the Creative Commons Attribution 4.0 License.
the Creative Commons Attribution 4.0 License.
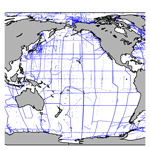
An updated version of the global interior ocean biogeochemical data product, GLODAPv2.2021
Siv K. Lauvset
Nico Lange
Toste Tanhua
Henry C. Bittig
Are Olsen
Alex Kozyr
Marta Álvarez
Susan Becker
Peter J. Brown
Brendan R. Carter
Leticia Cotrim da Cunha
Richard A. Feely
Steven van Heuven
Mario Hoppema
Masao Ishii
Emil Jeansson
Sara Jutterström
Steve D. Jones
Maren K. Karlsen
Claire Lo Monaco
Patrick Michaelis
Akihiko Murata
Fiz F. Pérez
Benjamin Pfeil
Carsten Schirnick
Reiner Steinfeldt
Toru Suzuki
Bronte Tilbrook
Anton Velo
Rik Wanninkhof
Ryan J. Woosley
Robert M. Key
The Global Ocean Data Analysis Project (GLODAP) is a synthesis effort providing regular compilations of surface-to-bottom ocean biogeochemical bottle data, with an emphasis on seawater inorganic carbon chemistry and related variables determined through chemical analysis of seawater samples. GLODAPv2.2021 is an update of the previous version, GLODAPv2.2020 (Olsen et al., 2020). The major changes are as follows: data from 43 new cruises were added, data coverage was extended until 2020, all data with missing temperatures were removed, and a digital object identifier (DOI) was included for each cruise in the product files. In addition, a number of minor corrections to GLODAPv2.2020 data were performed. GLODAPv2.2021 includes measurements from more than 1.3 million water samples from the global oceans collected on 989 cruises. The data for the 12 GLODAP core variables (salinity, oxygen, nitrate, silicate, phosphate, dissolved inorganic carbon, total alkalinity, pH, CFC-11, CFC-12, CFC-113, and CCl4) have undergone extensive quality control with a focus on systematic evaluation of bias. The data are available in two formats: (i) as submitted by the data originator but updated to World Ocean Circulation Experiment (WOCE) exchange format and (ii) as a merged data product with adjustments applied to minimize bias. For this annual update, adjustments for the 43 new cruises were derived by comparing those data with the data from the 946 quality controlled cruises in the GLODAPv2.2020 data product using crossover analysis. Comparisons to estimates of nutrients and ocean CO2 chemistry based on empirical algorithms provided additional context for adjustment decisions in this version. The adjustments are intended to remove potential biases from errors related to measurement, calibration, and data handling practices without removing known or likely time trends or variations in the variables evaluated. The compiled and adjusted data product is believed to be consistent with to better than 0.005 in salinity, 1 % in oxygen, 2 % in nitrate, 2 % in silicate, 2 % in phosphate, 4 µmol kg−1 in dissolved inorganic carbon, 4 µmol kg−1 in total alkalinity, 0.01–0.02 in pH (depending on region), and 5 % in the halogenated transient tracers. The other variables included in the compilation, such as isotopic tracers and discrete CO2 fugacity (fCO2), were not subjected to bias comparison or adjustments.
The original data, their documentation, and DOI codes are available at the Ocean Carbon Data System of NOAA NCEI (https://www.ncei.noaa.gov/access/ocean-carbon-data-system/oceans/GLODAPv2_2021/, last access: 7 July 2021). This site also provides access to the merged data product, which is provided as a single global file and as four regional ones – the Arctic, Atlantic, Indian, and Pacific oceans – under https://doi.org/10.25921/ttgq-n825 (Lauvset et al., 2021). These bias-adjusted product files also include significant ancillary and approximated data and can be accessed via https://www.glodap.info (last access: 29 June 2021). These were obtained by interpolation of, or calculation from, measured data. This living data update documents the GLODAPv2.2021 methods and provides a broad overview of the secondary quality control procedures and results.
- Article
(4622 KB) - Full-text XML
- Version 2016
- Version 2019
- Version 2020
- Version 2022
-
Supplement
(1139 KB) - BibTeX
- EndNote
The oceans mitigate climate change by absorbing both atmospheric CO2 corresponding to a significant fraction of anthropogenic CO2 emissions (Friedlingstein et al., 2019; Gruber et al., 2019) and most of the excess heat in the Earth system caused by the enhanced greenhouse effect (Cheng et al., 2020, 2017). The objective of GLODAP (Global Ocean Data Analysis Project; https://www.glodap.info, last access: 29 June 2021) is to ensure provision of high-quality and bias-corrected water column bottle data from the ocean surface to bottom. These data document the state and the evolving changes in physical and chemical ocean properties, e.g., the inventory of the excess CO2 in the ocean, natural oceanic carbon, ocean acidification, ventilation rates, oxygen levels, and vertical nutrient transports (Tanhua et al., 2021). The core quality controlled and bias-adjusted variables of GLODAP are salinity, dissolved oxygen, inorganic macronutrients (nitrate, silicate, and phosphate), seawater CO2 chemistry variables (dissolved inorganic carbon – TCO2; total alkalinity – TAlk; and pH on the total H+ scale), and the halogenated transient tracers chlorofluorocarbon-11 (CFC-11), CFC-12, CFC-113, and CCl4.
Table 1Variables in the GLODAPv2.2021 comma separated (csv) product files, their units, short and flag names, and corresponding names in the individual cruise exchange files. In the MATLAB product files that are also supplied a “G2” has been added to every variable name.
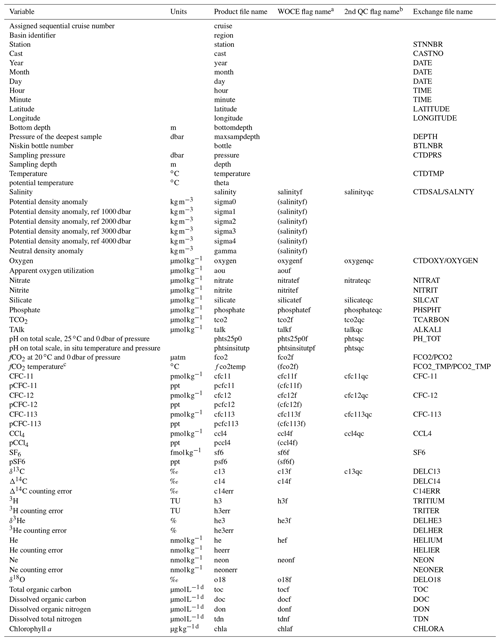
a The only derived variable assigned a separate WOCE (World Ocean Circulation Experiment) flag is AOU as it depends strongly on both temperature and oxygen (and less strongly on salinity). For the other derived variables, the applicable WOCE flag is given in parentheses. b Secondary quality control (QC) flags indicate whether data have been subjected to full secondary QC (1) or not (0), as described in Sect. 3. c Included for clarity, it is 20 ∘C for all occurrences. d Units have not been checked; some values in micromoles per kilogram (for TOC, DOC, DON, TDN) or microgram per liter (for Chl a) are probable.
Other chemical tracers that are usually measured on the cruises were included in GLODAP. In many cases a subset of these data is distributed as part of the product; however such data have not been extensively quality controlled or checked for measurement biases in this effort. For some of these variables better sources of data exist, for example the product by Jenkins et al. (2019) for helium isotope and tritium data. GLODAP also includes some derived variables to facilitate interpretation, such as potential density anomalies and apparent oxygen utilization (AOU). A full list of variables included in the product is provided in Table 1.
The oceanographic community largely adheres to principles and practices for ensuring open access to research data, such as the FAIR (Findable, Accessible, Interoperable, Reusable) initiative (Wilkinson et al., 2016), but the plethora of file formats and different levels of documentation, combined with the need to retrieve data on a per cruise basis from different access points, limit the realization of their full scientific potential. In addition, the manual data retrieval is time consuming and prone to data handling errors (Tanhua et al., 2021). For biogeochemical data there is the added complexity of different levels of standardization and calibration and even different units used for the same variable such that the comparability between datasets is often poor. Standard operating procedures have been developed for some variables (Dickson et al., 2007; Hood et al., 2010; Becker et al., 2020), and certified reference materials (CRMs) exist for seawater TCO2 and TAlk measurements (Dickson et al., 2003) and for nutrients in seawater (CRMNS; Aoyama et al., 2012; Ota et al., 2010). Despite this, biases in data still occur. These can arise from poor sampling and preservation practices, calibration procedures, instrument design, and inaccurate calculations. The use of CRMs does not by itself ensure accurate measurements of seawater CO2 chemistry (Bockmon and Dickson, 2015), and the CRMNS have only become available recently and are not universally used. For salinity and oxygen, lack of calibration of the data from conductivity–temperature–depth (CTD) profiler mounted sensors is an additional and widespread problem, particularly for oxygen (Olsen et al., 2016). For halogenated transient tracers, uncertainties in standard gas composition, extracted water volume, and purge efficiency typically provide the largest sources of uncertainty. In addition to bias, occasional outliers occur. In rare cases poor precision – many multiples worse than that expected with current measurement techniques – can render a set of data of limited use. GLODAP deals with these issues by presenting the data in a uniform format, including any metadata either publicly available or submitted by the data originator, and by subjecting the data to primary and secondary quality control assessments, focusing on precision and consistency, respectively. The secondary quality control focuses on deep data, in which natural variability is minimal. Adjustments are applied to the data to minimize cases of bias that could be confidently established relative to the measurement precision for the variables and cruises considered. Key metadata are provided in the header of each data file, and full cruise reports submitted by the data providers are accessible through the GLODAPv2 cruise summary table (https://www.ncei.noaa.gov/access/ocean-carbon-data-system/oceans/GLODAPv2_2021/cruise_table_v2021.html, last access: 7 July 2021).
GLODAPv2.2021 builds on earlier synthesis efforts for biogeochemical data obtained from research cruises, GLODAPv1.1 (Key et al., 2004; Sabine et al., 2005), Carbon dioxide in the Atlantic Ocean (CARINA) (Key et al., 2010), Pacific Ocean Interior Carbon (PACIFICA) (Suzuki et al., 2013), and notably GLODAPv2 (Olsen et al., 2016). GLODAPv1.1 combined data from 115 cruises with biogeochemical measurements from the global ocean. The vast majority of these were the sections covered during the World Ocean Circulation Experiment and the Joint Global Ocean Flux Study (WOCE/JGOFS) in the 1990s, but data from important “historical” cruises were also included, such as from the Geochemical Ocean Sections Study (GEOSECS), Transient Traces in the Ocean (TTO), and South Atlantic Ventilation Experiment (SAVE). GLODAPv2 was released in 2016 with data from 724 scientific cruises, including those from GLODAPv1.1, CARINA, and PACIFICA, as well as data from 168 additional cruises. A particularly important source of data was the cruises executed within the framework of the “repeat hydrography” program (Talley et al., 2016), instigated in the early 2000s as part of the Climate and Ocean – Variability, Predictability and Change (CLIVAR) program and since 2007 organized as the Global Ocean Ship-based Hydrographic Investigations Program (GO-SHIP) (Sloyan et al., 2019). GLODAPv2 is now updated regularly using the “living data process” of Earth System Science Data to document significant additions and changes to the dataset.
There are two types of GLODAP updates: full and intermediate. Full updates involve a reanalysis, notably crossover and inversion, of the entire dataset (both historical and new cruises) and all data points are subject to potential adjustment. This was carried out for GLODAPv2. For intermediate updates, recently available data are added following quality control procedures to ensure their consistency with the cruises included in the latest GLODAP release. Except for obvious outliers and similar types of errors (Sect. 3.3.1), the data included in previous releases are not changed during intermediate updates. Additionally, the GLODAP mapped climatologies (Lauvset et al., 2016) are not updated for these intermediate products. A naming convention has been introduced to distinguish intermediate from full product updates. For the latter the version number will change, while for the former the year of release is appended. The exact version number and release year (if appended) of the product used should always be reported in studies rather than making a generic reference to GLODAP.
Creating and interpreting inversions, as well as other checks of the full dataset needed for full updates, are too demanding in terms of time and resources to be performed every year or 2 years. The aim is to conduct a full analysis (i.e., including an inversion) again after the third GO-SHIP survey has been completed. This completion is currently scheduled for 2023, and we anticipate that GLODAPv3 will become available a few years thereafter. In the interim, the third intermediate update is presented here, which adds data from 43 new cruises to the last update, GLODAPv2.2020 (Olsen et al., 2020).
