeles County have been studied since the landmark paper of Davis (1933), who named the two most prominent landforms the Malibu (higher) and Dume (lower) terraces. These two terraces, plus an intermediate one called the Corral terrace, were mapped in detail by Birkeland (1972), who also measured the shoreline angle elevations. These elevations vary in a shore-parallel sense, ranging from 76 to 61 m (Malibu), 54 to 46 m (Corral), and 40 to 15 m (Dume). Szabo and Rosholt (1969) analyzed mollusks from the Corral (called “Terrace C” in their paper) and Dume terraces for U-series isotopes, including 231Pa and 235U. They recognized that mollusks are open systems with regard to U-series isotopes but devised an open-system model of age determination. From this model, they proposed ages of ∼154 to ∼115 ka (average of ∼131 ka) for the Corral terrace and ∼112 to ∼95 ka (average of ∼104 ka) for the Dume terrace. Szabo and Rosholt (1969) correlated the Corral terrace to the ∼120 ka Rendezvous Hill (“Barbados III”) terrace of Barbados and the Dume terrace to the ∼105 ka Ventnor (“Barbados II”) terrace of the same island. The open-system model received considerable criticism from Kaufman et al. (1971), who concluded that mollusks were not suitable for U-series dating, using either closed-system or open-system approaches. Interestingly, Simms et al. (2016) nevertheless accepted the ∼131 and ∼104 ka mollusk ages and correlated the Corral and Dume terraces with MIS 5e and 5c, respectively. Kennedy et al. (1982), however, reported amino acid data from the Dume terrace that suggested correlation with MIS 5e, supported by the presence of a warm-water fauna, studied earlier by Addicott (1964b). Although more work needs to be conducted on these terraces, it seems likely that the lowest, Dume terrace (RSL IDs 3689, 3690), is the most probable representative of MIS 5e.
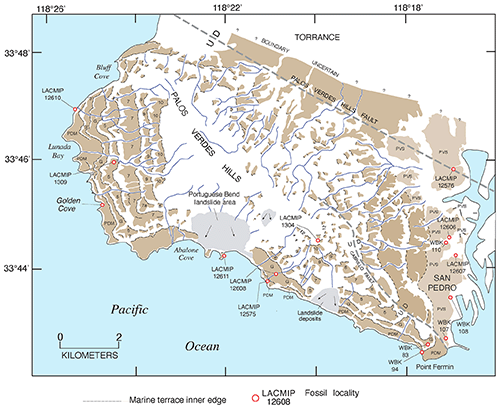
Figure 26Map of marine terrace deposits (brown shades), terrace inner edges (black lines), fossil localities (open red circles), landslide deposits (gray shades), and faults in the Palos Verdes Hills area, Los Angeles County, California. Redrawn from Woodring et al. (1946). PVS: Palos Verdes Sand, part of which is of MIS 5e age (Muhs and Groves, 2018); PDM: Paseo del Mar terrace (∼80 ka, MIS 5a); G: Gaffey terrace (∼120 ka, MIS 5e; Muhs et al., 2006). LACMIP fossil locality numbers; WBK: fossil localities of Woodring et al. (1946).
4.6.3 Palos Verdes Hills–San Pedro
The Palos Verdes Hills, also in Los Angeles County, is an uplifted crustal block with at least a dozen marine terraces (Fig. 26). The crustal block is bounded by faults on its southeast and northern sides. Based on mapping of terraces in a now-classic study by Woodring et al. (1946), the Palos Verdes Hills were likely an island during some point or points in their history, most recently during the last interglacial period, or MIS 5e. Woodring et al. (1946) numbered the terraces from “1” (the lowest terrace within the city of San Pedro) to “13” (the highest in the Palos Verdes Hills). The marine deposits overlying terrace 1 in San Pedro were referred to as the Palos Verdes Sand (RSL IDs 3691, 3772, 3773, 3795), and this unit was regarded as being of the same age throughout its mapped extent, although it was recognized that there are substantial differences in the faunal character from place to place (Woodring et al., 1946). To the west, on the Palos Verdes Hills, deposits of all the terraces were considered by Woodring et al. (1946) to be older than the Palos Verdes Sand, so the lowest-elevation terrace there was referred to as terrace 2. Aminostratigraphic work by Muhs et al. (1992) showed that the Palos Verdes Sand in northern San Pedro is correlative with terrace 4 (∼72 m) on the Palos Verdes Hills, and the Palos Verdes Sand in southern San Pedro is correlative with terrace 2 (∼47 m) on the Palos Verdes Hills (Fig. 26). These investigators correlated terrace 4 with MIS 5e and terrace 2 with MIS 5a, based on both aminostratigraphy and terrace faunas (warm-water faunas on terrace 4; cool-water faunas on terrace 2). Because of these correlations, Muhs et al. (2006) considered that the terrace numbering system of Woodring et al. (1946) was misleading and instead named terrace 2 the “Paseo del Mar” terrace and terrace 4 the “Gaffey” terrace (Figs. 26, 27). These correlations remained untested until corals were recovered from both terraces 2 (Paseo del Mar terrace) and 4 (Gaffey terrace; RSL IDs 3771, 3784). U-series ages by TIMS gave ages of ∼80 ka (MIS 5a) from three localities on terrace 2 and ages of ∼119 to ∼113 ka (MIS 5e) from a single locality on terrace 4 (Muhs et al., 2006). It is likely that intermediate-elevation terrace 3, found only on the west side of the Palos Verdes Hills, correlates with MIS 5c, but fossil corals or mollusks from this terrace have not yet been found.
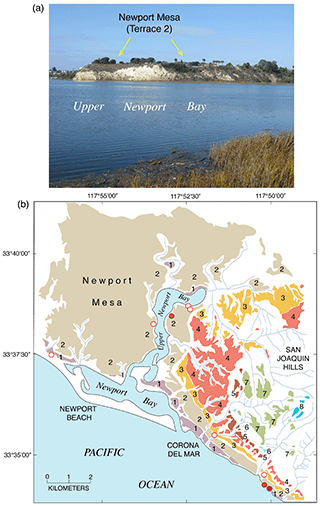
Figure 28(a) Photograph of Newport Mesa (terrace 2) of MIS 5e age, view to the west, upper Newport Bay in the foreground. (b) Map of marine terraces in the Newport Bay area, redrawn from Vedder et al. (1957, 1975) and Grant et al. (1999); terrace elevations and ages increase with terrace numbering (terrace 1 = youngest; terrace 8 = oldest). Filled red circles are fossil localities with U-series ages from Grant et al. (1999); open red circles are amino acid geochronology fossil localities of Wehmiller et al. (1977b). Photograph in (a) by Daniel R. Muhs.
4.6.4 Newport Bay area
The Newport Bay area of Orange County, California, south of Los Angeles, has long been known for its highly fossiliferous marine terrace deposits (RSL IDs 3692, 3693, 3796, 3820, 3821). Terraces were mapped by Vedder et al. (1957) and then remapped by Vedder et al. (1975). The most extensive of these is the area locally referred to as Newport Mesa (Fig. 28). Grant et al. (1999) measured the shoreline angle elevations of eight terraces in this area, and Newport Mesa corresponds to their “Terrace 2”. The shoreline angle elevations of this terrace range from ∼32 to 36 m. A lower-elevation surface (their “Terrace 1”) has shoreline angle elevations ranging from ∼19 to 22 m. From a fossil locality in the eastern part of Terrace 2, Kanakoff and Emerson (1959) reported what is likely the most abundant marine invertebrate fauna of Pleistocene age on the Pacific coast of North America, with at least 500 species of mollusks, corals, bryozoans, brachiopods, echinoids, crabs, barnacles, and worms. When the suitability of U-series dating of mollusks was still in a stage of assessment, Szabo and Vedder (1971) attempted dating fossils by this method from the lowest three terraces in the area. As is usually the case with mollusks, virtually all the specimens analyzed had evidence of open-system histories. Wehmiller et al. (1977b) and later Kennedy et al. (1982) analyzed mollusks from the area for amino acid geochronology primarily using the genera Saxidomus and Leukoma. They showed that mollusks from half a dozen localities on Terrace 2 likely date to MIS 5e, but at least three localities have evidence of older, pre-MIS 5e fossils, one of which is the main locality studied by Kanakoff and Emerson (1959). Grant et al. (1999) conducted TIMS U-series analyses of corals from both Terrace 2 (two localities) and Terrace 1 (one locality). One of their localities on Terrace 2 is close to the main locality studied by Kanakoff and Emerson (1959), and three analyses of one Paracyathus pedroensis coral colony gave ages of ∼124–120 ka, with minimal likely age bias, permitting correlation to MIS 5e. Three Balanophyllia elegans samples from the same terrace at another locality gave older apparent ages but with clear evidence of an open system history. A single Paracyathus pedroensis coral colony from Terrace 1 gave an apparent age of ∼106 ka, allowing correlation to MIS 5c. At least two of the localities correlated to MIS 5e by Wehmiller et al. (1977b) are on what Grant et al. (1999) later mapped as Terrace 1 and correlated to MIS 5c based on their U-series age from this terrace, highlighting the need for additional study of these terraces.
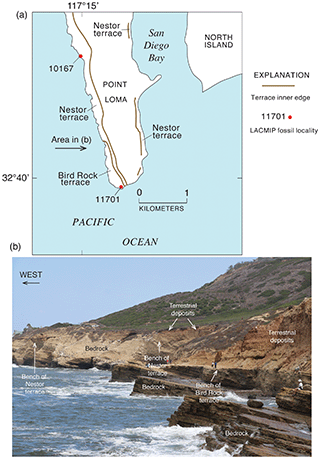
Figure 29(a) Map showing marine terrace inner edges (redrawn from Kern, 1977) and LACMIP fossil localities where U-series ages are reported by Muhs et al. (1994, 2002a); corals from the Nestor terrace date to ∼120 ka (MIS 5e) and ∼100 ka (MIS 5c); those from the Bird Rock terrace date to ∼80 ka (MIS 5a). (b) Photograph showing outer edges of the Nestor and Bird Rock terraces on the west coast of Point Loma, looking north (see location in a). Photograph by Daniel R. Muhs.
4.6.5 San Diego County
In the San Diego area (RSL IDs 3694 to 3701, 3785, 3822), multiple marine terraces have been documented (Kern and Rockwell, 1992). The lowest two terraces, mapped by Kern (1977), have received the most attention. These are the Bird Rock terrace (shoreline angle elevation of ∼8 m) and the Nestor terrace (shoreline angle elevation of ∼23 m), best exposed along the west coast of Point Loma (Fig. 29). Both terraces host deposits and fossils that are thought to represent high-energy, rocky intertidal environments (Kern, 1977). A more quiet-water, “bay” fauna characterizes what is called the Bay Point Formation at somewhat more protected localities. This formation is considered to be correlative to deposits of the Nestor terrace (Valentine, 1959; Kern, 1971). Ku and Kern (1974) reported three alpha-spectrometric U-series ages of corals from the Nestor terrace (∼109, ∼ 131, and ∼124 ka). Of these, the age of ∼109 ka is the only analysis that yielded an initial value within the range of modern seawater. Nevertheless, an “average” age of ∼121 ka (correlated to MIS 5e) for the Nestor terrace has been assumed by many subsequent investigators who have used this terrace as a calibration point for amino acid geochronology (Wehmiller et al., 1977b; Wehmiller and Belknap, 1978; Wehmiller and Emerson, 1980; Emerson et al., 1981; Kennedy et al., 1982; Wehmiller, 1982; Keenan et al., 1987). Stein et al. (1991) reported somewhat older U-series ages of individual corals, analyzed by TIMS, ranging from ∼145 to ∼133 ka, and offered the possibility that the Nestor terrace was not cut during the MIS 5e high-sea stand. The same investigators also reported an age of ∼97 ka for the Bird Rock terrace. Muhs et al. (1994) redated corals from both the Nestor and Bird Rock terraces using alpha-spectrometric U-series analyses and reported ages of 126±6 ka and 85±4 ka, respectively.
In some amino acid studies that have used the Nestor terrace fossils as calibration points (Wehmiller et al., 1977b; Kennedy et al., 1982), the fauna has been reported to be one characterized by warm-water forms, although no taxa are specifically mentioned. Using the detailed fauna presented by Valentine and Meade (1961) and Kern (1977), however, Muhs et al. (2002a) challenged the idea that the Nestor terrace hosts predominantly warm-water species. Although some extralimital southern forms are present, there are a larger number of northward-ranging species. Muhs et al. (2002a) also reported new TIMS U-series analyses of individual corals from deposits of the Nestor terrace. Nine of these have ages ranging from ∼128 to ∼113 ka, but three corals have ages ranging from ∼109 to ∼98 ka, similar to what was reported for Cayucos, California, as discussed earlier. These investigators interpreted the results from both localities to indicate that the deposits at Cayucos and on the Nestor terrace contain fossils representing MIS 5e (with warm-water mollusks) and MIS 5c (with cool-water mollusks).
Elsewhere in the San Diego area, at Torrey Pines State Park (Fig. 25), and near the Mexican border, Wehmiller et al. (1977b) and Kennedy et al. (1982) correlated low-elevation marine terrace deposits to the Nestor terrace using amino acid geochronology. These investigators correlated the Torrey Pines and “border locality” deposits with MIS 5e based on both amino acid ratios and reports of faunas with warm-water aspects (Emerson and Addicott, 1953; Valentine, 1960b). There have been, however, no U-series or amino acid studies of the quiet-water Bay Point Formation fossils, so assumed correlations to MIS 5e for these deposits remain hypothetical.
4.6.6 Channel Islands
The eight islands off the coast of southern California are called the Channel Islands because of the proximity of the northern chain of four islands to Santa Barbara Channel (Fig. 25). Of the eight islands, all but Santa Catalina Island are characterized by geomorphically well expressed marine terraces. In addition, some of the most fossiliferous and best-preserved terraces along the entire coast of North America are found on the Channel Islands. Five islands are preserved either in Channel Islands National Park or by The Nature Conservancy, and two (San Nicolas Island and San Clemente Island) are owned by the U.S. Navy. Thus, the urban development that has obscured much of the marine terrace geomorphology on mainland California is absent on the islands. A total of 14 terraces have been mapped on San Nicolas Island (Vedder and Norris, 1963) to an elevation of ∼240 m, and San Clemente Island hosts at least 20 terraces, the highest at an elevation of almost 600 m. Even tiny Santa Barbara Island, which has an area of less than 3 km2, hosts at least five marine terraces (Muhs and Groves, 2018).
The majority of work on last interglacial marine terrace records has been done on the southern islands. Muhs et al. (1994) reported alpha-spectrometric ages of the lowest marine terraces on San Clemente Island (RSL IDs 3775 and 3800) and San Nicolas Island (RSL IDs 3774 and 3813 to 3817). Their study showed that the second emergent terraces on both islands have deposits hosting fossils that likely date to MIS 5e, and the first terrace on San Nicolas Island dates to ∼80 ka, or MIS 5a. Later, higher precision TIMS U-series analyses confirmed these ages for both San Clemente Island and San Nicolas Island (Muhs et al., 2002a, 2006). More detailed work on San Nicolas Island, however, with both new terrace mapping and new TIMS U-series ages (Muhs et al., 2012) showed that although the first terrace is a single landform with deposits dating to ∼80 ka, the second terrace is a composite feature, with a broad, lower-elevation surface (terrace “2b”) and a narrow, higher-elevation surface (terrace “2a”). Fossils from terrace 2a date only to MIS 5e and do not contain cool-water mollusks, but fossils from terrace 2b date to both MIS 5e (∼120 ka) and MIS 5c (∼100 ka) and contain a mix of mollusks with both warm-water and cool-water aspects, similar to what was reported for Cayucos and the Nestor terrace at Point Loma. Muhs et al. (2012) interpreted these results to indicate that the MIS 5c high-sea stand was high enough and the uplift rate on San Nicolas Island was low enough that much of the MIS 5e terrace (2a) was removed by sea cliff retreat at ∼100 ka, and fossils from both the ∼120 and ∼100 ka sea stands were mixed into the deposits of terrace 2b. With these new findings in mind, Muhs et al. (2014a) examined the faunal record of the MIS 5e terrace on San Clemente Island, which is also a composite landform (i.e., two platforms, 2a and 2b, as on San Nicolas Island). This investigation showed that the “MIS 5e” terrace deposits on this island also contain a mix of both warm-water and cool-water fossils, chiefly mollusks, which also implies a mix of MIS 5e (warm) and MIS 5c (cool) taxa. This interpretation also explains a previously enigmatic molluscan oxygen isotope record, implying cooler waters in what had been thought to be solely ∼120 ka deposits (Muhs and Kyser, 1987).
The largest of the northern Channel Islands (San Miguel, Santa Rosa, and Santa Cruz) also have marine terraces that date to MIS 5e. TIMS U-series analyses give ages of ∼ 120 ka for the second emergent terraces (shoreline angle elevations of ∼20–24 m) on San Miguel (RSL ID 3778) and Santa Rosa (RSL IDs 3779 to 3782) islands (Muhs et al., 2014c). As is the case on San Nicolas and San Clemente islands, the fossil faunas from the deposits of these terraces host both warm-water and cool-water taxa (Orr, 1960; Muhs et al., 2014c), although currently there is only sparse geomorphic evidence of two high sea stands (i.e., both MIS 5e and 5c). At one locality on Santa Rosa Island, there is a marine terrace with an outer edge at ∼7 m above sea level with an uncertain shoreline angle elevation as the inner part of the terrace is covered by eolian sand. Apparent TIMS U-series ages of corals from this terrace range from ∼113 to ∼110 ka, and all have slightly elevated initial values, indicating at least some bias to older ages (Muhs et al., 2015). Further, mollusks from this terrace (SRI-1 in Fig. 12) have lower amino acid ratios than those in the 24 m high terrace (SRI-5F in Fig. 12) dated to ∼120 ka elsewhere on the island. Thus, it is possible that this isolated terrace fragment represents an MIS 5c record, but more work is needed to confirm this. On both islands, there is a lower-elevation terrace with a shoreline angle elevation of ∼3 m (San Miguel Island) and ∼7 m (Santa Rosa Island). TIMS U-series analyses give an age of ∼80 ka for this terrace on Santa Rosa Island, and amino acid ratios in mollusks indicate a similar age for the 3 m high terrace on San Miguel Island (Muhs et al., 2015, 2018). The lowest-elevation terrace on Santa Cruz Island (RSL IDs 3789, 3790, 3811, 3812) has shoreline angle elevations ranging from ∼6 to ∼17 m, and both U-series ages of corals and amino acid ratios in mollusks indicate that it dates to MIS 5e (Pinter et al., 1998; Muhs and Groves, 2018). Thus far, there is no evidence of terraces dating to MIS 5c or 5a on Santa Cruz Island, suggesting that the long-term uplift rate on this island is relatively low.
The two smallest of the Channel Islands, Anacapa Island (RSL ID 3791) and Santa Barbara Island (RSL IDs 3792, 3793), both have low-elevation terraces with shoreline angle elevations of ∼10–11 m above sea level. No coral ages are yet available for these terraces, but amino acid ratios indicate a mixed population of mollusk ages, correlated to MIS 5e and either MIS 5c or MIS 5a (Fig. 12). The terrace fauna on Santa Barbara Island is diverse, with a large number of warm-water forms and a smaller number of cool-water forms (Lipps et al., 1968; Muhs and Groves, 2018). The much more sparse fauna on Anacapa Island hosts some warm-water forms but only one northward-ranging species.
Santa Catalina Island's marine terrace record, or to put it more accurately, its apparent lack of a record, has been an enigma for decades (see Smith, 1933, for one of the earliest discussions). The island is situated between crustal blocks to the north (Palos Verdes Hills) and south (San Clemente Island) that both host abundant marine terraces. Bedrock is not a limiting factor because the Catalina Schist that characterizes much of the island is similar to that of the Franciscan rocks that host marine terraces elsewhere in central and northern California (e.g., Cayucos, Fig. 6). In support of this, Emery (1958) showed that submarine terraces are found off Santa Catalina Island. At a minimum, even with no uplift, with the likelihood of a higher than present sea level during MIS 5e, one should expect to see some evidence of a terrace that dates to ∼120 ka. One hypothesis that has been offered is that the island is subsiding and that the terrace record is largely submerged (Castillo et al., 2018). However, there is no a priori reason to suppose that in a tectonic setting similar to those elsewhere in southern California that Santa Catalina Island should be subsiding when all adjacent areas are uplifting. Schumann et al. (2012) presented evidence that in fact the opposite is true, i.e., Santa Catalina Island is experiencing uplift, possibly at a high enough rate that fluvial erosion has removed most evidence of any terraces. This remains the most viable explanation to date, but more work is needed to confirm this.
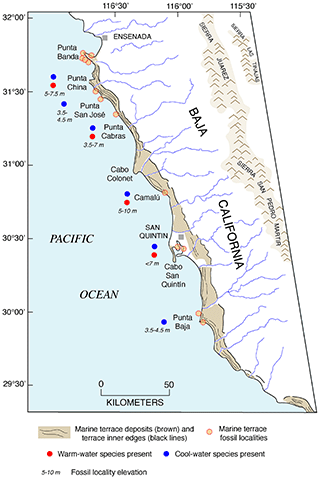
Figure 30Map of northwestern Baja California, showing marine terrace deposits (brown shades), terrace inner edges (black lines), and fossil localities (red–orange circles). Punta Banda fossil localities are shown in Fig. 31. South of Punta Banda, most fossil localities are undated, but based on elevations shown here, many likely date to some part of MIS 5 and could contain mixes of fossils of two ages, with cool-water (blue dots) and warm-water (red dots) faunas (see text for discussion). Marine terrace deposits and inner edge mapping redrawn from Orme (1980).
