the Creative Commons Attribution 4.0 License.
the Creative Commons Attribution 4.0 License.
An organic matter database (OMD): consolidating global residue data from agriculture, fisheries, forestry and related industries
Gudeta Weldesemayat Sileshi
Johannes Lehmann
Francesco Nicola Tubiello
Agricultural, fishery, forestry and agro-processing activities produce large quantities of residues, by-products and waste materials every year. Inappropriate disposal and inefficient use of these resources contribute to greenhouse gas emissions and non-point pollution, placing significant environmental and economic burdens on society. Since many nations do not keep statistics on these materials, it has not been possible to accurately quantify the amounts produced, their competing uses and the quantities potentially available for recycling at a local level. Therefore, the objectives of the present work were to provide (1) definitions, typologies and methods to aid consistent classification, estimation and reporting of the various residues and by-products, (2) a global organic matter database (OMD) of residues and by-products from agriculture, fisheries, forestry and related industries and (3) regional and global estimates of residues and by-products potentially available for use in a circular bio-economy. To the best of our knowledge, the OMD is the first of its kind to consolidate quantities and nutrient concentrations of residues and by-products globally from agriculture, fisheries, forestry and related industries (available at https://doi.org/10.5281/zenodo.10450921; Sileshi et al., 2024). The OMD will be updated continuously as new production data are published in FAOSTAT and country-specific conversion coefficients become available. This information is expected to contribute to evidence-based policies and actions in support of sustainable utilization and the transition towards a circular economy. The database could be used for a variety of purposes, including estimation of residue availability for soil amendment, livestock feed, bioenergy and other industrial applications as well as assessment of the environmental impacts of residue management practices such as soil application and burning. The estimates in the OMD are only available at the national level. Due to the lack of a uniform methodology, conversion coefficients and data on competing uses across countries, it was difficult to accurately estimate the quantities of all agricultural, fishery and forestry residues and by-products. Therefore, we strongly recommend investment in the inventory of agricultural, fishery and forestry residues as well as by-products and wastes at the national and sub-national levels for use in a circular bio-economy.
- Article
(440 KB) - Full-text XML
- BibTeX
- EndNote
Agricultural, fishery, forestry and agro-processing activities produce large quantities of residues, by-products and waste materials every year (Gontard et al., 2018; Lopes and Ligabue-Braun, 2021; Millati et al., 2019). Some of these residues are already being used in a variety of effective ways, such as animal feed and bioenergy feedstock. However, a large proportion of the residues and by-products is either burnt or inappropriately disposed of without further use (Domingues et al., 2017; FAO, 2022a; Venkatramanan et al., 2021). This has been widely documented as causing environmental pollution and the spread of disease. For example, manure produced on large-scale dairy, poultry and pig farms continues to cause non-point pollution where disposal is not well-regulated (Marin et al., 2023; Wang et al., 2017). Similarly, slaughterhouse residues and agro-processing wastes are often disposed of in open dumps and landfills, where they become a significant source of greenhouse gas (GHG) emissions (Mozhiarasi and Natarajan, 2025). Some of the waste from slaughterhouses and agro-processing plants is discharged as effluents into water bodies (Al-Gheethi et al., 2021). The burning of crop residues is a major contributor to dangerously high levels of air pollution and emissions of greenhouse gases (FAO, 2022a; Oanh et al., 2018; Venkatramanan et al., 2021). In 2019 alone, around 458×106 t of crop residue was burnt globally, resulting in 1238 kt of methane (CH4) and 32 kt of nitrous oxide (N2O) emissions (FAO, 2022a). Burning agricultural residue also results in substantial losses of nutrients in the residue. For example, burning crop residues results in nearly complete loss of organic carbon and nitrogen, 25 % of phosphorus, 20 % of potassium and 5 %–60 % of sulfur (Dobermann and Fairhurst, 2002). In addition to causing air pollution and respiratory ailments in humans, burning takes away opportunities to add value to crop residues (Lin and Begho, 2022; Oanh et al., 2018; Venkatramanan et al., 2021). Indeed, inefficient use of agricultural residues and wastes places significant environmental and economic burdens on society (Gontard et al., 2018). Conversely, a significant portion of these residues could enter a circular bio-economy, and their efficient use can ensure achievement of sustainability goals through reduction of GHG emissions and non-point pollution.