GLODAPv2.2021 contains data from 989 cruises covering the global ocean from 1972 to 2020, compared to 946 for the period 1972–2019 for GLODAPv2.2020 (Olsen et al., 2020). Information on the 43 cruises added to this version is provided in Table A1 in the Appendix. Cruise sampling locations are shown alongside those of GLODAPv2.2020 in Fig. 1, while the coverage in time is shown in Fig. 2. Not all cruises have data for all of the above-mentioned 12 core variables. For example, cruises with only seawater CO2 chemistry or transient tracer data are still included even without accompanying nutrient data due to their value towards computation of, for example, carbon inventories. In some other cases, cruises without any of these properties measured were included – this was because they did contain data for other carbon-related tracers such as carbon isotopes, with the main intention of ensuring their wider availability. The added cruises are from the years 1982–2020, with most being more recent than 2014. In the Arctic Ocean there are seven cruises from the Canadian Basin carried out on RV Louis S. St-Laurent and one in the Nordic Seas carried out on RV Johan Hjort. In the Pacific Ocean the majority of added cruises are occupations of Line P carried out on RV John P. Tully, as well as a recent occupation of P06 (two legs with different expedition codes, EXPOCODEs) on RV Nathaniel T. Palmer. Note that for some Line P cruises only stations with seawater CO2 chemistry data have been included in the product. Thus, all new Pacific Ocean cruises have seawater CO2 chemistry data. Four out of six cruises added in the Atlantic Ocean (06M220140607 and 06M220160331 on RV Maria S. Merian and 06MT20180213 and 06MT20160828 on RV Meteor) do not have seawater CO2 chemistry data but are included for their transient tracer data. Five new Indian Ocean cruises are added, including the first occupation of GO-SHIP line I07N since 1995. All new cruises from the Indian Ocean include seawater CO2 chemistry data, including pH on three of them, and transient tracers on all (with the exception of a 1982 cruise in the Red Sea on board the RV Marion Dufresne). Finally, three new cruises are added from the Southern Ocean. All of these include seawater CO2 chemistry.
All new cruises were subjected to primary (Sect. 3.1) and secondary (Sect. 3.2) quality control (QC). These procedures are the same as for GLODAPv2.2020, aiming to ensure the consistency of the data from the 43 new cruises with the previous release of this data product (in this case, the GLODAPv2.2020 adjusted data product).
For GLODAPv2.2021 we have also added a basin identifier to the product files, where 1 is the Atlantic Ocean, 4 is the Arctic Mediterranean Sea, 8 is the Pacific Ocean, and 16 is the Indian Ocean. These regions are abbreviated AO, AMS, PO, and IO, respectively, in the adjustment table. Data in the Mediterranean Sea are classified as AO. The basin identifier is now added to the product files to make it easier for users to identify in which ocean basin an individual cruise belongs without having to use one of the four regional files. Note that there is no overlap between the regional files nor our basin identifiers, and cruises in the Southern Ocean are placed in the region where most of the data were collected. In this update we have also included the DOI for each cruise in all product files, with the aim of easing access to the original data and metadata, as well as improving the visibility of data providers.
3.1 Data assembly and primary quality control
The data from the 43 new cruises were submitted directly to us or retrieved from data centers: typically the CLIVAR and Carbon Hydrographic Data Office (https://cchdo.ucsd.edu, last access: 3 June 2021), National Center for Environmental Information (https://www.ncei.noaa.gov, last access: 3 June 2021), and PANGAEA (https://pangaea.de, last access: 3 June 2021). Each cruise is identified by an expedition code (EXPOCODE). The EXPOCODE is guaranteed to be unique and constructed by combining the country code and platform code with the date of departure in the format YYYYMMDD. The country and platform codes were taken from the ICES (International Council for the Exploration of the Sea) library (https://vocab.ices.dk/, last access 3 June 2021).
The individual cruise data files were converted to the WOCE exchange format: a comma-delimited ASCII format for CTD and bottle data from hydrographic cruises. GLODAP only includes bottle data and CTD data at bottle trip depths, and their exchange format is briefly reviewed here with full details provided in Swift and Diggs (2008). The first line of each exchange file specifies the data type – in the case of GLODAP this is “BOTTLE” – followed by a date and time stamp and identification of the group and person who prepared the file; e.g., “PRINUNIVRMK” is Princeton University, Robert M. Key. Next follows the README section; this provides brief cruise-specific information, such as dates, ship, region, method plus quality notes for each variable measured, citation information, and references to any papers that used or presented the data. The README information was typically assembled from the information contained in the metadata submitted by the data originator. In some cases, issues noted during the primary QC and other information such as file update notes are included. The only rule for the README section is that it must be concise and informative. The README is followed by data column headers, units, and then the data. The headers and units are standardized and provided in Table 1 for the variables included in GLODAP. Exchange file preparation required unit conversion in some cases, most frequently from milliliters per liter (mL L−1; oxygen) or micromoles per liter (µmol L−1; nutrients) to micromoles per kilogram of seawater (µmol kg−1). The default conversion procedure for nutrients was to use seawater density at reported salinity, an assumed measurement-temperature of 22 ∘C, and pressure of 1 atm. For oxygen, the factor 44.66 was used for the “milliliters of oxygen” to “micromoles of oxygen” conversion, while the density required for the “per liter” to “per kilogram” conversion was calculated from the reported salinity and draw temperatures whenever possible. However, potential density was used instead when draw temperature was not reported. The potential errors introduced by any of these procedures are insignificant. Missing numbers are indicated by −999.
Table 2WOCE flags in GLODAPv2.2021 exchange-format original data files (briefly; for full details see Swift, 2010) and the simplified scheme used in the merged product files.
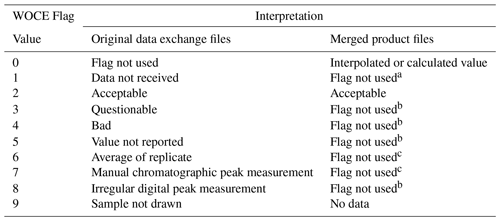
a Flag set to 9 in product files. b Data are not included in the GLODAPv2.2021 product files, and their flags are set to 9. c Data are included, but flag is set to 2.
Each data column (except temperature and pressure, which are assumed “good” if they exist) has an associated column of data flags. For the original data exchange files, these flags conform to the WOCE definitions for water samples and are listed in Table 2. For the merged and adjusted product files these flags are simplified: questionable (WOCE flag 3) and bad (WOCE flag 4) data are removed, and their flags are set to 9. The same procedure is applied to data flagged 8 (very few such data exist); WOCE flags of 1 (data not received) and 5 (data not reported) are also set to 9, while flags of 6 (mean of replicate measurements) and 7 (manual chromatographic peak measurement) are set to 2 if the data appear good. Also, in the merged product files a flag of 0 is used to indicate a value that could be measured but is approximated: for salinity, oxygen, phosphate, nitrate, and silicate, the approximation is conducted using vertical interpolation; for seawater CO2 chemistry variables (TCO2, TAlk, pH, and fCO2), the approximation is conducted using the calculation from two measured CO2 chemistry variables (Sect. 3.2.2). Importantly, interpolation of CO2 chemistry variables is never performed, and thus a flag value of 0 has a unique interpretation.
If no WOCE flags were submitted with the data, then they were assigned by us. Regardless, all incoming files were subjected to primary QC to detect questionable or bad data – this was carried out following Sabine et al. (2005) and Tanhua et al. (2010) primarily by inspecting property–property plots. Outliers showing up in two or more different such plots were generally defined as questionable and flagged. In some cases, outliers were detected during the secondary QC; the consequent flag changes have then also been applied in the GLODAP versions of the original cruise data files in agreement with the data submitter.
3.2 Secondary quality control
The aim of the secondary QC was to identify and correct any significant biases in the data from the 43 new cruises relative to GLODAPv2.2020 while retaining any signal due to temporal changes. To this end, secondary QC in the form of consistency analyses was conducted to identify offsets in the data. All identified offsets were scrutinized by the GLODAP reference group through a series of teleconferences during April 2021 in order to decide the adjustments to be applied to correct for the offset (if any). To guide this process, a set of initial minimum adjustment limits was used (Table 3). These represent the minimum bias that can be confidently established relative to the measurement precision for the variables and cruises considered and are the same as those used for GLODAPv2.2020. In addition to the average magnitude of the offsets, factors such as the precision of the offsets, persistence towards the various cruises used in the comparison, regional dynamics, and the occurrence of time trends or other variations were considered. Thus, not all offsets larger than the initial minimum limits have been adjusted. A guiding principle for these considerations was to not apply an adjustment whenever in doubt. Conversely, in some cases when data and offsets were very precise and the cruise had been conducted in a region where variability is expected to be small, adjustments lower than the minimum limits were applied. Any adjustment was applied uniformly to all values for a variable and cruise; i.e., an underlying assumption is that cruises suffer from either no or a single and constant measurement bias. Adjustments for salinity, TCO2, TAlk, and pH are always additive, while adjustments for oxygen, nutrients, and the halogenated transient traces are always multiplicative. Except where explicitly noted (Sect. 3.3.1), adjustments were not changed for data previously included in GLODAPv2.2020.
Table 3Initial minimum adjustment limits. These limits represent the minimum bias that can be confidently established relative to the measurement precision for the variables and cruises considered. Note that these limits are not uncertainties but rather a priori estimates of global inter-cruise consistency in the data product.
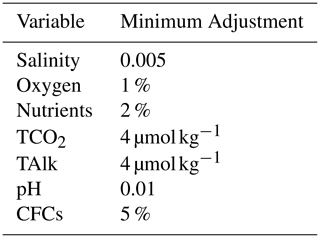
Crossover comparisons, multi-linear regressions (MLRs), and comparison of deep-water averages were used to identify offsets for salinity, oxygen, nutrients, TCO2, TAlk, and pH (Sect. 3.2.2 and 3.2.3). As in GLODAPv2.2020, but in contrast to GLODAPv2 and GLODAPv2.2019, the evaluation of the internal consistency of the seawater CO2 chemistry variables was not used for the evaluation of pH (Sect. 3.2.4). As in GLODAPv2.2020 we made extensive use of two predictions from two empirical algorithms – CArbonate system And Nutrients concentration from hYdrological properties and Oxygen using a Neural-network version B (CANYON-B) and CONsisTency EstimatioN and amounT (CONTENT) (Bittig et al., 2018) – for the evaluation of offsets in nutrients and seawater CO2 chemistry data (Sect. 3.2.5). For the halogenated transient tracers, comparisons of surface saturation levels and the relationships among the tracers were used to assess the data consistency (Sect. 3.2.6). For salinity and oxygen, CTD and bottle values were merged into a “hybrid” variable prior to the consistency analyses (Sect. 3.2.1).
3.2.1 Merging of sensor and bottle data
Salinity and oxygen data can be obtained by analysis of water samples (bottle data) and/or directly from the CTD sensor pack. These two measurement types are merged and presented as a single variable in the product. The merging was conducted prior to the consistency checks, ensuring their internal calibration in the product. The merging procedures were only applied to the bottle data files, which commonly include values recorded by the CTD at the pressures where the water samples are collected. Whenever both CTD and bottle data were present in a data file, the merging step considered the deviation between the two and calibrated the CTD values if required and possible. Altogether seven scenarios (Table 4) are possible for each of the CTD O2 sensor properties individually, in which the fourth and sixth never occurred during our analyses but are included to maintain consistency with GLODAPv2. The number of cases encountered for each scenario is summarized in Sect. 4.1.