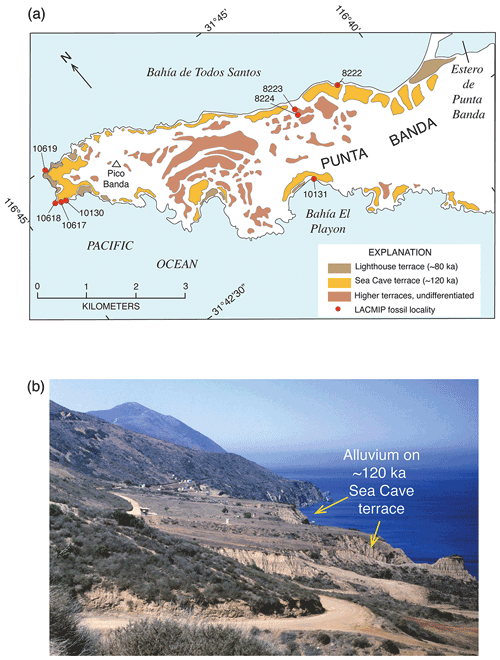
Figure 31(a) Map of the Punta Banda area, south of Ensenada, Baja California (see Fig. 30 for location). Lighthouse terrace is dated to MIS 5a (∼80 ka), and Sea Cave terrace is dated to MIS 5e (∼120 ka), both by U-series on corals (Rockwell et al., 1989; Muhs et al., 2002a). Terrace mapping is redrawn from Rockwell et al. (1989). (b) Photograph of the ∼120 ka Sea Cave terrace on the northeast side of Punta Banda, showing thick alluvial cover. Photograph by Daniel R. Muhs.
4.7 Baja California, Pacific coast
South of the city of Ensenada, Baja California, there is a prominent peninsula called Punta Banda (Fig. 30). Rockwell et al. (1989) mapped several marine terraces on this peninsula and provided alpha-spectrometric U-series ages of corals and hydrocorals from the lowest terraces. The third terrace, called the Sea Cave terrace (RSL IDs 3786, 3802), has a shoreline angle elevation that varies between ∼34 and ∼40 m (Fig. 31). The first terrace, called the Lighthouse terrace, has a shoreline angle elevation that varies between ∼15 and ∼18 m. An intermediate, unnamed terrace at ∼22 m elevation occurs only in a small area on the outer part of the peninsula. U-series ages indicate that the Sea Cave terrace is ∼120 ka (MIS 5e) and that the Lighthouse terrace is ∼80 ka (MIS 5a). The intermediate terrace at ∼22 m could represent MIS 5c, but no corals were found on the terrace. Muhs et al. (2002a) reported new, TIMS U-series analyses of corals from both the Sea Cave and Lighthouse terraces that confirm the earlier ages generated by alpha spectrometry. Many of the corals from Punta Banda show minimal age bias, based on back-calculated initial values (Fig. 10c). Corals from the Sea Cave terrace that show mostly closed-system histories have ages ranging from ∼124 to ∼118 ka, and those from the Lighthouse terrace range from ∼83 to ∼80 ka.
Isla Guadalupe is situated ∼260 km southwest of the Pacific coast of Baja California (Fig. 2). The island is attractive as a reference locality for estimating paleo-sea level during the last interglacial period because it is one of the few localities adjacent to North America that can be considered, a priori, to be tectonically stable. The island is distant from any plate boundary, has no active faults nearby, has no active volcanoes on it or near it, is bounded on its eastern side by a seafloor with undisturbed marine sediment, and has no history of recent earthquakes (Gonzalez-Garcia et al., 2003). Lindberg et al. (1980) reported that emergent marine deposits are found on the southern and eastern coasts of the island. These deposits have elevations of ∼1 to ∼8 m above sea level, with most localities described as ∼1 to ∼6 m above sea level (RSL IDs 3803 to 3805). From these deposits, Muhs et al. (2002a) reported ages of ∼123 to ∼118 ka for Pocillopora corals, and most show closed-system histories. An extensive faunal list by Lindberg et al. (1980) indicates that the ∼120 ka deposits host a large number of extralimital southern species of mollusks. In addition, Isla Guadalupe marks, thus far, the northernmost occurrence of hermatypic corals along the Pacific coast of North America during MIS 5e (Durham, 1980).
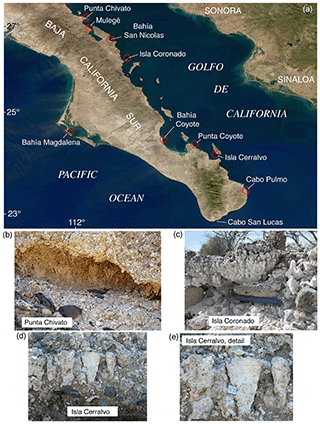
Figure 32(a) Satellite image of southernmost Baja California Sur and parts of Sonora and Sinaloa, acquired on 27 November 2011 using the MODIS instrument on the Aqua satellite (courtesy of the NASA Rapid Response Team). Red circles indicate marine terrace localities where U-series ages on corals have yielded MIS 5e ages (see Table S1). (b–e) Photographs of growth-position corals dated to MIS 5e from Punta Chivato, Isla Coronado, and Isla Cerralvo (see Johnson, 2002; Johnson et al., 2007; Tierney and Johnson, 2012). All coral photographs are courtesy of Markes Johnson, Williams College.
South of Punta Banda, marine terraces are prominent landforms all along the coast of Baja California. In a coastal reach from Punta Banda south for at least 300 km, multiple marine terraces are present, mapped by Orme (1980) (Fig. 30). Many low-elevation marine terrace deposits along this reach of coast are highly fossiliferous (Emerson, 1956, 1960; Emerson and Addicott, 1958; Addicott and Emerson, 1959; Valentine, 1960a, 1961) and are candidates for records as MIS 5e shorelines. Unfortunately, little work has been done on age determinations for most of these terraces. What geochronologic work has been done along the Pacific coast of both Baja California and Baja California Sur is aminostratigraphic correlation, using U-series-dated localities, such as the Nestor terrace to the north, and a single locality to the south, Bahía Magdalena (see Wehmiller and Emerson, 1980). Thus, before reviewing the results of the aminostratigraphic correlations, the work done at Bahía Magdalena is discussed first.
Bahía Magdalena (RSL IDs 3707, 3798) is situated on the Pacific side of Baja California Sur (Fig. 32), and marine deposits there have long been famous for their extensive Pleistocene fauna (Jordan, 1936). Near the village of Puerto Magdalena on the peninsula, one of Jordan's (1936) fossil sites (California Academy of Sciences, CAS, locality 754) contains fragments of the colonial coral Porites californica (now considered to be P. panamensis). These corals occur in marine terrace deposits that have a maximum elevation of ∼6 m and have been dated to ∼118 to ∼116 ka by alpha-spectrometric uranium-series methods (Omura et al., 1979). When the data of Omura et al. (1979) are recalculated using the more recent estimates of half-lives (Cheng et al., 2013), the coral ages from Bahía Magdalena are ∼114.8 to ∼114.0 ka.
The northernmost locality on the Pacific coast of Baja California that has been examined for paleontology and aminostratigraphic correlation is a low-elevation (outer edge elevation of ∼5 to ∼10 m) terrace at Camalú (RSL ID 3702, Fig. 30), studied by Valentine (1980). Amino acid ratios in Leukoma shells from the deposits of this terrace led Valentine (1980) to conclude that the terrace dates to MIS 5e. It is important to note here that although Valentine (1980) did not report actual amino acid ratios for Camalú, his interpretation is supported by data presented graphically by Keenan et al. (1987). Faunal data make an MIS 5e interpretation for the terrace at Camalú complicated, however, as there are several northward-ranging species of mollusks and only one southward-ranging species (Valentine, 1980). Approximately 300 km south of Camalú, at Punta Santa Rosalíllíta (RSL ID 3962), Woods (1980) mapped three emergent marine terraces, named Tomatal (shoreline angle of ∼7 m), Andres (∼25–30 m), and Aeropuerto (∼50–60 m). Although there are no U-series ages on corals from these terraces, Woods (1980) reported amino acid data from fossil mollusks that correlate the Tomatal terrace with the peak of the LIG at ∼120 ka. As is the case at Camalú, data presented graphically by Keenan et al. (1987) support this interpretation. Amino acid data from these localities are also reported in Wehmiller and Pellerito (2015).
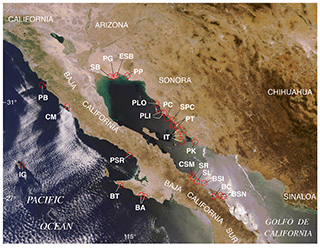
Figure 33Satellite image of northwestern Mexico (Baja California, Baja California Sur, Sonora, and parts of adjacent areas) showing localities (red circles) where amino acid geochronology of fossil mollusks has yielded MIS 5e ages (Ortlieb, 1987, 1991; Valentine, 1980; Woods, 1980; Emerson et al., 1981; Keenan et al., 1987). Also shown for reference are two U-series-dated MIS coral localities: Punta Banda (PB) and Isla Guadalupe (IG). Image acquired 28 May 2006 by the Medium Resolution Imaging Spectrometer (MERIS) on board the Envisat satellite, courtesy of the European Space Agency. Abbreviations of amino acid localities (see Table S2): CM: Camalú; PSR: Punta Santa Rosalíllíta; BT: Bahía Tortuga; BA: Bahía Asunción; BSN: Bahía San Nicolas North; BC: Bahía Concepción; BSI: Bahía Santa Inés; SL: San Lucas; SR: Santa Rosalia; CSM: Caleta Santa Maria; SB: Salina la Borrascosa; PG: Punta Gorda; ESB: east of Salina la Borrascosa; PLO: Puerto Lobos; PLI: Puerto Libertad; PC: Punta Cuevas; SPC: southeast of Punta Cuevas; PT: Punta Tepopa; IT: Isla Tiburón; PK: Punta Kino.
Farther south on the Pacific coast of northern Baja California Sur, at Bahía Tortugas and Bahía Asunción (Fig. 33), Emerson et al. (1981) and Keenan et al. (1987) reported amino acid data for low-elevation terraces that they correlated to MIS 5e. Two terraces are present at Bahía Tortugas (RSL IDs 3703, 3704), one at ∼27–24 m and the other at ∼12 m, although it is not clear if these elevations refer to shoreline angles or simply the fossil localities that were studied. In any case, amino acid ratios clearly distinguish the two terrace deposits, with the higher-elevation terrace attributed to MIS 5e, based on aminostratigraphic correlation between Bahía Magdalena to the south and the Nestor terrace to the north. The lower terrace, with lower ratios, is considered to be “∼95 ka”, but correlation to either MIS 5c or MIS 5a is possible. Deposits of both terraces contain both warm-water and cool-water species, but the upper terrace contains substantially more warm-water forms than the lower terrace.
At Bahía Asunción (RSL IDs 3705, 3706), Keenan et al. (1987) identified three age groups of marine terrace deposits based on amino acid ratios. The youngest of these is correlated with MIS 5e, based on aminostratigraphic correlation to Bahía Magdalena. The deposits correlated to MIS 5e are situated ∼6 m above sea level at one locality and ∼11–12 m above sea level at another locality. However, deposits hosting what these investigators considered to be older deposits, based on amino acid ratios, have elevations that fall within the same general range as those correlated to MIS 5e, so additional work in this area is warranted to clarify the age–elevation relations.
4.8 Golfo de California coasts of Baja California, Baja California Sur, and Sonora, Mexico
As discussed above, waters in the Golfo de California are distinctly warmer than those in the Pacific Ocean along the west coast of Baja California and Baja California Sur (Mitchell et al., 2002). Thus, the potential for finding coral-bearing marine deposits or even true coral reefs is greater in this region than on the outer coast of Baja California and Baja California Sur. Cabo Pulmo is located in the southernmost part of Baja California Sur (Fig. 32), adjacent to the Golfo de California. Ortlieb (1987) mapped emergent marine terraces near Cabo Pulmo, as well as to the southwest towards Cabo San Lucas and to the north. Squires (1959) described a coral-bearing marine terrace deposit near Cabo Pulmo (RSL ID 3806) which Ortlieb (1987) reported as having a shoreline angle elevation of ∼6 m. Muhs et al. (2002a) reported three TIMS U-series analyses of Porites and Pocillopora corals from this deposit. The Pocillopora colony gave an apparent age of ∼140 ka but is clearly biased old, based on an elevated initial value. On the other hand, Porites corals from these deposits gave ages of ∼127 and ∼120 ka and have only slightly elevated initial values. Thus, it is clear that this deposit represents MIS 5e.
North of Cabo Pulmo, Isla Cerralvo (RSL ID 3832) is situated off the eastern coast of Baja California Sur. Tierney and Johnson (2012) studied a section 8.7 m thick on the southwestern tip of the island, composed of alternating layers of growth-position corals (Fig. 32) and cobbles, the latter interpreted to be from storm transport. Five coral–cobble cycles are represented by the layers in this section, all interpreted to represent a single interglacial period. Tierney and Johnson (2012) report a U-series age of ∼126 ka from one of the Porites coral colonies found in the section. The highest growth-position reef layer is at an elevation of ∼3.9 m, overlain by sands interpreted to be from a prograding beach, up to an elevation of 7.1 m. Coral-bearing sediments (interpreted here to be from storm deposits) occur as high as ∼8.7 m above sea level.
Marine terrace deposits are exposed on both sides of Punta Coyote (RSL IDs 3828 to 3831), Baja California Sur (Fig. 32). U-series analyses, done by alpha spectrometry, have been conducted on both Porites and Pocillopora corals recovered in low-elevation terrace deposits here, reported by Sirkin et al. (1990) and Szabo et al. (1990). Apparent ages of corals from each of five localities would allow correlation of the deposits to MIS 5e, but one coral has a back-calculated initial value that is higher than modern seawater, and three have initial values that are lower than modern seawater, an unusual situation; how this affects apparent ages is not known. Based on what general information is given, it appears that these deposits have inner edge elevations that may be on the order of ∼8 to ∼10 m above sea level. Farther north, at Bahía Coyote (RSL IDs 3826 and 3827), DeDiego-Forbis et al. (2004) reported U-series ages of Porites corals from terraces exposed along the coast. The highest elevations of what appear to be growth-position Porites colonies are estimated to be ∼18 to ∼22 m above modern sea level. At least four of the corals analyzed appear to have experienced gain of bulk U, but two corals analyzed have acceptable U contents and apparent ages of ∼138 ka. Because both of these corals have initial values that are higher than modern seawater, both are biased old by some amount but permit correlation of the terraces to MIS 5e.
Studies by Johnson (2002) and Johnson et al. (2007) provide data on last interglacial coral reefs at two localities in Baja California Sur, Isla Coronado, and Punta Chivato (Fig. 32a–c). TIMS U-series analyses were conducted on these corals in laboratories of the U.S. Geological Survey, and complete analytical data are given in Muhs et al. (2014c). At Isla Coronado (RSL ID 3818), Johnson et al. (2007) report that a Porites panamensis colony formed one of the largest fossil structures yet reported in the Golfo de California. The top of the coral reef surface is ∼12 m above sea level. Analysis of coral from this reef gave an age of ∼127 ka, with an initial value higher than modern seawater. Thus, the age is likely biased old by some amount but still allows correlation to MIS 5e. At Punta Chivato (RSL ID 3819), a Porites panamensis colony ∼15 cm high, in growth position (Fig. 32), is situated on Pleistocene river gravels at a present elevation of 7.5 to 10 m above sea level (Johnson, 2002; Johnson et al., 2007). U-series analysis of a Porites sample from this colony gave an age of 117.7 ka, with an initial value indistinguishable from modern seawater, giving a high degree of confidence that this deposit correlates with MIS 5e. Just south of Punta Chivato, a low-elevation marine terrace is present along the coast near Mulegé (RSL IDs 3823 and 3824, Fig. 32). Rather than a constructional coral reef, this landform appears to be a California-style marine terrace, with a wave-cut platform and overlying deposits that contain corals. The terrace has a shoreline angle elevation of ∼12 m above sea level, and two alpha-spectrometric U-series analyses gave ages of ∼146 and ∼124 ka, with initial values only slightly higher than modern seawater (Ashby et al., 1987). Both corals have somewhat lower than optimum values, suggesting the possibility of some inherited 230Th, which would bias the apparent ages older. Nevertheless, it is likely that the terrace correlates with MIS 5e, as concluded by Ashby et al. (1987). Between Punta Chivato and Mulegé, ∼15 km north of the latter locality, Libbey and Johnson (1997) listed an extensive (>40 species) fossil molluscan fauna from a terrace that they report can be traced to, or nearly to, Punta Chivato. Many of these taxa have modern ranges that extend from the upper part of the Golfo de California to southern Mexico, Panama, Ecuador, or Peru, indicating a likely marine paleotemperature range at least as warm as that of the present.
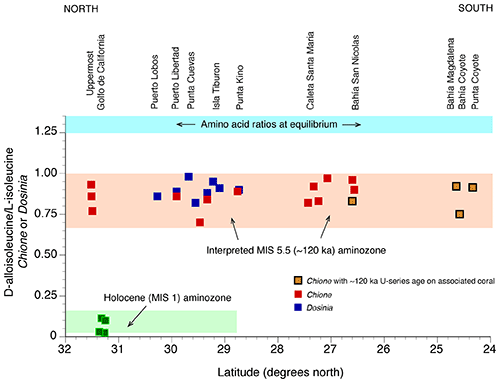
Figure 34Plot showing ratios of D-alloisoleucine to L-isoleucine in fossil Chione (red squares) and Dosinia (blue squares) as a function of latitude in Baja California, Baja California Sur, and Sonora (localities listed in Table S2). Amino acid data from Ortlieb (1987, 1991), and Umhoefer et al. (2014). Also shown (solid green squares) are ratios of D-alloisoleucine to L-isoleucine in late Holocene, radiocarbon-dated Chione shells from the upper Golfo de California (data from Martin et al., 1996), and ratios of D-alloisoleucine to L-isoleucine (blue shade) at equilibrium (from Miller and Mangerud, 1985). Pink shade defines aminozone correlated to MIS 5e, based on calibration with U-series-dated deposits that also contain Chione shells (gold squares).
North of Punta Chivato, coral-bearing marine terrace deposits have not been reported on either coast of the Golfo de California. Nevertheless, molluscan-rich terrace deposits are common and permit the possibility of amino acid geochronology. By far the most extensive studies of these deposits are those by Ortlieb (1987, 1991). Because of the relatively high mean annual air temperatures in the Golfo de California (∼20 ∘C in the north ranging to ∼23 ∘C in the south), even amino acid geochronology becomes problematic in identifying deposits that correlate to MIS 5e. The reason for this is that many species of mollusks will have reached, or be close to, racemic equilibrium for most amino acids after ∼120 ka. Ortlieb's (1987, 1991) approach to this problem was to consider that shells (the bivalves Chione and Dosinia) that were beyond radiocarbon range but yielded amino acid ratios not yet at equilibrium (but close to it) could be interpreted to be of MIS 5e age. This method is supported by his analyses of shells from both Bahía Magdalena, on the Pacific side of Baja California Sur, and at Bahía San Nicolas (RSL IDs 3741, 3742), on the Golfo de California side of Baja California (Figs. 32, 33), where U-series ages on corals have been obtained (Omura et al., 1979; Ortlieb, 1987). His interpretations are supported by more recent amino acid data on Chione reported by Umhoefer et al. (2014), calibrated to U-series data reported by DeDiego-Forbis et al. (2004) from Bahía Coyote (Fig. 34). Also shown in this figure are D-alloisoleucine / L-isoleucine values in radiocarbon-dated Chione shells of late Holocene age from Cholla Bay, Sonora (Martin et al., 1996), and a blue-shaded band that defines the equilibrium range for D-alloisoleucine / L-isoleucine (1.25–1.35; Miller and Mangerud, 1985). When all data are considered, it is apparent that shells falling within a range of ∼0.70 to ∼1.00 can be correlated to U-series-dated 120 ka localities. This range of values is substantially lower than the equilibrium range of 1.25–1.35 but considerably higher than the range of values in Holocene shells, ∼0.02 to ∼0.12. Ortlieb's (1987, 1991) results, along with those by Umhoefer et al. (2014), show, therefore, that an MIS 5e shoreline can be traced from the uppermost Golfo de California southward along the coasts of Baja California and Sonora for at least ∼850 km. Most of the MIS 5e shorelines studied by Ortlieb (1987, 1991) have relatively low elevations, ranging from ∼2 to ∼8 m above sea level (Table S2).
4.9 Pacific coast of southern Mexico
Although corals presently flourish along the Pacific coast of southern Mexico south of the Golfo de California (Reyes-Bonilla and López-Pérez, 1998), there are only scattered reports of fossil corals as reefs or in emergent terrace deposits along this reach of coastline. Emergent marine terraces or fossil coral reefs have been reported from both mainland Mexico, south of Oaxaca (Palmer, 1928a, b; Squires, 1959) and offshore Tres Marias Islands (Fig. 7) (Hertlein and Emerson, 1959; Foose, 1962). There do not appear to have been any recent studies of these deposits, nor are any geochronological data available. Given the elevations that are described in these studies, however, as well as the fossil records, they are candidates for MIS5e shoreline records and deserve further study.
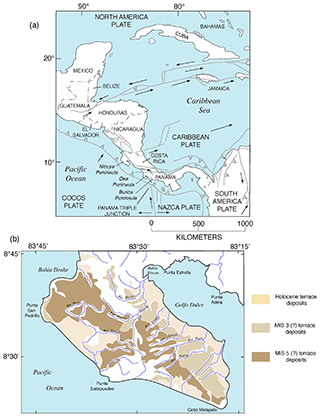
Figure 35(a) Map of Central America, showing structural features (redrawn from Mann, 2007, and Pindell and Kennan, 2009), lithospheric plates, directions of present plate movements (arrows), and localities referred to in text. (b) Map showing marine terrace deposits on the Osa Peninsula of Costa Rica (redrawn from Gardner et al., 2013); legend shows possible correlation to marine isotope stages (MIS).
4.10 Central America
The tectonic setting of the Pacific coast of Central America differs from that of Mexico to the north. Here, both the Cocos and Nazca plates are being actively subducted under either the North America plate or the Caribbean plate (Figs. 1, 35a). Furthermore, the Panama Triple Junction is situated offshore, just south of the Costa Rica–Panama border (Fig. 35a), making this area tectonically and structurally complex.
Ocean temperatures are, in principle, warm enough to support hermatypic coral reef growth off the Pacific coast of Central America from Guatemala to Panama. Toth et al. (2017) point out, however, that between Mexico and Nicaragua, there are very few if any true coral reefs on the Pacific coast, in what is referred to as the “Central American faunal gap” (Fig. 7). These investigators hypothesize that the lack of modern coral reefs along this reach of coast may be a function of a lack of hard substrate available for larval settlement, although severe and prolonged upwelling has also been offered as a contributing factor (Glynn et al., 2017). In all likelihood, this also limits the potential for finding emergent coral reefs that date to MIS 5e as well.
