the Creative Commons Attribution 4.0 License.
the Creative Commons Attribution 4.0 License.
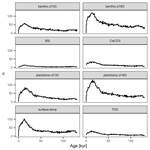
Integrating palaeoclimate time series with rich metadata for uncertainty modelling: strategy and documentation of the PalMod 130k marine palaeoclimate data synthesis
Olivier Cartapanis
Michael Langner
Nick McKay
Stefan Mulitza
Anne Strack
Michal Kucera
Palaeoclimate data hold the unique promise of providing a long-term perspective on climate change and as such can serve as an important benchmark for climate models. However, palaeoclimate data have generally been archived with insufficient standardisation and metadata to allow for transparent and consistent uncertainty assessment in an automated way. Thanks to improved computation capacity, transient palaeoclimate simulations are now possible, calling for data products containing multi-parameter time series rather than information on a single parameter for a single time slice. Efforts are underway to simulate a complete glacial–interglacial cycle using general circulation models (https://www.palmod.de/, last access: 6 May 2020), and to confront these simulations with palaeoclimate data, we have compiled a multi-parameter marine palaeoclimate data synthesis that contains time series spanning 0 to 130 000 years ago. We present the first version of the data product that focuses exclusively on time series for which a robust chronology based on benthic foraminifera δ18O and radiocarbon dating is available. The product contains 896 time series of eight palaeoclimate parameters from 143 individual sites, each associated with rich metadata, age–depth model ensembles, and information to refine and update the chronologies. This version contains 205 time series of benthic foraminifera δ18O; 169 of benthic foraminifera δ13C; 131 of seawater temperature; 174 and 119 of planktonic foraminifera δ18O and δ13C; and 44, 38 and 16 of carbonate, organic carbon and biogenic silica content, respectively. The data product is available in three formats (R, LiPD and netCDF) facilitating use across different software and operating systems and can be downloaded at https://doi.org/10.1594/PANGAEA.908831 (Jonkers et al., 2019). This data descriptor presents our data synthesis strategy and describes the contents and format of the data product in detail. It ends with a set of recommendations for data archiving.
- Article
(1500 KB) -
Supplement
(73 KB) - BibTeX
- EndNote
Global climate has varied dramatically over the last glacial–interglacial cycle. Since the previous interglacial (approximately 130 000 years ago) the Earth had slowly been cooling until the Last Glacial Maximum (LGM; approximately 21 000 years ago). This cooling was associated with the growth of massive ice sheets in North America and Eurasia, leading to a sea level drop of about 120 m (Waelbroeck et al., 2002) and pronounced climate variability on millennial timescales (Voelker and workshop participants, 2002). From the LGM, the Earth warmed rapidly until the onset of the current relatively stable warm period, the Holocene (Shakun et al., 2012). The ultimate cause of the large-scale variations in the Earth's climate is changes in the orbit of the Earth around the Sun (Hays et al., 1976). However, complex feedback and non-linear mechanisms, involving ocean (atmosphere, cryosphere) circulation and biogeochemical cycles, are required to explain how slow changes in the orbital configuration led to the observed evolution of global climate and how these processes led to the manifestation of abrupt climate change.
For these reasons the last glacial–interglacial cycle has been a key target for palaeoclimate modelling. Initially this only involved equilibrium simulations for key time slices, such as the LGM, or transient simulations for short periods, such as the last millennium. The motivation to simulate past climate states is given by the possibility of palaeoclimate data serving as a benchmark for the models. Indeed, this possibility contributed to the development of large palaeodata syntheses (CLIMAP project members, 1981; MARGO project, 2009). The time-slice modelling approach is still being pursued; for example in phase 4 of the Paleoclimate Modelling Intercomparison Project (PMIP), four of the five target intervals fall into the time frame of the last glacial cycle (Kageyama et al., 2018). However, with increasing computing power, the focus is now shifting towards transient climate simulations (Liu et al., 2009; Latif et al., 2016), and the simulation of the last deglaciation is now also considered in the PMIP protocol (Ivanovic et al., 2016).
This development calls for a different type of palaeodata synthesis, with its focus on time series rather than on time slices. Time series of climate data are needed to evaluate aspects of transient simulations that are not available in equilibrium simulations, such as rates of change, phase relationships and spectral properties of climate variability. It is also clear that an evaluation in multi-parameter space using different aspects of the climate system and multiple proxies will be more powerful and diagnostic (Kurahashi-Nakamura et al., 2017), calling for multi-parameter synthesis products.
Observations of the evolution of past climate are based on proxies (measurable approximations of climate-related variables) and hence are, by definition, indirect. Comparison of proxy-based reconstructions with climate model simulations is therefore far from straightforward, as discrepancies may arise from both model and proxy uncertainty. Proxy uncertainty derives from reconstruction uncertainty (related to calibration, recording bias, archive specifics and instrumental approach) and chronological uncertainty. The latter is particularly relevant to the comparison of transient climate change, and chronological uncertainty thus requires a comprehensive treatment in data syntheses of palaeoclimate time series.
Accounting for proxy uncertainties in a comprehensive and transparent manner requires not only expert knowledge but also the availability of extensive metadata in addition to the proxy data. However, due to a lack of standardisation and inconsistent archiving of metadata, synthesising palaeoclimate data in a way that allows for robust uncertainty assessment remains challenging and time consuming. Efforts are underway to alleviate these challenges. The largest palaeoclimate data repositories (World Data Service for Paleoclimatology, operated by the national centres for environmental information (NCEIs) at NOAA, and PANGAEA) are both striving for more standardisation and to store data in (more) machine-readable formats. In addition, standardisation is progressing through the use of existing data formats from other communities (netCDF; Langner and Mulitza, 2019) as well as the implementation of new data formats specifically targeted to palaeodata (Linked Paleo Data (LiPD); McKay and Emile-Geay, 2016). At the same time there is ongoing discussion on data and metadata requirements and standards (Khider et al., 2019). Traceability of datasets is also improved through data citations, not only ensuring that data producers receive proper credit for their work but also allowing for better linking of different datasets. Nevertheless, these initiatives have only recently been emerging and the majority of the palaeoclimate data remains inconsistently formatted, non-standardised and scattered over various data repositories. The need for synthesis products and documentation of potential synthesis approaches is therefore as large as ever.
Here we present the first version of a new multi-proxy marine palaeoclimate data synthesis that covers the past 130 000 years developed within the German climate modelling initiative PalMod (Latif et al., 2016). We focus on the ocean as it is a large reservoir of heat and CO2 and allows for global coverage with consistent chronological control. This synthesis goes beyond the time frame of many existing multi-proxy and multi-parameter data syntheses (PAGES2k Consortium et al., 2017; Routson et al., 2019), expands existing data products that provide long palaeoclimate time series to multiple parameters (Shakun et al., 2012; Marcott et al., 2013; Peterson and Lisiecki, 2018; Snyder, 2016), and is based on a strategy of semi-automated data harvesting (Cartapanis et al., 2016). This version of the synthesis contains data on nine climate-sensitive parameters: benthic and planktonic foraminifera stable oxygen and carbon isotopes, seawater temperature, radiocarbon and bulk sediment carbonate, organic carbon, and biogenic silica content.
In this paper we describe our synthesis approach, the contents and structure of version 1.0.0 of the data, plans for future updates, and recommendations for archiving new data and retrieving dark data in a way that allows for optimal future reuse. The data product is intended to be used to investigate spatio-temporal changes in a multi-parameter domain. Thanks to rich metadata that allow for the rigorous quantification of reconstruction uncertainties, we also envision that this data product will provide the building blocks for intelligent palaeoclimate data model comparison (Weitzel et al., 2019), for instance through proxy system modelling (Dolman and Laepple, 2018) or data assimilation (Breitkreuz et al., 2019).
The structure of this data descriptor is as follows. Section 2 describes the synthesis strategy, including the data discovery approach, standardisation and age modelling. In Sect. 3 we provide general information on palaeoclimate proxies from marine-sediment archives that is used to guide the metadata selection. Section 4 details the structure of the database, and the contents of version 1.0.0 are outlined in Sect. 5. The formats of the data product, future plans and versioning are described in Sects. 6 and 7. Section 8 describes where the data can be accessed. In the last section, Sect. 9, we reflect on the data synthesis effort and provide recommendations for data archiving and data rescue.
Our data product focuses on time series from marine-sediment archives. A single marine-sediment archive (sediment core) can be used for measurements of different parameters, each providing information on different aspects of the environmental conditions at the time of deposition. However, for the purpose of analysis, the various proxy time series must refer to a single age–depth model for the sediment core they are derived from. For this reason, the basis of our synthesis is formed by a collection of sediment cores, each associated with its own age–depth model.
Marine sediments are dated using absolute age controls, where specific layers are dated using, for instance, radiocarbon, tephra or palaeomagnetic properties, and/or relative age controls, where time series are aligned based on the hypothesised synchronicity of the changes recorded by some properties of the sediment. A well-established hypothesis-based age modelling approach with a solid theoretical basis is the alignment of benthic foraminifera stable-oxygen-isotope ratio (δ18O) time series (Lisiecki and Raymo, 2005). We thus base our chronological framework on a combination of radiocarbon dates and benthic foraminifera δ18O and have selected time series where both parameters are available as the foundation of this data product. This approach of blending absolute and relative age controls is required to provide age–depth models for sediment cores that extend beyond the radiocarbon dating range (∼40 000 years). If available, further proxy time series were then added, thus ensuring a common chronology among all proxy time series measured on the same sediment core.
We selected palaeoclimate parameters to synthesise the following discussion with climate modellers within the PalMod project. The high-priority selection includes both physically and biogeochemically relevant parameters, of which some are based on measurements that can be compared with climate model output using (forward proxy) models (e.g. benthic δ18O) and others represent inferred parameters that can be compared with model output more directly but for which proxy models are still in their infancy (e.g. temperature based on foraminifera Mg∕Ca). Also considered in parameter selection was the expected spatial and temporal coverage of data availability as well as the existence of previous data products. The high-priority parameters for which data are presented here are listed in Table 1. If available, raw data were synthesised and in cases where raw data were not available and it was possible to derive the raw data from the inferred palaeoclimate data, raw data were back-calculated. Raw data time series obtained in this way are flagged with a note describing the calculation.
Table 1Palaeoclimate parameters in the PalMod 130k marine data synthesis.
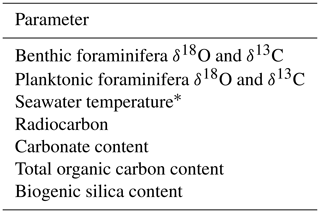
* Inferred from various proxies (foraminifera Mg∕Ca, alkenones, microfossil assemblages).
We note that our approach of first building the stratigraphic framework based on radiocarbon dates and benthic foraminifera δ18O means that the synthesis is not necessarily comprehensive as it does not include time series where one of the parameters of interest has been measured but where the components of the stratigraphic framework are not available. However, at this stage, we opted to include only sediment cores where an age modelling strategy that is consistent and comparable across the entire data product could be achieved.
2.1 Data discovery
In principle, data synthesis can proceed by expansion or reduction (Fig. 1). The first, more traditional, approach relies on expert knowledge of what data are available and/or on asystematic literature search. In this approach the synthesis grows by including more data until sufficient data that meet inclusion criteria are compiled. In this way, a lot of time is spent on discovering and retrieving datasets, and it is possible that valuable, but less exposed, data are missed. On the other hand, this approach has a chance of uncovering dark data that are not publicly available (Fig. 1).
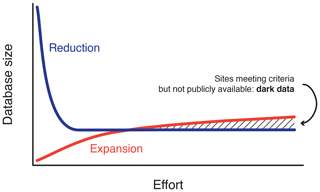
Figure 1Data synthesis approaches. In the expansion approach the database size increases slowly as records are added. The database size follows an opposite pathway using the reduction approach and reaches a stable size more quickly, with less effort. Since the expansion approach is not restricted to data that are available in the public domain, this approach may lead to a database that includes data that are not publicly available (dark data). The reduction approach on the other hand is arguably more objective, can be automated and is therefore more efficient. This approach also encourages good data stewardship.
The second approach starts from a large and crude synthesis of data from public sources and proceeds by weeding out data that do not meet criteria for inclusion in the data product. This approach faces different challenges: making sure that the initial bulk database is comprehensive (efficient data mining) and assuring that the data filtering is efficient (fast and accurate). In contrast to the expansion approach, this reduction approach cannot discover dark data. However, it is more objective (less reliant on expert knowledge), can be automated more easily and focuses on data that are already in the public domain so that no time is lost to finding data that ultimately prove unavailable. Because this second approach focuses on data that are publicly available, it also rewards and encourages good data stewardship.
In theory, both approaches can lead to a similarly sized and exhaustive synthesis, but they differ in the allocation of effort (Fig. 1). In practice both approaches are often combined, especially towards the end of a synthesis project, when the data product is benchmarked against existing syntheses.
2.2 Synthesis
2.2.1 Initial synthesis
We followed the reduction approach and used a semi-automated pipeline to compile data from public sources. Keywords (Supplement) were used to make lists of URLs of potentially relevant data on https://pangaea.de (last access: September 2016), and the linked files were then downloaded in bulk (n=108 239). A slightly different approach was followed for the NCEI archive. Here, all files that were machine-readable at the time of download (September 2016, n=1925) were obtained from the FTP server (ftp://ftp.ncdc.noaa.gov/pub/data/paleo/paleocean//sediment_files/complete, last access: September 2016). Custom scripts in R were used to put all data in a common format and merge time series that could be unambiguously assigned to the same core (based on name and x, y, z position). This resulted in a mixture of records that were merged to the same core and those that could not be, either because there was only one data file for the core or because of ambiguous labelling. We refer to the locations of these records as “sites”. In order to facilitate the analysis (filtering) of the sites, a uniform attribution of the various parameter names had to be developed. Because no standardised names exist for palaeoclimate parameters, the uniform attribution required the development of attribution libraries for each desired palaeoclimate parameter. The initial synthesis contained time series from 38 511 sites.
2.2.2 Data reduction and standardisation of ontologies
The initial synthesis was reduced by removing non-marine sites (using elevation) and further constrained by only considering sites where at least one data point of any of the parameters measured in that core fell within the target time frame (disambiguating age units in the synonym library of the category “age”) and the site had benthic oxygen isotope data. This resulted in 781 sites. At this stage, no criteria for length or resolution were applied but we prioritised processing time series that we estimated to contain at least 50 data points within the 130 000-year timeframe. Further data processing started with dereplication of the selected sites. This was necessary because no standards exist for the naming of cores and the repositories store data with different renditions of the same core name, sometimes even associated with erroneous geographic coordinates. This process was carried out manually and proceeded by constructing a list of disambiguated sites through sequential one-by-one comparison. Where a strict synonym was found (different labels for the same core but the same data), only unique data were retained. At this stage, disambiguation of site names was only performed for sites that had at least benthic δ18O, so time series of other parameters, which were associated with inconsistent core labels, could have been missed in the synthesis. However, those sites are contained in the initial bulk synthesis and are hence not lost but will be salvaged in updates of the data product (see Sect. 7).
Further steps required a manual standardisation of the names of the parameters and their attributes (such as the species name that was analysed for oxygen isotopes). This was accomplished by deciding on a final, uniform list of parameters and associated metadata and their possible values. Original parameter names were preserved to allow for cross-checking. By metadata, we refer to aspects of the individual parameters that were deemed essential to facilitate a meaningful analysis in a palaeoclimatic context, considering potential sources of uncertainty, such as species of foraminifera analysed or the calibration equation used for palaeotemperature estimates. The list of metadata is provided in Table 2. The standardisation was accompanied by further dereplication of individual time series that were already associated with the same site name but archived more than once.
2.2.3 Metadata and chronology
Subsequently, as far as possible, metadata values were added manually, often by scraping the information from the original publication. Next, all time series from a single site (core) were put on a common depth scale to allow for age modelling. Data that could not be put on a depth scale were excluded from the synthesis. This was the case where parameter values were archived only against age and where no other data file was available that allowed unambiguous reassignment to depth. Ambiguity also resulted from the use of multiple (composite) depth scales for the same archive. Finally, chronological data (all absolute markers, including radiocarbon dates and associated metadata) were manually added, where necessary also by consulting the original publications. Throughout the process, publication information (digital object identifier (DOI) or, if not available, full bibliographic details) and the data source (URL and/or DOI) were preserved in order to trace the source of the data. This applies both to the source of the individual data files from repositories and to the sources of the metadata and chronological data.
2.3 Age modelling
Whereas the initial steps of data discovery and synthesis could rely on published chronology, analysis of the complete dataset requires the development of a common chronological framework. This framework must be constructed in a way that not only allows for a consistent method of assignment of ages to depths within each core but also allows for the consistent and quantitative assessment of age uncertainty. To this end, we follow an approach that combines absolute ages (radiocarbon ages, tephra layers and palaeomagnetic events) with δ18O stratigraphy. As a result, our age models may differ from those reported in the original publication(s). This does not mean that the updated age models are better (constrained), but they are constructed in a way that allows for applicability and consistency across the synthesis. The consistent approach allows for an assessment of age uncertainty jointly for all records by a Bayesian approach, generating ensembles of sedimentation histories consistent with the available age control points for each core, allowing for uncertainty estimates at each depth by considering the distribution of ages given by the ensemble.