3.2.2 Crossover analyses
The crossover analyses were conducted with the MATLAB toolbox prepared by Lauvset and Tanhua (2015) and with GLODAPv2.2020 as the reference data product. The toolbox implements the “running-cluster” crossover analysis first described by Tanhua et al. (2010). This analysis compares data from two cruises on a station-by-station basis and calculates a weighted mean offset between the two and its weighted standard deviation. The weighting is based on the scatter in the data such that data that have less scatter have a larger influence on the comparison than data with more scatter. Whether the scatter reflects actual variability or data precision is irrelevant in this context as increased scatter nevertheless decreases the confidence in the comparison. Stations are compared when they are within 2 arcdeg distance (∼ 200 km) of each other. Only deep data are used to minimize the effects of natural variability. Either the 1500 or 2000 dbar pressure surface was used as upper bound, depending on the amount of available data, their variation at different depths, and the region in question. Evaluation was done on a case-by-case basis by comparing crossovers with the two depth limits and using the one that provided the clearest and most robust information. In regions where deep mixing or convection occurs, such as the Nordic, Irminger, and Labrador seas, the upper bound was always placed at 2000 dbar; while winter mixing in the first two regions is normally not deeper than this (Brakstad et al., 2019; Fröb et al., 2016), convection beyond this limit has occasionally been observed in the Labrador Sea (Yashayaev and Loder, 2017). However, using an upper depth limit deeper than 2000 dbar will quickly give too few data for robust analysis. In addition, even below the deepest winter mixed layers, properties do change over the time periods considered (e.g., Falck and Olsen, 2010), so this limit does not guarantee steady conditions. In the Southern Ocean deep convection beyond 2000 dbar seldom occurs, an exception being the processes accompanying the formation of the Weddell Polynya in the 1970s (Gordon, 1978). Deep and bottom water formation usually occurs along the Antarctic coasts, where relatively thin nascent dense water plumes flow down the continental slope. We avoid such cases, which are easily recognizable. In order to avoid removing persistent temporal trends, all crossover results are also evaluated as a function of time (see below).
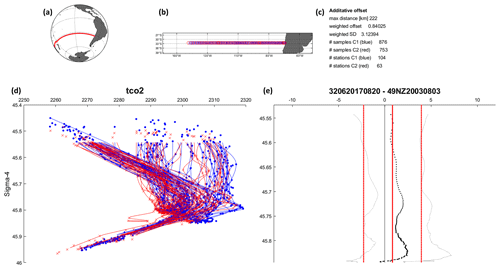
Figure 3Example crossover figure, for TCO2 for cruises 320620170820 (blue) and 49NZ20030803 (red), as was generated during the crossover analysis. Panel (a) shows all station positions for the two cruises, and (b) shows the specific stations used for the crossover analysis. Panel (d) shows the data of TCO2 (µmol kg−1) below the upper depth limit (in this case 2000 dbar) versus potential density anomaly referenced to 4000 dbar as points and the interpolated profiles as lines. Non-interpolated data either did not meet minimum depth separation requirements (Table 4 in Key et al., 2010) or are the deepest sampling depth. The interpolation does not extrapolate. Panel (e) shows the mean TCO2 (µmol kg−1) difference profile (black dots) with its standard deviation, as well as also the weighted mean offset (straight red lines) and weighted standard deviation. Summary statistics are provided in (c).
As an example of crossover analysis, the crossover for TCO2 measured on the two cruises 320620170820 (P06E), which is new to this version, and 49NZ20030803, which was included in GLODAPv2, is shown in Fig. 3. For TCO2 the offset is determined as the difference, in accordance with the procedures followed for GLODAPv2. The TCO2 values from 320620170820 are comparable, with a weighed mean offset of 0.84 ± 3.12 µmol kg−1 compared to those measured on 49NZ20030803.
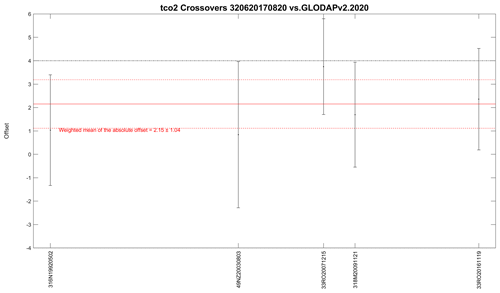
Figure 4Example summary figure, for TCO2 crossovers for 320620170820 versus the cruises in GLODAPv2.2020 (with cruise EXPOCODE listed on the x axis sorted according to year the cruise was conducted). The black dots and vertical error bars show the weighted mean offset and standard deviation for each crossover (in µmol kg−1). The weighted mean and standard deviation of all these offsets are shown in the red lines and are 2.15 ± 1.04 µmol kg−1. The dashed black line is the reference line for a +4 µmol kg−1 offset (the corresponding line for – 4 µmol kg−1 offset is right on top of the x axis and not visible).
For each of the 43 new cruises, such a crossover comparison was conducted against all possible cruises in GLODAPv2.2020, i.e., all cruises that had stations closer than 2 arcdeg distance to any station for the cruise in question. The summary figure for TCO2 on 320620170820 is shown in Fig. 4. The TCO2 data measured on this cruise are 2.15 ± 1.04 µmol kg−1 higher when compared to the data measured on nearby cruises included in GLODAPv2.2020. This is well within the initial minimum adjustment limit for TCO2 of 4 µmol kg−1 (Table 3) and as such does not qualify for an adjustment of the data in the merged data product. All other variables show the same high consistency (not shown); thus, no adjustment is given to any variable on cruise 320620170820 in GLODAPv2.2021. This is supported by the CANYON-B and CONTENT results (Sect. 3.2.5). Note that adjustments, when applied, are typically round numbers (e.g., −3 not −3.4 for TCO2 and 0.005 not 0.0047 for pH) to avoid communicating that the ideal adjustments are known to high precision.
3.2.3 Other consistency analyses
MLR analyses and deep water averages, broadly following Jutterström et al. (2010), were additionally used for the secondary QC of salinity, oxygen, nutrients, TCO2, and TAlk data. These approaches are particularly valuable when a cruise has either very few or no valid crossovers with GLODAPv2; they are used more generally to provide insight on the consistency of the data. For the 43 new cruises of the present update, no adjustment decisions were made on the basis of MLR and deep water average analyses alone. The presence of bias in the data was identified by comparing the MLR-generated values with the measured values. Both analyses were conducted on samples collected deeper than the 1500 or 2000 dbar pressure level to minimize the effects of natural variations, and both used available GLODAPv2.2020 data from within 2∘ of the cruise in question to generate the MLR or deep water average. The lower depth limit was set to the deepest sample for the cruise in question. For the MLRs, all of the above-mentioned variables could be included among the independent variables (e.g., for a TAlk MLR, salinity, oxygen, nutrients, and TCO2 were allowed), with the exact selection determined based on the statistical robustness of the fit, as evaluated using the coefficient of determination (r2) and root mean square error (RMSE). MLRs based on variables that were suspect for the cruise in question were avoided (e.g., if oxygen appeared biased it was not included as an independent variable). The MLRs could be based on 10 to 500 samples, and the robustness of the fit (r2, RMSE) and quantity of fitting data were considered when using the results to guide whether to apply a correction. The same applies for the deep-water averages (i.e., the standard deviation of the mean). MLR and deep-water average results showing offsets above the minimum adjustment limits were carefully scrutinized, along with available crossover values and CANYON-B and CONTENT estimates, to determine whether or not to apply an adjustment.
3.2.4 pH scale conversion and quality control
Altogether 13 of the 43 new cruises included measured pH data, and none required adjustment (Sect. 4.2). All new pH data were reported on the total scale and at 25 ∘C, and so no scale and/or temperature conversion was necessary. For details on scale and temperature conversions in previous versions of GLODAPv2, we refer the reader to Olsen et al. (2020). In contrast to past quality control of GLODAP pH data, evaluation of the internal consistency of CO2 system variables was not used for the secondary quality control of the pH data of the 13 new cruises; only crossover analysis was used, supplemented by CONTENT and CANYON-B comparisons (Sect. 3.2.5). Recent literature has demonstrated that internal consistency evaluation procedures are subject to errors owing to incomplete understanding of the thermodynamic constants, major ion concentrations, measurement biases, and potential contribution of organic compounds or other unknown protolytes to alkalinity. These complications lead to pH-dependent offsets in calculated pH compared with cruise spectrophotometric pH measurements (Álvarez et al., 2020; Carter et al., 2018; Fong and Dickson, 2019) but not with those derived in lab conditions using ISFET (ion sensitive field effect transistor) sensors (Takeshita et al., 2020). The pH-dependent offsets may be interpreted as biases and generate false corrections. The offsets are particularly strong at pH levels below 7.7, when calculated and measured pH are different by on average between 0.01 and 0.02 units. For the North Pacific this is a problem as pH values below 7.7 can occur at the depths interrogated during the QC (> 1500 dbar for this region; Olsen et al., 2016). Since any correction, which may be an artifact, would be applied to the full profiles, we use a minimum adjustment of 0.02 for the North Pacific pH data in the merged product files. Elsewhere, the inconsistencies that may have arisen are smaller since deep pH is typically higher than 7.7 (Lauvset et al., 2020), and at such levels the difference between calculated and measured pH is less than 0.01 on average (Álvarez et al., 2020; Carter et al., 2018). Outside the North Pacific, we believe, therefore, that the pH data are consistent to 0.01. Avoiding interconsistency considerations for these intermediate products helps to reduce the problem, but since the reference dataset (as also used for the generation of the CANYON-B and CONTENT algorithms) has these issues, a full re-evaluation, envisioned for the future GLODAPv3, is needed to address the problem completely.
3.2.5 CANYON-B and CONTENT analyses
CANYON-B and CONTENT (Bittig et al., 2018) were used to support decisions regarding application of adjustments (or not). CANYON-B is a neural network for estimating nutrients and seawater CO2 chemistry variables from temperature, salinity, and oxygen concentration. CONTENT additionally considers the consistency among the estimated CO2 chemistry variables to further refine them. These approaches were developed using the data included in the GLODAPv2 data product (i.e., the 2016 version without any more recent updates). Their advantage compared to crossover analyses for evaluating consistency among cruise data is that effects of water mass changes on ocean properties are represented in the nonlinear relationships in the underlying neural network. For example, if elevated nutrient values measured on a cruise are not due to a measurement bias but actual aging of the water masses that have been sampled and as such accompanied by a decrease in oxygen concentrations, the measured values and the CANYON-B estimates are likely to be similar. Vice versa, if the nutrient values are biased, the measured values and CANYON-B predictions will be dissimilar.
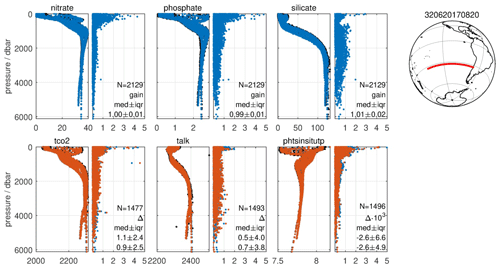
Figure 5Example summary figure for CANYON-B and CONTENT analyses for 320620170820. Any data from regions where CONTENT and CANYON-B were not trained are excluded. The top row shows the nutrients and the bottom row the seawater CO2 chemistry variables. All are shown versus sampling pressure (dbar) and the unit is micromoles per kilogram (µmol kg−1) for all except pH, which is on the total scale at in situ temperature and pressure. Black dots (which to a large extent are hidden by the predicted estimates) are the measured data, blue dots are CANYON-B estimates, and red dots are the CONTENT estimates. Each variable has two figure panels. The left shows the depth profile, while the right shows the absolute difference between measured and estimated values divided by the CANYON-B and CONTENT uncertainty estimate, which is determined for each estimated value. These values are used to gauge the comparability; a value below 1 indicates a good match as it means that the difference between measured and estimated values is less than the uncertainty of the latter. The statistics in each panel are for all data deeper than 500 dbar, and N is the number of samples considered. A multiplicative adjustment and its interquartile range are given for the nutrients. For the seawater CO2 chemistry variables the numbers in each panel are the median difference between measured and predicted values for CANYON-B (upper) and CONTENT (lower). Both are given with their interquartile range.
Used in the correct way and with caution this tool is a powerful supplement to the traditional crossover analyses which form the basis of our analyses. Specifically, we gave no weight to comparisons in which the crossover analyses had suggested that the S and/or O2 data were biased as this would lead to error in the predicted values. We also considered the uncertainties of the CANYON-B and CONTENT estimates. These uncertainties are determined for each predicted value, and for each comparison the ratio of the difference (between measured and predicted values) to the local uncertainty was used to gauge the comparability. As an example, the CANYON-B and CONTENT analyses of the data obtained for 320620170820 are presented in Fig. 5. The CANYON-B and CONTENT results confirmed the crossover comparisons for TCO2 discussed in Sect. 3.2.2. The magnitude of the inconsistency for both the CONTENT and the CANYON-B estimates was 0.6 µmol kg−1, i.e., less than the weighted mean crossover offset of 2.1 µmol kg−1 (Fig. 4). The differences between these consistency estimates is owed to differences in the actual approach, the weighting across stations, stations considered (i.e., crossover comparisons use only stations within ∼ 200 km of each other, while CANYON-B and CONTENT consider all stations where necessary variables are sampled), and depth range considered (> 500 dbar for CANYON-B and CONTENT vs. > 1500/2000 dbar for crossovers). The specific difference between the CANYON-B and CONTENT estimates is a result of the seawater CO2 chemistry considerations by the latter. For the other variables, the inconsistencies are low and agree with the crossover results (not shown here, but results can be accessed through the adjustment table).