Farther south, along the coasts of Costa Rica and Panama, modern coral reefs are much more common, and many developed as early as ∼7000 years ago (see summary in Toth et al., 2017). This is also an area where subduction of the Cocos plate beneath the Caribbean plate is currently active, and uplift rates are high (Gardner et al., 1992; Marshall and Anderson, 1995). Much of the work on emergent marine terraces here has been focused on the Nicoya Peninsula and Osa Peninsula of Costa Rica and the Burica Peninsula of Panama (Fig. 35a). Gardner et al. (1992) reported marine terraces of Holocene age (∼7000 to 980 years) at elevations of ∼3 to ∼9 m on the Osa Peninsula, and Marshall and Anderson (1995) report Holocene marine terraces with ages of ∼4700 to ∼500 years at elevations of ∼4 to ∼16 m on the Nicoya Peninsula, demonstrating that the uplift rate in this region of active subduction is relatively high. Fisher et al. (1998), Gardner et al. (2001), and Sak et al. (2004) all pointed out that uplift in this region is controlled primarily by roughness of the subducting plate: forearc uplift on the Caribbean plate corresponds to the position of migrating seamounts on the northeastward-moving Cocos plate.
With such high uplift rates, based on the elevations of Holocene marine terraces, any MIS 5e marine terraces on the Pacific coast of Costa Rica would have to be at relatively high elevations now. On the Nicoya Peninsula, Marshall and Anderson (1995) recognized two marine terraces. The younger of these is the suite of Holocene marine deposits, called the “Cabuya” terrace. The higher terrace, called the “Cobano” terrace, is a broad coastal mesa, situated at an average elevation of ∼180 m, and it is hypothesized to have formed during MIS 5e, although no geochronologic data are presented in support of this (Marshall and Anderson, 1995). Using Holocene uplift and rotation rates, Gardner et al. (2001) estimated the Cobano terrace to have formed between ∼200 and ∼100 ka, also permitting an interpretation of an MIS 5e age.
On the Osa Peninsula of Costa Rica, Gardner et al. (2013) mapped Quaternary marine deposits of three ages, from youngest to oldest, the Jiménez (Holocene), Tigre (MIS 3?), and Rincón (MIS 5?) members, of what they called the Marenco Formation (Fig. 35b). They reported an OSL age of 109±28 ka for deposits of the Rincón member and correlated this unit to MIS 5e. Based on marine terrace shell radiocarbon ages, the Jiménez member dates to the Holocene. Gardner et al. (2013) also dated shells from the Tigre member, which, when calibrated, range from ∼31 to ∼48 ka with a few samples yielding apparently infinite ages. They correlated the Tigre member with MIS 3.
Gardner et al.'s (2013) correlation of the Tigre member to MIS 3, based on their radiocarbon ages, requires some scrutiny. A critical examination applies to similar radiocarbon ages reported by Gardner et al. (1992) and Sak et al. (2004), also on the Osa Peninsula, as do ages reported by Morell et al. (2011) for terrace shells on the Burica Peninsula of Panama (Fig. 35a). Indeed, some of the marine terrace radiocarbon ages reported by Morell et al. (2011) date not only to MIS 3 but actually give apparent ages dating to the late last glacial period (MIS 2), at a time when sea level was several tens of meters below present.
Emergent marine deposit shells giving apparent radiocarbon ages of MIS 3 age have been reported on coastlines in various parts of the globe for decades, with some investigators claiming that such ages require a paleo-sea level close to, or even above, present sea level during this interstadial period. Periodically, there have been critiques of such claims (Thom, 1973; Bloom, 1983; Colman et al., 1989), but many investigators continue to regard shell radiocarbon ages of ∼30 to ∼45 ka as truly finite and accurate. The problem is that modern carbon is nearly everywhere and has considerable mobility. This means that old shells are notorious for incorporating at least small amounts of modern carbon. Thus, even very small amounts of modern carbon can make an infinitely old shell yield an apparently “finite” radiocarbon age (Pigati et al., 2007). An 80 ka sample, for example, with a very small amount of modern carbon, can easily yield an apparent radiocarbon age of ∼40 to ∼45 ka. More work needs to be done on the ages of the older marine terrace fossils of both the Osa Peninsula of Costa Rica and the Burica Peninsula before any inferences about terraces of either MIS 3 or MIS 5 age can be made.
One of the issues that has been actively debated in the past few decades is whether MIS 5e was characterized by a single sea-level high stand or multiple high stands. Some of the original evidence for more than one high stand came from the Huon Peninsula of New Guinea, where reefs VIIa and VIIb were interpreted to represent early and later high-sea stands of MIS 5e, respectively (Bloom et al., 1974; Chappell, 1974). U-series dating of corals from these two terraces using TIMS methods confirmed that both terraces likely date to MIS 5e (Stein et al., 1993). Such a record on New Guinea does not, however, require that this was a global phenomenon because coseismic uplift has been well documented for this coast, with as many as six coral reefs emerging in the Holocene alone (Ota et al., 1993). On Barbados, the uplift rate is much lower than that on New Guinea, but more than one high stand of sea during MIS 5e has been proposed here as well (Schellmann and Radtke, 2004; Thompson and Goldstein, 2005). Unlike New Guinea, however, multiple Holocene terraces have not been reported on Barbados, and coseismic uplift is a less likely explanation for possible multiple LIG terraces.
In addition to tectonically active coastlines, there have been claims of multiple sea stands during MIS 5e from deep-sea records and reefs on tectonically stable coastlines. Rohling et al. (2008), studying the oxygen isotope record in planktonic foraminifera recovered from Red Sea sediment cores, suggested that there could have been as many as four separate high stands of sea during MIS 5e. Thompson et al. (2011) reported TIMS U-series ages of corals from San Salvador Island and Great Inagua Island in the Bahamas, proposing at least two high stands during MIS 5e and possibly as many as four high stands, similar to the Red Sea record of Rohling et al. (2008).
Modeling efforts have also addressed the question of a dual high-sea stand during the LIG. Kopp et al. (2009) conducted a statistical analysis of a database generated from many reported MIS 5e deposits worldwide from both tectonically active and stable coastlines. These investigators concluded that early within MIS 5e there was a sea-level high, followed by a drop of ∼4 m, succeeded by another sea-level high. Unfortunately, some of the hypothesized MIS 5e sites used by Kopp et al. (2009) are either poorly dated or not dated at all, rendering this reconstruction uncertain. In a more recent review of both field and modeling evidence, Barlow et al. (2018) concluded that there is no evidence of more than one high-sea stand during MIS 5e. Along the Pacific coast of North America, there has been, thus far, no evidence of more than one high-sea stand during MIS 5e along either tectonically stable or uplifting coasts.
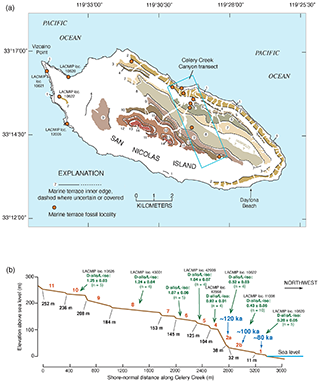
Figure 36(a) Map of marine terraces on San Nicolas Island, California (from Vedder and Norris, 1963; Muhs et al., 2012); orange dots are fossil localities. (b) Topographic profile across the lowest 11 terraces in the Celery Creek Canyon area (blue-boxed area in a), showing terrace numbers, shoreline angle elevations (from Muhs et al., 2018), and (green lettering) ratios of the amino acids D-alloisoleucine to L-isoleucine in the fossil gastropod Tegula (data mostly from Muhs, 1985). Assuming a constant rate of uplift, terrace 4 could be dated to ∼390 ka (∼ MIS 11). Note that the large jump in elevation between terraces 2a and 4 is mirrored by a substantial increase in amino acid ratios, indicating the likelihood that terraces representing MIS 9 (∼300 ka) and MIS 7 (∼200 ka) were removed by sea cliff retreat during MIS 5e.
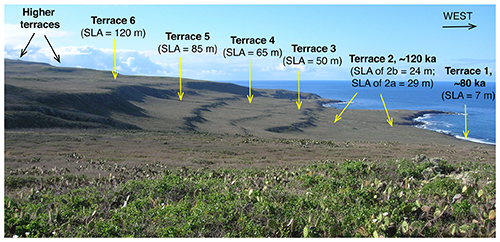
Figure 37View of the lowest six marine terraces on the west coast of San Clemente Island, California, looking south. Terrace 2b has been dated to MIS 5e (∼120 ka) by TIMS U-series methods on coral (Muhs et al., 2002a); terrace 1 is estimated to be dated to ∼80 ka (MIS 5a) based on amino acid ratios in mollusks (Muhs, 1983). Terrace 6 could be as old as ∼600 ka or more, if the rate of uplift has been constant over time. SLA: shoreline angle elevation, in meters above modern sea level. Photograph by Daniel R. Muhs.
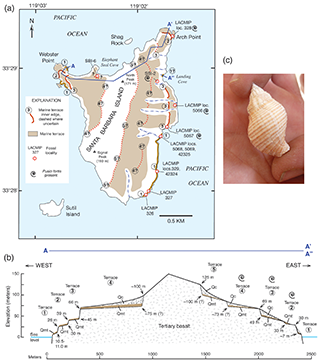
Figure 38Use of an extinct species as a biostratigraphic marker. (a) Map of marine terraces and fossil localities on Santa Barbara Island, California (from Muhs and Groves, 2018), along with (b) topographic profile of terraces (location shown in a). Also shown in both (a) and (b) are terrace deposits that host the extinct fossil gastropod Pusio fortis (formerly Calicantharus fortis), shown in (c). Note that in (a) and (b), this taxon is not found on terrace 1, whose deposits contain a mix of fossils dating to MIS 5e and 5c, but the species is found on higher terraces, indicating that it likely became extinct before MIS 5e. Photograph in (c) is from 69 m high terrace deposits on San Miguel Island and is by Daniel R. Muhs.
6.1 Interglacials prior to MIS 5e
Because of ongoing tectonic processes during the Quaternary, multiple marine terraces are recorded along much of the Pacific coast of North America from southern Oregon to Baja California. As noted earlier, Woodring et al. (1946) mapped 13 marine terraces in the Palos Verdes Hills (Fig. 26), the highest of which is at an elevation of ∼400 m. Vedder et al. (1957, 1975) and Grant et al. (1999) documented at least six marine terraces above the Newport Mesa terrace, correlated to MIS 5e, in the Newport Beach area. Vedder and Norris (1963) mapped 14 marine terraces on San Nicolas Island (Fig. 36a), with the highest at an elevation of ∼270 m. Fossils are found in deposits of all 14 terraces. On this island, amino acid ratios in fossil Tegula specimens show a steady increase with terrace elevation (Fig. 36b). By the time the 8th and 10th terraces are reached, D-alloisoleucine L-isoleucine values in Tegula are at equilibrium values of ∼1.25, indicating considerable antiquity. San Clemente Island hosts more than 20 marine terraces, and these landforms show superb geomorphic preservation (Fig. 37). Fossil-bearing marine terrace deposits are found as high as ∼265 m (Cockerell, 1939), similar to San Nicolas Island, and the highest marine terrace is found at an elevation of nearly 600 m. If the late Quaternary uplift rate has been steady over the history of the island (Muhs et al., 2014a), the highest terrace on San Clemente Island could be dated to ∼3 Ma. Even some of the smallest islands off the California coast host a long-term history of interglacial high-sea stands superimposed on steady uplift. Santa Barbara Island has an area of only ∼2.6 km2, yet it hosts at least five marine terraces up to an elevation of ∼100 m (Fig. 38). On many of the California islands, pre-MIS 5e marine terraces are distinguished from younger terraces by the presence of the extinct fossil gastropod Pusio fortis (formerly Calicantharus fortis). For example, on Santa Barbara Island, this taxon is found in deposits of the second, third, and fourth terraces (Fig. 38) but is not found in deposits of the first terrace, which appears to contain a mix of fossils dating to MIS 5e and MIS 5c (Muhs and Groves, 2018). Multiple marine terraces are found along the Pacific coast of Mexico as well. Rockwell et al. (1989) recognized 14 marine terraces on Punta Banda, in northern Baja California, with the highest at an elevation of ∼347 m. Farther south, Orme (1980) mapped multiple marine terraces, with the highest between Cabo San Quintin and Punta Baja (Fig. 30), at an elevation of ∼300 to ∼357 m.
Unfortunately, there are few data on the possible ages of pre-MIS 5e terraces on the Pacific coast of North America. Indeed, numerical ages of terraces dating from MIS 7, 9, and 11 have yet to be confirmed for any part of the Pacific coast of the continent, although it is likely that marine terraces representing these high-sea stands are preserved. Although corals are present in deposits of several higher-elevation terraces, open-system histories have likely prevailed in many of these fossils. For example, Muhs et al. (2004) presented U-series data for corals from the 10th terrace (elevation ∼236 m) on San Nicolas Island, indicating possible ages of ∼600 to ∼450 ka. These apparent ages are, however, not consistent with the late Quaternary uplift rate, nor are they consistent with amino acid ratios at equilibrium values in fossil mollusks from this terrace (Fig. 36b). A more promising isotopic method of age determination for fossils of pre-MIS 5e terraces on the Pacific coast is Sr isotope stratigraphy, a calibrated method of geochronology. Early experiments with this method in California showed promise (Ludwig et al., 1992), and since that time, better calibration curves have been developed (Howarth and McArthur, 1997).
Latitudinal, north–south-trending aminozones parallel or subparallel to MIS 5a and MIS 5e aminozones show the potential for at least lateral correlation of older, pre-MIS 5e marine terraces. Wehmiller (1982) used such an approach on the Pacific coast of North America from southern Baja California Sur to Oregon. His data showed the possibility for marine records prior to MIS 5e, including high-sea stands associated with MIS 7, 9, 11, 13, and 15.
Another possibility for dating older terraces is the use of kinetic modeling with amino acid ratios. In this approach, a theoretical kinetic pathway is used with a calibrated amino acid ratio for shells from a deposit that is independently dated (such as by U-series on coral). Clarke and Murray-Wallace (2006) review the various mathematical expressions for different kinetic pathways. One of the most widely used methods is the parabolic kinetic model, derived from heating experiments that simulate long periods of geologic time (Mitterer and Kriausakul, 1989). When this method is applied to the terrace sequence on San Nicolas Island (Fig. 36b), using the ∼120 ka age for terrace 2a and its D-alloisoleucine L-isoleucine value of 0.52, the higher amino acid ratios for the older terraces yield apparent ages of ∼375 ka (terrace 4), ∼480 ka (terrace 5), ∼510 ka (terrace 6), and ≥680 ka for terraces 8 and 10. If these ages are correct, they would permit correlation of terrace 4 with MIS 11 and terraces 5 and 6 with MIS 13. Along the terrace transect shown in Fig. 36b, this would also imply that terraces that formed during MIS 7 and MIS 9 were likely removed by erosion during MIS 5e. Interestingly, the ages and uplift rates derived from older terraces in this exercise are similar to the uplift rate derived from the MIS 5e terrace. While all of these implied results seem reasonable geologically, it is important to remember that kinetic modeling of amino acid racemization and epimerization is still theoretical, and age estimates derived from such an approach are simply possibilities for additional testing.
Still another method to address the question of ages of older marine terrace deposits is the use of cosmogenic isotopes. In the San Diego region, one of the oldest marine terrace deposits is called the Clairemont terrace, part of a larger complex of marine terrace and beach ridge deposits called the Lindavista terrace sequence. Early data on Tivela stultorum valves from the Lindavista unit showed D L values at or near equilibrium for all amino acids (Wehmiller et al., 1977b), implying considerable antiquity. Based on data in Lajoie et al. (1991), as many as 13 terraces occur above the MIS 5e terrace, each with wave-cut benches and prominent beach ridges. The Clairemont terrace is found at an elevation of ∼96 m above sea level, and Simms et al. (2020) used cosmogenic nuclides at two localities to estimate an age of ∼1.48 Ma for this terrace.
6.2 High-sea stands after MIS 5e
Whereas ages of pre-MIS 5e marine terraces on the Pacific coast of North America are rare, there are several marine terrace ages that postdate the peak of the last interglacial period, mostly for the relatively high-sea stands of MIS 5c (∼100 ka) and MIS 5a (∼80 ka). With regard to MIS 5c, TIMS U-series ages of corals dating to this high-sea stand have been confirmed but mixed with MIS 5e deposits at Cayucos, Point Loma, and San Nicolas Island, as discussed above (Stein et al., 1993; Muhs et al., 2002a, 2012). In two other areas, both of which have somewhat higher late Quaternary uplift rates, there are terraces that are good candidates for MIS 5c records, although both are as yet undated. On the Palos Verdes Hills, what Woodring et al. (1946) mapped as the “second” and “fourth” terraces have been dated to ∼80 and ∼120 ka, respectively (Muhs et al., 2006), as noted earlier. To avoid confusion with terrace numbering that is inconsistent from this area to nearby San Pedro, Muhs et al. (2006) named these (informally) as the Paseo del Mar (second) and Gaffey (fourth) terraces. In the western part of the Palos Verdes Hills (Fig. 26), there is an intermediate-elevation terrace that Woodring et al. (1946) mapped as the “third” terrace. Because it occupies a morphostratigraphic position between the ∼80 ka (second) Paseo del Mar and ∼120 ka (fourth) Gaffey terraces, it is very likely that this terrace records the MIS 5c high-sea stand. Woodring et al. (1946) did not report any fossil localities on this terrace, and 30 years of periodic searches by the present author have not resulted in any either, so the terrace remains undated.
The other locality that provides morphostratigraphic evidence of a possible MIS 5c record is Punta Banda, in northern Baja California. At this locality (Fig. 31), Rockwell et al. (1989) reported a small terrace fragment at ∼22 m above sea level above the Lighthouse (first) terrace at ∼15 m and below the Sea Cave (third) terrace at ∼34 m. Similar to the Palos Verdes Hills, the Lighthouse and Sea Cave terraces are dated to ∼80 and ∼120 ka, respectively, by both alpha-spectrometric U-series (Rockwell et al., 1989) and TIMS U-series methods (Muhs et al., 2002a). Unfortunately, as with the Palos Verdes Hills, no corals have yet been found on the second, ∼22 m terrace on Punta Banda.
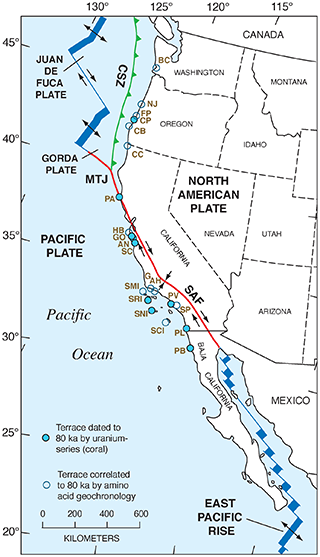
Figure 39Map of the Pacific coast of North America with structural features as shown in Fig. 2, but also plotted are localities (filled blue circles) where U-series ages of corals dating to MIS 5a (∼80 ka) have been reported and localities (open blue circles) where amino acid ratios in mollusks permit correlation to MIS 5a. Structural features are redrawn in simplified form from Drummond (1981) and Simkin et al. (2006). Abbreviations are keyed to Table S3 and are as follows: BC: Bay Center, Willapa Bay; NJ: Newport Jetty; FP: Five Mile Point; CP: Coquille Point; CB: Cape Blanco; CC: Crescent City; PA: Point Arena; HB: Half Moon Bay; GO: Green Oaks Creek; AN: Point Año Nuevo; SC: Santa Cruz; G: Gaviota; AH: Arroyo Hondo; SMI: San Miguel Island; SRI: Santa Rosa Island; SNI: San Nicolas Island; PV: Palos Verdes Hills; SP: San Pedro; SCI: San Clemente Island; PL: Point Loma; PB: Punta Banda; CSZ: Cascadia Subduction Zone; MTJ: Mendocino Triple Junction; SAF: San Andreas Fault.
While marine terraces dated to MIS 5c are rare on the Pacific coast of North America, terraces dated to MIS 5a are abundant (Fig. 39 and Table S3). Corals have been acquired and dated by TIMS U-series methods at Coquille Point (Oregon), Point Arena (northern California), three localities between Point Año Nuevo and Santa Cruz (central California), Santa Rosa and San Nicolas islands, the Palos Verdes Hills, and Point Loma (all in southern California), as well as Punta Banda (northern Baja California). Analytical and faunal data for these terraces are given in Addicott (1966), Zullo (1969), Kern (1977), Kennedy (1978), Rockwell et al. (1989), and Muhs et al. (2002a, 2006, 2012, 2015). In all cases, the faunas are characterized by cool-water forms, with several extralimital northern and northward-ranging species.
In addition to U-series-dated localities, a large number of localities lack corals but have mollusks that permit aminostratigraphic correlation to MIS 5a, following the approach pioneered by Wehmiller et al. (1977b) and Kennedy et al. (1982). These localities can be found from near Newport, Oregon, into northern California, and to the Channel Islands of southern California (Fig. 39). Like their U-series-dated counterparts, these terraces host faunas with extralimital northern or northward-ranging species of mollusks.
It is very likely that there are terraces dating to MIS 5a and/or MIS 5c in Baja California, Baja California Sur, and Sonora as well, based on amino acid and faunal studies by Emerson et al. (1981) and Ortlieb (1987). In addition, along the Pacific coast of northern Baja California, numerous fossil localities, shown earlier in Fig. 30, contain mixes of warm-water and cool-water molluscan faunas. Although none of these terraces have either U-series or aminostratigraphic data for age control (with the exception of Camalú, as noted earlier), their low elevations allow for the possibility that they record some part of MIS 5. The mixes of cool-water and warm-water mollusks invite comparison to similar mixes of faunas with contrasting thermal aspects, found at Cayucos, Point Loma, and San Nicolas Island, that have U-series ages on corals that include both MIS 5e and 5c.