With respect to the reporting of the chronology, we follow a transparent approach, preserving the initial age model and providing the new age models as well as all information needed to revise or update the new age models. In the final step, the age information from absolute ages and δ18O tie points was combined and the age model and its uncertainty was assessed in a Bayesian framework using “Bacon” (Blaauw and Christen, 2011). The entire age modelling routine was carried out in PaleoDataView (PDV; Langner and Mulitza, 2019).
To ensure a common chronological framework for all time series in the synthesis, radiocarbon ages were recalibrated using the IntCal13 curve (Reimer et al., 2013). Since reservoir ages vary in space as well as in time, we used reservoir age estimates based on a comprehensive ocean general circulation model (Butzin et al., 2017) to account for this variability in a physically plausible way. To derive the reservoir age and uncertainty for a measured radiocarbon age, PDV (i) extracts all modelled radiocarbon ages from the nearest grid cell in the modelled dataset, (ii) finds all modelled radiocarbon ages that are possible within the error of the measured radiocarbon age, and (iii) takes the mean and the standard deviation of all corresponding reservoir ages to correct for the measured radiocarbon age. By definition, this approach cannot account for processes affecting the reservoir ages on subgrid spatial scales. Given the relatively coarse resolution of the model, this means that processes such as upwelling are not fully accounted for. In addition, no modelled reservoir age data are available for the Mediterranean and Red seas; we use the reservoir ages reported by the authors of the original publication and an assumed uncertainty of 100 years for these basins (five sites). Absolute ages based on North Atlantic tephra layers and palaeomagnetic events were updated and harmonised using Svensson et al. (2008).
In addition, and beyond the 14C dating realm (∼40 000 years), the age models rely on manual tuning of the benthic foraminifera δ18O time series from each core to regional benthic foraminifera δ18O stacks (Lisiecki and Stern, 2016). Stable-isotope stratigraphy in theory provides a range of events to correlate; however, in order to not inflate confidence in the tuned age models and to ensure comparability between different cores, in our approach, the tuning was carried out as far as possible by only matching the position of marine isotope stage boundaries. We updated the age–depth models only for the 0–130 000 years time frame of this synthesis, but data and original age models extending beyond 130 000 years are preserved in the data product. To obtain uncertainty for the age control points obtained by δ18O tuning, we used the chronological uncertainty in the δ18O stacks, as reported by Lisiecki and Stern (2016). Additional uncertainty associated with the identification of the control points in the individual records or with the assumption on synchronicity was ignored, as these are difficult, if not impossible, to quantify.
This synthesis contains climate-sensitive proxy data based on measurements using various biological sensors. It is not the intention here to provide a full overview of marine palaeoclimate proxies and their uncertainties (for this, see for example Hillaire-Marcel and De Vernal, 2007; Moffa-Sánchez et al., 2019), but the fact that the proxies are based on biological sensors means that they are affected by different ecological bias in addition to observational noise. Basic knowledge of the recording system is therefore essential for the interpretation of the data and may aid in explaining differences between proxies for the same climate parameter. These considerations were also essential to choosing the range of metadata to be recorded alongside each palaeoclimatic parameter to allow for a proxy-specific assessment of uncertainty.
Foraminifera are among the most widely used proxy sensors in palaeoceanography. They are unicellular marine zooplankton. The species used here all build a calcite skeleton that is preserved in the sediment. Foraminifera can be divided into two main groups: benthic foraminifera living at the seafloor level or at shallow depth in the sediment and planktonic foraminifera living in the upper hundreds of metres of the ocean. In the data product, proxies measured on these two groups are clearly distinguished by a benthic or planktonic prefix. The chemical composition of foraminifera reflects environmental conditions of the seawater that the organisms calcified in. For the purpose of this data product, the parameters of interest are stable-oxygen-isotope, stable-carbon-isotope and Mg∕Ca ratios. Stable-oxygen-isotope ratios in foraminifera calcite reflect a combination of temperature and δ18O of seawater (Urey, 1948), which is in turn related to ice volume and salinity. Species-specific calibrations exist to quantitatively link δ18Oforaminifera and δ18Oseawater to temperature (e.g. Marchitto et al., 2014; Bemis et al., 1998). Stable-carbon-isotope ratios (δ13C) reflect the δ13C of the dissolved inorganic carbon in seawater. In particular the benthic foraminifera species Cibicidoides wuellerstorfi generally incorporates δ13CDIC without a biological offset and can serve as a tracer of bottom-water δ13CDIC which is commonly used as non-passive circulation tracer (Curry and Oppo, 2005). The δ13C of other benthic foraminifera species is generally not indicative of bottom-water δ13CDIC, and the δ13C of planktonic foraminifera is also influenced by temperature and carbonate ion concentration, rendering interpretation complicated (Spero et al., 1997).
The Mg∕Ca ratio in foraminifera calcite can be used to infer calcification temperature and, in combination with δ18Oforaminifera, the δ18Oseawater (Elderfield and Ganssen, 2000). Similar to stable oxygen isotopes, species-specific calibrations exist to quantitatively reconstruct past temperature from Mg∕Ca ratios (Anand et al., 2003; Lear et al., 2002), and whenever indicated in the original publication, the calibration is included in the metadata. Carbonate system parameters and salinity have a secondary influence on Mg∕Ca ratios in foraminifera calcite (Gray et al., 2018b). Whereas benthic foraminifera live in a generally stable environment, the near-sea-surface habitat of planktonic foraminifera shows large seasonal and vertical gradients. Species-specific seasonal and/or depth habitat preferences may therefore leave a considerable imprint on the proxy signal contained in their shells (Jonkers and Kučera, 2017; Mix, 1987). For all proxies based on foraminifera, it is relevant to record the species as well as the number of individuals that were pooled for geochemical analysis. The latter is because the short lifespan and variable habitat of foraminifera species cause large variability among individuals. Planktonic foraminifera shell size may for several reasons also affect their chemistry (Jonkers et al., 2013; Friedrich et al., 2012) as well as their assemblage composition (Al-Sabouni et al., 2007). Therefore, the size fraction of the analysed shells was included in the metadata whenever this information was available.
Besides planktonic foraminifera Mg∕Ca ratios, the unsaturation index can provide information about near-sea-surface temperature. The index is based on the relative degree of unsaturation of C37 alkenones, which is linearly related to temperature (Prahl et al., 1988). Alkenones are produced by coccolithophores, marine phytoplankton living in the photic zone. The production of alkenones is in many regions not constant during the year, thus potentially causing a seasonal recording bias in the temperature proxy (Rosell-Melé and Prahl, 2013). Several calibrations exist that relate the index to sea surface temperature, and if the calibration was mentioned in the original publication, it was preserved in the metadata.
A large proportion of the temperature estimates in this data product are based on microfossil (planktonic foraminifera, diatoms, Radiolaria, dinoflagellate cysts) assemblages. These reconstructions are based on a statistical relationship between species assemblages and temperature (Imbrie and Kipp, 1971). In theory, microfossil assemblages can be used to reconstruct temperatures of different seasons or different environmental parameters from the same assemblage. However, it is not always clear that such reconstructions are truly independent (Telford and Birks, 2011). Several different methods exist to relate fossil assemblages to temperature, and researchers often apply more than a single method in their reconstructions to increase confidence (Kucera et al., 2005). When available, these different reconstructions are included in the data product.
The bulk sediment data (CaCO3, TOC and BSi) form a category of their own. They are not proxies in the strict sense but properties of the sediment that reflect a combination of export productivity, sedimentation and preservation. However, they can provide crucial information about the ocean–climate system, in particular about biogeochemical cycles (Cartapanis et al., 2016). With the advent of explicit sediment modules in climate models (Heinze et al., 1999; Kurahashi-Nakamura et al., 2020), sediment composition can also be directly compared with model output and potentially provide additional constraints on the simulations.
Following the data synthesis strategy outlined above, we generated a first data product for time series of eight parameters in sediment cores with radiocarbon and benthic δ18O stratigraphy. Following the logic of our approach, the synthesis is organised by the physical object from which the records were extracted (cores), here called site, to account for the inclusion of records from spliced cores.
Each site in the data product has information on seven different themes (Fig. 2):
-
Geographic data contain the site name, latitude and longitude (in decimal degrees N and E), elevation or water depth (in metres), and possible notes that are relevant to the site or core as a whole. All fields except notes are essential and always included.
-
Metadata include the original parameter name as given in the online data file, a standardised parameter name, parameter type (measured or inferred), unit, an estimate of analytical error as determined from repeat measurements of a standard and an estimate of reproducibility as determined by repeat measurements on samples. For measured parameters, information on the instrument and laboratory is given. All metadata terms are listed in Table 2, and an overview of the standardised parameter names is provided in Table 3.
-
Chronology data contain raw data on absolute age control points used for age modelling. This includes not only depth, radiocarbon ages including their uncertainty, dated material and laboratory codes but also calendar ages of tephra layers and palaeomagnetic events. Age control points not used in the age model by the authors of the original publication(s) are indicated, and if available, the source (DOI or URL) of the data is shown in addition to the original publication DOI. A complete list of chronology data terms is given in Table 4.
-
The actual time series data are provided on a common depth scale. Original age models are preserved alongside the data time series as they may differ for different time series from the same site. The data also contain information on the sample number or label (mainly for DSDP, ODP and IODP cores) for spliced records and sample-specific notes.
-
The revised age model contains ages for depths bracketed by age control points (absolute and relative). Mean and median ages are given as well as an uncertainty range (2.5th and 97.5th percentiles) based on the full suite of age model ensembles. No attempts were made to extrapolate the age models beyond the tie points in the 130 000-year time frame, so original age models may extend to either side.
-
The Bacon data contain all information to reproduce the revised age model. Besides the 14C and absolute age control points, this includes the tie points for the alignment, the alignment target and all parameters used to construct the age–depth model.
-
Age ensembles are provided for further assessment of chronological uncertainty. In order to keep file sizes manageable, 1000 randomly selected age model ensembles are preserved.
The data product contains 896 time series of the palaeoclimate parameters listed in Table 1 from 143 sites. An overview of all datasets used in this synthesis, including URLs to the data, can be found at https://doi.org/10.5281/zenodo.3739019 (Jonkers et al., 2020). Data sources for each site are listed in the Appendix. By design all sites have both benthic stable-oxygen-isotope and radiocarbon data.. The majority of the sites are close to the continents and in the Northern Hemisphere, with a concentration in the North Atlantic Ocean (Fig. 3). This reflects both research attention as well as the challenges of obtaining sediment cores with high accumulation rates and well-preserved foraminifera. The effect of research focus is also visible in the temporal coverage of the time series, where every parameter is characterised by a clear maximum around the last glacial termination ca. 15 ka (Fig. 4). The median resolution of the time series varies by 2 orders of magnitude but is generally better than one sample per 1000 years and fairly similar among the different parameters (Fig. 5). The updated age models are based on chronological control points (tie points) from radiocarbon dating and absolutely dated layers (using tephra and/or palaeomagnetic event stratigraphy) as well as alignment to the regional benthic δ18O stacks. The majority of the time series has a chronological control point at least every 5000 years (Fig. 6). Taken together, the coverage in space, time and across parameters indicates that the PalMod marine palaeoclimate data product allows for analysis of palaeoclimate on a supra-regional scale over the entire 130 000-year time frame.
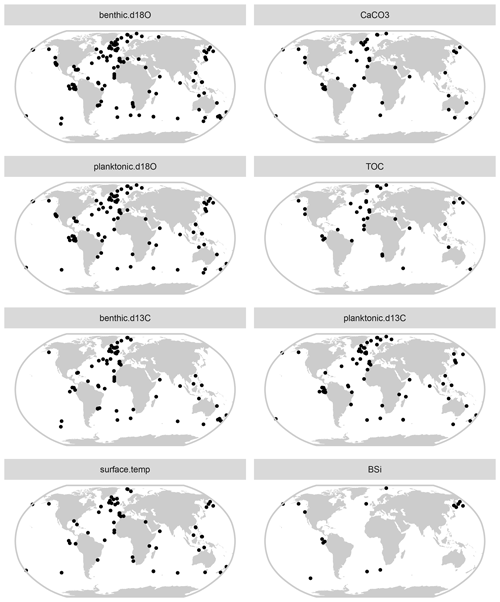
Figure 3Spatial distribution of sites in version 1.0.0 of the PalMod 130k marine palaeoclimate data synthesis. The distribution of the sites reflects research effort and the possibility of obtaining sediment cores containing well-preserved foraminifera and is hence skewed towards the Northern Hemisphere (Atlantic) and the continental margins. Since no explicit search was carried out for parameters other than benthic foraminifera δ18O, the distribution of the other parameters is restricted to sites that have benthic foraminifera δ18O. For benthic foraminifera δ13C, only sites with data based on the genus Cibicidoides are shown.
This data product builds upon previous syntheses. Virtually all of the sites are also part of the benthic foraminifera δ18O and δ13C compilations of Lisiecki and Stern (2016) and Peterson and Lisiecki (2018). Our synthesis, however, also includes data on other palaeoclimate parameters and contains more metadata and information on the age–depth models. Some of the planktonic foraminifera δ18O and Mg∕Ca time series in the PalMod 130k data product are also included in the Iso2k synthesis effort (Konecky et al., 2018), and a number of sea surface temperature time series are also part of the Temperature12k synthesis (Kaufman et al., 2020).
We provide the data products in three different formats in order to facilitate access and analysis using different software and across operating systems. Given the structure of the formats, each representation is slightly different in its level of metadata detail and the way metadata and data are stored. The differences are described below.
-
Since the data product was built using R, the data product is presented in R-readable RDS files that contain for each site a list with data for each theme (Sect. 4). This is the format that is most complete, yet in the interest of memory space it preserves a random selection of 1000 age models from the larger ensemble produced using Bacon. In this format, all data and metadata for each site are contained within a single file. Example scripts (https://github.com/lukasjonkers/PALMODutils, last access: 6 May 2020) allow the user to extract a quick overview of the contents of the data product with information on the temporal range, resolution and age control of the time series. Additional code is available to query the data product by parameter, parameter detail, sensor species, temporal range, resolution and age control.
-
The data product is also provided in the LiPD format, which is built around JSON-LD and CSV formats and is widely readable across different platforms. As with RDS, all data for each site are presented in a single file. Utilities to interact with LiPD files in R, Matlab and Python are available at https://github.com/nickmckay/LiPD-utilities (last access: 6 May 2020).
-
Finally, the data are also provided in netCDF format in a way that allows reading with PDV. This means that a single site has separate files for each individual palaeoclimate parameter as well as for the age model. In this format, some metadata are stored as concatenated strings rather than easily searchable attributes. The netCDF format allows however for the storage of the full suite of age model ensembles without excessive file sizes. The PDV software to read and process the data can be downloaded at https://www.marum.de/en/Stefan-Mulitza/PaleoDataView.html (last access: 6 May 2020).
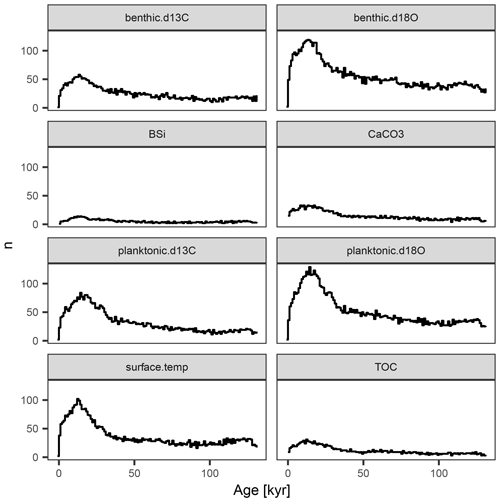
Figure 4Temporal distribution of time series in version 1.0.0 of the PalMod 130k marine palaeoclimate data synthesis. The number of time series (y axis) is counted per 1000-year bin. The temporal availability of all parameters shows a clear maximum around 15 ka, reflecting the research focus on the last glacial termination. For benthic foraminifera δ13C, only time series with data based on the genus Cibicidoides are shown.
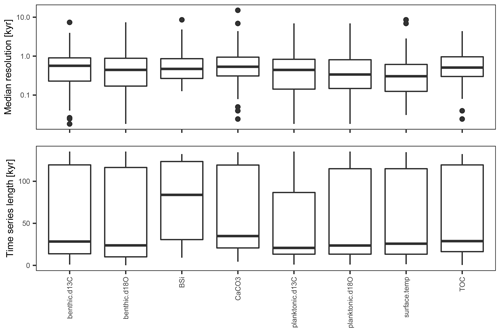
Figure 5Median resolution of the time series in version 1.0.0 of the PalMod 130k marine palaeoclimate data synthesis. Box-and-whisker plots show the spread of resolution per parameter. For benthic foraminifera δ13C the resolution data are restricted to time series containing data measured on shells of the genus Cibicidoides. For all parameters the median resolution is more than one data point per 1000 years.
To increase the spatio-temporal coverage over the entire 130 000-year time frame of the database, updates of this data product will first aim for quantitative growth of the database by adding more time series with chronological control based on benthic foraminifera δ18O and absolute age control points other than 14C. If available, these updates will also include the parameters listed in Table 1. They will be named using the counter following the first decimal separator. The structure of the data product is designed to be flexible, allowing for the addition of different metadata fields and parameters. Further updates that include new parameters and/or require a new age modelling approach (i.e. no benthic δ18O alignment) will be named using the counter before the first decimal separator. Any updates to add or correct (meta)data to an existing version that do not increase the number of sites will be indicated using a counter following the second decimal separator. Future versions will be made available on PANGAEA, and links to the updates will be provided at https://doi.org/10.1594/PANGAEA.908831 (Jonkers et al., 2019).