Another advantage of the CANYON-B and CONTENT comparisons is that these procedures provide estimates at the level of individual data points; e.g., pH values are determined for every sampling location and depth where T, S, and O2 data are available. Cases of strong differences between measured and estimated values are always examined. This has helped us to identify primary QC issues for some cruises and variables, for example a case of an inverted pH profile on cruise 32PO20130829, which was identified and amended in GLODAPv2.2020.
3.2.6 Halogenated transient tracers
For the halogenated transient tracers (CFC-11, CFC-12, CFC-113, and CCl4; CFCs for short) inspection of surface saturation levels and evaluation of relationships between the tracers for each cruise were used to identify biases rather than crossover analyses. Crossover analysis is of limited value for these variables given their transient nature and low concentrations at depth. As for GLODAPv2, the procedures were the same as those applied for CARINA (Jeansson et al., 2010; Steinfeldt et al., 2010). No QC is performed for SF6 in GLODAP, but there are plans to include this in future versions.
3.3 Merged product generation
The merged product file for GLODAPv2.2021 was created by correcting known issues in the GLODAPv2.2020 merged file and then appending a merged and bias-corrected file containing the 43 new cruises to this error-corrected GLODAPv2.2020 file.
3.3.1 Updates and corrections for GLODAPv2.2020
Several minor omissions and errors have been identified in the GLODAPv2.2020 data product since the release in 2020. Most of these have been corrected in this release, but some issues, such as those relating to pH in the North Pacific (Sect. 3.2.4), will not be remedied before GLODAPv3. In addition, some recently available data have been added for a few cruises. The changes are as follows:
-
Individual suspicious samples, identified and reported by users and data providers, have been deleted from the product. This affects oxygen on cruises 31DS19940126 and 29HE20130320; nutrients on cruises 316N19950829 and 06BE20001128; salinity on cruises 06BE20001128, 316N19921006, 318M19730822, 35A319950221, 49K619940107, and 32PO20130829; and TAlk on cruises 58P320011031, 33RO20071215, and 316N19821201.
-
For data with missing (except Gerard barrels; Sect. 3.3.2) or bad temperature all other data have been set to NaN (not a number). For future updates we will attempt to find the missing temperatures and, when possible, restore the now deleted data.
-
All cases are corrected where a secondary QC flag of 1 had been erroneously assigned. This happened for cases in which the secondary QC flag was 1, but the data fields of the entire cruise were only NaN. The only case where this would be correct is if a −777 is given in the adjustment table; all other cases were changed to a secondary QC flag of 0.
-
All fCO2 data are reported at a constant temperature of 20 ∘C as described in Olsen et al. (2020). In some cases temperature was not reported for calculated fCO2, and so where missing, a temperature of 20 ∘C has been assigned to calculated fCO2 data.
-
Cruise 18SN19950803 has been given a 8 % downward adjustment on phosphate, and cruise 49NZ20020822 has been given a 6 % upward adjustment for phosphate. Both were identified as clear outliers when analyzing crossovers for the seven new cruises in the area (JOIS, Table A1), and the addition of so many new crossovers allowed for robust assessment of necessary adjustments.
-
TAlk has been updated for station 106 on cruise 33RO19980123.
-
Updated data for dissolved total nitrogen (tdn), pH, and TAlk were submitted and included for cruise 33RR20160208. Missing carbon variables have also been calculated for these updated data, and assigned a flag of 0.
-
Δ14C data on 33MW19910711 have been updated.
-
On cruise 33RO20161119 Δ14C and δ13C data have been added, and BTLNBR updated.
-
CTDPRS for station 5 (cast 2) on cruise 33RO20131223 has been corrected.
3.3.2 Merging
The new data were merged into a bias-minimized product file following the procedures used for GLODAPv2.2020 (Olsen et al., 2020) with some modifications:
-
Data from the 43 new cruises were merged and sorted according to EXPOCODE, station, and pressure. GLODAP cruise numbers were assigned consecutively, starting from 3001, so they can be distinguished from the GLODAPv2.2020 cruises, which ended at 2106.
-
For some cruises the combined concentration of nitrate and nitrite was reported instead of nitrate. If explicit nitrite concentrations were also given, these were subtracted to get the nitrate values. If not, the combined concentration was renamed to nitrate. As nitrite concentrations are very low in the open ocean, this has no practical implications.
-
When bottom depths were not given, they were approximated as the deepest sample pressure +10 dbar or extracted from ETOPO1 (Amante and Eakins, 2009), whichever was greater. For GLODAPv2, bottom depths were extracted from the Terrain Base (National Geophysical Data Center/NESDIS/NOAA/U.S. Department of Commerce, 1995). The intended use of this variable is only drawing approximate bottom topography for sections.
-
Whenever temperature was missing in the original data file, all data for that record were removed, and their flags set to 9. The same was done when both pressure and depth were missing. For all surface samples collected using buckets or similar, the bottle number was set to zero. There are some exceptions to this, in particular for cruises that also used Gerard barrels for sampling. These may have valuable tracer data that are not accompanied by a temperature, so such data have been retained.
-
All data with WOCE quality flags of 3, 4, 5, or 8 were excluded from the product files, and their flags were set to 9. Hence, in the product files a flag of 9 can indicate not measured (as is also the case for the original exchange formatted data files) or excluded from the product; in any case, no data value appears. All flags of 6 (replicate measurement) and 7 (manual chromatographic peak measurement) were set to 2, provided the data appeared good.
-
Missing sampling pressures (depths) were calculated from depths (pressures) following UNESCO (1981).
-
For both oxygen and salinity, CTD and bottle values were merged following procedures summarized in Sect. 3.2.1.
-
Missing salinity, oxygen, nitrate, silicate, and phosphate values were vertically interpolated whenever practical using a quasi-Hermitian piecewise polynomial. “Whenever practical” means that interpolation was limited to the vertical data separation distances given in Table 4 in Key et al. (2010). Interpolated salinity, oxygen, and nutrient values have been assigned a WOCE quality flag of 0.
-
The data for the 12 core variables were corrected for bias using the adjustments determined during the secondary QC.
-
Values for potential temperature and potential density anomalies (referenced to 0, 1000, 2000, 3000, and 4000 dbar) were calculated using Fofonoff (1977) and Bryden (1973). Neutral density for all 989 cruises was calculated using Jackett and McDougall (1997).
-
Apparent oxygen utilization was determined using the combined fit in Garcia and Gordon (1992).
-
Partial pressures for CFC-11, CFC-12, CFC-113, CCl4, and SF6 were calculated using the solubilities by Warner and Weiss (1985), Bu and Warner (1995), Bullister and Wisegarver (1998), and Bullister et al. (2002).
-
Missing seawater CO2 chemistry variables were calculated whenever possible. The procedures for these calculations have been slightly altered as the product now contains four such variables; earlier versions of GLODAPv2 (Olsen et al., 2016, 2019) included only three, so whenever two were included, the one to calculate was unequivocal. Four CO2 chemistry variables gives more degrees of freedom in this respect; e.g., a particular record may have measured data for TCO2, TAlk, and pH, and then a choice needs to be made with regard to which pair to use for the calculation of fCO2. We followed two simple principles. First, TCO2 and TAlk were the preferred pair to calculate pH and fCO2 because we have higher confidence in the TCO2 and TAlk data than pH (given the issues summarized in Sect. 3.2.4) and fCO2 (because it was not subjected to secondary QC). Second, if either TCO2 or TAlk was missing and both pH and fCO2 data existed, pH was preferred (because fCO2 has not been subjected to secondary QC). All other combinations involve only two measured variables. The calculations were conducted using CO2SYS (Lewis and Wallace, 1998) for MATLAB (van Heuven et al., 2011), with the carbonate dissociation constants of Lueker et al. (2000), the bisulfate dissociation constant of Dickson (1990), and the borate-to-salinity ratio of Uppström (1974), as in GLODAPv2.2020 and earlier versions (Olsen et al., 2020). We are aware that the borate-to-salinity ratio of Lee et al. (2010) is becoming the community standard but here maintain Uppström (1974) in order to maintain consistency between versions. For calculations involving TCO2, TAlk, and pH, if less than a third of the total number of values, measured and calculated combined, for a specific cruise were measured, then all these were replaced by calculated values. The reason for this is that secondary QC of the few measured values was often not possible in such cases, for example, due to a limited amount of deep data available. Such replacements were not done for calculations involving fCO2, as this would either overwrite all measured fCO2 values or would entail replacing a measured variable that has been subjected to secondary QC (i.e., TCO2, TAlk, or pH) with one calculated from a variable that has not been subjected to secondary QC (i.e., fCO2). Calculated seawater CO2 chemistry values have been assigned a WOCE flag of 0. Seawater CO2 chemistry values have not been interpolated, so the interpretation of the 0 flag is unique.
-
The resulting merged file for the 43 new cruises was appended to the merged product file for GLODAPv2.2020.
All material produced during the secondary QC is available via the online GLODAP adjustment table hosted by GEOMAR, Kiel, Germany, at https://glodapv2-2021.geomar.de/ (last access: 29 June 2021), which can also be accessed through https://www.glodap.info. This is similar in form and function to the GLODAPv2 adjustment table (Olsen et al., 2016) and includes a brief written justification for any adjustments applied.
4.1 Sensor and bottle data merge for salinity and oxygen
Table 4 summarizes the actions taken for the merging of the CTD and bottle data for salinity and oxygen. For 75 % of the 43 new cruises both CTD and bottle data of salinity were included in the original cruise data files, and for all these cruises the two data types were found to be consistent. This is similar to the GLODAPv2.2020 results. For oxygen, 63 % of the new cruises included both CTD O2 and bottle values, which is much more than for GLODAPv2.2020 (25 %) but comparable to GLODAPv2.2019. Having both CTD and bottle values in the data files is highly preferred as the information is valuable for quality control (bottle mistrips, leaking Niskin bottles, and oxygen sensor drift are among the issues that can be revealed). The extent to which the bottle data (i.e., OXYGEN in the individual cruise exchange files) is in reality mislabeled CTD data (i.e., should be CTDOXY) is uncertain. Regardless, the large majority of the CTD and bottle oxygen were consistent and did not need any further calibration of the CTD values (23 out of 27 cruises), while for four cruises no good fit could be obtained and their CTD O2 data are not included in the product.
4.2 Adjustment summary
The secondary QC has five possible outcomes which are summarized in Table 5, along with the corresponding codes that appear in the online adjustment table and that are also occasionally used as shorthand for decisions in the text below. Some cruises could not get full secondary QC. Specifically, in some cases data were too shallow or geographically too isolated for full and conclusive consistency analyses. A secondary QC flag has been included in the merged product files to enable their identification, with “0” used for variables and cruises not subjected to full secondary QC (corresponding to code −888 in Table 5) and “1” for variables and cruises that were subjected to full secondary QC. The secondary QC flags are assigned per cruise and variable, not for individual data points, and are independent of – and included in addition to – the primary (WOCE) QC flag. For example, interpolated (salinity, oxygen, nutrients) or calculated (TCO2, TAlk, pH) values, which have a primary QC flag of 0, may have a secondary QC flag of 1 if the measured data these values are based on have been subjected to full secondary QC. Conversely, individual data points may have a secondary QC flag of 0 even if their primary QC flag is 2 (good data). A 0 flag means that data were too shallow or geographically too isolated for consistency analyses or that these analyses were inconclusive but for which we have no reasons to believe that the data in question are of poor quality. Prominent examples for this version are the two new cruises in the Salish Sea: no data were available in this region in GLODAPv2.2020, which, combined with quite shallow sampling depths, rendered conclusive secondary QC impossible. As a consequence, most, but not all, of these data (some being excluded because of poor precision after consultation with the principal investigator) are included with a secondary QC flag of 0.
Table 5Possible outcomes of the secondary QC and their codes in the online adjustment table.