Agricultural residues are used in many different ways, including soil amendment, animal feed and bedding, bioenergy generation, as fuel in industrial and domestic set-ups, mushroom production, industrial applications such as textiles, natural fibres, polymers, biosorbents and reinforcement material in composites (Siqueira et al., 2022; Smerald et al., 2023; Zhao et al., 2024; see also the discussion in Sect. 4.1–4.4). A growing body of evidence suggests that currently unused residues could in principle be more effectively managed to increase soil fertility and productivity and to mitigate greenhouse gas emissions (Lu et al., 2015).
Over the years, agriculture has depended increasingly on synthetic fertilizers to meet crop nutrient demands. Increased fertilizer use and inefficient fertilizer management practices have led to large nutrient losses to the environment in some regions (FAO, 2022b; Singh and Craswell, 2021). On the other hand, farmers in low-income countries have limited access to fertilizer inputs, and this has led to depletion of native soil nutrient stocks from croplands. Access to fertilizers has been limited further by the recent war by Russia on Ukraine, which disrupted a large portion of the global fertilizer supply. The resultant increases in prices are likely to constrain fertilizer use by farmers in the foreseeable future (FAO, 2022c; Behnassi and El Haiba, 2022). In 2020, the production and use of synthetic fertilizers resulted in GHG emissions of 1.0 Gt CO2, of which 62 % (or 0.63 Gt CO2) is emitted when nitrogen fertilizers are used on croplands (FAO, 2022d; Tubiello et al., 2022).
A growing body of evidence from meta-analyses suggests that the combined use of organic inputs and inorganic fertilizers can increase fertilizer use efficiency (Bai et al., 2022; Chivenge et al., 2011; Sileshi et al., 2019; Wang et al., 2020; Zhang et al., 2020; Melo et al., 2022). There is also a growing consensus that judicious use of agricultural residues can partially substitute inorganic fertilizers (Fan et al., 2021; Huang et al., 2013; Zhang et al., 2020) and thereby contribute to enhancing the sustainability of food production by reducing costs and carbon footprints while reducing pollution caused by nitrate leaching (Zhang et al., 2020). The savings from recycling agricultural residues and wastes can also be an important contribution to national and local economies. Recycling of organic residues, by-products and wastes can also address waste management problems and reduce GHG emissions from residues and wastes (Andrews et al., 2021; FAO, 2022a).
However, the potential contributions of agricultural, fishery and forestry residues and by-products to soil health improvement and carbon management have not been estimated fully. This is largely due to a lack of country statistics on the production of residues and by-products from agriculture, fisheries and forestry, which makes it difficult to accurately quantify the amounts produced and available for recycling. The designation of residues as a resource, by-product or waste may also not always align with how the material is subsequently managed or its potential utility as a soil amendment. For example, livestock manure may be classified as a waste in some jurisdictions but not in others, whether or not it is subsequently used as an organic fertilizer. Importantly, a clear typology of residues and by-products also does not exist in many regions. This hinders the systematic documentation and reporting of the different categories of organic resources. Information is also scant on the quality of most of the residues produced. The quality of the organic resources varies with the plant species, plant parts and their maturity level (Palm et al., 2001; Cobo et al., 2002), and determination of the quality attributes using traditional laboratory methods is both timely and costly (Shepherd et al., 2003). Despite these challenges, Palm et al. (2001) published an organic resource database containing data on plant species and plant parts, resource quality, decomposition rates, N release rates, digestibility indices and site characteristics. Rapid plant nutrient analysis based on spectroscopic methods has been developed (Shepherd et al., 2003) and complemented by methods assessing functional differences (e.g. carbon and nitrogen release rates as well as digestibility) (Vanlauwe et al., 2005). Additional efforts to make these organic resource data useful included a decision support system for different categories of organic resources (Palm et al., 2001; Vanlauwe et al., 2005). A related effort is the Phyllis database developed by the Energy Research Centre of the Netherlands (ECN, 2018) that primarily focuses on biomass properties that are relevant to bioenergy and biochar production. Data on primary crop and animal products are available through FAOSTAT, but equivalent data for quantities of residues are not available (Ludemann et al., 2023; Woolf, 2020).