The PalMod 130k marine palaeoclimate data product can be downloaded in R, LiPD and netCDF format at https://doi.org/10.1594/PANGAEA.908831 (Jonkers et al., 2019). The data can also be visualised and downloaded in LiPD and CSV formats at http://lipdverse.org/PalMod/current_version/. We encourage users of the data product to also cite the primary source of the data when using (individual time series of) this product.
Data reuse and sharing are both made easier when data are archived in a standardised manner. Even though a large number of palaeoceanographic data are publicly available, metadata to facilitate interpretation of the raw or inferred palaeodata are often not, or only partially, made available and need to be obtained from the original publication, which may be behind a paywall and not freely accessible. Synthesis efforts therefore still require a lot of time and effort to find, compile and standardise data and metadata. Only recently has the palaeoclimate community started to discuss data-archiving standards (Khider et al., 2019). However, implementation of the proposed Paleoclimate Community reporTing Standard (PaCTS 1.0) will only affect new uploads to public repositories, and data already available (legacy data) are likely only going to be made compliant with the PaCTS through dedicated synthesis efforts. Below we list some of the main issues that we encountered during data synthesis. Our aims with mentioning these are to raise awareness of how the lack of standardisation affects data synthesis and thereby to encourage best practice in data reporting. We encourage researchers and also reviewers to treat data handling not as an afterthought but as an integral part of their study. After all, compared to generating the data, data handling is not a time-consuming task. Time spent on proper documenting and archiving is not wasted as it facilitates reuse of data and enables scientific progress in our field.
Disambiguate core names. An apparently trivial, but surprisingly common, first-order issue is that core names are inconsistently archived. Different names for the same core arise not only from differences in hyphenation; truncation of (long) names; and minor variations in the same name that can, with expert knowledge, be linked but also from the use of altogether different names for the same core, e.g. reflecting differences in the labelling during an expedition and in the repository. This naming confusion renders it difficult to combine datasets from the same core, especially in an automated way, and to assess the uniqueness of time series from the same core for dereplication. We recommend using the full name as indicated in the cruise report where the core was first described.
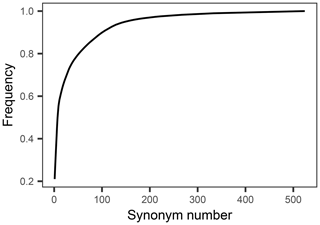
Figure 7The problem of the absence of standardisation in parameter names. The cumulative frequency of synonyms for seawater temperature in our initial database (Sect. 2.2.1), showing that there are over 500 different names for the same parameter and that many of these are unique.
Standardise vocabularies. Even though a vast number of palaeoclimate data are available in public repositories, the lack of standardisation of parameter vocabularies hinders efficient data processing. This problem is clearly illustrated by the fact that, for this synthesis, long synonym lists needed to be generated in order to group parameters. Each parameter in this synthesis had tens to hundreds of different names, of which many were unique (Fig. 7). This issue can be partly addressed by a consistent separation of parameter and attribute names (e.g. parameter δ18O, species Globigerina bulloides, instead of a single parameter “d18OGbul”), but even that calls for a standardisation of parameters and attributes.
Report sampling depth. All data reported here are based on measurements of discrete samples from a specific depth interval in a given core. Therefore, the synthesis requires information on the position of each sample. Since ages of the samples are always estimates and may differ among studies of the same archive, unambiguous information on sample depth is essential to reproduce and update the time series. Despite this, many studies fail to report sample depth and instead report only age. This problem is worse for spliced records that rely on a composite depth scale. Splicing approaches are often opaque, and original sample depths, or sample codes that identify unique samples, are not always available. We recommend therefore that sample depth should be essential for palaeodata time series from (marine) sediments and that sample labels are archived for spliced records. This includes (I)OPD or DSDP sample labels (in full) or IGSN (if available).
Publish raw data. To ensure the reproducibility of inferred parameters (in this synthesis only temperature) and to ensure the harmonisation or updating of the calibration, the raw measured data are needed. Provided that the calibration is known, measured data can in some cases be calculated from the inferred data, but this is impossible for data based on microfossil transfer functions. Related to this is unclear information about the calibration that was used, particularly if the publication describing the calibration includes multiple different equations.
Include metadata. To assess ecological imprints on proxy signals (recording bias), temperature estimates as well as oxygen and carbon isotope data based on planktonic foraminifera also require that key metadata, such as species name, are archived in a standardised way. This is not universally carried out, and for example the species information is often only available in the original publication. Additional information to assess the uncertainty in proxy measurements, such as foraminifera shell size, the sample size (e.g. number of shells, concentration of alkenones) or reproducibility of repeat measurements, was often not available from the paper or from the archived data, and we encourage the archiving of such data in a standardised way. A similar issue applies to chronological data. Thanks to a longer history of reporting standards, radiocarbon (Stuiver and Polach, 1977) (meta)data are often rather complete. However, this information is often not included alongside the digitally available data, and for this synthesis a large proportion of the radiocarbon (meta)data had to be scraped from the literature. In our age modelling approach, we took reservoir age uncertainty into account, using data derived from the modelled reservoir ages (see Sect. 2.3). Alternative approaches are hindered by the fact that reservoir age uncertainty is almost never reported.
Avoid redundancy. A considerable amount of time was spent on the dereplication of time series of the same parameter from the same core that were archived multiple times. Repeat archiving happens when data are reused or, less commonly, updated. The dereplication task is not made easier by (incomplete or inconsistent) metadata reporting and can be avoided through better linking of existing datasets when, instead of re-uploading the data, the DOI or URL of the original data is provided.
Help rescue dark data. This synthesis is based on data that are publicly available, yet many palaeoceanographic time series are not archived in public repositories. Even though the proportion of this so-called dark data is by definition not known exactly, it likely affects every branch of palaeoceanography, and as a result palaeoceanographical data syntheses cannot be exhaustive. This problem is clearly exacerbated for syntheses relying on automated data mining. There are several shades of dark data, each requiring their own approach to retrieve them and make them available. Some data are only partially available, for instance datasets that lack sample depths. Such data only require additional data to make them reusable. These additional data can sometimes be calculated or obtained from cross-referencing different data files but in many cases will need to be retrieved from the data producers. Other datasets, in particular those from before the digital age, are presented in tables in the original publications. Progress has been made with digitising those datasets (especially in PANGAEA), but this work is not finished, and more effort is needed to make this data available to the community. There are also data that are used in publications but are not made available in any way (print or digital). This third shade of data can so far only be obtained from the original data producers or authors of the original publication or, if this proves impossible, needs to be digitised from graphs. Digitisation inevitably leads to a loss of accuracy of the data, and a dataset retrieved in this way should be flagged. A final category of dark data consists of data that are not part of a publication. Such datasets can be made publicly available and be associated with a DOI to ensure traceability. To reward data sharing, the use of data citations needs to be encouraged and data citations should be included in the evaluation of a researcher's impact.
Table A1Data sources for version 1.0.0 of the PalMod 130k marine palaeoclimate data synthesis. An extended version of this table can be accessed at https://doi.org/10.5281/zenodo.3739019 (Jonkers et al., 2020).
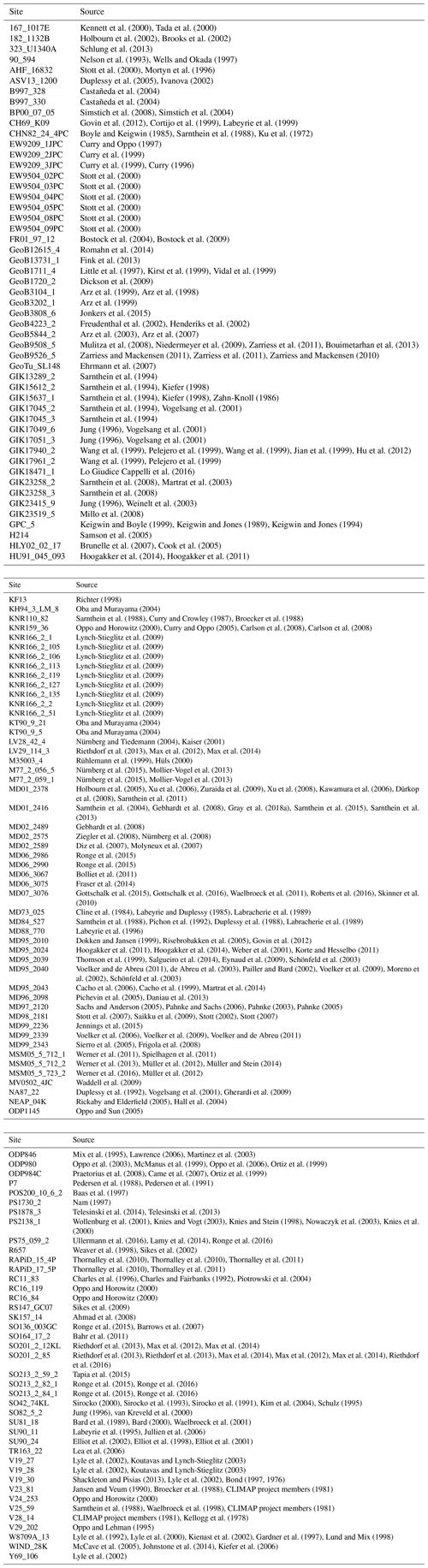
The supplement related to this article is available online at: https://doi.org/10.5194/essd-12-1053-2020-supplement.
LJ, MK and SM conceptualised the study; LJ, OC and MK developed the workflow; LJ designed the database structure with help from OC and MK; LJ and AS compiled (meta)data; LJ carried out quality control; LJ updated age–depth models; LJ, SM and ML ensured integration with the PDV format; LJ and NM ensured the integration with the LiPD format; MK and SM acquired funding; LJ and MK wrote the manuscript, and all authors reviewed and commented on the manuscript.
The authors declare that they have no conflict of interest.
This article is part of the special issue “Paleoclimate data synthesis and analysis of associated uncertainty (BG/CP/ESSD inter-journal SI)”. It is not associated with a conference.
This research was supported by PalMod, the German palaeoclimate modelling initiative (http://www.palmod.de, last access: 6 May 2020). PalMod is part of the Research for Sustainable Development initiative (FONA; http://www.fona.de, last access: 6 May 2020) funded by the German Federal Ministry of Education and Research (BMBF). We thank colleagues in working group 3 from PalMod for discussion on data and metadata requirements and Rangelys Sorrentino for help with data processing. We also thank Blanca Ausin and the anonymous reviewer for their constructive feedback on an earlier version of this manuscript.
This research has been supported by the Bundesministerium für Bildung und Forschung (PalMod grant). Olivier Cartapanis was funded by the Swiss National Science Foundation (grant no. PP00P2-144811).
This paper was edited by David Carlson and reviewed by Blanca Ausin and one anonymous referee.
Ahmad, S. M., Babu, G. A., Padmakumari, V. M., and Raza, W.: Surface and deep water changes in the northeast Indian Ocean during the last 60 ka inferred from carbon and oxygen isotopes of planktonic and benthic foraminifera, Palaeogeography, Palaeoclimatology, Palaeoecology, 262, 182–188, https://doi.org/10.1016/j.palaeo.2008.03.007, 2008.
Al-Sabouni, N., Kucera, M., and Schmidt, D. N.: Vertical niche separation control of diversity and size disparity in planktonic foraminifera, Mar. Micropaleontol., 63, 75–90, https://doi.org/10.1016/j.marmicro.2006.11.002, 2007.
Anand, P., Elderfield, H., and Conte, M. H.: Calibration of Mg∕Ca thermometry in planktonic foraminifera from a sediment trap time series, Paleoceanography, 18, 1050, https://doi.org/10.1029/2002PA000846, 2003.
Arz, H. W., Pätzold, J., and Wefer, G.: Correlated Millennial-Scale Changes in Surface Hydrography and Terrigenous Sediment Yield Inferred from Last-Glacial Marine Deposits off Northeastern Brazil, Quaternary Res., 50, 157–166, https://doi.org/10.1006/qres.1998.1992, 1998.
Arz, H. W., Pätzold, J., and Wefer, G.: The deglacial history of the western tropical Atlantic as inferred from high resolution stable isotope records off northeastern Brazil, Earth Planet. Sc. Lett., 167, 105–117, https://doi.org/10.1016/s0012-821x(99)00025-4, 1999.
Arz, H. W., Pätzold, J., Müller, P. J., and Moammar, M. O.: Influence of Northern Hemisphere climate and global sea level rise on the restricted Red Sea marine environment during termination I, Paleoceanography, 18, 1053, https://doi.org/10.1029/2002pa000864, 2003.
Arz, H. W., Lamy, F., Ganopolski, A., Nowaczyk, N., and Pätzold, J.: Dominant Northern Hemisphere climate control over millennial-scale glacial sea-level variability, Quaternary Sci. Rev., 26, 312–321, https://doi.org/10.1016/j.quascirev.2006.07.016, 2007.
Baas, J. H., Mienert, J., Abrantes, F., and Prins, M. A.: Late Quaternary sedimentation on the Portuguese continental margin: climate-related processes and products, Palaeogeogr. Palaeocl., 130, 1–23, https://doi.org/10.1016/s0031-0182(96)00135-6, 1997.
Bahr, A., Nürnberg, D., Schönfeld, J., and Garbe-Schönberg, D.: Hydrological variability in Florida Straits during Marine Isotope Stage 5 cold events, Paleoceanography, 26, PA2214, https://doi.org/10.1029/2010pa002015, 2011.
Bard, E.: Hydrological Impact of Heinrich Events in the Subtropical Northeast Atlantic, Science, 289, 1321–1324, https://doi.org/10.1126/science.289.5483.1321, 2000.
Bard, E., Fairbanks, R., Arnold, M., Maurice, P., Duprat, J., Moyes, J., and Duplessy, J.-C.: Sea-Level Estimates during the Last Deglaciation Based on δ18O and Accelerator Mass Spectrometry 14C Ages Measured in Globigerina bulloides, Quaternary Res., 31, 381–391, https://doi.org/10.1016/0033-5894(89)90045-8, 1989.
Barrows, T. T., Juggins, S., De Deckker, P., Calvo, E., and Pelejero, C.: Long-term sea surface temperature and climate change in the Australian-New Zealand region, Paleoceanography, 22, PA2215, https://doi.org/10.1029/2006pa001328, 2007.
Bemis, B. E., Spero, H. J., Bijma, J., and Lea, D. W.: Reevaluation of the Oxygen Isotopic Composition of Planktonic Foraminifera: Experimental Results and Revised Paleotemperature Equations, Paleoceanography, 13, 150–160, https://doi.org/10.1029/98pa00070, 1998.
Blaauw, M. and Christen, J. A. S.: Flexible paleoclimate age-depth models using an autoregressive gamma process, Bayesian Anal., 6, 457–474, 2011.
Bolliet, T., Holbourn, A., Kuhnt, W., Laj, C., Kissel, C., Beaufort, L., Kienast, M., Andersen, N., and Garbe-Schönberg, D.: Mindanao Dome variability over the last 160 kyr: Episodic glacial cooling of the West Pacific Warm Pool, Paleoceanography, 26, PA1208, https://doi.org/10.1029/2010pa001966, 2011.
Bond, G.: A Pervasive Millennial-Scale Cycle in North Atlantic Holocene and Glacial Climates, Science, 278, 1257–1266, https://doi.org/10.1126/science.278.5341.1257, 1997.
Bostock, H. C., Opdyke, B. N., Gagan, M. K., and Fifield, L. K.: Carbon isotope evidence for changes in Antarctic Intermediate Water circulation and ocean ventilation in the southwest Pacific during the last deglaciation, Paleoceanography, 19, PA4013, https://doi.org/10.1029/2004pa001047, 2004.
Bostock, H. C., Opdyke, B. N., Gagan, M. K., and Fifield, L. K.: Late Quaternary siliciclastic/carbonate sedimentation model for the Capricorn Channel, southern Great Barrier Reef province, Australia, Mar. Geol., 257, 107–123, https://doi.org/10.1016/j.margeo.2008.11.003, 2009.
Bouimetarhan, I., Groeneveld, J., Dupont, L., and Zonneveld, K.: Low- to high-productivity pattern within Heinrich Stadial 1: Inferences from dinoflagellate cyst records off Senegal, Global Planet. Change, 106, 64–76, https://doi.org/10.1016/j.gloplacha.2013.03.007, 2013.
Boyle, E. A. and Keigwin, L. D.: Comparison of Atlantic and Pacific paleochemical records for the last 215,000 years: changes in deep ocean circulation and chemical inventories, Earth Planet. Sc. Lett., 76, 135–150, https://doi.org/10.1016/0012-821x(85)90154-2, 1985.
Breitkreuz, C., Paul, A., and Schulz, M.: A dynamical reconstruction of the Last Glacial Maximum ocean state constrained by global oxygen isotope data, Clim. Past Discuss., https://doi.org/10.5194/cp-2019-52, in review, 2019.
Broecker, W. S., Andree, M., Bonani, G., Wolfli, W., Klas, M., Mix, A., and Oeschger, H.: Comparison between radiocarbon ages obtained on coexisting planktonic foraminifera, Paleoceanography, 3, 647–657, https://doi.org/10.1029/pa003i006p00647, 1988a.
Broecker, W. S., Andree, M., Bonani, G., Wolfli, W., Oeschger, H., and Klas, M.: Can the Greenland Climatic Jumps be Identified in Records from Ocean and Land?, Quaternary Res., 30, 1–16, https://doi.org/10.1016/0033-5894(88)90082-8, 1988b.