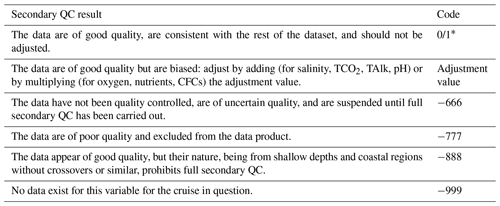
∗ The value of 0 is used for variables with additive adjustments (salinity, TCO2, TAlk, pH) and 1 for variables with multiplicative adjustments (for oxygen, nutrients, CFCs). This is mathematically equivalent to “no adjustment” in both cases.
Table 6Summary of secondary QC results for the 43 new cruises, in number of cruises per result and per variable.
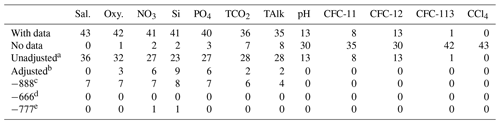
a The data are included in the data product file as is, with a secondary QC flag of 1. b The adjusted data are included in the data product file with a secondary QC flag of 1. c Data appear of good quality but have not been subjected to full secondary QC. They are included in data product with a secondary QC flag of 0. d Data are of uncertain quality and suspended until full secondary QC has been carried out; they are excluded from the data product. e Data are of poor quality and excluded from the data product.
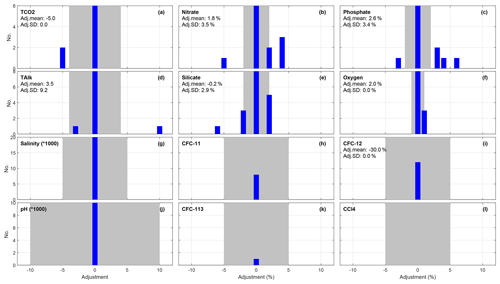
Figure 6Distribution of applied adjustments for each core variable that received secondary QC in micromoles per kilogram (µmol kg−1) for TCO2 and TAlk and unitless for salinity and pH (but multiplied by 1000 in both cases so a common x axis can be used), while for the other properties adjustments are given in percent ((adjustment ratio − 1) × 100). Gray areas depict the initial minimum adjustment limits. The figure includes numbers for data subjected to secondary quality control only. Note also that the y-axis scale is set to render the number of adjustments visible, so the bar showing zero offset (the 0 bar) for each variable is cut off (see Table 6 for these numbers).
The secondary QC actions for the 12 core variables and the distribution of applied adjustments are summarized in Table 6 and Fig. 6, respectively. For most variables only a small fraction of the data are adjusted: no salinity or pH data, 4.5 % of TCO2 and TAlk data, 7 % of oxygen data, 14 % of nitrate and phosphate data, and 21 % of silicate data. For the CFCs, no data required adjustment. Overall, the magnitudes of the various adjustments applied are also small. There is a larger fraction of data requiring adjustments to nutrients in GLODAPv2.2021 compared to GLODAPv2.2020. However, the tendency observed during the production of GLODAPv2.2019 and GLODAPv2.2020 remains, namely that the large majority of recent cruises are consistent with earlier releases of the GLODAP data product.
Only 13 out of the 43 new cruises included measured pH data, and none received an adjustment. However, we have not performed a new crossover and inversion analysis of all pH data in the northwestern Pacific (though such an analysis is planned for the next full update of GLODAP, i.e., GLODAPv3). Therefore, for now the conclusion from GLODAPv2.2020 remains, and some caution should be exercised if looking at trends in ocean pH in the northwestern Pacific using GLODAPv2.2020 or GLODAPv2.2021.
For the nutrients, adjustments were applied to maintain consistency with data included in GLODAPv2, GLODAPv2.2019, and GLODAPv2.2020. An alternative goal for the adjustments would be maintaining consistency with data from cruises that employed CRMNS to ensure accuracy of nutrient analyses. Such a strategy was adopted by Aoyama (2020) for preparation of the Global Nutrients Dataset 2013 (GND13) and is being considered for GLODAP as well. However, as this would require a re-evaluation of the entire dataset, this will not occur until the next full update of GLODAP, i.e., GLODAPv3. For now, we note the overall agreement between the adjustments applied in these two efforts (Aoyama, 2020) and that most disagreements appear to be related to cases to which no adjustments were applied in GLODAP. This can be related to the strategy followed for nutrients for GLODAPv2, in which data from GO-SHIP lines were considered more accurate than other data (Olsen et al., 2016). CRMNS are used for nutrients on most GO-SHIP lines.
Table 7Improvements resulting from quality control of the 43 new cruises per basin and for the global dataset. The values in the table are the weighted mean of the absolute offset of unadjusted and adjusted data versus GLODAPv2.2020. The total number of valid crossovers in the global ocean for the variable in question is n. The values in this table represent the inter-cruise consistency in the GLODAPv2.2021 product.
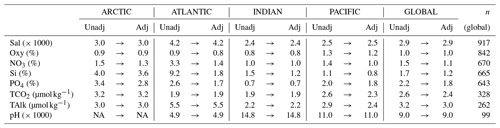
NA: not available.
The improvement in data consistency due to the secondary QC process is evaluated by comparing the weighted mean of the absolute offsets for all crossovers before and after the adjustments have been applied. This “consistency improvement” for core variables is presented in Table 7. The data for CFCs were omitted from these analyses for previously discussed reasons (Sect. 3.2.6). Globally, the improvement is modest. Considering the initial data quality, this result was expected. However, this does not imply that the data initially were consistent everywhere. Rather, for some regions and variables there are substantial improvements when the adjustments are applied. For example, silicate in the Atlantic Ocean shows a considerable improvement, and nutrients in general show improvements in almost all regions, including globally.
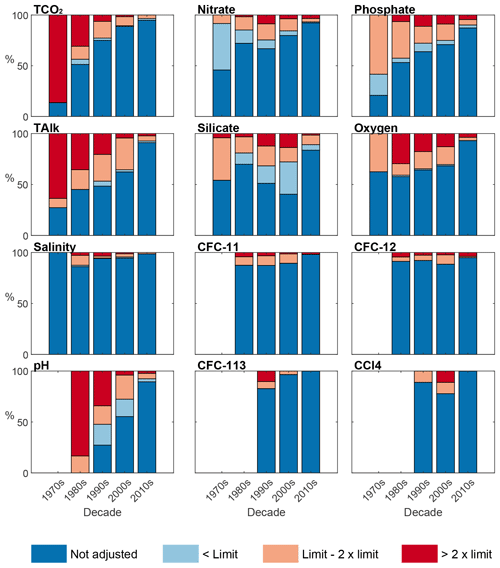
Figure 7Magnitude of applied adjustments relative to minimum adjustment limits (Table 3) per decade for the 989 cruises included in GLODAPv2.2021.
The various iterations of GLODAP provide insight into initial data quality covering more than 4 decades. Figure 7 summarizes the applied absolute adjustment magnitude per decade. These distributions are broadly unchanged compared to GLODAPv2.2020 (Fig. 8 in Olsen et al., 2020). Most TCO2 and TAlk data from the 1970s needed an adjustment, but this fraction steadily declines until only a small percentage is adjusted in recent years. This is encouraging and demonstrates the value of standardizing sampling and measurement practices (Dickson et al., 2007), the widespread use of CRMs (Dickson et al., 2003), application of best practices and standardized procedures, and instrument automation. The pH adjustment frequency also has a downward trend; however, there remain issues with the pH adjustments, and this is a topic for future development in GLODAP, with the support from the OCB (Ocean Carbonate System) Intercomparison Forum (OCSIF; https://www.us-ocb.org/ocean-carbonate-system-intercomparison-forum/, last accessed: 3 June 2021) working group (Álvarez et al., 2020). For the nutrients and oxygen, only the phosphate adjustment frequency decreases from decade to decade. However, we do note that the more recent data from the 2010s receive the fewest adjustments. This may reflect recent increased attention that seawater nutrient measurements have received through an operation manual (Becker et al., 2020; Hydes et al., 2010), availability of CRMNS (Aoyama et al., 2012; Ota et al., 2010), and the Scientific Committee on Oceanic Research (SCOR) working group #147, towards comparability of global oceanic nutrient data (COMPONUT). For silicate, the fraction of cruises receiving adjustments peaks in the 1990s and 2000s. This is related to the 2 % offset between US and Japanese cruises in the Pacific Ocean that was revealed during production of GLODAPv2 and discussed in Olsen et al. (2016). For salinity and the halogenated transient tracers, the number of adjusted cruises is small in every decade.
The GLODAPv2.2021 merged and adjusted data product is archived at NOAA NCEI at https://doi.org/10.25921/ttgq-n825 (Lauvset et al., 2021). These data and ancillary information are also available via our web pages https://www.glodap.info and https://www.ncei.noaa.gov/access/ocean-carbon-data-system/oceans/GLODAPv2_2021/ (last access: 7 July 2021). The data are available as comma-separated ascii files (*.csv) and as binary MATLAB files (*.mat) that use the open-source Hierarchical Data Format version 5 (HDF5). The data product is also made available as an Ocean Data View (ODV) file which can be easily explored using the “webODV Explore” online data service (https://explore.webodv.awi.de/, last access: 7 July 2021). Regional subsets are available for the Arctic, Atlantic, Pacific, and Indian oceans. There are no data overlaps between regional subsets, and each cruise exists in only one basin file even if data from that cruise crosses basin boundaries. The station locations in each basin file are shown in Fig. 8. The product file variables are listed in Table 1. A lookup table for matching the EXPOCODE of a cruise with GLODAP cruise number is provided with the data files, and a similar table is provided for matching the GLODAP cruise number with the data DOI. In the MATLAB files this information (EXPOCODE and DOI) is available as a cell array. A “known issues document” accompanies the data files and provides an overview of known errors and omissions in the data product files. It is regularly updated, and users are encouraged to inform us whenever any new issues are identified. It is critical that users consult this document whenever the data products are used.
The original cruise files, with updated flags determined during additional primary GLODAP QC, are available through the GLODAPv2.2021 cruise summary table (CST) hosted by NOAA NCEI: https://www.ncei.noaa.gov/access/ocean-carbon-data-system/oceans/GLODAPv2_2021/cruise_table_v2021.html (last access: 7 July 2021). Each of these files has been assigned a DOI, which is included in the data product files but not listed here. The CST also provides brief information on each cruise and access to metadata, cruise reports, and its adjustment table entry.
While GLODAPv2.2021 is made available without any restrictions, users of the data should adhere to the fair data use principles: for investigations that rely on a particular (set of) cruise(s), recognize the contribution of GLODAP data contributors by at least citing the articles where the data are described and, preferably, contacting principal investigators for exploring opportunities for collaboration and co-authorship. To this end, relevant articles and principal investigator names are provided in the cruise summary table. Contacting principal investigators comes with the additional benefit that the principal investigators often possess expert insight into the data and/or specific region under investigation. This can improve scientific quality and promote data sharing.
This paper should be cited in any scientific publications that result from usage of the product. Citations provide the most efficient means to track use, which is important for attracting funding to enable the preparation of future updates.
GLODAPv2.2021 is an update of GLODAPv2.2020. Data from 43 new cruises have been added to supplement the earlier release and extend temporal coverage by 1 year. GLODAP now includes 47 years, 1972–2020, of global interior ocean biogeochemical data from 989 cruises.
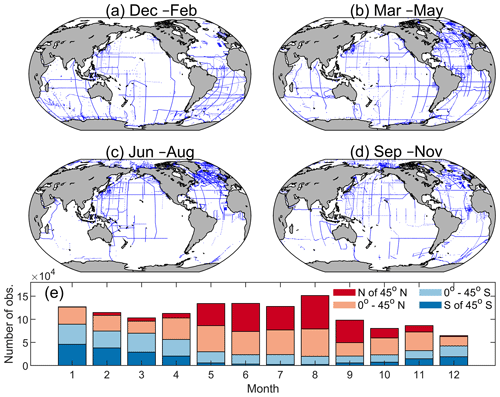
Figure 9Distribution of data in GLODAPv2.2021 in (a) December–February, (b) March–May, (c) June–August, and (d) September–November, as well as (e) number of observations for each month in four latitude bands.