Two localities in southern California have U-series and amino acid evidence for emergent marine terraces dating to MIS 3. Both localities are south of the “big bend” in the San Andreas Fault (Figs. 1, 2) where this large constraining bend brings about a shift from lateral fault movement to predominantly crustal compression between the Pacific and North America plates. The result is unusually high rates of uplift, such that terraces formed when sea level was substantially lower than present are now emergent. Isla Vista, a university community (Fig. 25), is built on a marine terrace whose outer edge is at an elevation of ∼7 m. Based on amino acid ratios in Saxidomus valves, Wehmiller et al. (1977b), Wehmiller (1982), and Kennedy et al. (1982) thought that this terrace likely postdated MIS 5a. This conclusion was supported by the fact that the fauna within the deposits of this terrace contains a large number of extralimital northern species (Wright, 1972), consistent with very cold waters off the California coast at this time. This inference is expected for the time period postdating MIS 5a, based on independent evidence (Kennett and Venz, 1995). Gurrola et al. (2014) reported U-series ages of ∼49 and ∼47 ka for corals from this terrace, which support the original age interpretations (see Muhs et al., 2014c, for isotopic data for one of these specimens). All these investigators correlated this terrace with MIS 3.
Also in southern California, there is a terrace that has been correlated to MIS 3 near a locality simply called “Sea Cliff”, northwest of Ventura (Fig. 25). Along an ∼6 km reach of coastline here, there are two marine terraces: a low-elevation surface dated to the Holocene (see discussion below) and a higher-elevation terrace of Pleistocene age above it. The Pleistocene terrace has a variable elevation in a shore-parallel sense, from just over ∼100 m to just over ∼200 m above sea level (Wehmiller et al., 1978). Although no corals have yet been found in the deposits of the Pleistocene terrace, amino acid ratios indicate that it is likely ∼50 ka, similar to the terrace at Isla Vista (Wehmiller et al., 1978; Kennedy et al., 1982; Wehmiller, 1982). The elevation of this terrace, along with its young age and formation at a time of relatively low sea level, indicates that this reach of coastline has experienced an extremely high rate of uplift.
In northern California, near the Mendocino Triple Junction of the Gorda, Pacific, and North America plates, there is a third locality with a marine terrace correlated to MIS 3 (McLaughlin et al., 1983a, b). This terrace is found near Point Delgada (Fig. 21) and has a maximum elevation of ∼7 m above sea level. Correlation of this terrace to MIS 3 is based on a radiocarbon age of ∼45 ka from fossil wood found in terrestrial deposits that overlie the marine terrace deposits. Although radiocarbon ages on wood are usually reliable, this apparent age is near or at the limit of the method and in addition is found within overlying deposits, not the marine terrace deposits themselves. Thus, the cautions discussed earlier with regard to modern carbon contamination would apply here as well. Although the age from Point Delgada is interpreted to be a close, minimum-limiting age, it is in fact just a minimum-limiting age, and the terrace itself could be older.
Based on early results of amino acid geochronology in Kennedy et al. (1982), it was originally thought that marine terrace deposits at a fourth locality, Cape Blanco, Oregon (Figs. 18–20), could correlate with MIS 3. Later amino acid studies, linked with a nearby U-series-dated, coral-bearing locality (Coquille Point, Oregon), showed that the low terrace at Cape Blanco likely dates to MIS 5a (Muhs et al., 1990). The fauna at Cape Blanco, with its cool-water species, is similar to that at Coquille Point (Muhs et al., 2006). Furthermore, oxygen isotope ratios in fossil Saxidomus gigantea and Mya truncata collected from the two localities do not have significant differences (Muhs et al., 1990).
6.3 Holocene sea-level indicators
Emergent Holocene marine deposits are found at several localities along the Pacific coast of North America. Within the southern Puget Sound area of Washington State (Fig. 16), emergent marine terraces or peat-covered tidal flats are found at five localities, as much as ∼7 m above sea level, with ages ranging between ∼1000 and 1100 yr BP (Bucknam et al., 1992). It has long been recognized (e.g., Kelsey, 1990) that there is the potential for coseismic uplift along the zone where the Juan de Fuca plate is being subducted beneath the North America plate (Fig. 2). What is interesting about the Holocene terraces in the Puget Sound area, however, is that they are some distance inland from this subduction zone. Bucknam et al. (1992) attribute Holocene uplift here to reverse slip along an inferred fault within the crust of the North America plate.
Near the Mendocino Triple Junction area of northern California (Figs. 2, 21), Holocene marine terraces have also been documented (Lajoie et al., 1991). The most recent of these produced 1.4 m of uplift associated with the MS 7.1 earthquake at Cape Mendocino in 1992 CE (Carver et al., 1994). Merritts (1996) reported that earlier Holocene, coseismic uplift events had occurred prior to the 1992 CE earthquake. Based primarily on radiocarbon ages of marine shells, at least four such events occurred between ∼7 and ∼0.6 ka.
South of the “big bend” area of the San Andreas Fault (Figs. 2, 25), crustal compression is the dominant tectonic style. Thus, in the area to the south, uplift rates are very high. Sarna-Wojcicki et al. (1987) mapped two marine terraces in this area between Ventura and Santa Barbara. The higher of the two terraces ranges in elevation from ∼120 to ∼210 m, and amino acid data in mollusks reported by Wehmiller et al. (1978) indicate that it is likely dated to ∼45 ka (MIS 3), as discussed above. The lower of the two terraces has elevations that range from ∼6 to ∼35 m. Radiocarbon ages of marine mollusks from this terrace range from ∼5 to ∼1.8 ka (Sarna-Wojcicki et al., 1987). More recent detailed work by Rockwell et al. (2016) identified four Holocene terraces in this area, with radiocarbon ages of ∼6.7, ∼4.4, ∼2.1, and ∼0.95 ka. Each terrace represents a separate coseismic uplift event.
Still farther south, the coast of Central America is adjacent to a subduction zone, where the northeast-moving Cocos plate is being subducted beneath the Caribbean plate (Fig. 35). In addition, the Panama Triple Junction is situated just south of the Costa Rica–Panama border, where the Cocos, Caribbean, and Nazca plates intersect. On the Nicoya Peninsula of Costa Rica, Marshall and Anderson (1995) reported marine terraces at elevations of ∼4 to ∼16 m above sea level with radiocarbon ages of ∼4.1 to ∼0.4 ka. Gardner et al. (2001), working the same general area, reported similar elevations and ages for two terraces, in agreement with, but adding detail to, the study of Marshall and Anderson (1995). Fisher et al. (1998) ascribed uplift in this region to subduction of seamount chains on the Cocos plate. Marine terraces of Holocene age have also been reported for the nearby Osa Peninsula of Costa Rica (Gardner et al., 2013) and the Burica Peninsula of Panama (Fig. 35) by Morell et al. (2011).
6.4 Implications for paleozoogeography
There has been considerable interest in MIS 5e not only for its implications for future sea-level rise but also for warming of the oceans. Indeed, sea-level rise is linked to ocean warming due to the possibility of thermal expansion of the world's oceans. In addition, however, possible ocean warming during MIS 5e has importance for understanding how modern marine ecosystems might respond to future warming.
Global-scale studies of MIS 5e have been carried out using proxy paleoclimate data from deep-sea cores with the goal of estimating sea surface temperatures (SSTs). Results of these investigations have not been entirely consistent. CLIMAP Project Members (1984) concluded that overall, the last interglacial ocean was not significantly different from the modern ocean. It is important to note, however, that for many regions of the world, including much of the ocean around Australia, the Mediterranean Basin, the Bering Sea, the central Pacific Ocean, and the eastern Pacific Ocean off North America, there were few cores available. Using a larger dataset, Turney and Jones (2010) concluded that MIS 5e global temperatures were on average ∼1.5 ∘C warmer than present, although part of this conclusion is based on ice and terrestrial records. In yet another compilation, McKay et al. (2011) concluded that on a global scale, SST during MIS 5e was not significantly different from the present. From this, these investigators inferred that thermal expansion likely played only a minor role, if any, in the higher than present sea level during MIS 5e. However, as the same investigators also pointed out, some regions are exceptions to this generalization.
In some regions where core data are sparse, shallow invertebrate marine fossil faunas serve as an important record of SST. For example, extralimital species of mollusks and corals, indicating warmer SST during MIS 5e, have been documented in the Indian Ocean along the western coast of Australia (Kendrick et al., 1991), around New Zealand and the southern coast of Australia (Murray-Wallace et al., 2000), along the Bering Sea and Arctic Ocean coasts of Alaska (Brigham-Grette and Hopkins, 1995), in the eastern Atlantic Ocean off Africa and the Mediterranean Basin (Cuerda, 1975, 1987, 1989; Cuerda and Sacarès, 1992; Hearty et al., 1986; Meco et al., 2002, 2006; Muhs et al., 2014b), in the western Atlantic Ocean around Bermuda (Richards et al., 1969; Muhs et al., 2002b), and in the Pacific Ocean along the shores of Oahu in the Hawaiian Islands (Kosuge, 1969; Muhs et al., 2002b; Groves, 2011). The sites studied in Australia, the central Pacific, the western Atlantic Ocean, the eastern Atlantic Ocean off Africa, the Mediterranean, and the central Pacific are all localities anchored by reliable MIS 5e U-series ages on corals.
In the context of both deep-sea core proxy climate data and shallow-water marine invertebrate records from around the globe, it is interesting to consider what the SST off the Pacific coast of North America was during MIS 5e. Herbert et al. (1998) showed that alkenone unsaturation indices, derived from modern core-top samples, correlate in a linear fashion with modern SST. Using this relation, Herbert et al. (2001) generated both oxygen isotope values in foraminifera (to identify MIS 5e) and alkenone unsaturation indices to estimate SST in five cores taken off northern California to south of Cabo San Lucas, Baja California Sur. In all cases, SST during MIS 5e is substantially higher than at present or during earlier parts of the Holocene. One of the cores examined (Ocean Drilling Project, or ODP 893) is from Santa Barbara Basin, the same locality studied for temperature-sensitive foraminiferal species by Kennett and Venz (1995). The latter workers found that MIS 5e was the only time, other than the Holocene, when warm-water foraminifera were present in Santa Barbara Basin, in good agreement with the alkenone unsaturation index data. Two other cores, one off central California and one off southern California, studied by Yamamoto et al. (2007), also gave alkenone-based SST indicating substantially warmer waters off the Pacific coast during MIS 5e compared to present.
Given these findings, it is pertinent to evaluate the shallow-water marine terrace records of mollusks and other invertebrates of MIS 5e age from the Pacific coast of North America. As noted earlier, pioneering amino acid studies by Wehmiller et al. (1977b) and Kennedy et al. (1982) considered that marine terrace deposits correlated to MIS 5a (∼ 80 ka) had cool-water faunas, and those correlated to MIS 5e (∼120 ka) had warm-water faunas. While the cool-water forms that are so prominent in terraces correlated to MIS 5a by amino acid geochronology (Kennedy et al., 1982) have been largely confirmed to indeed be ∼80 ka, based on TIMS U-series ages of corals (Muhs et al., 2002a, 2006), the GIA-related fossil mixing of ∼120 and ∼100 ka (MIS 5c) fossils into single terrace deposits complicates matters. Nevertheless, using those localities where there is good evidence for MIS 5e age fossils and assuming that the cool-water forms represent MIS 5c, the shallow-water marine invertebrate record still allows some inferences about SST during the peak of the last interglacial period. Here, examples of bivalves, gastropods, and corals are examined from dated deposits to illustrate what can be inferred about ocean temperatures during MIS 5e.
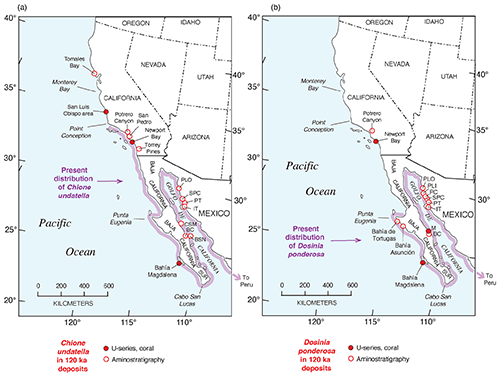
Figure 40Examples of extralimital southern species of bivalves indicating sea surface temperatures higher than present during MIS 5e (filled and open circles); also shown, in light purple shading, are the modern ranges of these taxa (from Coan and Valentich-Scott, 2012): (a) Chione undatella and (b) Dosinia ponderosa. Age and fossil data compiled from sources as follows: Jordan (1936), Valentine (1956, 1960), Kanakoff and Emerson (1959), Johnson (1962), Omura et al. (1979), Emerson (1980), Emerson et al. (1981), Kennedy et al. (1982), Ashby et al. (1987), Keenan et al. (1987), Ortlieb (1987, 1991), Grove et al. (1995), Grant et al. (1999), Kennedy (2000), and Muhs and Groves (2018).
Two species of bivalves that live dominantly in tropical waters off the Pacific coast of North America are Chione undatella and Dosinia ponderosa. C. undatella is one of two species of Chione (C. californiensis is the other) that presently lives only south of the Point Conception area (Fig. 40a). Although C. undatella is found only as far north as Goleta (near Santa Barbara), California, it ranges south along the coast of Mexico, including the Golfo de California, to Peru and is also found on the Galapagos Islands (Coan and Valentich-Scott, 2012). In fossil form, C. undatella is found in several marine terrace deposits either directly dated to MIS 5e or correlated to it on the basis of amino acids from Bahía Magdalena, Baja California Sur, north to Potrero Canyon near Los Angeles, California (Fig. 40a). In addition, however, it has also been reported from a terrace correlated to MIS 5e from near San Luis Obispo Bay by Kennedy (2000) and also has been found at Tomales Bay, California. The presence of C. undatella at Tomales Bay is particularly significant because this locality is ∼500 km northwest of its modern northern limit. Dosinia ponderosa at present ranges only as far north as Laguna Ojo de Liebre on the Pacific coast of Baja California Sur, just east of Punta Eugenia (Fig. 40b). Like C. undatella, D. ponderosa ranges south along the coast of Baja California Sur, including the Golfo de California, all the way to Peru and including the Galapagos Islands (Coan and Valentich-Scott, 2012). In fossil form, it is found at several localities dated or correlated to MIS 5e in Baja California Sur and Sonora, all within its present range, but also as far north as Potrero Canyon near Los Angeles, California, and at Newport Bay (Fig. 40b). These California localities are ∼750 km northwest of the modern northern limit of D. ponderosa.
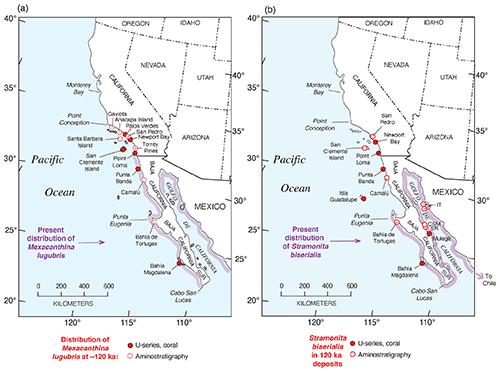
Figure 41Examples of extralimital southern species of gastropods indicating sea surface temperatures higher than present during MIS 5e (filled and open circles); also shown, in light purple shading, are the modern ranges of these taxa (from Bertsch and Aguilar Rosas, 2016, and Keen, 1971): (a) Mexacanthina lugubris and (b) Stramonita biserialis. Age and faunal data compiled from Jordan (1936), Kanakoff and Emerson (1959), Valentine (1962, 1980), Lipps et al. (1968), Kern (1977), Ashby et al. (1987), Omura et al. (1979), Lindberg et al. (1980), Emerson et al. (1981), Ortlieb (1987), Rockwell et al. (1989), Muhs (1983), Muhs et al. (1992, 2002a, 2014a), Grant et al. (1999), and Wehmiller and Pellerito (2015). Note that the presence of Stramonita biserialis on San Clemente Island is from a recent discovery of this taxon by Lindsey T. Groves, Natural History Museum of Los Angeles County, from the NOTS Pier terrace (Muhs, 1983), correlated to the Eel Point terrace (∼120 ka; Muhs et al., 2002a) by amino acid ratios in mollusks.
The gastropod fossil records from MIS 5e terrace deposits also show that what are now southern species lived farther north during the last interglacial period. Mexacanthina lugubris is a gastropod only rarely found as far north as San Diego. Bertsch and Aguilar Rosas (2016) report that on the Pacific coast, M. lugubris presently lives from San Diego to Cabo San Lucas, and on the eastern Golfo de California coast, the species is found from Bahía Kino, Sonora, to Mazatlán, Sinaloa. Fossil occurrences of Mexacanthina lugubris in deposits dated to ∼120 ka are found all along this taxon's modern Pacific coast distribution, from Bahía de Magdalena, Baja California Sur, to Point Loma, near San Diego. However, there are also some occurrences reported in MIS 5e deposits well north of the modern range endpoint for Mexacanthina lugubris (Fig. 41a). Although warmer waters during the last interglacial period allowed Chione undatella to migrate north of its modern range by several hundred kilometers, Point Conception was apparently a barrier to northward migration of Mexacanthina lugubris beyond the Santa Barbara region. Another gastropod, Stramonita biserialis, presently lives from Cedros Island, just north of Punta Eugenia, south along Baja California Sur, throughout the Golfo de California, and all the way to Chile, as well as being on the Galapagos Islands (Keen, 1971). In MIS 5e deposits, it is found at localities in the Golfo de California and along the coast of Baja California Sur, all within its modern range (Fig. 41b). However, it is also found in MIS 5e deposits on Isla Guadalupe, along the northwestern coast of Baja California, and in some southern California localities as far north as San Pedro, California, near Los Angeles (Fig. 41b). The occurrence of S. biserialis in San Pedro is a northward extension of its modern range by nearly 700 km.
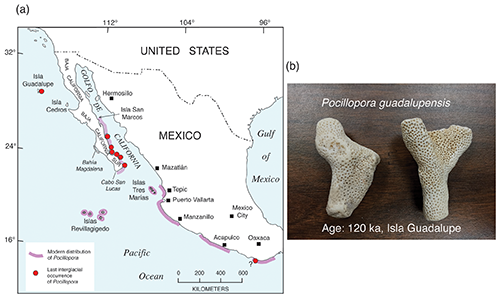
Figure 42(a) Map showing the modern distribution of species of the hermatypic coral genus Pocillopora (purple shading; from Reyes-Bonilla and López-Pérez, 1998) and U-series-dated MIS 5e occurrences of this genus elsewhere in the region. MIS 5e age and faunal data from Durham (1980), Lindberg et al. (1980), Sirkin et al. (1990), Szabo et al. (1990), Muhs et al. (2002a), DeDiego-Forbis et al. (2004), Johnson et al. (2007), and Tierney and Johnson (2012). Occurrence of fossil Pocillopora on the Mexican coast south of Oaxaca is undated but could be of MIS 5e age and is from Palmer (1928a, b). (b) Photograph of fragments of Pocillopora guadalupensis from Isla Guadalupe, Mexico, dated to ∼120 ka (Muhs et al., 2002a). Photograph by Daniel R. Muhs.
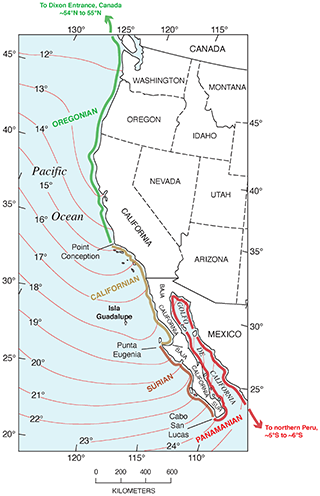
Figure 43Map of a part of the Pacific coast of North America showing marine invertebrate faunal zones (from Valentine, 1966) and their correlation with mean annual sea surface temperatures (temperature data from U.S. National Oceanic and Atmospheric Administration).
One particularly interesting locality, with U-series ages of ∼120 ka on corals, is the low-elevation marine deposit on Isla Guadalupe, off the Pacific coast of Baja California (Figs. 42, 43). Isla Guadalupe is interesting zoogeographically even at present because its modern marine invertebrate fauna has elements of both the Californian and Panamanian faunal provinces (Lindberg et al., 1980), making it a particularly sensitive area. The MIS 5e marine deposits here have a fauna that has been reported by Lindberg et al. (1980) and Durham (1980). Muhs et al. (2002a) summarized the modern geographic ranges of this fauna, showing that it has a substantial number of extralimital southern species of taxa, along with what was then thought to be two northward-ranging species. With new data on ranges of species that have been published since that time (Coan and Valentich-Scott, 2012; Berschauer and Clark, 2018), that paleozoogeographic analysis has been redone here. Results indicate that the fauna contains no northward-ranging species but hosts 13 extralimital southern species (Fig. 44). All but three of these taxa have southern range endpoints south of the Equator, and six species have northern range endpoints no farther north than Bahía Magdalena, which is over 700 km southeast of Isla Guadalupe.
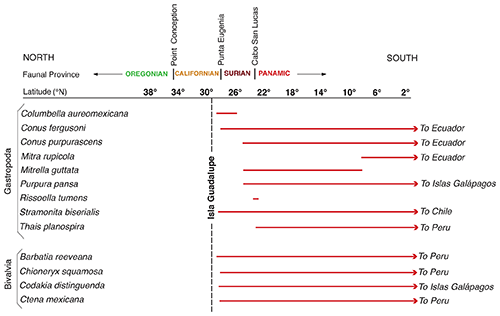
Figure 44Extralimital southern species of gastropods and bivalves (from Lindberg et al., 1980) in marine deposits of Isla Guadalupe, Mexico (see Fig. 42 for location), dated to MIS 5e by Muhs et al. (2002a), as well as their modern latitudinal distribution (from Keen, 1971, for gastropods; from Coan and Valentich-Scott, 2012, for bivalves). Note also that the deposits also contain the extralimital southern genus of coral Pocillopora (see Fig. 42) and the Indo-Pacific gastropod Naria cernica (see text for discussion).
In addition to the extralimital southern species of bivalves and mollusks within the MIS 5e fauna of Isla Guadalupe, there are two other taxa which merit additional discussion for the paleozoogeographic significance. The MIS 5e deposits of Isla Guadalupe host the North American Pacific coast's northernmost MIS 5e occurrence of a hermatypic colonial coral, Pocillopora guadalupensis (Durham, 1980). This species is not known to be living in the eastern Pacific at present (Reyes-Bonilla and López-Pérez, 1998), nor has it been found in other Pleistocene or Pliocene marine deposits in the region (López Pérez, 2008). The eastern Pacific region at present hosts five species of Pocillopora, with one species (P. verrucosa) found as far north as Isla San Marcos, on the eastern coast of Baja California Sur, and four species currently living offshore near Cabo San Lucas (Reyes-Bonilla and López-Pérez, 1998). However, Isla Guadalupe is ∼1000 km northwest of Cabo San Lucas (Fig. 42). Durham (1980) pointed out that P. guadalupensis more closely resembles Pocillopora species of the central and western Pacific Ocean (such as P. ligulata) than it does species of this genus found in the southeastern Pacific, a relationship he described as “strange”. However, it is now known that living P. ligulata is found not only in the central Pacific Ocean (including the Hawaiian Islands) but is also found off the coasts of Colombia and Ecuador (Glynn et al., 2017). Thus, one could hypothesize that perhaps there is a last interglacial evolutionary link between P. ligulata from tropical waters of northwestern South America and P. guadalupensis of Isla Guadalupe.