Brooks, G. R., Hine, A. C., Mallinson, D., and Drexler, T. M.: Data Report: Texture and Composition of Quaternary Upper-Slope Sediments in the Great Australian Bight: Sites 1130 and 1132, in: Proceedings of the Ocean Drilling Program, 182 Scientific Results, Ocean Drilling Program, 2002.
Brunelle, B. G., Sigman, D. M., Cook, M. S., Keigwin, L. D., Haug, G. H., Plessen, B., Schettler, G., and Jaccard, S. L.: Evidence from diatom-bound nitrogen isotopes for subarctic Pacific stratification during the last ice age and a link to North Pacific denitrification changes, Paleoceanography, 22, PA1215, https://doi.org/10.1029/2005pa001205, 2007.
Butzin, M., Köhler, P., and Lohmann, G.: Marine radiocarbon reservoir age simulations for the past 50,000 years, Geophys. Res. Lett., 44, 8473–8480, https://doi.org/10.1002/2017GL074688, 2017.
Cacho, I., Grimalt, J. O., Pelejero, C., Canals, M., Sierro, F. J., Flores, J. A., and Shackleton, N.: Dansgaard-Oeschger and Heinrich event imprints in Alboran Sea paleotemperatures, Paleoceanography, 14, 698–705, https://doi.org/10.1029/1999pa900044, 1999.
Cacho, I., Shackleton, N., Elderfield, H., Sierro, F. J., and Grimalt, J. O.: Glacial rapid variability in deep-water temperature and δ18O from the Western Mediterranean Sea, Quaternary Sci. Rev., 25, 3294–3311, https://doi.org/10.1016/j.quascirev.2006.10.004, 2006.
Came, R. E., Oppo, D. W., and McManus, J. F.: Amplitude and timing of temperature and salinity variability in the subpolar North Atlantic over the past 10 k.y, Geology, 35, 315–318, https://doi.org/10.1130/g23455a.1, 2007.
Carlson, A. E., Oppo, D. W., Came, R. E., LeGrande, A. N., Keigwin, L. D., and Curry, W. B.: Subtropical Atlantic salinity variability and Atlantic meridional circulation during the last deglaciation, Geology, 36, 991–994, https://doi.org/10.1130/g25080a.1, 2008.
Cartapanis, O., Bianchi, D., Jaccard, S. L., and Galbraith, E. D.: Global pulses of organic carbon burial in deep-sea sediments during glacial maxima, Nat. Commun., 7, 10796, https://doi.org/10.1038/ncomms10796, 2016.
Castañeda, I. S., Smith, L. M., Kristjánsdóttir, G. B., and Andrews, J. T.: Temporal changes in Holocene δ18O records from the northwest and central North Iceland Shelf, J. Quaternary Sci., 19, 321–334, https://doi.org/10.1002/jqs.841, 2004.
Charles, C. D. and Fairbanks, R. G.: Evidence from Southern Ocean sediments for the effect of North Atlantic deep-water flux on climate, Nature, 355, 416–419, https://doi.org/10.1038/355416a0, 1992.
Charles, C. D., Lynch-Stieglitz, J., Ninnemann, U. S., and Fairbanks, R. G.: Climate connections between the hemisphere revealed by deep sea sediment core/ice core correlations, Earth Planet. Sc. Lett., 142, 19–27, https://doi.org/10.1016/0012-821x(96)00083-0, 1996.
CLIMAP: The Surface of the Ice-Age Earth, Science, 191, 1131–1137, https://doi.org/10.1126/science.191.4232.1131, 1976.
CLIMAP project members: Seasonal reconstructions of the Earth's surface at the last Glacial Maximum, Geol. Soc. Am., Boulder, CO, 1981.
Cline, R. M. L., Hays, J. D., Prell, W. L., Ruddiman, W. F., Moore, T. C., Kipp, N. G., Molfino, B. E., Denton, G. H., Hughes, T. J., Balsam, W. L., Brunner, C. A., Duplessy, J.-C., Esmay, A. G., Fastook, J. L., Imbrie, J., Keigwin, L. D., Kellogg, T. B., McIntyre, A., Matthews, R. K., Mix, A. C., Morley, J. J., Shackleton, N. J., Streeter, S. S., and Thompson, P. R.: The Last Interglacial Ocean, Quaternary Res., 21, 123–224, https://doi.org/10.1016/0033-5894(84)90098-x, 1984.
Cook, M. S., Keigwin, L. D., and Sancetta, C. A.: The deglacial history of surface and intermediate water of the Bering Sea, Deep-Sea Res. Pt. II, 52, 2163–2173, https://doi.org/10.1016/j.dsr2.2005.07.004, 2005.
Cortijo, E., Lehman, S., Keigwin, L., Chapman, M., Paillard, D., and Labeyrie, L.: Changes in Meridional Temperature and Salinity Gradients in the North Atlantic Ocean (30∘-72∘ N) during the Last Interglacial Period, Paleoceanography, 14, 23–33, https://doi.org/10.1029/1998pa900004, 1999.
Curry, W. B.: Late Quaternary Deep Circulation in the Western Equatorial Atlantic, in: The South Atlantic, Springer Berlin Heidelberg, 1996.
Curry, W. B. and Crowley, T. J.: The δ13C of equatorial Atlantic surface waters: Implications for Ice Age pCO2 levels, Paleoceanography, 2, 489–517, https://doi.org/10.1029/pa002i005p00489, 1987.
Curry, W. B. and Oppo, D. W.: Synchronous, high-frequency oscillations in tropical sea surface temperatures and North Atlantic Deep Water production during the Last Glacial Cycle, Paleoceanography, 12, 1–14, https://doi.org/10.1029/96pa02413, 1997.
Curry, W. B. and Oppo, D. W.: Glacial water mass geometry and the distribution of δ13C of ΣCO2 in the western Atlantic Ocean, Paleoceanography, 20, PA1017, https://doi.org/10.1029/2004pa001021, 2005.
Curry, W. B., Marchitto, T. M., McManus, J. F., Oppo, D. W., and Laarkamp, K. L.: Millennial-scale changes in ventilation of the thermocline, intermediate, and deep waters of the glacial North Atlantic, in: Mechanisms of Global Climate Change at Millennial Time Scales, American Geophysical Union, 1999.
Daniau, A. L., Sanchez Goni, M. F., Martinez, P., Urrego, D. H., Bout-Roumazeilles, V., Desprat, S., and Marlon, J. R.: Orbital-scale climate forcing of grassland burning in southern Africa, P. Natl. Acad. Sci. USA, 110, 5069–5073, https://doi.org/10.1073/pnas.1214292110, 2013.
de Abreu, L., Shackleton, N. J., Schönfeld, J., Hall, M., and Chapman, M.: Millennial-scale oceanic climate variability off the Western Iberian margin during the last two glacial periods, Mar. Geol., 196, 1–20, https://doi.org/10.1016/s0025-3227(03)00046-x, 2003.
Dickson, A. J., Beer, C. J., Dempsey, C., Maslin, M. A., Bendle, J. A., McClymont, E. L., and Pancost, R. D.: Oceanic forcing of the Marine Isotope Stage 11 interglacial, Nat. Geosci., 2, 428–433, https://doi.org/10.1038/ngeo527, 2009.
Diz, P., Hall, I. R., Zahn, R., and Molyneux, E. G.: Paleoceanography of the southern Agulhas Plateau during the last 150 ka: Inferences from benthic foraminiferal assemblages and multispecies epifaunal carbon isotopes, Paleoceanography, 22, PA4218, https://doi.org/10.1029/2007pa001511, 2007.
Dokken, T. M. and Jansen, E.: Rapid changes in the mechanism of ocean convection during the last glacial period, Nature, 401, 458–461, https://doi.org/10.1038/46753, 1999.
Dolman, A. M. and Laepple, T.: Sedproxy: a forward model for sediment-archived climate proxies, Clim. Past, 14, 1851–1868, https://doi.org/10.5194/cp-14-1851-2018, 2018.
Duplessy, J. C., Shackleton, N. J., Fairbanks, R. G., Labeyrie, L., Oppo, D., and Kallel, N.: Deepwater source variations during the last climatic cycle and their impact on the global deepwater circulation, Paleoceanography, 3, 343–360, https://doi.org/10.1029/pa003i003p00343, 1988.
Duplessy, J. C., Labeyrie, L., Arnold, M., Paterne, M., Duprat, J., and van Weering, T. C. E.: Changes in surface salinity of the North Atlantic Ocean during the last deglaciation, Nature, 358, 485–488, https://doi.org/10.1038/358485a0, 1992.
Duplessy, J. C., Cortijo, E., Ivanova, E., Khusid, T., Labeyrie, L., Levitan, M., Murdmaa, I., and Paterne, M.: Paleoceanography of the Barents Sea during the Holocene, Paleoceanography, 20, PA4004, https://doi.org/10.1029/2004pa001116, 2005.
Dürkop, A., Holbourn, A., Kuhnt, W., Zuraida, R., Andersen, N., and Grootes, P. M.: Centennial-scale climate variability in the Timor Sea during Marine Isotope Stage 3, Mar. Micropaleontol., 66, 208–221, https://doi.org/10.1016/j.marmicro.2007.10.002, 2008.
Ehrmann, W., Schmiedl, G., Hamann, Y., Kuhnt, T., Hemleben, C., and Siebel, W.: Clay minerals in late glacial and Holocene sediments of the northern and southern Aegean Sea, Palaeogeogr. Palaeocl., 249, 36–57, https://doi.org/10.1016/j.palaeo.2007.01.004, 2007.
Elderfield, H. and Ganssen, G.: Past temperature and δ18O of surface ocean waters inferred from foraminiferal Mg∕Ca ratios, Nature, 405, 442–445, 2000.
Elliot, M., Labeyrie, L., Bond, G., Cortijo, E., Turon, J.-L., Tisnerat, N., and Duplessy, J.-C.: Millennial-scale iceberg discharges in the Irminger Basin during the Last Glacial Period: Relationship with the Heinrich events and environmental settings, Paleoceanography, 13, 433–446, https://doi.org/10.1029/98pa01792, 1998.
Elliot, M., Labeyrie, L., Dokken, T., and Manthé, S.: Coherent patterns of ice-rafted debris deposits in the Nordic regions during the last glacial (10–60 ka), Earth Planet. Sc. Lett., 194, 151–163, https://doi.org/10.1016/s0012-821x(01)00561-1, 2001.
Elliot, M., Labeyrie, L., and Duplessy, J.-C.: Changes in North Atlantic deep-water formation associated with the Dansgaard–Oeschger temperature oscillations (60–10 ka), Quaternary Sci. Rev., 21, 1153–1165, https://doi.org/10.1016/s0277-3791(01)00137-8, 2002.
Eynaud, F., de Abreu, L., Voelker, A., Schönfeld, J., Salgueiro, E., Turon, J.-L., Penaud, A., Toucanne, S., Naughton, F., Sánchez Goñi, M. F., Malaizé, B., and Cacho, I.: Position of the Polar Front along the western Iberian margin during key cold episodes of the last 45 ka, Geochem. Geophy. Geosy., 10, Q07U05, https://doi.org/10.1029/2009gc002398, 2009.
Fink, H. G., Wienberg, C., De Pol-Holz, R., Wintersteller, P., and Hebbeln, D.: Cold-water coral growth in the Alboran Sea related to high productivity during the Late Pleistocene and Holocene, Mar. Geol., 339, 71–82, https://doi.org/10.1016/j.margeo.2013.04.009, 2013.
Fraser, N., Kuhnt, W., Holbourn, A., Bolliet, T., Andersen, N., Blanz, T., and Beaufort, L.: Precipitation variability within the West Pacific Warm Pool over the past 120 ka: Evidence from the Davao Gulf, southern Philippines, Paleoceanography, 29, 1094–1110, https://doi.org/10.1002/2013pa002599, 2014.
Freudenthal, T., Meggers, H., Henderiks, J., Kuhlmann, H., Moreno, A., and Wefer, G.: Upwelling intensity and filament activity off Morocco during the last 250,000 years, Deep-Sea Res. Pt. II, 49, 3655–3674, https://doi.org/10.1016/s0967-0645(02)00101-7, 2002.
Friedrich, O., Schiebel, R., Wilson, P. A., Weldeab, S., Beer, C. J., Cooper, M. J., and Fiebig, J.: Influence of test size, water depth, and ecology on Mg∕Ca, Sr∕Ca, δ18O and δ13C in nine modern species of planktic foraminifers, Earth Planet. Sc. Lett., 319–320, 133–145, https://doi.org/10.1016/j.epsl.2011.12.002, 2012.
Frigola, J., Moreno, A., Cacho, I., Canals, M., Sierro, F. J., Flores, J. A., and Grimalt, J. O.: Evidence of abrupt changes in Western Mediterranean Deep Water circulation during the last 50 kyr: A high-resolution marine record from the Balearic Sea, Quatern. Int., 181, 88–104, https://doi.org/10.1016/j.quaint.2007.06.016, 2008.
Gardner, J. V., Dean, W. E., and Dartnell, P.: Biogenic sedimentation beneath the California Current System for the past 30 kyr and its paleoceanographic significance, Paleoceanography, 12, 207–225, https://doi.org/10.1029/96pa03567, 1997.
Gebhardt, H., Sarnthein, M., Grootes, P. M., Kiefer, T., Kuehn, H., Schmieder, F., and Röhl, U.: Paleonutrient and productivity records from the subarctic North Pacific for Pleistocene glacial terminations I to V, Paleoceanography, 23, PA4212, https://doi.org/10.1029/2007pa001513, 2008.
Gherardi, J. M., Labeyrie, L., Nave, S., Francois, R., McManus, J. F., and Cortijo, E.: Glacial-interglacial circulation changes inferred from 231Pa/230Th sedimentary record in the North Atlantic region, Paleoceanography, 24, PA2204, https://doi.org/10.1029/2008pa001696, 2009.
Gottschalk, J., Skinner, L. C., and Waelbroeck, C.: Contribution of seasonal sub-Antarctic surface water variability to millennial-scale changes in atmospheric CO2 over the last deglaciation and Marine Isotope Stage 3, Earth Planet. Sc. Lett., 411, 87–99, https://doi.org/10.1016/j.epsl.2014.11.051, 2015.
Gottschalk, J., Skinner, L. C., Lippold, J., Vogel, H., Frank, N., Jaccard, S. L., and Waelbroeck, C.: Biological and physical controls in the Southern Ocean on past millennial-scale atmospheric CO2 changes, Nat. Commun., 7, 11539, https://doi.org/10.1038/ncomms11539, 2016.
Govin, A., Braconnot, P., Capron, E., Cortijo, E., Duplessy, J.-C., Jansen, E., Labeyrie, L., Landais, A., Marti, O., Michel, E., Mosquet, E., Risebrobakken, B., Swingedouw, D., and Waelbroeck, C.: Persistent influence of ice sheet melting on high northern latitude climate during the early Last Interglacial, Clim. Past, 8, 483–507, https://doi.org/10.5194/cp-8-483-2012, 2012.
Gray, W. R., Rae, J. W. B., Wills, R. C. J., Shevenell, A. E., Taylor, B., Burke, A., Foster, G. L., and Lear, C. H.: Deglacial upwelling, productivity and CO2 outgassing in the North Pacific Ocean, Nat. Geosci., 11, 340–344, https://doi.org/10.1038/s41561-018-0108-6, 2018a.
Gray, W. R., Weldeab, S., Lea, D. W., Rosenthal, Y., Gruber, N., Donner, B., and Fischer, G.: The effects of temperature, salinity, and the carbonate system on Mg∕Ca in Globigerinoides ruber (white): A global sediment trap calibration, Earth Planet. Sc. Lett., 482, 607–620, https://doi.org/10.1016/j.epsl.2017.11.026, 2018b.
Hall, I. R., Bianchi, G. G., and Evans, J. R.: Centennial to millennial scale Holocene climate-deep water linkage in the North Atlantic, Quaternary Sci. Rev., 23, 1529–1536, https://doi.org/10.1016/j.quascirev.2004.04.004, 2004.
Hays, J. D., Imbrie, J., and Shackleton, N. J.: Variations in the Earth's orbit: pacemaker of the ice ages, Science, 194, 1121–1132, 1976.
Heinze, C., Maier-Reimer, E., Winguth, A. M. E., and Archer, D.: A global oceanic sediment model for long-term climate studies, Global Biogeochem. Cy., 13, 221–250, https://doi.org/10.1029/98gb02812, 1999.
Henderiks, J., Freudenthal, T., Meggers, H., Nave, S., Abrantes, F., Bollmann, J., and Thierstein, H. R.: Glacial–interglacial variability of particle accumulation in the Canary Basin: a time-slice approach, Deep-Sea Res. Pt. II, 49, 3675–3705, https://doi.org/10.1016/s0967-0645(02)00102-9, 2002.
Hillaire-Marcel, C. and De Vernal, A.: Proxies in Late Cenozoic Paleoceanography, Elsevier, 2007.
Holbourn, A., Kuhnt, W., and James, N.: Late Pleistocene bryozoan reef mounds of the Great Australian Bight: Isotope stratigraphy and benthic foraminiferal record, Paleoceanography, 17, 14-11–14-13, https://doi.org/10.1029/2001pa000643, 2002.
Holbourn, A., Kuhnt, W., Kawamura, H., Jian, Z., Grootes, P., Erlenkeuser, H., and Xu, J.: Orbitally paced paleoproductivity variations in the Timor Sea and Indonesian Throughflow variability during the last 460 kyr, Paleoceanography, 20, PA3002, https://doi.org/10.1029/2004pa001094, 2005.