The total number of data records is 1 334 269. Records with measurements for all 12 core variables (salinity, oxygen, nitrate, silicate, phosphate, TCO2, TAlk, pH, CFC-11, CFC-12, CFC-113, and CCl4) are very rare; only 2029 records have measured data for all 12 in the merged product file (interpolated and calculated data excluded). Requiring only two out of the four measured seawater CO2 chemistry variables, in addition to all the other core variables, brings the number of available records up to 9231, and so this is also very rare. A major limiting factor to having all core variables is the simultaneous availability of data for all four transient tracer species: only 26 137 records have measurements of CFC-11, CFC-12, CFC-113, and CCl4 while 422 029 have data for at least one of these (not considering availability of other core variables). A total of 423 544 records have measured data for two out of the three CO2 chemistry core variables. The number of records of measured fCO2 data is 33 844; note again that these data were not subjected to quality control. The number of records with measured data for salinity, oxygen, and nutrients is 832 566, while the number of records with salinity and oxygen data is 1 127 477. All of the above numbers concern measured data, not interpolated or calculated values. A total of 2 % (27 538) of the total data records do not have salinity. There are several reasons for this, the main one being the inability to vertically interpolate due to a separation that is too large (Sect. 3.3.2) between measured samples. Other reasons for missing salinity include salinity not being reported and missing depth or pressure. Note that there are slightly fewer records with fCO2 and all CFC data in GLODAPv2.2021 compared to GLODAPv2.2020. This is due to the removal of data with missing temperatures (Sect. 3.3.1).
Figure 9 illustrates the seasonal distribution of the data. As for previous versions there is a bias around summertime in the data in both hemispheres; most data are collected during April through November in the Northern Hemisphere, while most data are collected during November through April in the Southern Hemisphere. These tendencies are strongest for the poleward regions and reflect the harsh conditions during winter months which make fieldwork difficult. Figure 10 illustrates the distribution of data with depth. The upper 100 m is the best-sampled part of the global ocean, both in terms of number (Fig. 10a) and density (Fig. 10b) of observations. The number of observations steadily declines with depth. In part, this is caused by the reduction in ocean volume towards greater depths. Below 1000 m the density of observations stabilizes and even increases between 5000 and 6000 m; the latter is a zone where the volume of each depth surface decreases sharply (Weatherall et al., 2015). In the deep trenches, i.e., areas deeper than ∼ 6000 m, both number and density of observations are low.
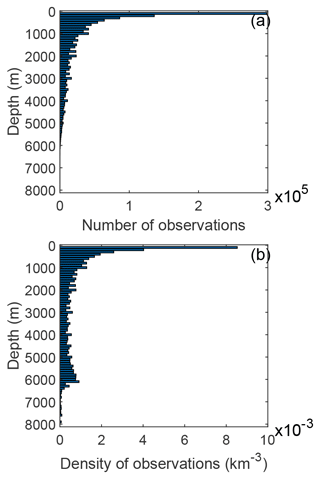
Figure 10Number (a) and density (b) of observations in 100 m depth layers. The latter was calculated by dividing the number of observations in each layer by its global volume calculated from ETOPO2 (National Geophysical Data Center/NESDIS/NOAA/U.S. Department of Commerce, 2006). For example, in the layer between 0 and 100 m there are on average 0.0075 observations per cubic kilometer. One observation is one water sampling point and has data for several variables.
Except for salinity and oxygen, the core data were collected exclusively through chemical analyses of collected water samples. The data of the 12 core variables were subjected to primary quality control to identify questionable or bad data points (outliers) and secondary quality control to identify systematic measurement biases. The data are provided in two ways: as a set of individual exchange-formatted original cruise data files with assigned WOCE flags and as globally and regionally merged data product files with adjustments applied to the data according to the outcome of the consistency analyses. Importantly, no adjustments were applied to data in the individual cruise files, while primary-QC changes were applied.
The consistency analyses were conducted by comparing the data from the 43 new cruises to the previous data product GLODAPv2.2020. Adjustments were only applied when the offsets were believed to reflect biases relative to the earlier data product release related to measurement calibration and/or data handling practices, and not to natural variability or anthropogenic trends. The adjustment table at https://glodapv2-2021.geomar.de/ (last access: 29 June 2021) lists all applied adjustments and provides a brief justification for each. The consistency analyses rely on deep ocean data (> 1500 or 2000 dbar depending on region), but supplementary CANYON-B and CONTENT analyses consider data below 500 dbar. Data consistency for cruises with exclusively shallow sampling was not examined. All new pH data for this version were comprehensively reviewed using crossover analysis, and none required adjustment. Regardless, full reanalysis of all available pH data, particularly in the North Pacific, will be conducted for GLODAPv3.
Secondary QC flags are included for the 12 core variables in the product files. These flags indicate whether (1) or not (0) the data successfully received secondary QC. A secondary QC flag of 0 does not by itself imply that the data are of lower quality than those with a flag of 1. It means these data have not been as thoroughly checked. For δ13C, the QC results by Becker et al. (2016) for the North Atlantic were applied, and a secondary QC flag was therefore added to this variable.
The primary WOCE QC flags in the product files are simplified (e.g., all questionable and bad data were removed). For salinity, oxygen, and the nutrients, any data flagged 0 are interpolated rather than measured. For TCO2, TAlk, pH, and fCO2 any data flags of 0 indicate that the values were calculated from two other measured seawater CO2 variables. Finally, while questionable (WOCE flag = 3) and bad (WOCE flag = 4) data have been excluded from the product files, some may have gone unnoticed through our analyses. Users are encouraged to report on any data that appear suspicious.
Based on the initial minimum adjustment limits and the improvement of the consistency resulting from the adjustments (Table 7), the data subjected to consistency analyses are believed to be consistent to better than 0.005 in salinity, 1 % in oxygen, 2 % in nitrate, 2 % in silicate, 2 % in phosphate, 4 µmol kg−1 in TCO2, 4 µmol kg−1 in TAlk, and 5 % for the halogenated transient tracers. For pH, the consistency among all data is estimated as 0.01–0.02, depending on region. As mentioned above, the included fCO2 data have not been subjected to quality control; therefore no consistency estimate is given for this variable. This should be conducted in future efforts.
Table A1Cruises included in GLODAPv2.2021 that did not appear in GLODAPv2.2020. Complete information on each cruise, such as variables included, and chief scientist and principal investigator names is provided in the cruise summary table at https://www.ncei.noaa.gov/access/ocean-carbon-data-system/oceans/GLODAPv2_2021/cruise_table_v2021.html (last access: 7 July 2021).
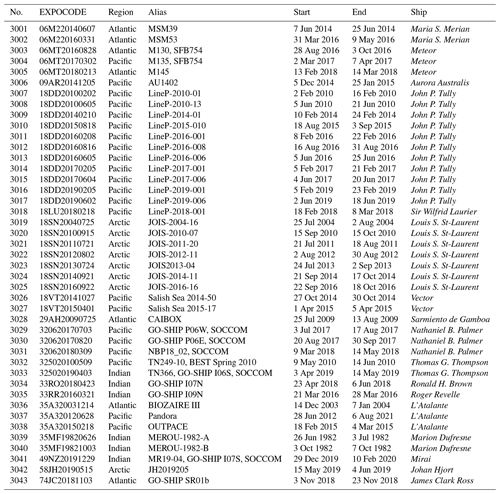
Former versions of this article were published on 15 August 2016, 25 September 2019, and 23 December 2020 and are available at https://doi.org/10.5194/essd-8-297-2016, https://doi.org/10.5194/essd-11-1437-2019, and https://doi.org/10.5194/essd-12-3653-2020.
The supplement related to this article is available online at: https://doi.org/10.5194/essd-13-5565-2021-supplement.
SKL and TT led the team that produced this update. RMK, AK, BP, SDJ, and MKK compiled the original data files. NL conducted the primary and secondary QC analyses. HCB conducted the CANYON-B and CONTENT analyses. CS manages the adjustment table e-infrastructure. AK maintains the GLODAPv2 web pages at NCEI/OCADS. PM prepared Python scripts for the merging of the data and works on converting all code used for the GLODAP effort to Python. All authors contributed to the interpretation of the secondary QC results and decisions on whether to apply actual adjustments. Many conducted ancillary QC analyses. SKL and AO wrote the manuscript with contributions from all authors.
Anton Velo is a topical editor for Earth System Science Data. The peer-review process was guided by an independent editor, and the authors also have no other competing interests to declare.
Publisher's note: Copernicus Publications remains neutral with regard to jurisdictional claims in published maps and institutional affiliations.
GLODAPv2.2021 would not have been possible without the effort of the many scientists who secured funding and dedicated time to collect and share the data that are included. Chief scientists at the various cruises and principal investigators for specific variables are listed in the online cruise summary table. The author team also want to thank the large GLODAP user community for useful input and notification about potential issues in the data products. Such input is invaluable and helps ensure that GLODAP maintains its high quality and consistency over time. Nico Lange was funded by EU Horizon 2020 through the EuroSea action (grant agreement 862626). Siv K. Lauvset acknowledges internal strategic funding from NORCE Climate. Leticia Cotrim da Cunha was supported by Prociencia/UERJ grant 2019–2021. Marta Álvarez was supported by IEO RADIALES and RADPROF projects. Peter J. Brown was partly funded by the UK Climate Linked Atlantic Sector Science (CLASS) NERC National Capability Long-term Single Centre Science Programme (grant NE/R015953/1). Anton Velo and Fiz F. Pérez were supported by the BOCATS2 Project (PID2019-104279GB-C21/AEI/10.13039/501100011033) funded by the Spanish Research Agency and contributing to WATER:iOS CSIC PTI. Rik Wanninkhof and Brendan R. Carter acknowledge the NOAA Global Observations and Monitoring Division (fund reference 100007298) and the Office of Oceanic and Atmospheric Research of NOAA. Henry C. Bittig gratefully acknowledges financial support from the BONUS INTEGRAL project (grant no. 03F0773A). Bronte Tilbrook was supported through the Australian Antarctic Program Partnership and the Integrated Marine Observing System. Mario Hoppema acknowledges EU Horizon 2020 action SO-CHIC (grant no. 821001). We acknowledge funding from the Initiative and Networking Fund of the Helmholtz Association through the project “Digital Earth” (ZT-0025). This paper is CICOES and PMEL contribution numbers 2021-1153 and 5253, respectively. This activity is supported by the International Ocean Carbon Coordination Project (IOCCP).
This research has been supported by EU Horizon 2020 through the EuroSea action (grant no. 862626), internal strategic funding from NORCE Climate, Prociencia/UERJ (grant 2019–2021), IEO RADIALES and RADPROF projects, the UK Climate Linked Atlantic Sector Science (CLASS) NERC National Capability Long-term Single Centre Science Programme (grant no. NE/R015953/1), the BOCATS2 (PID2019-104279GB-C21) project funded by MCIN/AEI/10.13039/501100011033 and contributing to WATER:iOS CSIC PTI, the NOAA Global Observations and Monitoring Division (fund reference 100007298), the Office of Oceanic and Atmospheric Research of NOAA, the BONUS INTEGRAL project (grant no. 03F0773A), the Australian Antarctic Program Partnership and the Integrated Marine Observing System, EU Horizon 2020 action SO-CHIC (grant no. 821001), and the Initiative and Networking Fund of the Helmholtz Association through the project “Digital Earth” (grant no. ZT-0025).
This paper was edited by David Carlson and reviewed by Matthew Humphreys and one anonymous referee.
Álvarez, M., Fajar, N. M., Carter, B. R., Guallart, E. F., Pérez, F. F., Woosley, R. J., and Murata, A.: Global ocean spectrophotometric pH assessment: consistent inconsistencies, Environ. Sci. Technol., 54, 10977–10988, https://doi.org/10.1021/acs.est.9b06932, 2020.
Amante, C. and Eakins, B. W.: ETOPO1 1 Arc-minute global relief model: procedures, data sources and analysis, NOAA Technical Memorandum NESDIS NGDC-24, National Geophysial Data Center, Marine Geology and Geophysics Division, Boulder, CO, USA, 2009.
Aoyama, M.: Global certified-reference-material- or reference-material-scaled nutrient gridded dataset GND13, Earth Syst. Sci. Data, 12, 487–499, https://doi.org/10.5194/essd-12-487-2020, 2020.
Aoyama, M., Ota, H., Kimura, M., Kitao, T., Mitsuda, H., Murata, A., and Sato, K.: Current status of homogeneity and stability of the reference materials for nutrients in Seawater, Anal. Sci., 28, 911–916, 2012.