The other particularly noteworthy fossil reported by Lindberg et al. (1980) from the MIS 5e fauna of Isla Guadalupe is the cowry Cypraea (Erosaria) cernica, now called Naria cernica. This species is not known to occur anywhere along the Pacific coasts of the Americas, and it currently lives in the tropical waters of the Indo-West Pacific provinces (Burgess, 1985). The closest living populations of this species to Isla Guadalupe are in the Hawaiian Islands (Severns, 2011), although interestingly, the species has not yet been reported as a fossil within MIS 5e (or older) deposits on the Hawaiian Islands (Groves, 2011). In any interpretation, however, Isla Guadalupe is thousands of kilometers away from any present location where Naria cernica can be found, making its presence on this island during MIS 5e a remarkable find.
Collectively, the MIS 5e fossil record for bivalves (Chione undatella, Dosinia ponderosa), gastropods (Mexacanthina lugubris, Stramonita biserialis, Naria cernica), and coral (Pocillopora guadalupensis) from several localities, from Baja California to northern California, indicates that water temperatures off the Pacific coast of North America were substantially warmer than present. It does not appear that there were wholesale shifts of entire faunal provinces, such as the present-day Californian province being replaced entirely by Panamic species (Fig. 43). Lindberg et al. (1980) point out that of the modern fauna of Isla Guadalupe, ∼75 % are from the Californian province, ∼6 % are Panamic, and ∼19 % are biprovincial. In contrast, the MIS 5e fauna of Isla Guadalupe consists of ∼39 % Californian species, 32 % Panamic species, and ∼29 % biprovincial species. Thus, while it is clear that greater numbers of warm-water species lived in more northerly locations than is the case today, certain species existed within each faunal province as they do today. Furthermore, it appears that some of the physical geographic barriers that define provincial boundaries also served as barriers during MIS 5e despite northward migrations. A good example of this is the migration of Mexacanthina lugubris north of where its present northern limit is situated, but apparently Point Conception prevented this taxon from migration farther north into what is now the Oregonian province (Fig. 43). Still, while it may be difficult to quantify the degree of ocean warming off the Pacific coast during MIS 5e, the presence of numerous extralimital species in terrace deposits at many localities is consistent with the alkenone and foraminiferal data from deep sea cores indicating that eastern Pacific Ocean SSTs were higher than present from northern California to southern Baja California.
6.5 Controversies
In some of the earliest studies of late Quaternary sea-level history, supported by what was then the relatively new U-series dating method, there was general agreement that sea level stands during MIS 5c (∼100 ka) and MIS 5a (∼80 ka) were substantially below modern sea level by as much as 10 to 20 m (Broecker et al., 1968; Mesolella et al., 1969; Veeh and Chappell, 1970; Bloom et al., 1974; Chappell, 1974). These early studies were on Barbados and New Guinea, two areas far apart from one another, unrelated tectonically, and having quite different long-term uplift rates. The broad agreement in paleo-sea-level estimates for MIS 5c and 5a at both localities seemed to provide support for a “global” eustatic sea-level history for the late Quaternary. Later, a third locality with emergent reef terraces, the northwest coast of Haiti, showed general agreement with Barbados and New Guinea for paleo-sea levels at MIS 5c and 5a (Dodge et al., 1983), which reinforced the concept of a global eustatic sea-level curve. For New Guinea, the original paleo-sea-level estimates were refined by Chappell and Shackleton (1986).
Because marine terraces on many coastlines lack materials suitable for dating, a number of graphical methods emerged in an attempt to compare the elevation spacing of a suite of undated marine terraces with a “global” sea-level curve. Most of these schemes assumed that the detailed paleo-sea-level record of the Huon Peninsula of New Guinea (Chappell and Shackleton, 1986) is a faithful representation of global, eustatic sea-level change. Lajoie (1986) even ventured the opinion that dating a suite of marine terraces was simply a matter of correlating the undated landforms with the appropriate peaks on the New Guinea sea-level curve. Similarly, Bull (1985) proposed that the dating of an entire suite of otherwise undated marine terraces could be accomplished solely by graphical means. In Bull's (1985) method, a given terrace was assigned an age and paleo-sea level corresponding to a possible correlative terrace on New Guinea. The resultant uplift rate, along with the New Guinea sea-level curve, was used to plot inferred amounts of uplift for other terraces. The process was repeated for different assumed ages of the original terrace chosen and different uplift rates. Whichever of the resultant plots yielded the best-fit linear array of points on an inferred uplift vs. age plot was interpreted to be the correct correlation and uplift rate. In this method, once the “correct” uplift rate was identified, all terraces in the suite were dated simultaneously. The technique and variations of it have been applied to undated or partially dated terrace sequences in New Zealand (Bull and Cooper, 1986), northern California (Merritts and Bull, 1989; McCrory, 2000), central California (Hanson et al., 1994), southern California (Trecker et al., 1998), Mexico (Mayer and Vincent, 1999), and Italy (Calanchi et al., 2002).
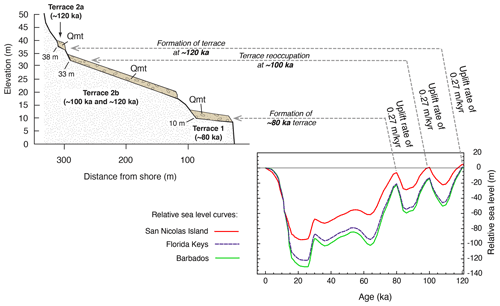
Figure 45Cross section of the lowest marine terraces on San Nicolas Island, California, and modeled sea-level curves for this island, the Florida Keys, and Barbados (from Muhs et al., 2012), showing the link between sea-level history, uplift rate, and terrace geomorphology, influenced by glacial isostatic adjustment (GIA) processes. Qmt: Quaternary marine terrace deposits.
Despite the apparent agreement for a global eustatic sea-level curve, there were always localities with marine terrace elevations that did not seem to fit the Barbados–New Guinea sea-level curve for the late Quaternary. On the Atlantic coastal plain of the USA, emergent marine deposits, a few meters above sea level, gave U-series ages on coral of ∼80 ka (Cronin et al., 1981), unexpected on a passive continental margin given the sea-level estimates at this time from Barbados and New Guinea. Similar results were obtained on tectonically stable Bermuda, where the marine facies of the Southampton Formation, at 1–2 m above sea level, yielded U-series ages on coral averaging ∼80 ka (Harmon et al., 1983). Later studies on both Bermuda and the Atlantic coastal plain, with more elevation measurements and precise TIMS U-series dating, gave the same results as these early studies (Muhs et al., 2002b; Wehmiller et al., 2004). On the tectonically active Ryukyu Islands of Japan, where reef terraces dating to ∼120, ∼100, and ∼80 ka are all present, elevations yield paleo-sea levels at MIS 5c and 5a that are close to present (Ota and Omura, 1992). U-series ages and terrace elevations from the Pacific coast of North America (California and Mexico) also give paleo-sea-level estimates for MIS 5c and 5a that are much closer to present sea level than what would be expected from the Barbados–New Guinea records (Muhs et al., 1994). Thus, despite the attractiveness of being able to date, using graphical techniques, an entire suite of marine terraces with no independent age control, it is in fact a hazardous practice.
The explanation for the disagreement between some paleo-sea-level estimates and the Barbados–New Guinea sea-level history is likely due to GIA processes. Indeed, GIA effects can and should be expected in high-latitude and mid-latitude regions of the Northern Hemisphere where large, continental ice sheets were found during glacial periods. Thus, using the Barbados–New Guinea sea-level curve for terrace correlations via elevation spacing in such regions will likely yield spurious results. In some far-field regions, distant from the Laurentide, Cordilleran, and Fennoscandian ice sheets, the elevation-spacing method of terrace correlation might be applicable, but in virtually all mid-latitude and high-latitude regions, the approach is untenable. Creveling et al. (2015) modeled apparent sea levels around the world, assuming a true “eustatic” high sea level of +6 m. These investigators showed that relative sea level, at the end of MIS 5e, could have varied from ∼5.3 to 5.7 m above present (in far-field regions such as Australia and South Africa) to as much as ∼9 to 11 m above present (on coastlines and islands of North America or around it). Further efforts along these lines by Dendy et al. (2017) confirm these differences and provide additional insights on how ice sheet configuration during the penultimate glaciation (MIS 6) influenced sea levels during MIS 5e. A combined field and modeling study on San Nicolas Island, California, showed that simulations of GIA processes over the period since MIS 5e yielded a sea-level history that matched the elevation spacing of marine terraces dating to MIS 5c and 5a (Muhs et al., 2012). Later modeling by Creveling et al. (2017) refined paleo-sea-level estimates for both of these time periods and extended the concept of differing sea-level histories to much of the globe. Simms et al. (2016, 2020) conducted GIA modeling specifically on the Pacific coast of North America and also confirmed MIS 5c and 5a paleo-sea levels were higher than what would be predicted by the Barbados–New Guinea terrace records.
GIA processes may also help explain what in the past had been an enigmatic observation about MIS 5e marine terrace faunas and controversy about their origins. It was noted above that there is no persuasive field evidence of more than one high-sea stand during MIS 5e on the Pacific coast of North America. What has been documented, however, is evidence that in areas of low uplift rate, marine terraces that formed during MIS 5e were reoccupied by the high-sea stand that followed it, MIS 5c (∼100 ka). The evidence of this sequence of events has actually been in existence for more than a century, with the recognition of “thermally anomalous” faunas, i.e., those fossil faunas with both extralimital northern and extralimital southern species of mollusks within the same deposit. Many hypotheses have been proposed to account for this (see review in Muhs and Groves, 2018), but TIMS U-series dating finally demonstrated that corals of both MIS 5e and MIS 5c age exist in the same marine terrace deposits at localities in central and southern California (see data in Table S1). At Cayucos (central California) and Point Loma (southern California), both ∼120 and ∼100 ka corals exist within the same terrace deposits, and terrace deposits at both localities also host a mix of warm-water (∼120 ka?) and cool-water (∼100 ka?) mollusks (Muhs et al., 2002a). On San Nicolas Island, the same mix of ∼120 and ∼100 ka corals and warm and cool mollusks is present in what Muhs et al. (2012) called terrace 2b. Remnants of a slightly higher-elevation terrace (“2a”) have only ∼120 ka corals and no cool-water mollusks. Where corals are lacking from other Channel Islands marine terrace deposits, amino acid data show the likelihood of two ages of shells, along with a mix of warm-water and cool-water mollusks (see Muhs and Groves, 2018, for examples). Muhs et al. (2012) showed that the likely explanation for these observations is a low uplift rate combined with GIA processes that resulted in a higher sea level during MIS 5c (Fig. 45).
Despite the general agreement between GIA models and field evidence from the Pacific coast, there is an unresolved issue. As noted, GIA modeling for MIS 5a and MIS 5c conducted by Muhs et al. (2012), Creveling et al. (2017), and Simms et al. (2016) fits the elevation differences seen in terraces of these ages in California. A problem that remains, however, is the relative elevation of the MIS 5e sea level on the Pacific coast. Simms et al. (2016) modeled relative sea level at ∼119 ka, including a correction for the eustatic component of sea-level rise (taken to be 6±3 m, relative to present), from Washington State to southernmost Baja California Sur. Their results indicate a paleo-sea level as high as +13 m relative to present along much of this coast from Washington to the southern Channel Islands, decreasing to +12 m in northern Baja California, and ultimately decreasing to +10 m in southernmost Baja California Sur. Along with their modeled paleo-sea levels for MIS 5a and 5c, there is a good match to the elevational spacing of a number of terrace sequences along the coast. In addition, there is good agreement with the Simms et al. (2016) modeling for MIS 5e and similar modeling done for selected sites on the Pacific coast by Creveling et al. (2015). Despite these promising results, Muhs et al. (2021a) pointed out that there are several localities, from central California to southernmost Baja California Sur, where elevations of MIS 5e terraces are lower than the GIA model results. These sites include Cayucos, Point San Luis, Santa Cruz Island, Anacapa Island, and Santa Barbara Island, California, as well as Isla Guadalupe, Baja California. Older, higher-elevation terraces at many of these localities preclude an explanation of subsidence as these higher terraces indicate a trend of steady, long-term uplift in the Quaternary. The reason for the differences between the field data and these well-conceptualized GIA models is not understood and needs more study.
It is interesting to note that GIA processes and their effect on relative sea levels may not be limited to MIS 5 paleo-sea levels. Returning to San Nicolas Island, it was noted that there are 14 terraces on this island (Fig. 36). Vedder and Norris (1963) reported faunal data from most of these terraces. Deposits of the 5th, 8th, and 10th terraces all contain mixes of both warm-water and cool-water species of mollusks, suggesting a similar sequence of events as that described above for MIS 5e and MIS 5c. Higher, older terraces elsewhere in California have not yet been investigated for this same kind of record but would be a worthwhile effort.
A controversy that exists for MIS 5e along the Pacific coast of North America is the amount of sea surface warming during the last interglacial period derived from the fossil record compared to that from climate modeling. As noted earlier, faunal evidence from a variety of marine terrace localities, from southern Baja California Sur to north of San Francisco Bay, indicates substantial warming during MIS 5e, relative to present. Northward migration of what are now subtropical or tropical species into mid-latitudes is documented at several localities (Figs. 13, 40, 41, 42, 44). These observations from the marine terrace record are mirrored in the foraminiferal and alkenone records found in deep-sea cores off the Pacific coast of North America (Kennett and Venz, 1995; Herbert et al., 2001; Yamamoto et al., 2007), as summarized earlier. Modeling of SSTs (as well as land surface temperatures) by Otto-Bliesner et al. (2013) indicates, however, that there was little annual surface temperature change during MIS 5e, compared to pre-industrial modern times, on a global basis. In addition, for the Pacific coast of North America specifically, their model results mirror that of the global simulation, i.e., very little difference in MIS 5e times compared to pre-industrial modern times. Otto-Bliesner et al. (2013) noted that their modeling was not able to reproduce many proxy paleoclimate records that indicate greater warmth during MIS 5e. The reason for the disagreement between the model results and the marine terrace faunal records (as well as those from deep-sea cores) is not understood at present and needs more investigation.
Data from this study are open access and available at the following link: https://doi.org/10.5281/zenodo.5557355 (Muhs et al., 2021b). Data were exported from the WALIS database on 14 April 2021, and database descriptions can be found at the following link: https://doi.org/10.5281/zenodo.3961544 (Rovere et al., 2020). Further information about the database can be examined here: https://warmcoasts.eu/world-atlas.html (last access: 14 April 2021).
In examining the work done to date on the Pacific coast of North America, several topics that could merit additional work have been mentioned. However, some specific needs that would be particularly useful are described here. Terrace mapping can certainly be improved in many areas that have not received much attention. Particularly needed are good maps of marine terraces in certain parts of northern California, central California, Baja California, and Sonora. More U-series ages on corals are needed, particularly in Oregon, northern California, and northern Baja California. Preliminary field studies that I have conducted indicate that the solitary coral Balanophyllia elegans is present in marine terrace deposits in some parts of northern California where geochronology has yet to be conducted. Similarly, around the Golfo de California, colonial corals are likely present in many marine terrace deposits that have not yet been studied, both on the Baja California peninsula and on the coast of mainland Mexico. More characterization of fossil faunas is needed. Very little work has yet been done on the coast of the Golfo de California but could be carried out in concert with new U-series dating of colonial corals. Continued refinement of GIA models and testing of those models is needed, particularly in view of the growing appreciation that paleo-sea levels around the world during MIS 5e, 5c, and 5a are going to differ from coast to coast. Higher relative sea levels during MIS 5c and 5a, with faunal mixing, are confirmed at only three localities at present in coastal California but should be investigated at other localities, particularly to see if there is a GIA gradient, which some models suggest should have existed (Simms et al., 2016). Examination of fossil faunas and dating, perhaps with Sr isotopes, of pre-MIS 5e interglacials is an endeavor that could be very usefully pursued. Records of older interglacials are found on the Palos Verdes Hills (Woodring et al., 1946), in the Newport Bay area (Vedder et al., 1957), and on San Nicolas Island (Vedder and Norris, 1963). Such studies could test the degree to which GIA effects were active during the middle and early Quaternary. Development of new dating methods for marine terrace deposits that lack corals or even mollusks is encouraged. Cosmogenic and luminescence methods have promise but need to be investigated more thoroughly, particularly in those areas where there is independent geochronologic control using U-series methods on corals. Finally, it would be useful to continue exploration of why climate modeling has shown very little evidence for warming of the eastern Pacific Ocean off North America during MIS 5e, whereas the fossil records (terrace faunas, foraminifera, alkenones) all point to substantial warming during this period. Because of the expectation of future warming of the eastern Pacific Ocean, understanding the cause of different reconstructions from models and geologic records is a worthy goal.
The supplement related to this article is available online at: https://doi.org/10.5194/essd-14-1271-2022-supplement.
The manuscript was written by DRM, who also drew all figures and compiled Tables S1, S2, and S3.
The contact author has declared that there are no competing interests.
Publisher's note: Copernicus Publications remains neutral with regard to jurisdictional claims in published maps and institutional affiliations.
This article is part of the special issue “WALIS – the World Atlas of Last Interglacial Shorelines”. It is not associated with a conference.
Much appreciation goes to Alessio Rovere, who kindly invited me to contribute this review and provided an excellent review and much editorial guidance. Many thanks go to Deirdre Ryan, who ably assisted with the details of compiling amino acid data. A big thank-you goes to John Wehmiller (University of Delaware), who helped me sort through amino acid data from nearly 40 years ago. Thanks go to Markes Johnson (Williams College) and Lauren Toth (U.S. Geological Survey) for contributing beautiful photos of fossil and modern corals, respectively. Laura Brothers and Janet Slate (U.S. Geological Survey), Jessica (JC) Creveling (Oregon State University), John Wehmiller, Hayley C. Cawthra (Council for Geoscience Western Cape office and Nelson Mandela University), and Barbara Mauz (University of Liverpool and University of Salzburg) read an earlier version of the paper and made many helpful comments for its improvement, which I appreciate very much. Daniel R. Muhs, Alessio Rovere, Deirdre Ryan, and John F. Wehmiller all contributed to the WALIS database for this review.
This research has been supported by the U.S. Geological Survey (Climate Research and Development Program).
This paper was edited by Colin V. Murray-Wallace and reviewed by Jessica Creveling, Hayley C. Cawthra, and John Wehmiller.
Abbott, R. T.: American Seashells: The Marine Mollusca of the Atlantic and Pacific Coasts of North America, 2nd edn., Van Nostrand Reinhold Company, New York, 663 pp., ISBN 0-442-20228-8, 1974.
Abbott, D. F. and Haderlie, E. C.: Prosobranchia: Marine snails, in: Intertidal Invertebrates of California, edited by: Morris, R. H., Abbott, D. P., and Haderlie, E. C., Stanford University Press, Stanford, California, 230–307, ISBN 0-8047-1045-7, 1980.
Addicott, W. O.: A late Pleistocene invertebrate fauna from southwestern Oregon, J. Paleontol., 38, 650–661, 1964a.
Addicott, W. O.: Pleistocene invertebrates from the Dume terrace, western Santa Monica Mountains, California, Bulletin of the Southern California Academy of Sciences, 63, 141–150, 1964b.
Addicott, W. O.: Late Pleistocene marine paleoecology and zoogeography in central California, U.S. Geological Survey Professional Paper, 523-C, C1–C21, 1966.
Addicott, W. O. and Emerson, W. K.: Late Pleistocene invertebrates from Punta Cabras, Baja California, Mexico, American Museum Novitates, 1925, 1–33, 1959.
Alexander, C. S.: The marine and stream terraces of the Capitola-Watsonville area, University of California Publications in Geography, 10, 1–44, 1953.
Alley, N. F. and Hicock, S. R.: The stratigraphy, palynology, and climatic significance of pre-middle Wisconsin Pleistocene sediments, southern Vancouver Island, British Columbia, Can. J. Earth Sci., 23, 369–382, 1986.
Alvarado, J. J., Reyes-Bonilla, H., Álvarez del Castillo Cardenas, P. A., Buitrago, F., and Aguirre-Rubí, J.: Coral reefs of the Pacific coast of Nicaragua, Coral Reefs, 29, 201, https://doi.org/10.1007/s00338-009-0570-0, 2010.
Anderson, R. S. and Anderson, S. P.: Geomorphology: The Mechanics and Chemistry of Landscapes, Cambridge University Press, Cambridge, 637 pp., ISBN 978-0-521-51978-6, 2010.
Anderson, R. S. and Menking, K. M.: The Quaternary marine terraces of Santa Cruz, California: Evidence for coseismic uplift on two faults, Geol. Soc. Am. B., 106, 649–664, 1994.
Arrhenius, G.: Sediment cores from the east Pacific, Swedish Deep-Sea Expedition 1947–1948, Reports 5, fasc. 1, 227 pp., 1952.
Ashby, J. R., Ku, T. L., and Minch, J. A.: Uranium-series ages of corals from the upper Pleistocene Mulege terrace, Baja California Sur, Mexico, Geology, 15, 139–141, 1987.
Barlow, N. L. M., McClymont, E. L., Whitehouse, P. L., Stokes, C. R., Jamieson, S. S. R., Woodroffe, S. A., Bentley, M. J., Callard, S. L., Ó Cofaigh, C., Evans, D. J. A., Horrocks, J. R., Lloyd, J. M., Long, A. J., Margold, M., Roberts, D. H., and Sanchez-Montes, M. L.: Lack of evidence for a substantial sea-level fluctuation within the Last Interglacial, Nat. Geosci., 11, 627–634, 2018.
Berger, G. W. and Easterbrook, D. J.: Thermoluminescence dating tests for lacustrine, glaciomarine, and floodplain sediments from western Washington and British Columbia, Can. J. Earth Sci., 30, 1815–1828, 1993.