Hoogakker, B. A. A., Chapman, M. R., McCave, I. N., Hillaire-Marcel, C., Ellison, C. R. W., Hall, I. R., and Telford, R. J.: Dynamics of North Atlantic Deep Water masses during the Holocene, Paleoceanography, 26, https://doi.org/10.1029/2011pa002155, 2011.
Hoogakker, B. A. A., McCave, I. N., Elderfield, H., Hillaire-Marcel, C., and Simstich, J.: Holocene climate variability in the Labrador Sea, J. Geol. Soc., 172, 272–277, https://doi.org/10.1144/jgs2013-097, 2014.
Hu, D., Böning, P., Köhler, C. M., Hillier, S., Pressling, N., Wan, S., Brumsack, H. J., and Clift, P. D.: Deep sea records of the continental weathering and erosion response to East Asian monsoon intensification since 14 ka in the South China Sea, Chem. Geol., 326–327, 1–18, https://doi.org/10.1016/j.chemgeo.2012.07.024, 2012.
Hüls, M.: Millennial-scale SST variability as inferred from planktonic foraminifera sensus counts in the western subtropical Atlantic, PhD thesis, GEOMAR Research Center for Marine Geosciences, Christian Albrechts University in Kiel, Germany, 118 pp., 2000.
Imbrie, J. and Kipp, N. G.: A new micropaleontological method for quantitative paleoclimatology: application to a late Pleistocene Caribbean core, in: The late Cenozoic glacial ages, edited by: Turekian, K. K., Yale University Press, New Haven, 71–181, 1971.
Ivanova, E.: Late Weichselian to Holocene paleoenvironments in the Barents Sea, Global Planet. Change, 34, 209–218, https://doi.org/10.1016/s0921-8181(02)00116-9, 2002.
Ivanovic, R. F., Gregoire, L. J., Kageyama, M., Roche, D. M., Valdes, P. J., Burke, A., Drummond, R., Peltier, W. R., and Tarasov, L.: Transient climate simulations of the deglaciation 21–9 thousand years before present (version 1) – PMIP4 Core experiment design and boundary conditions, Geosci. Model Dev., 9, 2563–2587, https://doi.org/10.5194/gmd-9-2563-2016, 2016.
Jansen, E. and Veum, T.: Evidence for two-step deglaciation and its impact on North Atlantic deep-water circulation, Nature, 343, 612–616, https://doi.org/10.1038/343612a0, 1990.
Jennings, A., Andrews, J., Pearce, C., Wilson, L., and Ólfasdótttir, S.: Detrital carbonate peaks on the Labrador shelf, a 13–7 ka template for freshwater forcing from the Hudson Strait outlet of the Laurentide Ice Sheet into the subpolar gyre, Quaternary Sci. Rev., 107, 62–80, https://doi.org/10.1016/j.quascirev.2014.10.022, 2015.
Jian, Z., Wang, L., Kienast, M., Sarnthein, M., Kuhnt, W., Lin, H., and Wang, P.: Benthic foraminiferal paleoceanography of the South China Sea over the last 40,000 years, Mar. Geol., 156, 159–186, https://doi.org/10.1016/s0025-3227(98)00177-7, 1999.
Johnstone, H. J. H., Kiefer, T., Elderfield, H., and Schulz, M.: Calcite saturation, foraminiferal test mass, and Mg/Ca-based temperatures dissolution corrected using XDX-A 150 ka record from the western Indian Ocean, Geochem. Geophy. Geosy., 15, 781–797, https://doi.org/10.1002/2013gc004994, 2014.
Jonkers, L. and Kučera, M.: Quantifying the effect of seasonal and vertical habitat tracking on planktonic foraminifera proxies, Clim. Past, 13, 573–586, https://doi.org/10.5194/cp-13-573-2017, 2017.
Jonkers, L., van Heuven, S., Zahn, R., and Peeters, F. J. C.: Seasonal patterns of shell flux, δ18O and δ13C of small and large N. pachyderma (s) and G. bulloides in the subpolar North Atlantic, Paleoceanography, 28, 164–174, https://doi.org/10.1002/palo.20018, 2013.
Jonkers, L., Zahn, R., Thomas, A., Henderson, G., Abouchami, W., François, R., Masque, P., Hall, I. R., and Bickert, T.: Deep circulation changes in the central South Atlantic during the past 145 kyrs reflected in a combined 231Pa∕230Th, Neodymium isotope and benthic δ13C record, Earth Planet. Sc. Lett., 419, 14–21, https://doi.org/10.1016/j.epsl.2015.03.004, 2015.
Jonkers, L., Cartapanis, O., Langner, M., McKay, N., Mulitza, S., Strack, A., and Kucera, M.: PALMOD 130k marine palaeoclimate data synthesis V1.0, https://doi.org/10.1594/PANGAEA.908831, 2019.
Jonkers, L., Cartapanis, O., Langner, M., McKay, N., Mulitza, S., Strack, A., and Kucera, M.: Overview of the time series in the PALMOD 130k marine palaeoclimate data synthesis [Data set], Zenodo, https://doi.org/10.5281/zenodo.3739019, 2020.
Jullien, E., Grousset, F. E., Hemming, S. R., Peck, V. L., Hall, I. R., Jeantet, C., and Billy, I.: Contrasting conditions preceding MIS3 and MIS2 Heinrich events, Global Planet. Change, 54, 225–238, https://doi.org/10.1016/j.gloplacha.2006.06.021, 2006.
Jung, S. J. A.: Wassermassenaustausch zwischen NE-Atlantik und Nordmeer während der letzten 300.000/80.000 Jahre im Abbild stabiler 0- und C-lsotope, Christian-Albrechts-Universität zu Kiel0942-119X, Veränderungen der Umwelt-Der Nördliche Nordatlantik, 61, ISSN 0942, 1996.
Kageyama, M., Braconnot, P., Harrison, S. P., Haywood, A. M., Jungclaus, J. H., Otto-Bliesner, B. L., Peterschmitt, J.-Y., Abe-Ouchi, A., Albani, S., Bartlein, P. J., Brierley, C., Crucifix, M., Dolan, A., Fernandez-Donado, L., Fischer, H., Hopcroft, P. O., Ivanovic, R. F., Lambert, F., Lunt, D. J., Mahowald, N. M., Peltier, W. R., Phipps, S. J., Roche, D. M., Schmidt, G. A., Tarasov, L., Valdes, P. J., Zhang, Q., and Zhou, T.: The PMIP4 contribution to CMIP6 – Part 1: Overview and over-arching analysis plan, Geosci. Model Dev., 11, 1033–1057, https://doi.org/10.5194/gmd-11-1033-2018, 2018.
Kaiser, A.: Ozeanographie, Produktivität und Meereisverbreitung im Ochotskischen Meer während der letzten ca. 350 ka, PhD thesis, Mathematisch-Naturwissenschaftliche Fakultät, Christian-Albrechts-Universität zu Kiel, Kiel, Germany, 114 pp., 2001.
Kaufman, D., McKay, N., Routson, C., Erb, M., Davis, B., Heiri, O., Jaccard, S., Tierney, J., Dätwyler, C., Axford, Y., Brussel, T., Cartapanis, O., Chase, B., Dawson, A., de Vernal, A., Engels, S., Jonkers, L., Marsicek, J., Moffa-Sánchez, P., Morrill, C., Orsi, A., Rehfeld, K., Saunders, K., Sommer, P. S., Thomas, E., Tonello, M., Tóth, M., Vachula, R., Andreev, A., Bertrand, S., Biskaborn, B., Bringué, M., Brooks, S., Caniupán, M., Chevalier, M., Cwynar, L., Emile-Geay, J., Fegyveresi, J., Feurdean, A., Finsinger, W., Fortin, M.-C., Foster, L., Fox, M., Gajewski, K., Grosjean, M., Hausmann, S., Heinrichs, M., Holmes, N., Ilyashuk, B., Ilyashuk, E., Juggins, S., Khider, D., Koinig, K., Langdon, P., Larocque-Tobler, I., Li, J., Lotter, A., Luoto, T., Mackay, A., Magyari, E., Malevich, S., Mark, B., Massaferro, J., Montade, V., Nazarova, L., Novenko, E., Pařil, P., Pearson, E., Peros, M., Pienitz, R., Płóciennik, M., Porinchu, D., Potito, A., Rees, A., Reinemann, S., Roberts, S., Rolland, N., Salonen, S., Self, A., Seppä, H., Shala, S., St-Jacques, J.-M., Stenni, B., Syrykh, L., Tarrats, P., Taylor, K., van den Bos, V., Velle, G., Wahl, E., Walker, I., Wilmshurst, J., Zhang, E., and Zhilich, S.: A global database of Holocene paleotemperature records, Scientific Data, 7, 115, https://doi.org/10.1038/s41597-020-0445-3, 2020.
Kawamura, H., Holbourn, A., and Kuhnt, W.: Climate variability and land–ocean interactions in the Indo Pacific Warm Pool: A 460-ka palynological and organic geochemical record from the Timor Sea, Marine Micropaleontology, 59, 1–14, https://doi.org/10.1016/j.marmicro.2005.09.001, 2006.
Keigwin, L. D. and Boyle, E. A.: Surface and deep ocean variability in the northern Sargasso Sea during marine isotope stage 3, Paleoceanography, 14, 164–170, https://doi.org/10.1029/1998pa900026, 1999.
Keigwin, L. D. and Jones, G. A.: Glacial-Holocene stratigraphy, chronology, and paleoceanographic observations on some North Atlantic sediment drifts, Deep-Sea Res. Pt. I, 36, 845–867, https://doi.org/10.1016/0198-0149(89)90032-0, 1989.
Keigwin, L. D. and Jones, G. A.: Western North Atlantic evidence for millennial-scale changes in ocean circulation and climate, J. Geophys. Res., 99, 12397, https://doi.org/10.1029/94jc00525, 1994.
Kellogg, T. B., Duplessy, J. C., and Shackleton, N. J.: Planktonic foraminiferal and oxygen isotopic stratigraphy and paleoclimatology of Norwegian Sea deep-sea cores, Boreas, 7, 61–73, https://doi.org/10.1111/j.1502-3885.1978.tb00051.x, 1978.
Kennett, J. P., Roark, E. B., Cannariato, K. G., Ingram, B. L., and Tada, R.: Latest Quaternary paleoclimatic and radiocarbon chronology, Hole 1017E, southern California margin, in: Proceedings of the Ocean Drilling Program, Ocean Drilling Program, 2000.
Khider, D., Emile-Geay, J., McKay, N. P., Gil, Y., Garijo, D., Ratnakar, V., Alonso-Garcia, M., Bertrand, S., Bothe, O., Brewer, P., Bunn, A., Chevalier, M., Comas-Bru, L., Csank, A., Dassié, E., DeLong, K., Felis, T., Francus, P., Frappier, A., Gray, W., Goring, S., Jonkers, L., Kahle, M., Kaufman, D., Kehrwald, N. M., Martrat, B., McGregor, H., Richey, J., Schmittner, A., Scroxton, N., Sutherland, E., Thirumalai, K., Allen, K., Arnaud, F., Axford, Y., Barrows, T. T., Bazin, L., Pilaar Birch, S. E., Bradley, E., Bregy, J., Capron, E., Cartapanis, O., Chiang, H.-W., Cobb, K., Debret, M., Dommain, R., Du, J., Dyez, K., Emerick, S., Erb, M. P., Falster, G., Finsinger, W., Fortier, D., Gauthier, N., George, S., Grimm, E., Hertzberg, J., Hibbert, F., Hillman, A., Hobbs, W., Huber, M., Hughes, A. L. C., Jaccard, S., Ruan, J., Kienast, M., Konecky, B., Le Roux, G., Lyubchich, V., Novello, V. F., Olaka, L., Partin, J. W., Pearce, C., Phipps, S. J., Pignol, C., Piotrowska, N., Poli, M.-S., Prokopenko, A., Schwanck, F., Stepanek, C., Swann, G. E. A., Telford, R., Thomas, E., Thomas, Z., Truebe, S., von Gunten, L., Waite, A., Weitzel, N., Wilhelm, B., Williams, J., Williams, J. J., Winstrup, M., Zhao, N., and Zhou, Y.: PaCTS 1.0: A Crowdsourced Reporting Standard for Paleoclimate Data, Paleoceanography and Paleoclimatology, 1570–1596, https://doi.org/10.1029/2019pa003632, 2019.
Kiefer, T.: Produktivität und Temperaturen im subtropischen Nordatlantik: zyklische und abrupte Veränderungen im späten Quartär, Geologisch-Paläontologisches Institut und Museum, Christian-Albrechts-Universität, Kiel0175-9302, Kiel, 90, ISSN 0175-9302, 1998.
Kiefer, T., McCave, I. N., and Elderfield, H.: Antarctic control on tropical Indian Ocean sea surface temperature and hydrography, Geophys. Res. Lett., 33, L24612, https://doi.org/10.1029/2006gl027097, 2006.
Kienast, S. S., Calvert, S. E., and Pedersen, T. F.: Nitrogen isotope and productivity variations along the northeast Pacific margin over the last 120 kyr: Surface and subsurface paleoceanography, Paleoceanography, 17, 7-1–7-17, https://doi.org/10.1029/2001pa000650, 2002.
Kim, J.-H., Rimbu, N., Lorenz, S. J., Lohmann, G., Nam, S.-I., Schouten, S., Rühlemann, C., and Schneider, R. R.: North Pacific and North Atlantic sea-surface temperature variability during the Holocene, Quaternary Sci. Rev., 23, 2141–2154, https://doi.org/10.1016/j.quascirev.2004.08.010, 2004.
Kirst, G. J., Schneider, R. R., Müller, P. J., von Storch, I., and Wefer, G.: Late Quaternary Temperature Variability in the Benguela Current System Derived from Alkenones, Quaternary Res., 52, 92–103, https://doi.org/10.1006/qres.1999.2040, 1999.
Knies, J. and Stein, R.: New aspects of organic carbon deposition and its paleoceanographic implications along the Northern Barents Sea Margin during the last 30,000 years, Paleoceanography, 13, 384–394, https://doi.org/10.1029/98pa01501, 1998.
Knies, J. and Vogt, C.: Freshwater pulses in the eastern Arctic Ocean during Saalian and Early Weichselian ice-sheet collapse, Quaternary Res., 60, 243–251, https://doi.org/10.1016/j.yqres.2003.07.008, 2003.
Knies, J., Nowaczyk, N., Müller, C., Vogt, C., and Stein, R.: A multiproxy approach to reconstruct the environmental changes along the Eurasian continental margin over the last 150 000 years, Mar. Geol., 163, 317–344, https://doi.org/10.1016/s0025-3227(99)00106-1, 2000.
Konecky, B., Comas-Bru, L., Dassié, E., DeLong, K., and Partin, J. W.: Piecing together the big picture on water and climate, EOS, 99, https://doi.org/10.1029/2018EO095283, 2018.
Korte, C. and Hesselbo, S. P.: Shallow marine carbon and oxygen isotope and elemental records indicate icehouse-greenhouse cycles during the Early Jurassic, Paleoceanography, 26, PA4219, https://doi.org/10.1029/2011pa002160, 2011.
Koutavas, A. and Lynch-Stieglitz, J.: Glacial-interglacial dynamics of the eastern equatorial Pacific cold tongue-Intertropical Convergence Zone system reconstructed from oxygen isotope records, Paleoceanography, 18, 1089, https://doi.org/10.1029/2003pa000894, 2003.
Ku, T.-L., Bischoff, J. L., and Boersma, A.: Age studies of Mid-Atlantic Ridge sediments near 42∘ N and 20∘ N, Deep Sea Research and Oceanographic Abstracts, 19, 233–247, https://doi.org/10.1016/0011-7471(72)90033-2, 1972.
Kucera, M., Weinelt, M., Kiefer, T., Pflaumann, U., Hayes, A., Weinelt, M., Chen, M.-T., Mix, A. C., Barrows, T. T., Cortijo, E., Duprat, J., Juggins, S., and Waelbroeck, C.: Reconstruction of sea-surface temperatures from assemblages of planktonic foraminifera: multi-technique approach based on geographically constrained calibration data sets and its application to glacial Atlantic and Pacific Oceans, Quaternary Sci. Rev., 24, 951–998, 2005.
Kurahashi-Nakamura, T., Paul, A., and Losch, M.: Dynamical reconstruction of the global ocean state during the Last Glacial Maximum, Paleoceanography, 32, 326–350, https://doi.org/10.1002/2016pa003001, 2017.
Kurahashi-Nakamura, T., Paul, A., Munhoven, G., Merkel, U., and Schulz, M.: Coupling of a sediment diagenesis model (MEDUSA) and an Earth system model (CESM1.2): a contribution toward enhanced marine biogeochemical modelling and long-term climate simulations, Geosci. Model Dev., 13, 825–840, https://doi.org/10.5194/gmd-13-825-2020, 2020.
Labeyrie, L. D. and Duplessy, J. C.: Changes in the oceanic ratio during the last 140 000 years: High-latitude surface water records, Palaeogeography, Palaeoclimatology, Palaeoecology, 50, 217–240, https://doi.org/10.1016/0031-0182(85)90069-0, 1985.