Becker, M., Andersen, N., Erlenkeuser, H., Humphreys, M. P., Tanhua, T., and Körtzinger, A.: An internally consistent dataset of δ13C-DIC in the North Atlantic Ocean – NAC13v1, Earth Syst. Sci. Data, 8, 559–570, https://doi.org/10.5194/essd-8-559-2016, 2016.
Becker, S., Aoyama, M., Woodward, E. M. S., Bakker, K., Coverly, S., Mahaffey, C., and Tanhua, T.: GO-SHIP Repeat Hydrography Nutrient Manual: The Precise and Accurate Determination of Dissolved Inorganic Nutrients in Seawater, Using Continuous Flow Analysis Methods, Frontiers in Marine Science, 7, 908 pp., https://doi.org/10.3389/fmars.2020.581790, 2020.
Bittig, H. C., Steinhoff, T., Claustre, H., Fiedler, B., Williams, N. L., Sauzède, R., Körtzinger, A., and Gattuso, J.-P.: An alternative to static climatologies: Robust estimation of open ocean CO2 variables and nutrient concentrations from T, S, and O2 data using Bayesian Neural Networks, Frontiers in Marine Science, 5, 328, https://doi.org/10.3389/fmars.2018.00328, 2018.
Bockmon, E. E. and Dickson, A. G.: An inter-laboratory comparison assessing the quality of seawater carbon dioxide measurements, Mar. Chem., 171, 36–43, 2015.
Brakstad, A., Våge, K., Håvik, L., and Moore, G. W. K.: Water Mass Transformation in the Greenland Sea during the Period 1986.-2016, J. Phys. Oceanogr., 49, 121–140, 2019.
Bryden, H. L.: New polynomials for thermal-expansion, adiabatic temperature gradient and potential temperature of sea-water, Deep-Sea Res., 20, 401–408, 1973.
Bu, X. and Warner, M. J.: Solubility of chlorofluorocarbon-113 in water and seawater, Deep-Sea Res. Pt. I, 42, 1151–1161, 1995.
Bullister, J. L. and Wisegarver, D. P.: The solubility of carbon tetrachloride in water and seawater, Deep-Sea Res. Pt. I, 45, 1285–1302, 1998.
Bullister, J. L., Wisegarver, D. P., and Menzia, F. A.: The solubility of sulfur hexafluoride in water and seawater, Deep-Sea Res. Pt. I, 49, 175–187, 2002.
Carter, B. R., Feely, R. A., Williams, N. L., Dickson, A. G., Fong, M. B., and Takeshita, Y.: Updated methods for global locally interpolated estimation of alkalinity, pH, and nitrate, Limnol. Oceanogr.-Meth., 16, 119–131, 2018.
Cheng, L. J., Trenberth, K. E., Fasullo, J., Boyer, T., Abraham, J., and Zhu, J.: Improved estimates of ocean heat content from 1960 to 2015, Sci. Adv., 3, e1601545, https://doi.org/10.1126/sciadv.1601545, 2017.
Cheng, L. J., Abraham, J., Zhu, J., Trenberth, K. E., Fasullo, J., Boyer, T., Locarnini, R., Zhang, B., Yu, F. J., Wan, L. Y., Chen, X. R., Song, X. Z., Liu, Y. L., and Mann, M. E.: Record-setting ocean warmth continued in 2019, Adv. Atmos. Sci, 37, 137–142, 2020.
Dickson, A. G.: Standard potential of the reaction: AgCl(s) + 12H2(g) = Ag(s) + HCl(aq), and and the standard acidity constant of the ion in synthetic sea water from 273.15 to 318.15 K, J. Chem. Thermodyn., 22, 113–127, 1990.
Dickson, A. G., Afghan, J. D., and Anderson, G. C.: Reference materials for oceanic CO2 analysis: a method for the certification of total alkalinity, Mar. Chem., 80, 185–197, 2003.
Dickson, A. G., Sabine, C. L., and Christian, J. R.: Guide to Best Practices for Ocean CO2 measurements, PICES Special Publication 3, North Pacific Marine Science Organization, 191 pp., 2007.
Falck, E. and Olsen, A.: Nordic Seas dissolved oxygen data in CARINA, Earth Syst. Sci. Data, 2, 123–131, https://doi.org/10.5194/essd-2-123-2010, 2010.
Fofonoff, N. P.: Computation of potential temperature of seawater for an arbitrary reference pressure, Deep-Sea Res., 24, 489–491, 1977.
Fong, M. B. and Dickson, A. G.: Insights from GO-SHIP hydrography data into the thermodynamic consistency of CO2 system measurements in seawater, Mar. Chem., 211, 52–63, https://doi.org/10.1016/j.marchem.2019.03.006, 2019.
Friedlingstein, P., Jones, M. W., O'Sullivan, M., Andrew, R. M., Hauck, J., Peters, G. P., Peters, W., Pongratz, J., Sitch, S., Le Quéré, C., Bakker, D. C. E., Canadell, J. G., Ciais, P., Jackson, R. B., Anthoni, P., Barbero, L., Bastos, A., Bastrikov, V., Becker, M., Bopp, L., Buitenhuis, E., Chandra, N., Chevallier, F., Chini, L. P., Currie, K. I., Feely, R. A., Gehlen, M., Gilfillan, D., Gkritzalis, T., Goll, D. S., Gruber, N., Gutekunst, S., Harris, I., Haverd, V., Houghton, R. A., Hurtt, G., Ilyina, T., Jain, A. K., Joetzjer, E., Kaplan, J. O., Kato, E., Klein Goldewijk, K., Korsbakken, J. I., Landschützer, P., Lauvset, S. K., Lefèvre, N., Lenton, A., Lienert, S., Lombardozzi, D., Marland, G., McGuire, P. C., Melton, J. R., Metzl, N., Munro, D. R., Nabel, J. E. M. S., Nakaoka, S.-I., Neill, C., Omar, A. M., Ono, T., Peregon, A., Pierrot, D., Poulter, B., Rehder, G., Resplandy, L., Robertson, E., Rödenbeck, C., Séférian, R., Schwinger, J., Smith, N., Tans, P. P., Tian, H., Tilbrook, B., Tubiello, F. N., van der Werf, G. R., Wiltshire, A. J., and Zaehle, S.: Global Carbon Budget 2019, Earth Syst. Sci. Data, 11, 1783–1838, https://doi.org/10.5194/essd-11-1783-2019, 2019.
Fröb, F., Olsen, A., Våge, K., Moore, G. W. K., Yashayaev, I., Jeansson, E., and Rajasakaren, B.: Irminger Sea deep convection injects oxygen and anthropogenic carbon to the ocean interior, Nat. Commun., 7, 13244, https://doi.org/10.1038/ncomms13244, 2016.
Garcia, H. E. and Gordon, L. I.: Oxygen solubility in seawater – Better fitting equations, Limnol. Oceanogr., 37, 1307–1312, 1992.
Gordon, A. L.: Deep Antarctic covection west of Maud Rise, J. Phys. Oceanogr., 8, 600–612, 1978.
Gruber, N., Clement, D., Carter, B. R., Feely, R. A., van Heuven, S., Hoppema, M., Ishii, M., Key, R. M., Kozyr, A., Lauvset, S. K., Lo Monaco, C., Mathis, J. T., Murata, A., Olsen, A., Perez, F. F., Sabine, C. L., Tanhua, T., and Wanninkhof, R.: The oceanic sink for anthropogenic CO2 from 1994 to 2007, Science, 363, 1193–1199, 2019.
Hood, E. M., Sabine, C. L., and Sloyan, B. M. (Eds.).: The GO-SHIP hydrography manual: A collection of expert reports and guidelines, IOCCP Report Number 14, ICPO Publication Series Number 134, available at: http://www.go-ship.org/HydroMan.html (last access: 16 October 2020), 2010.
Hydes, D. J., Aoyama, A., Aminot, A., Bakker, K., Becker, S., Coverly, S., Daniel, A., Dickson, A. G., Grosso, O., Kerouel, R., van Ooijen, J., Sato, K., Tanhua, T., Woodward, E. M. S., and Zhang, J.-Z.: Determination of dissolved nutrients in seawater with high precision and intercomparability using gas-segmented continuous flow analysers, in: The GO SHIP Repeat Hydrography Manual: A Collection of Expert Reports and Guidelines, edited by: Hood, E. M., Sabine, C., and Sloyan, B. M., IOCCP Report Number 14, ICPO Publication Series Number 134, ICPO, available at: http://www.go-ship.org/HydroMan.html (last access: 29 November 2021), 2010.
Jackett, D. R. and McDougall, T. J.: A neutral density variable for the world's oceans, J. Phys. Oceanogr., 27, 237–263, 1997.
Jeansson, E., Olsson, K. A., Tanhua, T., and Bullister, J. L.: Nordic Seas and Arctic Ocean CFC data in CARINA, Earth Syst. Sci. Data, 2, 79–97, https://doi.org/10.5194/essd-2-79-2010, 2010.
Jenkins, W. J., Doney, S. C., Fendrock, M., Fine, R., Gamo, T., Jean-Baptiste, P., Key, R., Klein, B., Lupton, J. E., Newton, R., Rhein, M., Roether, W., Sano, Y., Schlitzer, R., Schlosser, P., and Swift, J.: A comprehensive global oceanic dataset of helium isotope and tritium measurements, Earth Syst. Sci. Data, 11, 441–454, https://doi.org/10.5194/essd-11-441-2019, 2019.
Jutterström, S., Anderson, L. G., Bates, N. R., Bellerby, R., Johannessen, T., Jones, E. P., Key, R. M., Lin, X., Olsen, A., and Omar, A. M.: Arctic Ocean data in CARINA, Earth Syst. Sci. Data, 2, 71–78, https://doi.org/10.5194/essd-2-71-2010, 2010.
Key, R. M., Kozyr, A., Sabine, C. L., Lee, K., Wanninkhof, R., Bullister, J. L., Feely, R. A., Millero, F. J., Mordy, C., and Peng, T. H.: A global ocean carbon climatology: Results from Global Data Analysis Project (GLODAP), Global Biogeochem. Cy., 18, GB4031, https://doi.org/10.1029/2004GB002247, 2004.
Key, R. M., Tanhua, T., Olsen, A., Hoppema, M., Jutterström, S., Schirnick, C., van Heuven, S., Kozyr, A., Lin, X., Velo, A., Wallace, D. W. R., and Mintrop, L.: The CARINA data synthesis project: introduction and overview, Earth Syst. Sci. Data, 2, 105–121, https://doi.org/10.5194/essd-2-105-2010, 2010.
Lauvset, S. K. and Tanhua, T.: A toolbox for secondary quality control on ocean chemistry and hydrographic data, Limnol. Oceanogr.-Meth., 13, 601–608, 2015.
Lauvset, S. K., Key, R. M., Olsen, A., van Heuven, S., Velo, A., Lin, X., Schirnick, C., Kozyr, A., Tanhua, T., Hoppema, M., Jutterström, S., Steinfeldt, R., Jeansson, E., Ishii, M., Perez, F. F., Suzuki, T., and Watelet, S.: A new global interior ocean mapped climatology: the 1∘ × 1∘ GLODAP version 2, Earth Syst. Sci. Data, 8, 325–340, https://doi.org/10.5194/essd-8-325-2016, 2016.
Lauvset, S. K., Carter, B. R., Perez, F. F., Jiang, L.-Q., Feely, R. A., Velo, A., and Olsen, A.: Processes Driving Global Interior Ocean pH Distribution, Global Biogeochem. Cy., 34, e2019GB006229, https://doi.org/10.1029/2019gb006229, 2020.
Lauvset, S. K., Lange, N., Tanhua, T., Bittig, H. C., Olsen, A., Kozyr, A., Álvarez, M., Becker, S., Brown, P. J., Carter, B. R., Cotrim da Cunha, L., Feely, R. A., van Heuven, S., Hoppema, M., Ishii, M., Jeansson, E., Jutterström, S., Jones, S. D., Karlsen, M. K., Lo Monaco, C., Michaelis, P., Murata, A., Pérez, F. F., Pfeil, B., Schirnick, C., Steinfeldt, R., Suzuki, T., Tilbrook, B., Velo, A., Wanninkhof, R., Woosley, R. J., and Key, R. M.: Global Ocean Data Analysis Project version 2.2021 (GLODAPv2.2021) (NCEI Accession 0237935), NOAA National Centers for Environmental Information [data set], https://doi.org/10.25921/ttgq-n825, 2021.