Berger, G. W., Burke, R. M., Carver, G. A., and Easterbrook, D. J.: Test of thermoluminescence dating with coastal sediments from northern California, Chem. Geol., 87, 21–37, 1991.
Berschauer, D. P. and Clark, R. N.: Sea Shells of Southern California: Marine Shells of the Californian Province, San Diego Shell Club, Encinitas, California, 131 pp., ISBN 978-0-692-11420-9, 2018.
Bertsch, H. and Aguilar Rosas, L. E.: Marine Invertebrates of Northwest Mexico, Instituto de Investigaciones Oceanológicas, UABC, Ensenada, Mexico, 432 pp., ISBN 978-0-692-76682-8, 2016.
Birkeland, P. W.: Late Quaternary eustatic sea-level changes along the Malibu coast, Los Angeles County, California, J. Geol., 80, 432–448, 1972.
Bloom, A. L.: Sea level and coastal morphology of the United States through the late Wisconsinan glacial maximum, in: Late Quaternary Environments of the United States, Volume 1, The Late Pleistocene, edited by: Porter, S. C., University of Minnesota Press, Minneapolis, Minnesota, 215–229, ISBN 0-8166-1169-6, 1983.
Bloom, A. L., Broecker, W. S., Chappell, J. M. A., Matthews, R. K., and Mesolella, K. J.: Quaternary sea level fluctuations on a tectonic coast: New dates from the Huon Peninsula, New Guinea, Quaternary Res., 4, 185–205, 1974.
Blunt, D. J.: Geochemistry of amino acids in mollusks and wood, Pacific Northwest, United States, MSc thesis, California State University, Hayward, California, 1982.
Blunt, D. J., Easterbrook, D. J., and Rutter, N. W.: Chronology of Pleistocene sediments in the Puget Lowland, Washington, Washington Division of Geology and Earth Resources Bulletin, 77, 321–353, 1987.
Bockheim, J. G., Kelsey, H. M., and Marshall III, J. G.: Soil development, relative dating, and correlation of late Quaternary marine terraces in southwestern Oregon, Quaternary Res., 37, 60–74, 1992.
Bradley, W. C. and Addicott, W. O.: Age of first marine terrace near Santa Cruz, California, Geol. Soc. Am. B., 79, 1203–1210, 1968.
Bradley, W. C. and Griggs, G. B.: Form, genesis, and deformation of central California wave-cut platforms, Geolog. Soc. Am. B., 87, 433–449, 1976.
Brigham-Grette, J. and Hopkins, D. M.: Emergent marine record and paleoclimate of the last interglaciation along the northwest Alaskan coast, Quaternary Res., 43, 159–173, 1995.
Broecker, W. S., Thurber, D. L., Goddard, J., Ku, T.-L., Matthews, R. K., and Mesolella, K. J.: Milankovitch hypothesis supported by precise dating of coral reefs and deep-sea sediments, Science, 159, 297–300, 1968.
Bucknam, R. C., Hemphill-Haley, E., and Leopold, E. B.: Abrupt uplift within the past 1700 years at southern Puget Sound, Washington, Science, 258, 1611–1614, 1992.
Bull, W. B.: Correlation of flights of global marine terraces, in: Proceedings of the 15th Annual Geomorphology Symposium, edited by: Morisawa, M. and Hack, J. T., Allen and Unwin, Boston, 129–152, ISBN 978-0-04-551098-6, 1985.
Bull, W. B. and Cooper, A. F.: Uplifted marine terraces along the Alpine Fault, New Zealand, Science, 234, 1225–1228, 1986.
Burgess, C. M.: Cowries of the World, Gordon Verhoef Seacomber Publications, Cape Town, South Africa, 289 pp., ISBN 0-620-05483-2, 1985.
Calanchi, N., Lucchi, F., Pirazzoli, P. A., Romagnoli, C., Tranne, C. A., Radtke, U., Reyss, J. L., and Rossi, P. L.: Late Quaternary relative sea-level changes and vertical movements at Lipari (Aeolian Islands), J. Quaternary Sci., 17, 459–467, 2002.
Carver, G. A.: Late Cenozoic tectonics of coastal northern California, in: Field Guide to the Late Cenozoic Subduction Tectonics & Sedimentation of North Coastal California, Pacific Section, American Association of Petroleum Geologists, 1–9, https://doi.org/10.32375/1992-GB71.1, 1992.
Carver, G. A., Jayko, A. S., Valentine, D. W., and Li, W. H.: Coastal uplift associated with the 1992 Cape Mendocino earthquake, northern California, Geology, 22, 195–198, 1994.
Castillo, C. M., Klemperer, S. L., Ingle Jr., J. C., Powell II, C. L., Legg, M. L., and Francis, R. D.: Late Quaternary subsidence of Santa Catalina Island, California Continental Borderland, demonstrated by seismic-reflection data and fossil assemblages from submerged marine terraces, Geol. Soc. Am. B., 131, 21–42, 2018.
Chappell, J.: Geology of coral terraces, Huon Peninsula, New Guinea: A study of Quaternary tectonic movements and sea-level changes, Geol. Soc. Am. B., 85, 553–570, 1974.
Chappell, J. and Shackleton, N. J.: Oxygen isotopes and sea level, Nature, 324, 137–140, 1986.
Chappell, J., Omura, A., Esat, T., McCulloch, M., Pandolfi, J., Ota, Y., and Pillans, B.: Reconciliation of late Quaternary sea levels derived from coral terraces at Huon Peninsula with deep sea oxygen isotope records, Earth Planet. Sc. Lett., 141, 227–236, 1996.
Chen, J. H., Edwards, R. L., and Wasserburg, G. J.: 238U, 234U, and 232Th in seawater, Earth Planet. Sc. Lett., 80, 241–251, 1986.
Cheng, H., Edwards, R. L., Shen, C.-C., Polyak, V. J., Asmerom, Y., Woodhead, J., Hellstrom, J., Wang, Y., Kong, X., Spötl, C., Wang, X., and Alexander Jr., E. C.: Improvements in 230Th dating, 230Th and 234U half-life values, and U-Th isotopic measurements by multi-collector inductively coupled plasma mass spectrometry, Earth Planet. Sc. Let., 371–372, 82–91, 2013.
Chutcharavan, P. M. and Dutton, A.: A global compilation of U-series-dated fossil coral sea-level indicators for the Last Interglacial period (Marine Isotope Stage 5e), Earth Syst. Sci. Data, 13, 3155–3178, https://doi.org/10.5194/essd-13-3155-2021, 2021.
Clague, J. J., Easterbrook, D. J., Hughes, O. L., and Matthews Jr., J. V.: The Sangamonian and early Wisconsinan stages in western Canada and northwestern United States, Geol. Soc. Am. Special Paper, 270, 253–268, 1992.
Clarke, S. J. and Murray-Wallace, C. V.: Mathematical expressions used in amino acid racemisation geochronology – A review, Quat. Geochron., 1, 261–278, 2006.
CLIMAP Project Members: The last interglacial ocean, Quaternary Res., 21, 123–224, 1984.
Coan, E. V. and Valentich-Scott, P.: Bivalve seashells of tropical west America, Santa Barbara Museum of Natural History, Santa Barbara, 1258 pp., ISBN 978-0-936494-43-2, 2012.
Coan, E. V., Valentich-Scott, P., and Bernard, F. R.: Bivalve seashells of western North America: marine bivalve mollusks from Arctic Alaska to Baja California, Santa Barbara Museum of Natural History, Santa Barbara, 764 pp., ISBN 0-936494-30-1, 2000.
Cockerell, T. D. A.: Pleistocene shells from San Clemente Island, California, The Nautilus, 53, 22–23, 1939.
Colman, S. M., Mixon, R. B., Rubin, M., Bloom, A. L., and Johnson, G. H.: Comments and reply on “Late Pleistocene barrier-island sequence along the southern Delmarva Peninsula: Implications for middle Wisconsin sea levels”, Geology, 17, 84–88, 1989.
Creveling, J. R., Mitrovica, J. X., Hay, C. C., Austermann, J., and Kopp, R. E.: Revisiting tectonic corrections applied to Pleistocene sea-level highstands, Quaternary Sci. Rev., 111, 72–80, 2015.
Creveling, J. R., Mitrovica, J. X., Clark, P. U., Waelbroeck, C., and Pico, T.: Predicted bounds on peak global mean sea level during marine isotope stages 5a and 5c, Quaternary Sci. Rev., 163, 193–208, 2017.
Cronin, T. M., Szabo, B. J., Ager, T. A., Hazel, J. E., and Owens, J. P.: Quaternary climates and sea levels of the U.S. Atlantic Coastal Plain, Science, 211, 233–240, 1981.
Cuerda, J.: Los tiempos Cuaternarios en Baleares, Inst. de Estudios Baleáricos de la Diputación Provincal de Baleares, Palma, 304 pp., ISBN 978-8450072273, 1975.
Cuerda, J.: Moluscos marinos y salobres del Pleistoceno Balear, La Caja de Baleares, “Sa Nostra”, Palma, 421 pp., ISBN 978-8475351100, 1987.
Cuerda, J.: Los tiempos Cuaternarios en Baleares, 2nd edn., Dir. Gral. Cultura. Conselleria de Cultura, Educación i Esports, Govern Balear, Mallorca, Spain, 356 pp., ISBN 84-86815-07-X, 1989.
Cuerda, J. and Sacarés, J.: El Quaternari al Migjorn de Mallorca, Direcció General de Cultura, Conseeleria de Cultura. Educació i Esports, Govern Balear Spain, 130 pp., ISBN 978-84-86815-33-2, 1992.
Cutler, K. B., Edwards, R. L., Taylor, F. W., Cheng, H., Adkins, A., Gallup, C. D., Cutler, P. M., Burr, G. S., and Bloom, A. L.: Rapid sea-level fall and deep-ocean temperature change since the last interglacial period, Earth Planet. Sc. Lett., 206, 253–271, 2003.
Davis, W. M.: Glacial epochs of the Santa Monica Mountains, California, Geol. Soc. Am. B., 44, 1041–1133, 1933.
DeDiego-Forbis, T., Douglas, R., Gorsline, D., Nava-Sanchez, E., Mack, L., and Banner, J.: Late Pleistocene (Last Interglacial) terrace deposits, Bahia Coyote, Baja California Sur, México, Quaternary Int., 120, 29–40, 2004.
Delanghe, D., Bard, E., and Hamelin, B.: New TIMS constraints on the uranium-238 and uranium-234 in seawaters from the main ocean basins and the Mediterranean Sea, Marine Chem., 80, 79–93, 2002.
Delattre, M. and Rosinski, A.: Preliminary geologic map of portions of the Crescent City and Orick quadrangles, California, California Geological Survey, scale 1:100 000, 2012.
Dendy, S., Austermann, J., Creveling, J. R., and Mitrovica, J. X.: Sensitivity of Last Interglacial sea-level high stands to ice sheet configuration during Marine Isotope Stage 6, Quaternary Sci. Rev., 171, 234–244, 2017.
Dethier, D. P., Dragovich, J. D., Sarna-Wojcicki, A. M., and Fleck, R. J.: Pumice in the interglacial Whidbey Formation at Blowers Bluff, central Whidbey Island, WA, USA, Quaternary Int., 178, 229–237, 2008.
Dodge, R. E., Fairbanks, R. G., Benninger, L. K., and Maurrasse, F.: Pleistocene sea levels from raised coral reefs of Haiti, Science, 219, 1423–1425, 1983.
Drummond, K. J.: Plate-tectonic Map of the Circum-Pacific Region, American Association of Petroleum Geologists, Tulsa, Oklahoma, scale 1:10 000 000, 1981.
Durham, J. W.: A new fossil Pocillopora (coral) from Guadalupe Island, Mexico, in: The California Islands: Proceedings of a Multidisciplinary Symposium, edited by: Power, D. M., Santa Barbara, Santa Barbara Museum of Natural History, 63–70, ISBN 0-936494-01-8, 1980.
Easterbrook, D. J.: Pleistocene stratigraphy of Island County, State of Washington, Department of Water Resources, Water Supply Bulletin 25, Part I, 1–34, 1968.
Easterbrook, D. J.: Pleistocene chronology of the Puget Lowland and San Juan Islands, Washington, Geol. Soc. Am. B., 80, 2273–2286, 1969.
Easterbrook, D. J.: Chronology of pre-late Wisconsin Pleistocene sediments in the Puget Lowland, Washington, Washington Division of Geology and Earth Resources Bulletin, 80, 191–206, 1994.
Easterbrook, D. J., Crandell, D. R., and Leopold, E. B.: Pre-Olympia Pleistocene stratigraphy and chronology in the central Puget Lowland, Washington, Geol. Soc. Am. B., 78, 13–20, 1967.
Edwards, R. L., Cheng, H., Murrell, M. T., and Goldstein, S. J.: Protactinium-231 dating of carbonates by thermal ionization mass spectrometry: implications for Quaternary climate change, Science 276, 782–786, 1997.
Emerson, W. K.: Pleistocene invertebrates from Punta China, Baja California, Mexico, Bulletin of the American Museum of Natural History, 111, 313–342, 1956.
Emerson, W. K.: Pleistocene invertebrates from near Punta San José, Baja California, Mexico, American Museum Novitates, 2002, 1–7, 1960.
Emerson, W. K.: Invertebrate faunules of late Pleistocene age, with zoogeographic implications, from Turtle Bay, Baja California Sur, Mexico, The Nautilus, 94, 67–89, 1980.
Emerson, W. K. and Addicott, W. O.: A Pleistocene invertebrate fauna from the southwest corner of San Diego County, California, Transactions of the San Diego Society of Natural History, 11, 429–444, 1953.
Emerson, W. K. and Addicott, W. O.: Pleistocene invertebrates from Punta Baja, Baja California, Mexico, American Museum Novitates, 1909, 1–11, 1958.
Emerson, W. K., Kennedy, G. L., Wehmiller, J. F., and Keenan, E.: Age relations and zoogeographic implications of late Pleistocene marine invertebrate faunas from Turtle Bay, Baja California Sur, Mexico, The Nautilus, 95, 105–116, 1981.
Emery, K. O.: Shallow submerged marine terraces of southern California, Geol. Soc. Am. B., 69, 39–60, 1958.
Emiliani, C.: Pleistocene temperatures, J. Geol., 63, 538–578, 1955.
Fisher, D. M., Gardner, T. W., Marshall, J. S., Sak, P. B., and Protti, M.: Effect of subducting sea-floor roughness on fore-arc kinematics, Pacific coast, Costa Rica, Geology, 26, 467–470, 1998.
Foose, R. M.: Reconnaissance geology of Maria Cleopha Island, Tres Marias Islands, Mexico, Bulletin of the American Association of Petroleum Geologists, 46, 1740–1745, 1962.
Gallup, C. D., Edwards, R. L., and Johnson, R. G.: The timing of high sea levels over the past 200 000 years, Science, 263, 796–800, 1994.
Gallup, C. D., Cheng, H., Taylor, F. W., and Edwards, R. L.: Direct determination of the timing of sea level change during Termination II, Science, 295, 310–313, 2002.
Gardner, T., Marshall, J., Merritts, D., Bee, B., Burgette, R., Burton, E., Cooke, J., Kehrwald, N., Protti, M., Fisher, D., and Sak, P.: Holocene forearc block rotation in response to seamount subduction, southeastern Península de Nicoya, Costa Rica, Geology, 29, 151–154, 2001.
Gardner, T. W., Verdonck, D., Pinter, N. M., Slingerland, R., Furlong, K. P., Bullard, T. F., and Wells, S. G.: Quaternary uplift astride the aseismic Cocos Ridge, Pacific coast, Costa Rica, Geol. Soc. Am. B., 104, 219–232, 1992.
Gardner, T. W., Fisher, D. M., Morell, K. D., and Cupper, M. L.: Upper-plate deformation in response to flat slab subduction inboard of the aseismic Cocos Ridge, Osa Peninsula, Costa Rica, Lithosphere, 5, 247–264, 2013.
Gerrodette, T.: Equatorial submergence in a solitary coral, Balanophyllia elegans, and the critical life stage excluding the species from shallow water in the south, Marine Ecology-Progress Series, 1, 227–235, 1979.
Glynn, P. W. and Ault, J. S.: A biogeographic analysis and review of the far eastern Pacific coral reef region, Coral Reefs, 19, 1–23, 2000.
Glynn, P. W., Alvarado, J. J., Banks, S., Cortés, J., Feingold, J. S., Jiménez, C., Maragos, J. E., Martínez, P., Maté, J. L., Moanga, D. A., Navarrete, S., Reyes-Bonilla, H., Riegl, B., Rivera, F., Vargas-Ángel, B., Wieters, E. A., and Zapata, F. A.: Eastern Pacific coral reef provinces, coral community structure and composition: An overview, in: Coral Reefs of the Eastern Tropical Pacific, edited by: Glynn, P. W., Manzello, D. P., and Enochs, I. C., Springer, Dordrecht, the Netherlands, 107–176, https://doi.org/10.1007/978-94-017-7499-4_5, 2017.
Gonzalez-Garcia, J. J., Prawirodirdjo, L., Bock, Y., and Agnew, D.: Guadalupe Island, Mexico as a new constraint for Pacific plate motion, Geophys. Res. Lett., 30, 1872, https://doi.org/10.1029/2003GL017732, 2003.
Grant, L. B., Mueller, K. J., Gath, E. M., Cheng, H., Edwards, R. L., Munro, R., and Kennedy, G. L.: Quaternary uplift and earthquake potential of the San Joaquin Hills, southern Los Angeles Basin, California, Geology, 27, 1031–1034, 1999.
Grant IV, U. S. and Gale, H. R.: Catalogue of the marine Pliocene and Pleistocene of California and adjacent regions, Memoirs of the San Diego Society of Natural History, 1, 1–1036, 1931.
Graymer, R. W., Moring, B. C., Saucedo, G. J., Wentworth, C. M., Brabb, E. E., and Knudsen, K. L.: Geologic map of the San Francisco Bay region, U.S. Geological Survey Scientific Investigations Map, 2918, scale 1:275 000, http://pubs.usgs.gov/sim/2006/2918/ (last access: 14 March 2021), 2006.
Griggs, A. B.: Chromite-bearing sands of the southern part of the coast of Oregon, U.S. Geol. Surv. B., 945E, 113–150, 1945.
Grove, K., Colson, K., Binkin, M., Dull, R., and Garrison, C.: Stratigraphy and structure of the late Pleistocene Olema Creek Formation, San Andreas fault zone north of San Francisco, California, in: Recent Geologic Studies in the San Francisco Bay Area, edited by: Sanginés, E. M., Andersen, D. W., and Buising, A. V., Pacific Section of the Society of Economic Paleontologists and Mineralogists, 76, 55–76, 1995.
Groves, L. T.: Fossil marine molluscs of the Hawaiian Islands, in: Shells of the Hawaiian Islands–The Sea Shells, edited by: Severns, M., ConchBooks, Hackenheim, Germany, 536–546, ISBN 978-3-939767-35-0, 2011.
Gurrola, L. D., Keller, E. A., Chen, J. H., Owen, L. A., and Spencer, J. Q.: Tectonic geomorphology of marine terraces: Santa Barbara fold belt, California, Geol. Soc. Am. B., 126, 219–233, 2014.
Hansen, H. P. and Mackin, J. H.: A pre-Wisconsin forest succession in the Puget Lowland, Washington, Am. J. Sci., 247, 833–855, 1949.
Hanson, K. L., Wesling, J. R., Lettis, W. R., Kelson, K. I., and Mezger, L.: Correlation, ages, and uplift rates of Quaternary marine terraces: South-central coastal California, Geol. Soc. Am. Special Paper, 292, 45–71, 1994.
Harmon, R. S., Mitterer, R. M., Kriausakul, N., Land, L. S., Schwarcz, H. P., Garrett, P., Larson, G. J., Vacher, H. L., and Rowe, M.: U-series and amino-acid racemization geochronology of Bermuda: Implications for eustatic sea-level fluctuation over the past 250,000 years, Palaeogeogr. Palaeocl., 44, 41–70, 1983.
Hearty, P. J., Miller, G. H., Stearns, C. E., and Szabo, B. J.: Aminostratigraphy of Quaternary shorelines in the Mediterranean basin, Geol. Soc. Am. B., 97, 850–858, 1986.
Herbert, T. D., Schuffert, J. D., Thomas, D., Lange, C., Weinheimer, A., Peleo-Alampay, A., and Herguera, J.-C.: Depth and seasonality of alkenone production along the California margin inferred from a core top transect, Paleoceanography, 13, 263–271, 1998.
Herbert, T. D., Schuffert, J. D., Andreasen, D., Heusser, L., Lyle, M., Mix, A., Ravelo, A. C., Stott, L. D., and Herguera, J. C.: Collapse of the California Current during glacial maxima linked to climate change on land, Science, 293, 71–76, 2001.
Hertlein, L. G., and Emerson, W. K.: Results of the Puritan-American Museum of Natural History expedition to western Mexico. 5. Pliocene and Pleistocene megafossils from the Tres Marías Islands, American Museum Novitates, 1940, 1–15, 1959.
Heusser, C. J. and Heusser, L. E.: Palynology and paleotemperature analysis of the Whidbey Formation, Puget Lowland, Washington, Can. J. Earth Sci., 18, 136–149, 1981.
Hicock, S. R.: Last interglacial Muir Point Formation, Vancouver Island, British Columbia, Géographie Physique et Quaternaire, 44, 337–340, 1990.
Hicock, S. R. and Armstrong, J. E.: Four Pleistocene formations in southwest British Columbia: their implications for patterns of sedimentation of possible Sangamonian to early Wisconsin age, Can. J. Earth Sci., 20, 1232–1247, 1983.
Howarth, R. J. and McArthur, J. M.: Statistics for strontium isotope stratigraphy: A robust LOWESS fit to the marine Sr-isotope curve for 0 to 206 Ma, with look-up table for derivation of numeric age, J. Geol., 105, 441–456, 1997.
Jennings, C. W.: Fault activity map of California and adjacent areas with locations and ages of recent volcanic eruptions, California Division of Mines and Geology, Geologic Data Map no. 6, scale 1:750 000, 1994.
Jennings, C. W. and Strand, R. G.: Geologic map of California, Ukiah sheet, State of California, Department of Natural Resources, Division of Mines, scale 1:250 000, 1960.
Johnson, M. E.: Discovering the Geology of Baja California: Six Hikes on the Southern Gulf Coast, University of Arizona Press, Tucson, Arizona, 220 pp., https://doi.org/10.2307/j.ctv1t4m2dk, 2002.