Labeyrie, L., Vidal, L., Cortijo, E., Paterne, M., Arnold, M., Duplessy, J. C., Vautravers, M., Labracherie, M., Duprat, J., Turon, J. L., Grousset, F. E., and van Weering, T. C. E.: Surface and deep hydrology of the Northern Atlantic Ocean during the past 150000 years, Philos. T. R. Soc. B, 348, 255–264, https://doi.org/10.1098/rstb.1995.0067, 1995.
Labeyrie, L., Labracherie, M., Gorfti, N., Pichon, J. J., Vautravers, M., Arnold, M., Duplessy, J.-C., Paterne, M., Michel, E., Duprat, J., Caralp, M., and Turon, J.-L.: Hydrographic changes of the Southern Ocean (southeast Indian Sector) Over the last 230 kyr, Paleoceanography, 11, 57–76, https://doi.org/10.1029/95pa02255, 1996.
Labeyrie, L., Leclaire, H., Waelbroeck, C., Cortijo, E., Duplessy, J.-C., Vidal, L., Elliot, M., Le Coat, B., and Auffret, G.: Temporal variability of the surface and deep waters of the North West Atlantic Ocean at orbital and millenial scales, in: Mechanisms of Global Climate Change at Millennial Time Scales, American Geophysical Union, 1999.
Labracherie, M., Labeyrie, L. D., Duprat, J., Bard, E., Arnold, M., Pichon, J.-J., and Duplessy, J.-C.: The Last Deglaciation in the Southern Ocean, Paleoceanography, 4, 629–638, https://doi.org/10.1029/pa004i006p00629, 1989.
Lamy, F., Gersonde, R., Winckler, G., Esper, O., Jaeschke, A., Kuhn, G., Ullermann, J., Martinez-Garcia, A., Lambert, F., and Kilian, R.: Increased Dust Deposition in the Pacific Southern Ocean During Glacial Periods, Science, 343, 403–407, https://doi.org/10.1126/science.1245424, 2014.
Langner, M. and Mulitza, S.: Technical note: PaleoDataView – a software toolbox for the collection, homogenization and visualization of marine proxy data, Clim. Past, 15, 2067–2072, https://doi.org/10.5194/cp-15-2067-2019, 2019.
Latif, M., Claussen, M., Schulz, M., and Brüchner, T.: Comprehensive Earth system models of the last glacial cycle, EOS, 97, https://doi.org/10.1029/2016EO059587, 2016.
Lawrence, K. T.: Evolution of the Eastern Tropical Pacific Through Plio-Pleistocene Glaciation, Science, 312, 79–83, https://doi.org/10.1126/science.1120395, 2006.
Lea, D. W., Pak, D. K., Belanger, C. L., Spero, H. J., Hall, M. A., and Shackleton, N. J.: Paleoclimate history of Galápagos surface waters over the last 135,000 yr, Quaternary Sci. Rev., 25, 1152–1167, https://doi.org/10.1016/j.quascirev.2005.11.010, 2006.
Lear, C. H., Rosenthal, Y., and Slowey, N.: Benthic foraminiferal Mg/Ca-paleothermometry: a revised core-top calibration, Geochim. Cosmochim. Ac., 66, 3375–3387, https://doi.org/10.1016/S0016-7037(02)00941-9, 2002.
Lisiecki, L. E. and Raymo, M. E.: A Pliocene-Pleistocene stack of 57 globally distributed benthic δ18O records, Paleoceanography, 20, PA1003, https://doi.org/10.1029/2004pa001071, 2005.
Lisiecki, L. E. and Stern, J. V.: Regional and global benthic δ18O stacks for the last glacial cycle, Paleoceanography, 31, 1368–1394, https://doi.org/10.1002/2016PA003002, 2016.
Little, M. G., Schneider, R. R., Kroon, D., Price, B., Bickert, T., and Wefer, G.: Rapid palaeoceanographic changes in the Benguela Upwelling System for the last 160,000 years as indicated by abundances of planktonic foraminifera, Palaeogeography, Palaeoclimatology, Palaeoecology, 130, 135–161, https://doi.org/10.1016/s0031-0182(96)00136-8, 1997.
Liu, Z., Otto-Bliesner, B. L., He, F., Brady, E. C., Tomas, R., Clark, P. U., Carlson, A. E., Lynch-Stieglitz, J., Curry, W., Brook, E., Erickson, D., Jacob, R., Kutzbach, J., and Cheng, J.: Transient Simulation of Last Deglaciation with a New Mechanism for Bolling-Allerod Warming, Science, 325, 310–314, https://doi.org/10.1126/science.1171041, 2009.
Lo Giudice Cappelli, E., Holbourn, A., Kuhnt, W., and Regenberg, M.: Changes in Timor Strait hydrology and thermocline structure during the past 130 ka, Palaeogeography, Palaeoclimatology, Palaeoecology, 462, 112–124, https://doi.org/10.1016/j.palaeo.2016.09.010, 2016.
Lund, D. C. and Mix, A. C.: Millennial-scale deep water oscillations: Reflections of the North Atlantic in the deep Pacific from 10 to 60 ka, Paleoceanography, 13, 10–19, https://doi.org/10.1029/97pa02984, 1998.
Lyle, M., Zahn, R., Prahl, F., Dymond, J., Collier, R., Pisias, N., and Suess, E.: Paleoproductivity and carbon burial across the California Current: The multitracers transect, 42∘ N, Paleoceanography, 7, 251–272, https://doi.org/10.1029/92pa00696, 1992.
Lyle, M., Mix, A., Ravelo, A. C., Andreasen, D., Heusser, L., and Olivarez, A.: Millennial-scale CaCO3 and Corg events along the northern and central California margins: stratigraphy and origins, in: Proceedings of the Ocean Drilling Program, Ocean Drilling Program, 2000.
Lyle, M., Mix, A., and Pisias, N.: Patterns of CaCO3 deposition in the eastern tropical Pacific Ocean for the last 150 kyr: Evidence for a southeast Pacific depositional spike during marine isotope stage (MIS) 2, Paleoceanography, 17, 3-1–3-13, https://doi.org/10.1029/2000pa000538, 2002.
Lynch-Stieglitz, J., Curry, W. B., and Lund, D. C.: Florida Straits density structure and transport over the last 8000 years, Paleoceanography, 24, PA3209, https://doi.org/10.1029/2008pa001717, 2009.
Marchitto, T. M., Curry, W. B., Lynch-Stieglitz, J., Bryan, S. P., Cobb, K. M., and Lund, D. C.: Improved oxygen isotope temperature calibrations for cosmopolitan benthic foraminifera, Geochim. Cosmochim. Ac., 130, 1–11, https://doi.org/10.1016/j.gca.2013.12.034, 2014.
Marcott, S. A., Shakun, J. D., Clark, P. U., and Mix, A. C.: A Reconstruction of Regional and Global Temperature for the Past 11,300 Years, Science, 339, 1198–1201, https://doi.org/10.1126/science.1228026, 2013.
MARGO project: Constraints on the magnitude and patterns of ocean cooling at the Last Glacial Maximum, Nat. Geosci., 2, 127–132, 2009.
Martínez, I., Keigwin, L., Barrows, T. T., Yokoyama, Y., and Southon, J.: La Niña-like conditions in the eastern equatorial Pacific and a stronger Choco jet in the northern Andes during the last glaciation, Paleoceanography, 18, 1033, https://doi.org/10.1029/2002pa000877, 2003.
Martrat, B., Grimalt, J. O., Villanueva, J., van Kreveld, S., and Sarnthein, M.: Climatic dependence of the organic matter contributions in the north eastern Norwegian Sea over the last 15,000 years, Org. Geochem., 34, 1057–1070, https://doi.org/10.1016/s0146-6380(03)00084-6, 2003.
Martrat, B., Jimenez-Amat, P., Zahn, R., and Grimalt, J. O.: Similarities and dissimilarities between the last two deglaciations and interglaciations in the North Atlantic region, Quaternary Sci. Rev., 99, 122–134, https://doi.org/10.1016/j.quascirev.2014.06.016, 2014.
Max, L., Riethdorf, J.-R., Tiedemann, R., Smirnova, M., Lembke-Jene, L., Fahl, K., Nürnberg, D., Matul, A., and Mollenhauer, G.: Sea surface temperature variability and sea-ice extent in the subarctic northwest Pacific during the past 15,000 years, Paleoceanography, 27, PA3213, https://doi.org/10.1029/2012pa002292, 2012.
Max, L., Belz, L., Tiedemann, R., Fahl, K., Nürnberg, D., and Riethdorf, J.-R.: Rapid shifts in subarctic Pacific climate between 138 and 70 ka, Geology, 42, 899–902, https://doi.org/10.1130/g35879.1, 2014a.
Max, L., Lembke-Jene, L., Riethdorf, J.-R., Tiedemann, R., Nürnberg, D., Kühn, H., and Mackensen, A.: Pulses of enhanced North Pacific Intermediate Water ventilation from the Okhotsk Sea and Bering Sea during the last deglaciation, Clim. Past, 10, 591–605, https://doi.org/10.5194/cp-10-591-2014, 2014b.
McCave, I. N., Kiefer, T., Thornalley, D. J. R., and Elderfield, H.: Deep flow in the Madagascar–Mascarene Basin over the last 150000 years, Philos. T. R. Soc. A, 363, 81–99, https://doi.org/10.1098/rsta.2004.1480, 2005.
McKay, N. P. and Emile-Geay, J.: Technical note: The Linked Paleo Data framework – a common tongue for paleoclimatology, Clim. Past, 12, 1093–1100, https://doi.org/10.5194/cp-12-1093-2016, 2016.
McManus, J. F., Oppo, D. W., and Cullen, J. L.: A 0.5-Million-Year Record of Millennial-Scale Climate Variability in the North Atlantic, Science, 283, 971–975, https://doi.org/10.1126/science.283.5404.971, 1999.
Millo, C., Sarnthein, M., Voelker, A., and Erlenkeuser, H.: Variability of the Denmark Strait Overflow during the Last Glacial Maximum, Boreas, 35, 50–60, https://doi.org/10.1111/j.1502-3885.2006.tb01112.x, 2008.
Mix, A. C.: The oxygen-isotope record of glaciation, The Geology of North America, 3, 111–135, 1987.
Mix, A. C., Le, J., and Shackleton, N. J.: Benthic Foraminiferal Stable Isotope Stratigraphy of Site 846: 0–1.8 Ma, in: Proceedings of the Ocean Drilling Program, 138 Scientific Results, Ocean Drilling Program, 1995.
Moffa-Sánchez, P., Moreno-Chamarro, E., Reynolds, D. J., Ortega, P., Cunningham, L., Swingedouw, D., Amrhein, D. E., Halfar, J., Jonkers, L., Jungclaus, J. H., Perner, K., Wanamaker, A., and Yeager, S.: Variability in the Northern North Atlantic and Arctic Oceans Across the Last Two Millennia: A Review, Paleoceanography and Paleoclimatology, 1399–1436, https://doi.org/10.1029/2018pa003508, 2019.
Mollier-Vogel, E., Leduc, G., Böschen, T., Martinez, P., and Schneider, R. R.: Rainfall response to orbital and millennial forcing in northern Peru over the last 18 ka, Quaternary Sci. Rev., 76, 29–38, https://doi.org/10.1016/j.quascirev.2013.06.021, 2013.
Molyneux, E. G., Hall, I. R., Zahn, R., and Diz, P.: Deep water variability on the southern Agulhas Plateau: Interhemispheric links over the past 170 ka, Paleoceanography, 22, PA4209, https://doi.org/10.1029/2006pa001407, 2007.
Moreno, E., Thouveny, N., Delanghe, D., McCave, I. N., and Shackleton, N. J.: Climatic and oceanographic changes in the Northeast Atlantic reflected by magnetic properties of sediments deposited on the Portuguese Margin during the last 340 ka, Earth Planet. Sc. Lett., 202, 465-480, https://doi.org/10.1016/s0012-821x(02)00787-2, 2002.
Mortyn, P. G., Thunell, R. C., Anderson, D. M., Stott, L. D., and Le, J.: Sea surface temperature changes in the southern California borderlands during the last glacial-Interglacial cycle, Paleoceanography, 11, 415–429, https://doi.org/10.1029/96pa01236, 1996.
Mulitza, S., Prange, M., Stuut, J.-B., Zabel, M., von Dobeneck, T., Itambi, A. C., Nizou, J., Schulz, M., and Wefer, G.: Sahel megadroughts triggered by glacial slowdowns of Atlantic meridional overturning, Paleoceanography, 23, PA4206, https://doi.org/10.1029/2008pa001637, 2008.
Müller, J. and Stein, R.: High-resolution record of late glacial and deglacial sea ice changes in Fram Strait corroborates ice–ocean interactions during abrupt climate shifts, Earth Planet. Sc. Lett., 403, 446–455, https://doi.org/10.1016/j.epsl.2014.07.016, 2014.
Müller, J., Werner, K., Stein, R., Fahl, K., Moros, M., and Jansen, E.: Holocene cooling culminates in sea ice oscillations in Fram Strait, Quaternary Sci. Rev., 47, 1–14, https://doi.org/10.1016/j.quascirev.2012.04.024, 2012.
Nam, S.-I.: Late Quaternary glacial history and paleoceanographic reconstructions along the East Greenland continental margin: Evidence from high-resolution records of stable isotopes and ice-rafted debris (Spätquartäre Vereisungsgeschichte und paläozeanographische Rekonstruktionen am ostgrönlandischen Kontinentalrand), Alfred-Wegener-Institut für Polar- und Meeresforschung, 1997.
Nelson, C. S., Cooke, P. J., Hendy, C. H., and Cuthbertson, A. M.: Oceanographic and climatic changes over the past 160,000 years at Deep Sea Drilling Project Site 594 off southeastern New Zealand, southwest Pacific Ocean, Paleoceanography, 8, 435–458, https://doi.org/10.1029/93pa01162, 1993.
Niedermeyer, E. M., Prange, M., Mulitza, S., Mollenhauer, G., Schefuß, E., and Schulz, M.: Extratropical forcing of Sahel aridity during Heinrich stadials, Geophys. Res. Lett., 36, L20707, https://doi.org/10.1029/2009gl039687, 2009.
Nowaczyk, N. R., Antonow, M., Knies, J., and Spielhagen, R. F.: Further rock magnetic and chronostratigraphic results on reversal excursions during the last 50 ka as derived from northern high latitudes and discrepancies in precise AMS14C dating, Geophys. J. Int., 155, 1065–1080, https://doi.org/10.1111/j.1365-246x.2003.02115.x, 2003.
Nürnberg, D. and Tiedemann, R.: Environmental change in the Sea of Okhotsk during the last 1.1 million years, Paleoceanography, 19, PA4011, https://doi.org/10.1029/2004pa001023, 2004.
Nürnberg, D., Schönfeld, J., and Dullo, W.: RASTA Rapid climate changes in the western tropical Atlantic – Assessment of the biogenous and sedimentary record, R/V SONNE cruise report SO164, GEOMAR Forschungszentrum für Marine Geowissenschaften, 151, 2003.
Nürnberg, D., Ziegler, M., Karas, C., Tiedemann, R., and Schmidt, M. W.: Interacting Loop Current variability and Mississippi River discharge over the past 400 kyr, Earth Planet. Sc. Lett., 272, 278–289, https://doi.org/10.1016/j.epsl.2008.04.051, 2008.
Nürnberg, D., Böschen, T., Doering, K., Mollier-Vogel, E., Raddatz, J., and Schneider, R.: Sea surface and subsurface circulation dynamics off equatorial Peru during the last ∼17 kyr, Paleoceanography, 30, 984–999, https://doi.org/10.1002/2014pa002706, 2015.
Oba, T. and Murayama, M.: Sea-surface temperature and salinity changes in the northwest Pacific since the Last Glacial Maximum, J. Quaternary Sci., 19, 335–346, https://doi.org/10.1002/jqs.843, 2004.
Oppo, D. W. and Horowitz, M.: Glacial deep water geometry: South Atlantic benthic foraminiferal Cd/Ca and δ13C evidence, Paleoceanography, 15, 147–160, https://doi.org/10.1029/1999pa000436, 2000.
Oppo, D. W. and Lehman, S. J.: Suborbital timescale variability of North Atlantic Deep Water during the past 200,000 years, Paleoceanography, 10, 901–910, https://doi.org/10.1029/95pa02089, 1995.
Oppo, D. W. and Sun, Y.: Amplitude and timing of sea-surface temperature change in the northern South China Sea: Dynamic link to the East Asian monsoon, Geology, 33, 785–788, https://doi.org/10.1130/g21867.1, 2005.
Oppo, D. W., McManus, J. F., and Cullen, J. L.: Deepwater variability in the Holocene epoch, Nature, 422, p. 277, https://doi.org/10.1038/422277b, 2003.
Oppo, D. W., McManus, J. F., and Cullen, J. L.: Evolution and demise of the Last Interglacial warmth in the subpolar North Atlantic, Quaternary Sci. Rev., 25, 3268–3277, https://doi.org/10.1016/j.quascirev.2006.07.006, 2006.
Ortiz, J., Mix, A., Harris, S., and O'Connell, S.: Diffuse spectral reflectance as a proxy for percent carbonate content in North Atlantic sediments, Paleoceanography, 14, 171–186, https://doi.org/10.1029/1998pa900021, 1999.