Lee, K., Kim, T. W., Byrne, R. H., Millero, F. J., Feely, R. A., and Liu, Y. M.: The universal ratio of boron to chlorinity for the North Pacific and North Atlantic oceans, Geochim. Cosmochim. Ac., 74, 1801–1811, https://doi.org/10.1016/j.gca.2009.12.027, 2010.
Lewis, E. and Wallace, D. W. R.: Program developed for CO2 system calculations, ORNL/CDIAC-105, Carbon Dioxide Information Analysis Center, Oak Ridge National Laboratory, Oak Ridge, TN, USA, 1998.
Lueker, T. J., Dickson, A. G., and Keeling, C. D.: Ocean pCO2 calculated from dissolved inorganic carbon, alkalinity, and equations for K-1 and K-2: validation based on laboratory measurements of CO2 in gas and seawater at equilibrium, Mar. Chem., 70, 105–119, 2000.
National Geophysical Data Center/NESDIS/NOAA/U.S. Department of Commerce: TerrainBase, Global 5 Arc-minute Ocean Depth and Land Elevation from the US National Geophysical Data Center (NGDC), Research Data Archive at the National Center for Atmospheric Research, Computational and Information Systems Laboratory [dataset], https://doi.org/10.5065/E08M-4482, 1995.
National Geophysical Data Center/NESDIS/NOAA/U.S. Department of Commerce: ETOPO2, Global 2 Arc-minute Ocean Depth and Land Elevation from the US National Geophysical Data Center (NGDC), Research Data Archive at the National Center for Atmospheric Research, Computational and Information Systems Laboratory [dataset], https://doi.org/10.5065/D6668B75, 2006.
Olsen, A., Key, R. M., van Heuven, S., Lauvset, S. K., Velo, A., Lin, X., Schirnick, C., Kozyr, A., Tanhua, T., Hoppema, M., Jutterström, S., Steinfeldt, R., Jeansson, E., Ishii, M., Pérez, F. F., and Suzuki, T.: The Global Ocean Data Analysis Project version 2 (GLODAPv2) – an internally consistent data product for the world ocean, Earth Syst. Sci. Data, 8, 297–323, https://doi.org/10.5194/essd-8-297-2016, 2016.
Olsen, A., Lange, N., Key, R. M., Tanhua, T., Álvarez, M., Becker, S., Bittig, H. C., Carter, B. R., Cotrim da Cunha, L., Feely, R. A., van Heuven, S., Hoppema, M., Ishii, M., Jeansson, E., Jones, S. D., Jutterström, S., Karlsen, M. K., Kozyr, A., Lauvset, S. K., Lo Monaco, C., Murata, A., Pérez, F. F., Pfeil, B., Schirnick, C., Steinfeldt, R., Suzuki, T., Telszewski, M., Tilbrook, B., Velo, A., and Wanninkhof, R.: GLODAPv2.2019 – an update of GLODAPv2, Earth Syst. Sci. Data, 11, 1437–1461, https://doi.org/10.5194/essd-11-1437-2019, 2019.
Olsen, A., Lange, N., Key, R. M., Tanhua, T., Bittig, H. C., Kozyr, A., Álvarez, M., Azetsu-Scott, K., Becker, S., Brown, P. J., Carter, B. R., Cotrim da Cunha, L., Feely, R. A., van Heuven, S., Hoppema, M., Ishii, M., Jeansson, E., Jutterström, S., Landa, C. S., Lauvset, S. K., Michaelis, P., Murata, A., Pérez, F. F., Pfeil, B., Schirnick, C., Steinfeldt, R., Suzuki, T., Tilbrook, B., Velo, A., Wanninkhof, R., and Woosley, R. J.: An updated version of the global interior ocean biogeochemical data product, GLODAPv2.2020, Earth Syst. Sci. Data, 12, 3653–3678, https://doi.org/10.5194/essd-12-3653-2020, 2020.
Ota, H., Mitsuda, H., Kimura, M., and Kitao, T.: Reference materials for nutrients in seawater: Their development and present homogenity and stability, in: Comparability of nutrients in the world's oceans, edited by: Aoyama, A., Dickson, A. G., Hydes, D. J., Murata, A., Oh, J. R., Roose, P., and Woodward, E. M. S., Mother Tank, Tsukuba, Japan, 2010.
Sabine, C., Key, R. M., Kozyr, A., Feely, R. A., Wanninkhof, R., Millero, F. J., Peng, T.-H., Bullister, J. L., and Lee, K.: Global Ocean Data Analysis Project (GLODAP): Results and Data, ORNL/CDIAC-145, NDP-083, Carbon Dioxide Information Analysis Center, Oak Ridge National Laboratory, U.S. Department of Energy, Oak Ridge, TN, USA, 2005.
Sloyan, B. M., Wanninkhof, R., Kramp, M., Johnson, G. C., Talley, L. D., Tanhua, T., McDonagh, E., Cusack, C., O'Rourke, E., McGovern, E., Katsumata, K., Diggs, S., Hummon, J., Ishii, M., Azetsu-Scott, K., Boss, E., Ansorge, I., Perez, F. F., Mercier, H., Williams, M. J. M., Anderson, L., Lee, J. H., Murata, A., Kouketsu, S., Jeansson, E., Hoppema, M., and Campos, E.: The Global Ocean Ship-Based Hydrographic Investigations Program (GO-SHIP): A Platform for Integrated Multidisciplinary Ocean Science, Frontiers in Marine Science, 6, 445 pp., https://doi.org/10.3389/fmars.2019.00445, 2019.
Steinfeldt, R., Tanhua, T., Bullister, J. L., Key, R. M., Rhein, M., and Köhler, J.: Atlantic CFC data in CARINA, Earth Syst. Sci. Data, 2, 1–15, https://doi.org/10.5194/essd-2-1-2010, 2010.
Suzuki, T., Ishii, M., Aoyama, A., Christian, J. R., Enyo, K., Kawano, T., Key, R. M., Kosugi, N., Kozyr, A., Miller, L. A., Murata, A., Nakano, T., Ono, T., Saino, T., Sasaki, K., Sasano, D., Takatani, Y., Wakita, M., and Sabine, C.: PACIFICA Data Synthesis Project, ORNL/CDIAC-159, NDP-092, Carbon Dioxide Information Analysis Center, Oak Ridge National Laboratory, U.S. Department of Energy, Oak Ridge, TN, USA, 2013.
Swift, J.: Reference-quality water sample data: Notes on aquisition, record keeping, and evaluation, in: The GO-SHIP Repeat Hydrography Manual: A Collection of Expert Reports and Guidelines, edited by: Hood, E. M., Sabine, C., and Sloyan, B. M., IOCCP Report Number 14, ICPO Publication Series Number 134, ICPO, available at: http://www.go-ship.org/HydroMan.html (last access: 29 November 2021), 2010.
Swift, J. and Diggs, S. C.: Description of WHP exchange format for CTD/Hydrographic data, CLIVAR and Carbon Hydrographic Data Office, UCSD Scripps Institution of Oceanography, San Diego, Ca, US, 2008.
Takeshita, Y., Johnson, K. S., Coletti, L. J., Jannasch, H. W., Walz, P. M., and Warren, J. K.: Assessment of pH dependent errors in spectrophotometric pH measurements of seawater, Mar. Chem., 223, 103801, https://doi.org/10.1016/j.marchem.2020.103801, 2020.
Talley, L. D., Feely, R. A., Sloyan, B. M., Wanninkhof, R., Baringer, M. O., Bullister, J. L., Carlson, C. A., Doney, S. C., Fine, R. A., Firing, E., Gruber, N., Hansell, D. A., Ishii, M., Johnson, G. C., Katsumata, K., Key, R. M., Kramp, M., Langdon, C., Macdonald, A. M., Mathis, J. T., McDonagh, E. L., Mecking, S., Millero, F. J., Mordy, C. W., Nakano, T., Sabine, C. L., Smethie, W. M., Swift, J. H., Tanhua, T., Thurnherr, A. M., Warner, M. J., and Zhang, J. Z.: Changes in ocean heat, carbon content, and ventilation: A review of the first decade of GO-SHIP global repeat hydrography, Annu. Rev. Mar. Sci., 8, 185–215, 2016.
Tanhua, T., van Heuven, S., Key, R. M., Velo, A., Olsen, A., and Schirnick, C.: Quality control procedures and methods of the CARINA database, Earth Syst. Sci. Data, 2, 35–49, https://doi.org/10.5194/essd-2-35-2010, 2010.
Tanhua, T., Lauvset, S. K., Lange, N., Olsen, A., Álvarez, M., Diggs, S., Bittig, H. C., Brown, P. J., Carter, B. R., da Cunha, L. C., Feely, R. A., Hoppema, M., Ishii, M., Jeansson, E., Kozyr, A., Murata, A., Pérez, F. F., Pfeil, B., Schirnick, C., Steinfeldt, R., Telszewski, M., Tilbrook, B., Velo, A., Wanninkhof, R., Burger, E., O'Brien, K., and Key, R. M.: A vision for FAIR ocean data products, Communications Earth & Environment, 2, 136, https://doi.org/10.1038/s43247-021-00209-4, 2021.
UNESCO: Tenth report of the joint panel on oceanographic tables and standards, UNESCO Technical Paper in Marine Science, 36, 13–21, Sidney, B.C., Canada, 1981.
Uppström, L. R.: Boron/Chlorinity ratio of deep-sea water from Pacific Ocean, Deep-Sea Res,, 21, 161–162, 1974.
van Heuven, S., Pierrot, D., Rae, J. W. B., Lewis, E., and Wallace, D. W. R.: MATLAB program developed for CO2 system calculations, ORNL/CDIAC-105b, Carbon Dioxide Information Analysis Center, Oak Ridge National Laboratory, Oak Ridge, TN, USA, 2011.
Warner, M. J. and Weiss, R. F.: Solubilities of chlorofluorocarbon-11 and chlorofluorocarbon-12 in water and seawater, Deep-Sea Res., 32, 1485–1497, 1985.
Weatherall, P., Marks, K. M., Jakobsson, M., Schmitt, T., Tani, S., Arndt, J. E., Rovere, M., Chayes, D., Ferrini, V., and Wigley, R.: A new digital bathymetric model of the world's oceans, Earth Space Sci., 2, 331–345, https://doi.org/10.1002/2015EA000107, 2015.
Wilkinson, M. D., Dumontier, M., Aalbersberg, I. J., Appleton, G., Axton, M., Baak, A., Blomberg, N., Boiten, J.-W., da Silva Santos, L. B., Bourne, P. E., Bouwman, J., Brookes, A. J., Clark, T., Crosas, M., Dillo, I., Dumon, O., Edmunds, S., Evelo, C. T., Finkers, R., Gonzalez-Beltran, A., Gray, A. J. G., Groth, P., Goble, C., Grethe, J. S., Heringa, J., 't Hoen, P. A. C., Hooft, R., Kuhn, T., Kok, R., Kok, J., Lusher, S. J., Martone, M. E., Mons, A., Packer, A. L., Persson, B., Rocca-Serra, P., Roos, M., van Schaik, R., Sansone, S.-A., Schultes, E., Sengstag, T., Slater, T., Strawn, G., Swertz, M. A., Thompson, M., van der Lei, J., van Mulligen, E., Velterop, J., Waagmeester, A., Wittenburg, P., Wolstencroft, K., Zhao, J., and Mons, B.: The FAIR Guiding Principles for scientific data management and stewardship, Scientific Data, 3, 160018, https://doi.org/10.1038/sdata.2016.18, 2016.
Yashayaev, I. and Loder, J. W.: Further intensification of deep convection in the Labrador Sea in 2016, Geophys. Res. Lett., 44, 1429–1438, https://doi.org/10.1002/2016GL071668, 2017.
- Abstract
- Introduction
- Key features of the update
- Methods
- Secondary quality control results and adjustments
- Data availability
- Summary
- Appendix A: Supplementary tables
- Note on former version
- Author contributions
- Competing interests
- Disclaimer
- Acknowledgements
- Financial support
- Review statement
- References
- Supplement
- Article
(4622 KB) - Full-text XML
- Abstract
- Introduction
- Key features of the update
- Methods
- Secondary quality control results and adjustments
- Data availability
- Summary
- Appendix A: Supplementary tables
- Note on former version
- Author contributions
- Competing interests
- Disclaimer
- Acknowledgements
- Financial support
- Review statement
- References
- Supplement