Johnson, M. E., López-Pérez, R. A., Ranson, C. R., and Ledesma-Vázquez, J.: Late Pleistocene coral-reef development on Isla Coronados, Gulf of California, Ciencias Marinas, 33, 105–120, 2007.
Johnson, R. G.: Mode of formation of marine fossil assemblages of the Pleistocene Millerton Formation of California, Geol. Soc. Am. B., 73, 113–130, 1962.
Jordan, E. K.: The Pleistocene fauna of Magdalena Bay, Lower California, Contributions from the Department of Geology of Stanford University, 1, 103–174, 1936.
Kanakoff, G. P. and Emerson, W. K.: Late Pleistocene invertebrates of the Newport Bay area, California, Los Angeles County Museum Contributions in Science, 31, 1–47, 1959.
Karrow, P. F., Ceska, A., Hebda, R. J., Miller, B. B., Seymour, K. L., and Smith, A. J.: Diverse nonmarine biota from the Whidbey Formation (Sangamonian) at Point Wilson, Washington, Quaternary Res., 44, 434–437, 1995.
Kaufman, A., Broecker, W. S., Ku, T.-L., and Thurber, D. L.: The status of U-series methods of mollusk dating, Geochim. Cosmochim. Ac., 35, 1155–1183, 1971.
Kaufman, D. S. and Manley, W. F.: A new procedure for determining DL amino acid ratios in fossils using reverse phase liquid chromatography, Quaternary Sci. Rev., 17, 987–1000, 1998.
Keen, A. M.: Sea Shells of Tropical West America: Marine Mollusks from Baja California to Peru, 2nd edn., Stanford University Press, Stanford, 1064 pp., ISBN 0-8047-0736-7, 1971.
Keenan, E. M., Ortlieb, L., and Wehmiller, J. F.: Amino acid dating of Quaternary marine terraces, Bahia Ascunción, Baja California Sur, Mexico, J. Coastal Res., 3, 297–305, 1987.
Kelsey, H. M.: Late Quaternary deformation of marine terraces on the Cascadia subduction zone near Cape Blanco, Oregon, Tectonics, 9, 983–1014, 1990.
Kelsey, H. M.: Geomorphological indicators of past sea levels, in: Handbook of Sea-Level Research, edited by: Shennan, I., Long, A. J., and Horton, B. P., John Wiley & Sons, Ltd., 66–82, https://doi.org/10.1002/9781118452547.ch5, 2015.
Kelsey, H. M. and Bockheim, J. G.: Coastal landscape evolution as a function of eustasy and surface uplift rate, Cascadia margin, southern Oregon, Geol. Soc. Am. B., 106, 840–854, 1994.
Kelsey, H. M., Engebretson, D. C., Mitchell, C. E., and Ticknor, R. L.: Topographic form of the Coast Ranges of the Cascadia Margin in relation to coastal uplift rates and plate subduction, J. Geophys. Res., 99, 12245–12255, 1994.
Kelsey, H. M., Ticknor, R. L., Bockheim, J. G., and Mitchell, C. E.: Quaternary upper plate deformation in coastal Oregon, Geol. Soc. Am. B., 108, 843–860, 1996.
Kendrick, G. W., Wyrwoll, K.-H., and Szabo, B. J.: Pliocene-Pleistocene coastal events and history along the western margin of Australia, Quaternary Sci. Rev., 10, 419–439, 1991.
Kennedy, G. L.: Pleistocene paleoecology, zoogeography and geochronology of marine invertebrate faunas of the Pacific Northwest Coast (San Francisco Bay to Puget Sound), PhD thesis, unpublished, University of California-Davis, 824 pp., 1978.
Kennedy, G. L.: Zoogeographic correlation of marine invertebrate faunas, in: Quaternary Geochronology, edited by: Noller, J. S., Sowers, J. M., and Lettis, W. R., American Geophysical Union Reference Shelf, Washington, D.C., 4, 413–424, 2000.
Kennedy, G. L., Lajoie, K. R., and Wehmiller, J. F.: Aminostratigraphy and faunal correlations of late Quaternary marine terraces, Pacific Coast, USA, Nature, 299, 545–547, 1982.
Kennedy, G. L., Wehmiller, J. K., and Rockwell, T. K.: Paleoecology and paleozoogeography of late Pleistocene marine-terrace faunas of southwestern Santa Barbara County, California, in: Quaternary Coasts of the United States: Marine and Lacustrine Systems, edited by: Fletcher III, C. H. and Wehmiller, J. F., SEPM (Society for Sedimentary Geology) Special Publication, 48, 343–361, 1992.
Kennett, J. P. and Venz, K.: Late Quaternary climatically related planktonic foraminiferal assemblage changes: Hole 893A, Santa Barbara Basin, California, Proceedings of the Ocean Drilling Program, Scientific Results, 146, 281–293, 1995.
Kern, J. P.: Paleoenvironmental analysis of a late Pleistocene estuary in southern California, J. Paleontol., 45, 810–823, 1971.
Kern, J. P.: Origin and history of upper Pleistocene marine terraces, San Diego, California, Geol. Soc. Am. B., 88, 1553–1566, 1977.
Kern, J. P. and Rockwell, T. K.: Chronology and deformation of Quaternary marine shorelines, San Diego County, California, in: Quaternary Coasts of the United States: Marine and Lacustrine Systems, edited by: Fletcher III, C. H. and Wehmiller, J. F., SEPM (Society for Sedimentary Geology) Special Publication, 48, 377–382, 1992.
Kopp, R. E., Simons, F. J., Mitrovica, J. X., Maloof, A. C., and Oppenheimer, M.: Probabilistic assessment of sea level during the last interglacial stage, Nature, 462, 863–868, 2009.
Kosuge, S.: Fossil mollusks of Oahu, Hawaii Islands, Bulletin of the National Science Museum [Tokyo, Japan], 12, 783–794, 1969.
Ku, T.-L. and Kern, J. P.: Uranium-series age of the upper Pleistocene Nestor terrace, San Diego, California, Geo. Soc. Am. B., 85, 1713–1716, 1974.
Kvenvolden, K. A., Blunt, D. J., and Clifton, H. E.: Amino-acid racemization in Quaternary shell deposits at Willapa Bay, Washington, Geochim. Cosmochim. Ac., 43, 1505–1520, 1979.
Kvenvolden, K. A., Blunt, D. J., McMenamin, M. A., and Straham, S. E.: Geochemistry of amino acids in shells of the clam Saxidomus, Phys. Chem. Earth, 12, 321–332, 1980.
Kvenvolden, K. A., Blunt, D. J., and Clifton, H. E.: Age estimations based on amino acid racemization: reply to comments of J. F. Wehmiller, Geochim. Cosmochim. Ac., 45, 265–267, 1981.
Lajoie, K. R.: Coastal tectonics, in: Active Tectonics, edited by: Wallace, R., National Academy Press, Washington, D.C., 95–124, ISBN 0-309-03638-0, 1986.
Lajoie, K. R., Wehmiller, J. F., and Kennedy, G. L.: Inter- and intrageneric trends in apparent racemization kinetics of amino acids in Quaternary mollusks, in: Biogeochemistry of Amino Acids, edited by: Hare, P. E., John Wiley and Sons, 305–340, ISBN 0-471-05493-3, 1980.
Lajoie, K. R., Ponti, D. J., Powell II, C. L., Mathieson, S. A., and Sarna-Wojcicki, A. M.: Emergent marine strandlines and associated sediments, coastal California; a record of Quaternary sea-level fluctuations, vertical tectonic movements, climatic changes, and coastal processes, in: Quaternary nonglacial geology, Conterminous U.S., edited by: Morrison, R. B., Geological Society of America, Boulder, Colorado, The Geology of North America, K-2, 190–203, ISBN 0-8137-5215-9, 1991.
Lawson, A. C.: The post-Pliocene diastrophism of the coast of southern California, University of California Bulletin of the Department of Geology, 1, 115–160, 1893.
Lian, O. B., Hu, J., Huntley, D. J., and Hicock, S. R.: Optical dating studies of Quaternary organic-rich sediments from southwestern British Columbia and northwestern Washington State, Can. J. Earth Sci., 32, 1194–1207, 1995.
Libbey, L. K. and Johnson, M. E.: Upper Pleistocene rocky shores and intertidal biotas at Playa La Palmita (Baja California Sur, Mexico), J. Coastal Res., 13, 216–225, 1997.
Lindberg, D. R., Roth, B., Kellogg, M. G., and Hubbs, C. L.: Invertebrate megafossils of Pleistocene (Sangamon interglacial) age from Isla de Guadalupe, Baja California, Mexico, in: The California Islands: Proceedings of a Multidisciplinary Symposium, edited by: Power, D. M., Santa Barbara Museum of Natural History, Santa Barbara, California, 41–62, ISBN 0-936494-01-8, 1980.
Lipps, J. H., Valentine, J. W., and Mitchell, E.: Pleistocene paleoecology and biostratigraphy, Santa Barbara Island, California, J. Paleontol., 42, 291–307, 1968.
López Pérez, R. A.: Fossil corals from the Gulf of California, México: Still a depauperate fauna, but it bears more species than previously thought, Proceedings of the California Academy of Sciences, 59, 503–519, 2008.
Ludwig, K. R., Muhs, D. R., Simmons, K. R., and Moore, J. G.: Sr-isotope record of Quaternary marine terraces on the California coast and off Hawaii, Quaternary Res., 37, 267–280, 1992.
Mann, P.: Global catalogue, classification and tectonic origins of restraining- and releasing bends on active and ancient strike-slip fault systems, Geological Society of London Special Publications, 290, 13–142, 2007.
Marshall, J. S. and Anderson, R. S.: Quaternary uplift and seismic cycle deformation, Península de Nicoya, Costa Rica, Geol. Soc. Am. B., 107, 463–473, 1995.
Martin, R. E., Wehmiller, J. F., Harris, M. S., and Liddell, W. D.: Comparative taphonomy of bivalves and foraminifera from Holocene tidal flat sediments, Bahia la Choya, Sonora, Mexico (Northern Gulf of California): taphonomic grades and temporal resolution, Paleobiology, 22, 80–90, 1996.
Martinson, D. G., Pisias, N. G., Hays, J. D., Imbrie, J., Moore Jr., T. C., and Shackleton, N. J.: Age dating and the orbital theory of the ice ages: Development of a high-resolution 0 to 300,000-year chronostratigraphy, Quaternary Res., 27, 1–29, 1987.
Maxson, J. H.: Economic geology of portions of Del Norte and Siskiyou counties, northwesternmost California, California Journal of Mines and Geology, 29, 123–160, 1933.
Mayer, L. and Vincent, K. R.: Active tectonics of the Loreto area, Baja California Sur, Mexico, Geomorphology, 27, 243–255, 1999.
McCrory, P. A.: Upper plate contraction north of the migrating Mendocino triple junction, northern California: Implications for partitioning of strain, Tectonics, 19, 1144–1160, 2000.
McInelly, G. W. and Kelsey, H. M.: Late Quaternary tectonic deformation in the Cape Arago-Bandon region of coastal Oregon as deduced from wave-cut platforms, J. Geophys. Res., 95, 6699–6713, 1990.
McKay, N. P., Overpeck, J. T., and Otto-Bliesner, B. L.: The role of ocean thermal expansion in Last Interglacial sea level rise, Geophys. Res. Lett., 38, L14605, https://doi.org/10.1029/2011GL048280, 2011.
McLaughlin, R. J., Lajoie, K. R., Sorg, D. H., Morrison, S. D., and Wolfe, J. A.: Tectonic uplift of a middle Wisconsin marine platform near the Mendocino triple junction, California, Geology, 11, 35–39, 1983a.
McLaughlin, R. J., Lajoie, K. R., Sorg, D. H., Morrison, S. D., and Wolfe, J. A.: Comment and reply on `Tectonic uplift of a middle Wisconsin marine platform near the Mendocino triple junction, California', Geology, 11, 621–622, 1983b.
McLaughlin, R. J., Ellen, S. D., Blake Jr., M. C., Jayko, A. S., Irwin, W. P., Aalto, K. R., Carver, G. A., and Clarke Jr., S. H.: Geology of the Cape Mendocino, Eureka, Garberville, and southwestern part of the Hayfork 30×60 minute quadrangles and adjacent offshore area, northern California, U.S. Geological Survey Miscellaneous Field Studies, MF-2336, scales 1:100 000 (2 sheets) and 1:137 000 (1 sheet), http://pubs.usgs.gov/mf/2000/2336/ (last access: 3 July 2014), 2000.
Meco, J., Guillou, H., Carracedo, J. C., Lomoschitz, A., Ramos, A. J. G., and Rodríguez Yánez, J. J.: The maximum warmings of the Pleistocene world climate recorded in the Canary Islands, Palaeogeogr. Palaeocl., 185, 197–210, 2002.
Meco, J., Ballester, J., Betancourt, J. F., Cilleros, A., Scaillet, S., Guillou, H., Carracedo, J. C., Lomoschitz, A., Petit-Maire, N., Ramos, A. J. G., Perera, N., and Meco, J. M.: Paleoclimatologia del Neógeno en las Islas Canarias: Geliense, Pleistoceno y Holoceno, Ministerio de Medio Ambiente, Universidad de las Palmas de Gran Canaria, 203 pp., ISBN 84-96502-70-8, 2006.
Merritts, D. J.: The Mendocino triple junction: Active faults, episodic coastal emergence, and rapid uplift, J. Geophys. Res., 101, 6051–6070, 1996.
Merritts, D. and Bull, W. B.: Interpreting Quaternary uplift rates at the Mendocino triple junction, northern California, from uplifted marine terraces, Geology, 17, 1020–1024, 1989.
Mesolella, K. J., Matthews, R. K., Broecker, W. S., and Thurber, D. L.: The astronomical theory of climatic change: Barbados data, J. Geol., 77, 250–274, 1969.
Miller, G. H. and Mangerud, J.: Aminostratigraphy of European marine interglacial deposits, Quaternary Sci. Rev., 4, 215–278, 1985.
Mitchell, D. L., Ivanova, D., Rabin, R., Brown, T. J., and Redmond, K.: Gulf of California sea surface temperatures and the North American monsoon: Mechanistic implications for observations, J. Climate, 17, 2261–2281, 2002.
Mitterer, R. M. and Kriausakul, N.: Calculation of amino acid racemization ages based on apparent parabolic kinetics, Quaternary Sci. Rev., 8, 353–357, 1989.
Morell, K. D., Fisher, D. M., Gardner, T. W., La Femina, P., Davidson, D., and Teletzke, A.: Quaternary outer fore-arc deformation and uplift inboard of the Panama Triple Junction, Burica Peninsula, J. Geophys. Res., 116, 1–24, https://doi.org/10.1029/2010JB007979, 2011.
Muhs, D. R.: Quaternary sea-level events on northern San Clemente Island, California, Quaternary Res., 20, 322–341, 1983.
Muhs, D. R.: Amino acid age estimates of marine terraces and sea levels, San Nicolas Island, California, Geology, 13, 58–61, 1985.
Muhs, D. R. and Groves, L. T.: Little islands recording global events: Late Quaternary sea-level history and paleozoogeography of Santa Barbara and Anacapa Islands, Channel Islands National Park, California, Western North American Naturalist, 78, 540–589, 2018.
Muhs, D. R. and Kyser, T. K.: Stable isotope compositions of fossil mollusks from southern California: Evidence for a cool last interglacial ocean, Geology, 15, 119–122, 1987.
Muhs, D. R. and Simmons, K. R.: Taphonomic problems in reconstructing sea-level history from the late Quaternary marine terraces of Barbados, Quaternary Res., 88, 409–429, 2017.
Muhs, D. R., Kelsey, H. M., Miller, G. H., Kennedy, G. L., Whelan, J. F., and McInelly, G. W.: Age estimates and uplift rates for late Pleistocene marine terraces: Southern Oregon portion of the Cascadia forearc, J. Geophys. Res., 95, 6685–6698, 1990.
Muhs, D. R., Miller, G. H., Whelan, J. F., and Kennedy, G. L.: Aminostratigraphy and oxygen isotope stratigraphy of marine-terrace deposits, Palos Verdes Hills and San Pedro areas, Los Angeles County, California, in: Quaternary Coasts of the United States: Marine and Lacustrine Systems, edited by: Fletcher III, C. H. and Wehmiller, J. F., SEPM (Society for Sedimentary Geology) Special Publication, 48, 363–376, 1992.
Muhs, D. R., Kennedy, G. L., and Rockwell, T. K.: Uranium-series ages of marine terrace corals from the Pacific coast of North America and implications for last-interglacial sea level history, Quaternary Res., 42, 72–87, 1994.
Muhs, D. R., Simmons, K. R., Kennedy, G. L., and Rockwell, T. K.: The last interglacial period on the Pacific Coast of North America: Timing and paleoclimate, Geol. Soc. Am. B., 114, 569–592, 2002a.
Muhs, D. R., Simmons, K. R., and Steinke, B.: Timing and warmth of the last interglacial period: New U-series evidence from Hawaii and Bermuda and a new fossil compilation for North America, Quaternary Sci. Rev., 21, 1355–1383, 2002b.
Muhs, D. R., Prentice, C., and Merritts, D. J.: Marine terraces, sea level history and Quaternary tectonics of the San Andreas fault on the coast of California, in: Quaternary Geology of the United States, INQUA 2003 Field Guide Volume, edited by: Easterbrook, D., Desert Research Institute, Reno, Nevada, 1–18, ISBN 0-945920-50-4, 2003.
Muhs, D. R., Wehmiller, J. F., Simmons, K. R., and York, L. L.: Quaternary sea level history of the United States, in: The Quaternary Period in the United States, edited by: Gillespie, A. R., Porter, S. C., and Atwater, B. F., Elsevier, Amsterdam, 147–183, https://doi.org/10.1016/S1571-0866(03)01008-X, 2004.
Muhs, D. R., Simmons, K. R., Kennedy, G. L., Ludwig, K. R., and Groves, L. T.: A cool eastern Pacific Ocean at the close of the last interglacial complex, Quaternary Sci. Rev., 25, 235–262, 2006.
Muhs, D. R., Simmons, K. R., Schumann, R. R., Groves, L. T., Mitrovica, J. X., and Laurel, D.: Sea-level history during the last interglacial complex on San Nicolas Island, California: Implications for glacial isostatic adjustment processes, paleozoogeography and tectonics, Quaternary Sci. Rev., 37, 1–25, 2012.
Muhs, D. R., Groves, L. T., and Schumann, R. R.: Interpreting the paleozoogeography and sea level history of thermally anomalous marine terrace faunas: A case study from the last interglacial complex of San Clemente Island, California, Monographs of the Western North American Naturalist, 7, 82–108, 2014a.
Muhs, D. R., Meco, J., and Simmons, K. R.: Uranium-series ages of corals, sea level history, and palaeozoogeography, Canary Islands, Spain: An exploratory study for two Quaternary interglacial periods, Palaeogeogr. Palaeocl., 394, 99–118, 2014b.
Muhs, D. R., Simmons, K. R., Schumann, R. R., Groves, L. T., DeVogel, S. B., Minor, S. A., and Laurel, D.: Coastal tectonics on the eastern margin of the Pacific Rim: Late Quaternary sea-level history and uplift rates, Channel Islands National Park, California, USA, Quaternary Sci. Rev., 105, 209–238, 2014c.
Muhs, D. R., Simmons, K. R., Groves, L. T., McGeehin, J. P., Schumann, R. R., and Agenbroad, L. D.: Late Quaternary sea-level history and the antiquity of mammoths (Mammuthus exilis and Mammuthus columbi), Channel Islands National Park, California, USA, Quaternary Res., 83, 502–521, 2015.
Muhs, D. R., Pigati, J. S., Schumann, R. R., Skipp, G. L., Porat, N., and DeVogel, S. B.: Quaternary sea-level history and the origin of the northernmost coastal aeolianites in the Americas: Channel Islands National Park, California, USA, Palaeogeogr. Palaeocl., 491, 38–76, 2018.
Muhs, D. R., Schumann, R. R., Groves, L. T., Simmons, K. R., and Florian, C. R.: The marine terraces of Santa Cruz Island, California: Implications for glacial isostatic adjustment models of last-interglacial sea-level history, Geomorphology, 389, 107826, https://doi.org/10.1016/j.geomorph.2021.107826, 2021a.
Muhs, D. R., Wehmiller, J. F., Ryan, D. D., and Rovere, A.: MIS 5e relative sea-level index points along the Pacific coast of North America (1.1), Zenodo [data set], https://doi.org/10.5281/zenodo.5903285, 2021b.
Muhs, D. R., Wehmiller, J. F., Ryan, D. D., and Rovere, A.: MIS 5e relative sea-level index points along the Pacific coast of North America (1.1), Zenodo [data set], https://doi.org/10.5281/zenodo.5903285, 2021b.
Murray-Wallace, C. V. and Woodroffe, C. D.: Quaternary Sea Level Changes: A Global Perspective, Cambridge University Press, Cambridge, 484 pp., https://doi.org/10.1017/CBO9781139024440, 2014.
Murray-Wallace, C. V., Beu, A. G., Kendrick, G. W., Brown, L. J., Belperio, A. P., and Sherwood, J. E.: Palaeoclimatic implications of the occurrence of the arcoid bivalve Andadara trapezia (Deshayes) in the Quaternary of Australasia, Quaternary Sci. Rev., 19, 559–590, 2000.
O'Clair, R. M. and O'Clair, C. E.: Southeast Alaska's Rocky Shores: Animals, Plant Press, Auke Bay, Alaska, 564 pp., ISBN 0-9664245-0-6, 1998.
Ogle, B. A.: Geology of the Eel River Valley area, Humboldt County, California, Bulletin of the California Division of Mines and Geology, 164, 1–128, 1953.
Omura, A., Emerson, W. K., and Ku, T. L.: Uranium-series ages of echinoids and corals from the upper Pleistocene Magdalena terrace, Baja California Sur, Mexico, The Nautilus, 94, 184–189, 1979.
Orme, A. R.: Marine terraces and Quaternary tectonism, northwest Baja California, Mexico, Physical Geography, 1, 138–161, 1980.
Orr, P. C.: Late Pleistocene marine terraces on Santa Rosa Island, California, Geol. Soc. Am. B., 71, 1113–1120, 1960.