PAGES2k Consortium, Emile-Geay, J., McKay, N. P., Kaufman, D. S., von Gunten, L., Wang, J., Anchukaitis, K. J., Abram, N. J., Addison, J. A., Curran, M. A. J., Evans, M. N., Henley, B. J., Hao, Z., Martrat, B., McGregor, H. V., Neukom, R., Pederson, G. T., Stenni, B., Thirumalai, K., Werner, J. P., Xu, C., Divine, D. V., Dixon, B. C., Gergis, J., Mundo, I. A., Nakatsuka, T., Phipps, S. J., Routson, C. C., Steig, E. J., Tierney, J. E., Tyler, J. J., Allen, K. J., Bertler, N. A. N., Björklund, J., Chase, B. M., Chen, M.-T., Cook, E., de Jong, R., DeLong, K. L., Dixon, D. A., Ekaykin, A. A., Ersek, V., Filipsson, H. L., Francus, P., Freund, M. B., Frezzotti, M., Gaire, N. P., Gajewski, K., Ge, Q., Goosse, H., Gornostaeva, A., Grosjean, M., Horiuchi, K., Hormes, A., Husum, K., Isaksson, E., Kandasamy, S., Kawamura, K., Kilbourne, K. H., Koç, N., Leduc, G., Linderholm, H. W., Lorrey, A. M., Mikhalenko, V., Mortyn, P. G., Motoyama, H., Moy, A. D., Mulvaney, R., Munz, P. M., Nash, D. J., Oerter, H., Opel, T., Orsi, A. J., Ovchinnikov, D. V., Porter, T. J., Roop, H. A., Saenger, C., Sano, M., Sauchyn, D., Saunders, K. M., Seidenkrantz, M.-S., Severi, M., Shao, X., Sicre, M.-A., Sigl, M., Sinclair, K., St. George, S., St. Jacques, J.-M., Thamban, M., Kuwar Thapa, U., Thomas, E. R., Turney, C., Uemura, R., Viau, A. E., Vladimirova, D. O., Wahl, E. R., White, J. W. C., Yu, Z., and Zinke, J.: A global multiproxy database for temperature reconstructions of the Common Era, Scientific Data, 4, 170088, https://doi.org/10.1038/sdata.2017.88 2017.
Pahnke, K.: 340,000-Year Centennial-Scale Marine Record of Southern Hemisphere Climatic Oscillation, Science, 301, 948–952, https://doi.org/10.1126/science.1084451, 2003.
Pahnke, K.: Southern Hemisphere Water Mass Conversion Linked with North Atlantic Climate Variability, Science, 307, 1741–1746, https://doi.org/10.1126/science.1102163, 2005.
Pahnke, K. and Sachs, J. P.: Sea surface temperatures of southern midlatitudes 0–160 kyr B.P, Paleoceanography, 21, PA2003, https://doi.org/10.1029/2005pa001191, 2006.
Pailler, D. and Bard, E.: High frequency palaeoceanographic changes during the past 140 000 yr recorded by the organic matter in sediments of the Iberian Margin, Palaeogeography, Palaeoclimatology, Palaeoecology, 181, 431–452, https://doi.org/10.1016/s0031-0182(01)00444-8, 2002.
Pedersen, T. F., Pickering, M., Vogel, J. S., Southon, J. N., and Nelson, D. E.: The response of benthic foraminifera to productivity cycles in the eastern equatorial Pacific: Faunal and geochemical constraints on glacial bottom water oxygen levels, Paleoceanography, 3, 157–168, https://doi.org/10.1029/pa003i002p00157, 1988.
Pedersen, T. F., Nielsen, B., and Pickering, M.: Timing of Late Quaternary productivity pulses in the Panama Basin and implications for atmospheric CO2, Paleoceanography, 6, 657–677, https://doi.org/10.1029/91pa02532, 1991.
Pelejero, C., Grimalt, J. O., Heilig, S., Kienast, M., and Wang, L.: High-resolution UK37 temperature reconstructions in the South China Sea over the past 220 kyr, Paleoceanography, 14, 224–231, https://doi.org/10.1029/1998pa900015, 1999.
Peterson, C. D. and Lisiecki, L. E.: Deglacial carbon cycle changes observed in a compilation of 127 benthic δ13C time series (20–6 ka), Clim. Past, 14, 1229–1252, https://doi.org/10.5194/cp-14-1229-2018, 2018.
Pichevin, L., Martinez, P., Bertrand, P., Schneider, R., Giraudeau, J., and Emeis, K.: Nitrogen cycling on the Namibian shelf and slope over the last two climatic cycles: Local and global forcings, Paleoceanography, 20, PA2006, https://doi.org/10.1029/2004pa001001, 2005.
Pichon, J.-J., Labeyrie, L. D., Bareille, G., Labracherie, M., Duprat, J., and Jouzel, J.: Surface water temperature changes in the high latitudes of the southern hemisphere over the Last Glacial-Interglacial Cycle, Paleoceanography, 7, 289–318, https://doi.org/10.1029/92pa00709, 1992.
Piotrowski, A. M., Goldstein, S. L., Hemming, S. R., and Fairbanks, R. G.: Intensification and variability of ocean thermohaline circulation through the last deglaciation, Earth Planet. Sc. Lett., 225, 205–220, https://doi.org/10.1016/j.epsl.2004.06.002, 2004.
Praetorius, S. K., McManus, J. F., Oppo, D. W., and Curry, W. B.: Episodic reductions in bottom-water currents since the last ice age, Nat. Geosci., 1, 449–452, https://doi.org/10.1038/ngeo227, 2008.
Prahl, F. G., Muehlhausen, L. A., and Zahnle, D. L.: Further evaluation of long-chain alkenones as indicators of paleoceanographic conditions, Geochim. Cosmochim. Ac., 52, 2303–2310, https://doi.org/10.1016/0016-7037(88)90132-9, 1988.
Reimer, P. J., Bard, E., Bayliss, A., Beck, J. W., Blackwell, P. G., Bronk Ramsey, C., Buck, C. E., Cheng, H., Edwards, R. L., Friedrich, M., Grootes, P. M., Guilderson, T. P., Haflidason, H., Hajdas, I., Hatté, C., Heaton, T. J., Hoffmann, D. L., Hogg, A. G., Hughen, K. A., Kaiser, K. F., Kromer, B., Manning, S. W., Niu, M., Reimer, R. W., Richards, D. A., Scott, E. M., Southon, J. R., Staff, R. A., Turney, C. S. M., and van der Plicht, J.: IntCal13 and Marine13 Radiocarbon Age Calibration Curves 0–50,000 Years cal BP, Radiocarbon, 55, 1869–1887, 2013.
Richter, T.: Sedimentary fluxes at the Mid-Atlantic Ridge: sediment sources, accumulation rates, and geochemical characterisation, GEOMAR Forschungszentrum für marine Geowissenschaften, 1998.
Rickaby, R. E. M. and Elderfield, H.: Evidence from the high-latitude North Atlantic for variations in Antarctic Intermediate water flow during the last deglaciation, Geochem. Geophy. Geosy., 6, Q05001, https://doi.org/10.1029/2004gc000858, 2005.
Riethdorf, J.-R., Max, L., Nürnberg, D., Lembke-Jene, L., and Tiedemann, R.: Deglacial development of (sub) sea surface temperature and salinity in the subarctic northwest Pacific: Implications for upper-ocean stratification, Paleoceanography, 28, 91–104, https://doi.org/10.1002/palo.20014, 2013a.
Riethdorf, J.-R., Nürnberg, D., Max, L., Tiedemann, R., Gorbarenko, S. A., and Malakhov, M. I.: Millennial-scale variability of marine productivity and terrigenous matter supply in the western Bering Sea over the past 180 kyr, Clim. Past, 9, 1345–1373, https://doi.org/10.5194/cp-9-1345-2013, 2013b.
Riethdorf, J.-R., Thibodeau, B., Ikehara, M., Nürnberg, D., Max, L., Tiedemann, R., and Yokoyama, Y.: Surface nitrate utilization in the Bering sea since 180 kA BP: Insight from sedimentary nitrogen isotopes, Deep-Sea Res. Pt. II, 125–126, 163–176, https://doi.org/10.1016/j.dsr2.2015.03.007, 2016.
Risebrobakken, B., Dokken, T., and Jansen, E.: Extent and variability of the meridional Atlantic circulation in the eastern Nordic seas during Marine Isotope Stage 5 and its influence on the inception of the last glacial, in: The Nordic Seas: An Integrated Perspective Oceanography, Climatology, Biogeochemistry, and Modeling, American Geophysical Union, 2005.
Roberts, J., Gottschalk, J., Skinner, L. C., Peck, V. L., Kender, S., Elderfield, H., Waelbroeck, C., Vázquez Riveiros, N., and Hodell, D. A.: Evolution of South Atlantic density and chemical stratification across the last deglaciation, P. Natl. Acad. Sci. USA, 113, 514–519, https://doi.org/10.1073/pnas.1511252113, 2016.
Romahn, S., Mackensen, A., Groeneveld, J., and Pätzold, J.: Deglacial intermediate water reorganization: new evidence from the Indian Ocean, Clim. Past, 10, 293–303, https://doi.org/10.5194/cp-10-293-2014, 2014.
Ronge, T. A., Steph, S., Tiedemann, R., Prange, M., Merkel, U., Nürnberg, D., and Kuhn, G.: Pushing the boundaries: Glacial/interglacial variability of intermediate and deep waters in the southwest Pacific over the last 350,000 years, Paleoceanography, 30, 23–38, https://doi.org/10.1002/2014pa002727, 2015.
Ronge, T. A., Tiedemann, R., Lamy, F., Köhler, P., Alloway, B. V., De Pol-Holz, R., Pahnke, K., Southon, J., and Wacker, L.: Radiocarbon constraints on the extent and evolution of the South Pacific glacial carbon pool, Nat. Commun., 7, 11487, https://doi.org/10.1038/ncomms11487, 2016.
Rosell-Melé, A. and Prahl, F. G.: Seasonality of temperature estimates as inferred from sediment trap data, Quaternary Sci. Rev., 72, 128–136, https://doi.org/10.1016/j.quascirev.2013.04.017, 2013.
Routson, C. C., McKay, N. P., Kaufman, D. S., Erb, M. P., Goosse, H., Shuman, B. N., Rodysill, J. R., and Ault, T.: Mid-latitude net precipitation decreased with Arctic warming during the Holocene, Nature, 568, 83–87, https://doi.org/10.1038/s41586-019-1060-3, 2019.
Rühlemann, C., Mulitza, S., Müller, P. J., Wefer, G., and Zahn, R.: Warming of the tropical Atlantic Ocean and slowdown of thermohaline circulation during the last deglaciation, Nature, 402, 511–514, https://doi.org/10.1038/990069, 1999.
Sachs, J. P. and Anderson, R. F.: Increased productivity in the subantarctic ocean during Heinrich events, Nature, 434, 1118–1121, https://doi.org/10.1038/nature03544, 2005.
Saikku, R., Stott, L., and Thunell, R.: A bi-polar signal recorded in the western tropical Pacific: Northern and Southern Hemisphere climate records from the Pacific warm pool during the last Ice Age, Quaternary Sci. Rev., 28, 2374–2385, https://doi.org/10.1016/j.quascirev.2009.05.007, 2009.
Salgueiro, E., Naughton, F., Voelker, A. H. L., de Abreu, L., Alberto, A., Rossignol, L., Duprat, J., Magalhães, V. H., Vaqueiro, S., Turon, J. L., and Abrantes, F.: Past circulation along the western Iberian margin: a time slice vision from the Last Glacial to the Holocene, Quaternary Sci. Rev., 106, 316–329, https://doi.org/10.1016/j.quascirev.2014.09.001, 2014.
Samson, C. R., Sikes, E. L., and Howard, W. R.: Deglacial paleoceanographic history of the Bay of Plenty, New Zealand, Paleoceanography, 20, PA4017, https://doi.org/10.1029/2004pa001088, 2005.
Sarnthein, M., Winn, K., Duplessy, J.-C., and Fontugne, M. R.: Global variations of surface ocean productivity in low and mid latitudes: Influence on CO2 reservoirs of the deep ocean and atmosphere during the last 21,000 years, Paleoceanography, 3, 361–399, https://doi.org/10.1029/pa003i003p00361, 1988.
Sarnthein, M., Winn, K., Jung, S. J. A., Duplessy, J.-C., Labeyrie, L., Erlenkeuser, H., and Ganssen, G.: Changes in East Atlantic Deepwater Circulation over the last 30,000 years: Eight time slice reconstructions, Paleoceanography, 9, 209–267, https://doi.org/10.1029/93pa03301, 1994.
Sarnthein, M., Gebhardt, H., Kiefer, T., Kucera, M., Cook, M., and Erlenkeuser, H.: Mid Holocene origin of the sea-surface salinity low in the subarctic North Pacific, Quaternary Sci. Rev., 23, 2089–2099, https://doi.org/10.1016/j.quascirev.2004.08.008, 2004.
Sarnthein, M., Kreveld, S., Erlenkeuser, H., Grootes, P. M., Kucera, M., Pflaumann, U., and Schulz, M.: Centennial-to-millennial-scale periodicities of Holocene climate and sediment injections off the western Barents shelf, 75∘ N, Boreas, 32, 447–461, https://doi.org/10.1111/j.1502-3885.2003.tb01227.x, 2008.
Sarnthein, M., Grootes, P. M., Holbourn, A., Kuhnt, W., and Kühn, H.: Tropical warming in the Timor Sea led deglacial Antarctic warming and atmospheric CO2 rise by more than 500 yr, Earth Planet. Sc. Lett., 302, 337–348, https://doi.org/10.1016/j.epsl.2010.12.021, 2011.
Sarnthein, M., Schneider, B., and Grootes, P. M.: Peak glacial 14C ventilation ages suggest major draw-down of carbon into the abyssal ocean, Clim. Past, 9, 2595–2614, https://doi.org/10.5194/cp-9-2595-2013, 2013.
Sarnthein, M., Balmer, S., Grootes, P. M., and Mudelsee, M.: Planktic and Benthic 14C Reservoir Ages for Three Ocean Basins, Calibrated by a Suite of 14C Plateaus in the Glacial-to-Deglacial Suigetsu Atmospheric 14C Record, Radiocarbon, 57, 129-151, https://doi.org/10.2458/azu_rc.57.17916, 2015.
Schlung, S. A., Christina Ravelo, A., Aiello, I. W., Andreasen, D. H., Cook, M. S., Drake, M., Dyez, K. A., Guilderson, T. P., LaRiviere, J. P., Stroynowski, Z., and Takahashi, K.: Millennial-scale climate change and intermediate water circulation in the Bering Sea from 90 ka: A high-resolution record from IODP Site U1340, Paleoceanography, 28, 54–67, https://doi.org/10.1029/2012pa002365, 2013.
Schönfeld, J., Zahn, R., and de Abreu, L.: Surface and deep water response to rapid climate changes at the Western Iberian Margin, Global Planet. Change, 36, 237–264, https://doi.org/10.1016/s0921-8181(02)00197-2, 2003.
Schulz, H.: Meeresoberflächentemperaturen vor 10.000 Jahren – Auswirkungen des frühholozänen Insolationsmaximums, Geologisch-Paläontologisches Institut und Museum, Christian-Albrechts-Universität, Kiel0175-9302, Kiel, 73, ISSN 0175-9302, 1995.
Shackleton, N. J. and Pisias, N. G.: Atmospheric Carbon Dioxide, Orbital Forcing, and Climate, in: The Carbon Cycle and Atmospheric CO2: Natural Variations Archean to Present, American Geophysical Union, 2013.
Shakun, J. D., Clark, P. U., He, F., Marcott, S. A., Mix, A. C., Liu, Z., Otto-Bliesner, B., Schmittner, A., and Bard, E.: Global warming preceded by increasing carbon dioxide concentrations during the last deglaciation, Nature, 484, 49–54, 2012.
Sierro, F. J., Hodell, D. A., Curtis, J. H., Flores, J. A., Reguera, I., Colmenero-Hidalgo, E., Bárcena, M. A., Grimalt, J. O., Cacho, I., Frigola, J., and Canals, M.: Impact of iceberg melting on Mediterranean thermohaline circulation during Heinrich events, Paleoceanography, 20, PA2019, https://doi.org/10.1029/2004pa001051, 2005.
Sikes, E. L., Howard, W. R., Neil, H. L., and Volkman, J. K.: Glacial-interglacial sea surface temperature changes across the subtropical front east of New Zealand based on alkenone unsaturation ratios and foraminiferal assemblages, Paleoceanography, 17, 2-1–2-13, https://doi.org/10.1029/2001pa000640, 2002.
Sikes, E. L., Howard, W. R., Samson, C. R., Mahan, T. S., Robertson, L. G., and Volkman, J. K.: Southern Ocean seasonal temperature and Subtropical Front movement on the South Tasman Rise in the late Quaternary, Paleoceanography, 24, PA2201, https://doi.org/10.1029/2008pa001659, 2009.
Simstich, J., Stanovoy, V., Bauch, D., Erlenkeuser, H., and Spielhagen, R. F.: Holocene variability of bottom water hydrography on the Kara Sea shelf (Siberia) depicted in multiple single-valve analyses of stable isotopes in ostracods, Mar. Geol., 206, 147–164, https://doi.org/10.1016/j.margeo.2004.01.008, 2004.
Simstich, J., Erlenkeuser, H., Harms, I., Spielhagen, R. F., and Stanovoy, V.: Modern and Holocene hydrographic characteristics of the shallow Kara Sea shelf (Siberia) as reflected by stable isotopes of bivalves and benthic foraminifera, Boreas, 34, 252–263, https://doi.org/10.1111/j.1502-3885.2005.tb01099.x, 2008.