Ortlieb, L.: Néotectonique et variations du niveau marin au Quaternaire dans la région du Golfe de Californie, Méxique, Institut Français de Recherche Scientifique pour le Développement en Coopération, Paris, France, Collection Études et Thèses, 1036 pp., ISBN 2-7099-0887-5, 1987.
Ortlieb, L.: Quaternary shorelines along the northeastern Gulf of California; geochronological data and neotectonic implications, Geol. Soc. Am. Special Paper, 254, 95–120, 1991.
Ota, Y. and Omura, O.: Contrasting styles and rates of tectonic uplift of coral reef terraces in the Ryukyu and Daito Islands, southwestern Japan, Quaternary Int., 15/16, 17–29, 1992.
Ota, Y., Chappell, J., Kelley, R., Yonekura, N., Matsumoto, E., Nishimura, T., and Head, J.: Holocene coral reef terraces and coseismic uplift of Huon Peninsula, Papua New Guinea, Quaternary Res., 40, 177–188, 1993.
Otto-Bliesner, B. L., Rosenbloom, N., Stone, E. J., McKay, N. P., Lunt, D. J., Brady, E. C., and Overpeck, J. T.: How warm was the last interglacial? New model-data comparisons, Philoso. T. Roy. Soc. A, 371, 20130097, https://doi.org/10.1098/rsta.2013.0097, 2013.
Padgett, J. S., Kelsey, H. M., and Lamphear, D.: Upper-plate deformation of Late Pleistocene marine terraces in the Trinidad, California, coastal area, southern Cascadia subduction zone, Geosphere, 15, 1323–1341, 2019.
Palmer, R. H.: Fossil and recent corals and coral reefs of western Mexico, P. Am. Philos. Soc., 67, 21–31, 1928a.
Palmer, R. H.: Geology of southern Oaxaca, Mexico, J. Geol., 36, 718–734, 1928b.
Perg, L. A., Anderson, R. S., and Finkel, R. C.: Use of a new 10Be and 26Al inventory method to date marine terraces, Santa Cruz, California, USA, Geology, 29, 879–882, 2001.
Pigati, J. S., Quade, J., Wilson, J., Jull, A. J. T., and Lifton, N. A.: Development of low-background vacuum extraction and graphitization systems for 14C dating of old (40–60 ka) samples, Quaternary Int., 166, 4–14, 2007.
Pindell, J. L. and Kennan, L.: Tectonic evolution of the Gulf of Mexico, Caribbean and northern South America in the mantle reference frame: an update, Geol. Soc. Lond. Special Pub., 328, 1–55, 2009.
Pinter, N., Lueddecke, S.B., Keller, E.A., and Simmons, K.R.: Late Quaternary slip on the Santa Cruz Island fault, California, Geological Society of America Bulletin, 110, 711-722, 1998.
Polenz, M. and Kelsey, H. M.: Development of a late Quaternary marine terraced landscape during on-going tectonic contraction, Crescent City coastal plain, California, Quaternary Res., 52, 217–228, 1999.
Polenz, M., Schasse, H. W., Kalk, M. L., and Petersen, B. B.: Geologic map of the Camano 7.5-minute quadrangle, Island County, Washington, Washington Department of Natural Resources, Division of Geology and Earth Resources Geologic Map, GM-68, scale 1:24 000, 2009.
Putnam, W. C.: Geomorphology of the Ventura region, California, Geol. Soc. Am. B., 53, 691–754, 1942.
Reyes-Bonilla, H. and López-Pérez, A.: Biogeography of the stony corals (Scleractinia) of the Mexican Pacific, Ciencias Marinas, 24, 211–224, 1998.
Reyes-Bonilla, H. and López-Pérez, A.: Corals and coral-reef communities in the Gulf of California, in: Atlas of Coastal Ecosystems in the Western Gulf of California, edited by: Johnson, M. E. and Ledesma-Vázquez, J., University of Arizona Press, Tucson, Arizona, 45–57, ISBN 978-0-8165-2530-0, 2009.
Richards, H. G., Abbott, R. T., and Skymer, T.: The marine Pleistocene mollusks of Bermuda, Notulae Naturae, 425, 1–10, 1969.
Rockwell, T. K., Muhs, D. R., Kennedy, G. L., Hatch, M. E., Wilson, S. H., and Klinger, R. E.: Uranium-series ages, faunal correlations and tectonic deformation of marine terraces within the Agua Blanca fault zone at Punta Banda, northern Baja California, Mexico, in: Geologic Studies in Baja California, edited by: Abbott, P. L., Pacific Section, Society of Economic Paleontologists and Mineralogists, Los Angeles, California, Book 63, 1–16, 1989.
Rockwell, T. K., Nolan, J., Johnson, D. L., and Patterson, R. H.: Ages and deformation of marine terraces between Point Conception and Gaviota, Western Transverse Ranges, California, in: Quaternary Coasts of the United States: Marine and Lacustrine Systems, edited by: Fletcher III, C. H. and Wehmiller, J. F., SEPM (Society for Sedimentary Geology) Special Publication, 48, 333–341, 1992.
Rockwell, T. K., Clark, K., Gamble, L., Oskin, M. E., Haaker, E. C., and Kennedy, G. L.: Large Transverse Range earthquakes cause coastal upheaval near Ventura, southern California, B. Seismol. Soc. Am., 106, 2706–2720, 2016.
Rohling, E. J., Grant, K., Hemleben, C., Siddall, M., Hoogakker, B. A. A., Bolshaw, M., and Kucera, M.: High rates of sea-level rise during the last interglacial period, Na. Geosci., 1, 38–42, 2008.
Rovere, A., Raymo, M. E., Vacchi, M., Lorscheid, T., Stocchi, P., Gómez-Pujol, L., Harris, D. L., Casella, E., O'Leary, M. J., and Hearty, P. J.: The analysis of Last Interglacial (MIS 5e) relative sea-level indicators: Reconstructing sea-level in a warmer world, Earth-Sci. Rev., 159, 404–427, 2016.
Rovere, A., Ryan, D., Murray-Wallace, C., Simms, A., Vacchi, M., Dutton, A., Lorscheid, T., Chutcharavan, P., Brill, D., Bartz, M., Jankowski, N., Mueller, D., Cohen, K., and Gowan, E.: Descriptions of database fields for the World Atlas of Last Interglacial Shorelines (WALIS) (1,0), Zenodo [data set], https://doi.org/10.5281/zenodo.3961544, 2020.
Sak, P. B., Fisher, D. M., and Gardner, T. W.: Effects of subducting seafloor roughness on upper plate vertical tectonism: Osa Peninsula, Costa Rica, Tectonics, 23, 1–16, https://doi.org/10.1029/2002TC001474, 2004.
Sarna-Wojcicki, A. M., Lajoie, K. R., and Yerkes, R. F.: Recurrent Holocene displacement on the Javon Canyon fault–A comparison of fault-movement history with calculated recurrence intervals, U.S. Geological Survey Professional Paper, 1339, 125–135, 1987.
Schellmann, G. and Radtke, U.: A revised morpho- and chronostratigraphy of the Late and Middle Pleistocene coral reef terraces on southern Barbados (West Indies), Earth-Sci. Rev., 64, 157–187, 2004.
Schumann, R. R., Minor, S. A., Muhs, D. R., Groves, L. T., and McGeehin, J. P.: Tectonic influences on the preservation of marine terraces: Old and new evidence from Santa Catalina Island, California, Geomorphology, 179, 208–224, 2012.
Severns, M.: Shells of the Hawaiian Islands – The Sea Shells, ConchBooks, Hackenheim, Germany, 562 pp., ISBN 978-3-939767-35-0, 2011.
Shackleton, N. J.: The last interglacial in the marine and terrestrial records, P. Roy. Soc. Lond. B, 174, 135–154, 1969.
Shaw, J. H. and Suppe, J.: Active faulting and growth folding in the eastern Santa Barbara Channel, California, Geol. Soc. Am. B., 106, 607–626, 1994.
Shennan, I.: Interpretation of Flandrian Sea-level data from the fenland, Proceedings of the Geologists' Association, 83, 53–63, 1982.
Shennan, I., Long, A. J., and Horton, B. P. (Eds.): Handbook of sea-level research, John Wiley & Sons, ISBN 978-1118452585, 2015.
Simkin, T., Tilling, R. I., Vogt, P. R., Kirby, S. H., Kimberly, P., and Stewart, D. B.: This dynamic planet; world map of volcanoes, earthquakes, impact craters, and plate tectonics, U.S. Geological Survey Geologic Investigations Series Map, I-2800, scale 1:30 000 000, http://pubs.usgs.gov/imap/2800/ (last access: 1 January 2010), 2006.
Simms, A. R., Rouby, H., and Lambeck, K.: Marine terraces and rates of vertical tectonic motion: The importance of glacio-isostatic adjustment along the Pacific coast of central North America, Geol. Soc. Am. B., 128, 81–93, 2016.
Simms, A. R., Rood, D. H., and Rockwell, T. K.: Correcting MIS5e and 5a sea-level estimates for tectonic uplift, an example from southern California, Quaternary Sci. Rev., 248, 1–9, https://doi.org/10.1016/j.quascirev.2020.106571, 2020.
Sirkin, L., Szabo, B. J., Padilla, A. G., Pedrin, A. S., and Diaz, R. E.: Uranium-series ages of marine terraces, La Paz Peninsula, Baja California Sur, Mexico, Coral Reefs, 9, 25–30, 1990.
Smith, W. S. T.: A topographic study of the islands of southern California, University of California Bulletin of the Department of Geology, 2, 179–230, 1900.
Smith, W. S. T.: Marine terraces on Santa Catalina Island, Am. J. Sci., 25, 123–136, 1933.
Squires, D. F.: Results of the Puritan–American Museum of Natural History expedition to western Mexico. 7. Corals and coral reefs in the Gulf of California, Bulletin of the American Museum of Natural History, 118, 367–432, 1959.
Stein, M., Wasserburg, G. J., Lajoie, K. R., and Chen, J. H.: U-series ages of solitary corals from the California coast by mass spectrometry, Geochim. Cosmochim. Ac., 55, 3709–3722, 1991.
Stein, M., Wasserburg, G. J., Aharon, P., Chen, J. H., Zhu, Z. R., Bloom, A., and Chappell, J.: TIMS U-series dating and stable isotopes of the last interglacial event in Papua New Guinea, Geochim. Cosmochim. Ac., 57, 2541–2554, 1993.
Szabo, B. J. and Rosholt, J. N.: Uranium-series dating of Pleistocene molluscan shells from southern California–An open system model, J. Geophys. Res., 74, 3253–3260, 1969.
Szabo, B. J. and Vedder, J. G.: Uranium-series dating of some Pleistocene marine deposits in southern California, Earth Planet. Sc. Lett., 11, 283–290, 1971.
Szabo, B. J., Hausback, B. P., and Smith, J. T.: Relative inactivity during the past 140,000 years of a portion of the La Paz fault, southern Baja California Sur, Mexico, Environ. Geol. Water Sci., 15, 119–122, 1990.
Thom, B. G.: The dilemma of high interstadial sea levels during the last glaciation, Prog. Geogr., 5, 167–246, 1973.
Thompson, W. G. and Goldstein, S. L.: Open-system coral ages reveal persistent suborbital sea-level cycles, Science, 308, 401–404, 2005.
Thompson, W. G., Spiegelman, M. W., Goldstein, S. L., and Speed, R. C.: An open-system model for U-series age determinations of fossil corals, Earth Planet. Sc. Lett., 210, 365–381, 2003.
Thompson, W. G., Curran, H. A., Wilson, M. A., and White, B.: Sea-level oscillations during the last interglacial highstand recorded by Bahamas corals, Nat. Geosci., 4, 684–687, 2011.
Tierney, P. W. and Johnson, M. E.: Stabilization role of crustose coralline algae during late Pleistocene reef development on Isla Cerralvo, Baja California Sur (Mexico), J. Coastal Res., 28, 244–254, 2012.
Toth, L. T., Macintyre, I. G., and Aronson, R. B.: Holocene reef development in the eastern tropical Pacific, in: Coral Reefs of the Eastern Tropical Pacific, edited by: Glynn, P. W., Manzello, D. P., and Enochs, I. C., Springer, Dordrecht, the Netherlands, 177–201, https://doi.org/10.1007/978-94-017-7499-4_6, 2017.
Trecker, M. A., Gurrola, L. D., and Keller, E. A.: Oxygen-isotope correlation of marine terraces and uplift of the Mesa Hills, Santa Barbara, California, USA, Geol. Soc. Lond. Special Pub., 146, 57–69, 1998.
Turney, C. S. M., and Jones, R. T.: Does the Agulhas Current amplify global temperatures during super-interglacials?, J. Quaternary Sci., 25, 839–843, 2010.
Umhoefer, P. J., Maloney, S. J., Buchanan, B., Arrowsmith, J. R., Martinez-Gutiérrez, G., Kent, G., Driscoll, N., Harding, A., Kaufman, D., and Rittenour, T.: Late Quaternary faulting history of the Carrizal and related faults, La Paz region, Baja California Sur, Mexico, Geosphere, 10, 476–504, 2014.
Upson, J. E.: Former marine shore lines of the Gaviota quadrangle, Santa Barbara County, California, J. Geol., 59, 415–446, 1951.
Uyeda, S. and Kanamori, H.: Back-arc opening and the mode of subduction, J. Geophys. Res., 84, 1049–1061, 1979.
Valentine, J. W.: Upper Pleistocene mollusca from Potrero Canyon, Pacific Palisades, California, Transactions of the San Diego Society of Natural History, 12, 181–205, 1956.
Valentine, J. W.: Late Pleistocene megafauna of Cayucos, California and its zoogeographic significance, J. Paleontol., 32, 687–696, 1958.
Valentine, J. W.: Pleistocene molluscan notes. I. The Bay Point Formation at its type locality, J. Paleontol., 33, 685–688, 1959.
Valentine, J. W.: Pleistocene molluscan notes, 3. Rocky coast faunule, Bahia San Quintin, Mexico, The Nautilus, 74, 18–23, 1960a.
Valentine, J. W.: Habitats and sources of Pleistocene mollusks at Torrey Pines Park, California, Ecology, 41, 161–165, 1960b.
Valentine, J. W.: Paleoecologic molluscan geography of the Californian Pleistocene, University of California Publications in Geological Sciences, 34, 309–442, 1961.
Valentine, J. W.: Pleistocene molluscan notes. 4. Older terrace faunas from Palos Verdes Hills, California, J. Geol., 70, 92–101, 1962.
Valentine, J. W.: Numerical analysis of marine molluscan ranges on the extra-tropical northeastern Pacific shelf, Limnol. Oceanogr., 11, 198–211, 1966.
Valentine, J. W.: Camalú: A Pleistocene terrace fauna from Baja California, J. Paleontol., 54, 1310–1318, 1980.
Valentine, J. W. and Meade, R. F.: Californian Pleistocene paleotemperatures, University of California Publications in Geological Sciences, 40, 1–45, 1961.
Valentine, J. W. and Veeh, H. H.: Radiometric ages of Pleistocene terraces from San Nicolas Island, Geol. Soc. Am. B., 80, 1415–1418, 1969.
Vedder, J. G. and Norris, R. M.: Geology of San Nicolas Island California, U.S. Geological Survey Professional Paper, 369, 1–65, 1963.
Vedder, J. G., Yerkes, R. F., and Schoellhamer, J. E.: Geologic map of the San Joaquin Hills-San Juan Capistrano area, Orange County, California, U.S. Geological Survey Oil and Gas Investigations Map, OM 193, scale, 1:24 000, https://doi.org/10.3133/om193, 1957.
Vedder, J. G., Yerkes, R. F., and Schoellhamer, J. E.: Revised geologic map, structure sections and well table, San Joaquin Hills-San Juan Capistrano area, California, U.S. Geological Survey Open-File Report, 75–552, https://doi.org/10.3133/ofr75552, 1975.
Veeh, H. H. and Chappell, J.: Astronomical theory of climatic change: Support from New Guinea, Science, 167, 862–865, 1970.
Veeh, H. H. and Valentine, J. W.: Radiometric ages of Pleistocene fossils from Cayucos, California, Geol. Soc. Am. B., 78, 547–550, 1967.
Wakabayashi, J.: The Franciscan Complex, San Francisco Bay area: A record of subduction complex processes, in: Geologic Field Trips in Northern California, edited by: Wagner, D. L., and Graham, S. A., California Department of Conservation, Division of Mines and Geology Special Publication, 119, 1–21, 1999.
Weber, G. E.: Late Pleistocene slip rates on the San Gregorio fault zone at Point Año Nuevo, San Mateo County, California, in: Geology and Tectonics of the Central California Coast Region, San Francisco to Monterey, edited by: Greene, H. G., Weber, G. E., and Wright, T. L., Pacific Section of the American Association of Petroleum Geologists, Bakersfield, California, 193–203, ISBN 978-1-73398-440-9, 1990.
Weber, G. E., Lajoie, K. R., and Griggs, G. B.: Coastal tectonics & coastal geologic hazards in Santa Cruz and San Mateo Counties, California, Geological Society of America (Cordilleran Section), Field Trip Guide, 187 pp., 1979.
Wehmiller, J. F.: Kinetic model options for interpretation of amino acid enantiomeric ratios in Quaternary mollusks: Comments on a paper by Kvenvolden et al. (1979), Geochim. Cosmochim. Ac., 45, 261–264, 1981.
Wehmiller, J. F.: A review of amino acid racemization studies in Quaternary mollusks: Stratigraphic and chronologic applications in coastal and interglacial sites, Pacific and Atlantic coasts, United States, United Kingdom, Baffin Island, and tropical islands, Quaternary Sci. Rev., 1, 83–120, 1982.
Wehmiller, J. F.: Aminostratigraphy of southern California Quaternary marine terraces, in: Quaternary Coasts of the United States: Marine and Lacustrine Systems, edited by: Fletcher III, C. H. and Wehmiller, J. F., SEPM (Society for Sedimentary Geology) Special Publication, 48, 317–321, 1992.
Wehmiller, J. F.: United States Quaternary coastal sequences and molluscan racemization geochronology – What have they meant for each other over the past 45 years?, Quat. Geochron., 16, 3–20, 2013a.
Wehmiller, J. F.: Amino acid racemization, coastal sediments, in: Encyclopedia of Scientific Dating Methods, edited by: Rink, W. J. and Thompson, J., Spring-Verlag, Berlin Heidelberg, 1–10, https://doi.org/10.1007/978-94-007-6326-5_59-6, 2013b.
Wehmiller, J. F. and Belknap, D. F.: Alternative kinetic models for the interpretation of amino acid enantiomeric ratios in Pleistocene mollusks: Examples from California, Washington, and Florida, Quaternary Res., 9, 330–348, 1978.
Wehmiller, J. F., and Emerson, W. K.: Calibration of amino acid racemization in late Pleistocene mollusks: Results from Magdalena Bay, Baja California Sur, Mexico, with dating applications and paleoclimatic implications, The Nautilus, 94, 31–36, 1980.
Wehmiller, J. F. and Pellerito, V.: Database of Quaternary coastal geochronologic information for the Atlantic and Pacific coasts of North America, Delaware Geological Survey Open File Report, 50, https://www.dgs.udel.edu/publications/ofr50-database-quaternary-coastal-geochronologic-information-atlantic-and-pacific (last access: 25 March 2015), 2015.
Wehmiller, J. F., Kennedy, G. L., and Lajoie, K. R.: Amino acid racemization age estimates for Pleistocene marine deposits in the Eureka-Fields Landing area, Humboldt County, California, U.S. Geological Survey Open-File Report, 77-517, 25 pp., https://doi.org/10.3133/ofr77680, 1977a.
Wehmiller, J. F., Lajoie, K. R., Kvenvolden, K. A., Peterson, E., Belknap, D. F., Kennedy, G. L., Addicott, W. O., Vedder, J. G., and Wright, R. W.: Correlation and chronology of Pacific coast marine terrace deposits of continental United States by fossil amino acid stereochemistry–technique evaluation, relative ages, kinetic model ages, and geologic implications, U.S. Geological Survey Open-File Report, 77-680, 1–196, https://doi.org/10.3133/ofr77517, 1977b.
Wehmiller, J. F., Lajoie, K. R., Sarna-Wojcicki, A. M., Yerkes, R. F., Kennedy, G. L., Stephens, T. A., and Kohl, R. F.: Amino-acid racemization dating of Quaternary mollusks, Pacific Coast United States, U.S. Geological Survey Open-File Report, 78-701, 445–448, https://doi.org/10.3133/ofr78701, 1978.
Wehmiller, J. F., Simmons, K. R., Cheng, H., Edwards, R. L., Martin-McNaughton, J., York, L. L., Krantz, D. E., and Shen, C.-C.: Uranium-series coral ages from the U.S Atlantic Coastal Plain – the “80 ka problem” revisited, Quaternary Int., 120, 3–14, 2004.
Woodring, W. P., Bramlette, M. N., and Kew, W. S. W.: Geology and paleontology of Palos Verdes Hills, California, U.S. Geological Survey Professional Paper, 207, 1–145, 1946.
Woods, A. J.: Geomorphology, deformation, and chronology of marine terraces along the Pacific Coast of central Baja California, Mexico, Quaternary Res., 13, 346–364, 1980.
Wright, R. H.: Late Pleistocene marine fauna, Goleta, California, J. Paleontol., 46, 688–695, 1972.
Yamamoto, M., Yamamuro, M., and Tanaka, Y.: The California current system during the last 136,000 years: response of the North Pacific High to precessional forcing, Quaternary Sci. Rev., 26, 405–414, 2007.
Zullo, V. A.: A late Pleistocene marine invertebrate fauna from Bandon, Oregon, Proceedings of the California Academy of Science, 36, 347–361, 1969.
- Abstract
- Introduction
- Sea-level indicators
- Elevation measurements and geochronology
- Relative sea-level indicators
- Last Interglacial sea-level fluctuations
- Other interglacials
- Data availability
- Future research directions
- Author contributions
- Competing interests
- Disclaimer
- Special issue statement
- Acknowledgements
- Financial support
- Review statement
- References
- Supplement
- Abstract
- Introduction
- Sea-level indicators
- Elevation measurements and geochronology
- Relative sea-level indicators
- Last Interglacial sea-level fluctuations
- Other interglacials
- Data availability
- Future research directions
- Author contributions
- Competing interests
- Disclaimer
- Special issue statement
- Acknowledgements
- Financial support
- Review statement
- References
- Supplement