Sirocko, F.: Processes controlling trace element geochemistry of Arabian Sea sediments during the last 25,000 years, Global Planet. Change, 26, 217–303, https://doi.org/10.1016/s0921-8181(00)00046-1, 2000.
Sirocko, F., Sarnthein, M., Lange, H., and Erlenkeuser, H.: Atmospheric summer circulation and coastal upwelling in the Arabian Sea during the Holocene and the last glaciation, Quaternary Res., 36, 72–93, https://doi.org/10.1016/0033-5894(91)90018-z, 1991.
Sirocko, F., Sarnthein, M., Erlenkeuser, H., Lange, H., Arnold, M., and Duplessy, J. C.: Century-scale events in monsoonal climate over the past 24,000 years, Nature, 364, 322–324, https://doi.org/10.1038/364322a0, 1993.
Skinner, L. C., Fallon, S., Waelbroeck, C., Michel, E., and Barker, S.: Ventilation of the Deep Southern Ocean and Deglacial CO2 Rise, Science, 328, 1147–1151, https://doi.org/10.1126/science.1183627, 2010.
Snyder, C. W.: Evolution of global temperature over the past two million years, Nature, 538, 226–228, https://doi.org/10.1038/nature19798, 2016.
Spero, H. J., Bijma, J., Lea, D. W., and Bemis, B. E.: Effect of seawater carbonate concentration on foraminiferal carbon and oxygen isotopes, Nature, 390, 497–500, 1997.
Spielhagen, R. F., Werner, K., Sorensen, S. A., Zamelczyk, K., Kandiano, E., Budeus, G., Husum, K., Marchitto, T. M., and Hald, M.: Enhanced Modern Heat Transfer to the Arctic by Warm Atlantic Water, Science, 331, 450–453, https://doi.org/10.1126/science.1197397, 2011.
Stott, L.: Super ENSO and Global Climate Oscillations at Millennial Time Scales, Science, 297, 222–226, https://doi.org/10.1126/science.1071627, 2002.
Stott, L., Timmermann, A., and Thunell, R.: Southern Hemisphere and Deep-Sea Warming Led Deglacial Atmospheric CO2 Rise and Tropical Warming, Science, 318, 435-438, https://doi.org/10.1126/science.1143791, 2007.
Stott, L. D.: Comment on “Anomalous radiocarbon ages for foraminifera shells” by W. Broecker et al.: A correction to the western tropical Pacific MD9821-81 record, Paleoceanography, 22, PA1211, https://doi.org/10.1029/2006pa001379, 2007.
Stott, L. D., Neumann, M., and Hammond, D.: Intermediate water ventilation on the Northeastern Pacific Margin during the Late Pleistocene inferred from benthic foraminiferal δ13C, Paleoceanography, 15, 161–169, https://doi.org/10.1029/1999pa000375, 2000.
Stuiver, M. and Polach, H. A.: Discussion Reporting of 14C Data, Radiocarbon, 19, 355–363, https://doi.org/10.1017/S0033822200003672, 1977.
Svensson, A., Andersen, K. K., Bigler, M., Clausen, H. B., Dahl-Jensen, D., Davies, S. M., Johnsen, S. J., Muscheler, R., Parrenin, F., Rasmussen, S. O., Röthlisberger, R., Seierstad, I., Steffensen, J. P., and Vinther, B. M.: A 60 000 year Greenland stratigraphic ice core chronology, Clim. Past, 4, 47–57, https://doi.org/10.5194/cp-4-47-2008, 2008.
Tada, R., Sato, S., Irino, T., Matsui, H., and Kennett, J. P.: Millennial-scale compositional variations in late Quaternary sediments at Site 1017, Southern California, in: Proceedings of the Ocean Drilling Program, Ocean Drilling Program, 2000.
Tapia, R., Nürnberg, D., Ronge, T., and Tiedemann, R.: Disparities in glacial advection of Southern Ocean Intermediate Water to the South Pacific Gyre, Earth Planet. Sc. Lett., 410, 152–164, https://doi.org/10.1016/j.epsl.2014.11.031, 2015.
Telesiński, M. M., Spielhagen, R. F., and Lind, E. M.: A high-resolution Lateglacial and Holocene palaeoceanographic record from the Greenland Sea, Boreas, 43, 273–285, https://doi.org/10.1111/bor.12045, 2013.
Telesiński, M. M., Spielhagen, R. F., and Bauch, H. A.: Water mass evolution of the Greenland Sea since late glacial times, Clim. Past, 10, 123–136, https://doi.org/10.5194/cp-10-123-2014, 2014.
Telford, R. J. and Birks, H. J. B.: A novel method for assessing the statistical significance of quantitative reconstructions inferred from biotic assemblages, Quaternary Sci. Rev., 30, 1272–1278, https://doi.org/10.1016/j.quascirev.2011.03.002, 2011.
Thomson, J., Nixon, S., Summerhayes, C. P., Schönfeld, J., Zahn, R., and Grootes, P.: Implications for sedimentation changes on the Iberian margin over the last two glacial/interglacial transitions from (230Thexcess)0 systematics, Earth Planet. Sc. Lett., 165, 255–270, https://doi.org/10.1016/s0012-821x(98)00265-9, 1999.
Thornalley, D. J. R., Elderfield, H., and McCave, I. N.: Intermediate and deep water paleoceanography of the northern North Atlantic over the past 21,000 years, Paleoceanography, 25, PA1211, https://doi.org/10.1029/2009pa001833, 2010a.
Thornalley, D. J. R., McCave, I. N., and Elderfield, H.: Freshwater input and abrupt deglacial climate change in the North Atlantic, Paleoceanography, 25, PA1201, https://doi.org/10.1029/2009pa001772, 2010b.
Thornalley, D. J. R., Elderfield, H., and McCave, I. N.: Reconstructing North Atlantic deglacial surface hydrography and its link to the Atlantic overturning circulation, Global Planet. Change, 79, 163–175, https://doi.org/10.1016/j.gloplacha.2010.06.003, 2011.
Ullermann, J., Lamy, F., Ninnemann, U., Lembke-Jene, L., Gersonde, R., and Tiedemann, R.: Pacific-Atlantic Circumpolar Deep Water coupling during the last 500 ka, Paleoceanography, 31, 639–650, https://doi.org/10.1002/2016pa002932, 2016.
Urey, H. C.: Oxygen isotopes in nature and in the laboratory, Science, 108, 489–496, 1948.
van Kreveld, S., Sarnthein, M., Erlenkeuser, H., Grootes, P., Jung, S., Nadeau, M. J., Pflaumann, U., and Voelker, A.: Potential links between surging ice sheets, circulation changes, and the Dansgaard-Oeschger Cycles in the Irminger Sea, 60–18 Kyr, Paleoceanography, 15, 425–442, https://doi.org/10.1029/1999pa000464, 2000.
Vidal, L., Schneider, R. R., Marchal, O., Bickert, T., Stocker, T. F., and Wefer, G.: Link between the North and South Atlantic during the Heinrich events of the last glacial period, Clim. Dynam., 15, 909–919, https://doi.org/10.1007/s003820050321, 1999.
Voelker, A. H. L. and workshop participants: Global distribution of centennial-scale records for Marine Isotope Stage (MIS) 3: a database, Quaternary Sci. Rev., 21, 1185–1212, https://doi.org/10.1016/S0277-3791(01)00139-1, 2002.
Voelker, A., Lebreiro, S., Schonfeld, J., Cacho, I., Erlenkeuser, H., and Abrantes, F.: Mediterranean outflow strengthening during northern hemisphere coolings: A salt source for the glacial Atlantic?, Earth Planet. Sc. Lett., 245, 39–55, https://doi.org/10.1016/j.epsl.2006.03.014, 2006.
Voelker, A. H. L., de Abreu, L., Schönfeld, J., Erlenkeuser, H., and Abrantes, F.: Hydrographic conditions along the western Iberian margin during marine isotope stage 2, Geochem. Geophy. Geosy., 10, Q12U08, https://doi.org/10.1029/2009gc002605, 2009.
Voelker, A. H. L. and de Abreu, L.: A Review of Abrupt Climate Change Events in the Northeastern Atlantic Ocean (Iberian Margin): Latitudinal, Longitudinal, and Vertical Gradients, in: Abrupt Climate Change: Mechanisms, Patterns, and Impacts, American Geophysical Union, 2011.
Vogelsang, E., Sarnthein, M., and Pflaumann, U.: δ18O Stratigraphy, chronology, and sea surface temperatures of Atlantic sediment records (GLAMAP-2000 Kiel), Institut für Geowissenschaften, Christian-Albrechts-Universität, Kiel, 0175-930 2, Institut für Geowissenschaften, 13, ISSN 0175-9302, 2001.
Waddell, L. M., Hendy, I. L., Moore, T. C., and Lyle, M. W.: Ventilation of the abyssal Southern Ocean during the late Neogene: A new perspective from the subantarctic Pacific, Paleoceanography, 24, PA3206, https://doi.org/10.1029/2008pa001661, 2009.
Waelbroeck, C., Labeyrie, L., Duplessy, J. C., Guiot, J., Labracherie, M., Leclaire, H., and Duprat, J.: Improving past sea surface temperature estimates based on planktonic fossil faunas, Paleoceanography, 13, 272–283, https://doi.org/10.1029/98pa00071, 1998.
Waelbroeck, C., Duplessy, J.-C., Michel, E., Labeyrie, L., Paillard, D., and Duprat, J.: The timing of the last deglaciation in North Atlantic climate records, Nature, 412, 724–727, https://doi.org/10.1038/35089060, 2001.
Waelbroeck, C., Labeyrie, L., Michel, E., Duplessy, J. C., McManus, J. F., Lambeck, K., Balbon, E., and Labracherie, M.: Sea-level and deep water temperature changes derived from benthic foraminifera isotopic records, Quaternary Sci. Rev., 21, 295–305, https://doi.org/10.1016/S0277-3791(01)00101-9, 2002.
Waelbroeck, C., Skinner, L. C., Labeyrie, L., Duplessy, J. C., Michel, E., Vazquez Riveiros, N., Gherardi, J. M., and Dewilde, F.: The timing of deglacial circulation changes in the Atlantic, Paleoceanography, 26, PA3213, https://doi.org/10.1029/2010pa002007, 2011.
Wang, L., Sarnthein, M., Erlenkeuser, H., Grimalt, J., Grootes, P., Heilig, S., Ivanova, E., Kienast, M., Pelejero, C., and Pflaumann, U.: East Asian monsoon climate during the Late Pleistocene: high-resolution sediment records from the South China Sea, Mar. Geol., 156, 245–284, https://doi.org/10.1016/s0025-3227(98)00182-0, 1999a.
Wang, L., Sarnthein, M., Grootes, P. M., and Erlenkeuser, H.: Millennial reoccurrence of century-scale abrupt events of East Asian Monsoon: A possible heat conveyor for the global deglaciation, Paleoceanography, 14, 725–731, https://doi.org/10.1029/1999pa900028, 1999b.
Weaver, P. P. E., Carter, L., and Neil, H. L.: Response of surface water masses and circulation to Late Quaternary climate change east of New Zealand, Paleoceanography, 13, 70–83, https://doi.org/10.1029/97pa02982, 1998.
Weber, M. E., Mayer, L. A., Hillaire-Marcel, C., Bilodeau, G., Rack, F., Hiscott, R. N., and Aksu, A. E.: Derivation of δ18O from sediment core log data: Implications for millennial-scale climate change in the Labrador Sea, Paleoceanography, 16, 503–514, https://doi.org/10.1029/2000pa000560, 2001.
Weinelt, M., Rosell-Melé, A., Pflaumann, U., Sarnthein, M., and Kiefer, T.: The role of productivity in the Northeast Atlantic on abrupt climate change over the last 80,000 years, Zeitschrift der Deutschen Geologischen Gesellschaft, 154, 47–66, https://doi.org/10.1127/zdgg/154/2003/47, 2003.
Weitzel, N., Wagner, S., Sjolte, J., Klockmann, M., Bothe, O., Andres, H., Tarasov, L., Rehfeld, K., Zorita, E., Widmann, M., Sommer, P., Schädler, G., Ludwig, P., Kapp, F., Jonkers, L., García-Pintado, J., Fuhrmann, F., Dolman, A., Dallmeyer, A., and Brücher, T.: Diving into the Past: A Paleo Data–Model Comparison Workshop on the Late Glacial and Holocene, B. Am. Meteorol. Soc., 100, ES1–ES4, https://doi.org/10.1175/bams-d-18-0169.1, 2019.
Wells, P. and Okada, H.: Response of nannoplankton to major changes in sea-surface temperature and movements of hydrological fronts over Site DSDP 594 (south Chatham Rise, southeastern New Zealand), during the last 130 kyr, Mar. Micropaleontol., 32, 341–363, https://doi.org/10.1016/s0377-8398(97)00025-x, 1997.
Werner, K., Spielhagen, R. F., Bauch, D., Hass, H. C., Kandiano, E., and Zamelczyk, K.: Atlantic Water advection to the eastern Fram Strait – Multiproxy evidence for late Holocene variability, Palaeogeography, Palaeoclimatology, Palaeoecology, 308, 264–276, https://doi.org/10.1016/j.palaeo.2011.05.030, 2011.
Werner, K., Spielhagen, R. F., Bauch, D., Hass, H. C., and Kandiano, E.: Atlantic Water advection versus sea-ice advances in the eastern Fram Strait during the last 9 ka: Multiproxy evidence for a two-phase Holocene, Paleoceanography, 28, 283–295, https://doi.org/10.1002/palo.20028, 2013.
Werner, K., Müller, J., Husum, K., Spielhagen, R. F., Kandiano, E. S., and Polyak, L.: Holocene sea subsurface and surface water masses in the Fram Strait – Comparisons of temperature and sea-ice reconstructions, Quaternary Sci. Rev., 147, 194–209, https://doi.org/10.1016/j.quascirev.2015.09.007, 2016.
Wollenburg, J. E., Kuhnt, W., and Mackensen, A.: Changes in Arctic Ocean paleoproductivity and hydrography during the last 145 kyr: The benthic foraminiferal record, Paleoceanography, 16, 65–77, https://doi.org/10.1029/1999pa000454, 2001.
Xu, J., Kuhnt, W., Holbourn, A., Andersen, N., and Bartoli, G.: Changes in the vertical profile of the Indonesian Throughflow during Termination II: Evidence from the Timor Sea, Paleoceanography, 21, PA4202, https://doi.org/10.1029/2006pa001278, 2006.
Xu, J., Holbourn, A., Kuhnt, W., Jian, Z., and Kawamura, H.: Changes in the thermocline structure of the Indonesian outflow during Terminations I and II, Earth Planet. Sc. Lett., 273, 152–162, https://doi.org/10.1016/j.epsl.2008.06.029, 2008.
Zahn-Knoll, R.: Spätquartäre Entwicklung von Küstenauftrieb und Tiefenwasserzirkulation im Nordost-Atlantik, Rekonstruktion anhand stabiler Isotope kalkschaliger Foraminiferen., Geologisch-Paläontologisches Institut, Christian-Albrechts-Universität, Kiel, Germany, 111 pp., 1986.
Zarriess, M. and Mackensen, A.: The tropical rainbelt and productivity changes off northwest Africa: A 31,000-year high-resolution record, Mar. Micropaleontol., 76, 76–91, https://doi.org/10.1016/j.marmicro.2010.06.001, 2010.
Zarriess, M. and Mackensen, A.: Testing the impact of seasonal phytodetritus deposition on δ13C of epibenthic foraminiferCibicidoides wuellerstorfi: A 31,000 year high-resolution record from the northwest African continental slope, Paleoceanography, 26, PA2202, https://doi.org/10.1029/2010pa001944, 2011.
Zarriess, M., Johnstone, H., Prange, M., Steph, S., Groeneveld, J., Mulitza, S., and Mackensen, A.: Bipolar seesaw in the northeastern tropical Atlantic during Heinrich stadials, Geophys. Res. Lett., 38, L04706, https://doi.org/10.1029/2010gl046070, 2011.
Ziegler, M., Nürnberg, D., Karas, C., Tiedemann, R., and Lourens, L. J.: Persistent summer expansion of the Atlantic Warm Pool during glacial abrupt cold events, Nat. Geosci., 1, 601–605, https://doi.org/10.1038/ngeo277, 2008.
Zuraida, R., Holbourn, A., Nürnberg, D., Kuhnt, W., Dürkop, A., and Erichsen, A.: Evidence for Indonesian Throughflow slowdown during Heinrich events 3–5, Paleoceanography, 24, PA2205, https://doi.org/10.1029/2008pa001653, 2009.
- Abstract
- Introduction
- Data synthesis strategy
- Notes on palaeoclimate proxies in marine-sediment archives and metadata
- Structure of the database
- PalMod 130k marine palaeoclimate data synthesis v1.0.0 contents
- Data formats
- Future plans and versioning
- Data availability
- Lessons learned: recommendations for data archiving
- Appendix A
- Author contributions
- Competing interests
- Special issue statement
- Acknowledgements
- Financial support
- Review statement
- References
- Supplement
- Abstract
- Introduction
- Data synthesis strategy
- Notes on palaeoclimate proxies in marine-sediment archives and metadata
- Structure of the database
- PalMod 130k marine palaeoclimate data synthesis v1.0.0 contents
- Data formats
- Future plans and versioning
- Data availability
- Lessons learned: recommendations for data archiving
- Appendix A
- Author contributions
- Competing interests
- Special issue statement
- Acknowledgements
- Financial support
- Review statement
- References
- Supplement