In 2020 the Food and Agriculture Organization (FAO) of the United Nations commissioned a scoping study to assess the state of organic resource databases in the agriculture sector and related industries (Woolf, 2020). The study arrived at the following conclusions. (1) Large uncertainties exist in the annual production of crop residues. (2) The fates and uses of residues and wastes are poorly quantified in many regions of the world. (3) Existing decision tools and classification schemes for residue biomass are not well suited to allocating resources in a comprehensive portfolio. (4) Data on residue biomass composition and properties are diffuse, have large gaps and rarely relate composition to production conditions. (5) Paucity of data on residue biomass production, composition and fate is a critical constraint on improving resource use efficiency (Woolf, 2020). Further, the study recommended the development of a global biomass resource database to support sustainable development goals. Therefore, a global database providing estimates of the different residues and by-products is urgently needed for practitioners and policy-makers to quickly refer to when making decisions. Accordingly, the objectives of the present work were to provide (1) definitions, typologies and methods to aid consistent classification, estimation and reporting of various residues and by-products, (2) a global organic matter database of residues and by-products from agriculture, fisheries, forestry and related industries and (3) regional and global estimates of residues and by-products that are potentially available for use in a circular bio-economy. Wherever possible, this work also tried to highlight the competing uses of the various residues and the challenges and opportunities for their uses as soil amendments. The database could be used for a variety of purposes, including estimation of the availability of residues for livestock feed, soil amendment, bioenergy production, industrial applications and assessment of environmental impacts (e.g. pollution, greenhouse gas emissions and nutrient flows) of residue management practices (e.g. residue retention, burning and disposal).
To guide the development of the OMD, a review of the literature was compiled. This was aimed at identifying the various categories and a typology (systematic classification) of organic residues and by-products, their competing uses and the challenges and opportunities for their use as soil amendments. The review also aimed at identifying industry best practices and conversion factors for estimating agricultural, fishery and forestry residues as well as agro-processing by-products.
2.1 Data used to create the OMD
The OMD was designed to provide data on both the quantity and quality of residues and by-products (Sileshi et al., 2024; https://doi.org/10.5281/zenodo.10450921). Residue datasets were estimated from the FAOSTAT and FishStatJ databases. FAOSTAT provides free access to historical data on food, agriculture, forestry, trade and land use for over 200 countries and territories. Data on the production of primary crop and animal products were extracted from FAOSTAT's Crop and Livestock Products database (https://www.fao.org/faostat/en/#data/QCL, last access: 21 June 2023), while data on forestry residues came from FAOSTAT's Forestry Production and Trade database (https://www.fao.org/faostat/en/#data/FO, last access: 21 June 2023) (FAOSTAT, 2023). In the case of capture fisheries and aquaculture, production data (in tonnes live weight) came from the FAO's FishStatJ statistical software (https://www.fao.org/fishery/static/FishStatJ, last access: 21 June 2023) for the period 2015–2019 for selected species in each country and territory. In all cases, production refers to the total quantity produced per country in a given year.
Not only the quantity, but also the quality, of residues is important for their use in soil amendment. Therefore, a supplementary database was created that consolidates data on the nutrient concentrations of various residues to complement the OMD. The concentrations of carbon, macronutrients (nitrogen, phosphorus and potassium), micronutrients (sulfur, calcium and magnesium), lignin, polyphenols and ratios for crop residues and manure were compiled from existing databases (e.g. the Cornell Substrate Composition Table, FAOSTAT and the Phyllis database), International Panel on Climate Change (IPCC) default values (Hergoualc'h et al., 2019) and the scientific literature (e.g. Ludemann et al., 2023, on crop residues; Shen et al., 2015, and Sileshi et al., 2017, on manure). Wherever available, the range of values (minimum and maximum) available in the OMD and IPCC default values are summarized in Table 1. All values were reported on a dry-matter basis. The moisture contents of most residues were not reported in the original publications, and therefore values should be used with caution.
Table 1Range of values (minimum and maximum) reported for the carbon, nitrogen (N), phosphorus (P), potassium (K), calcium (Ca) and magnesium (Mg) concentrations of crop residues and manure (on a dry-matter basis). Values were summarized from the OMD supplementary database available at https://doi.org/10.5281/zenodo.10450921 (Sileshi et al., 2024).
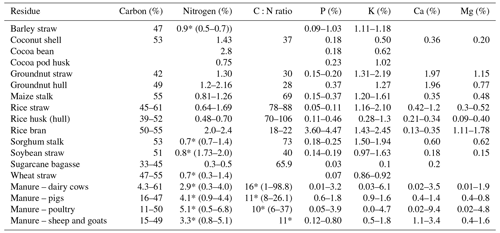
*IPCC default values.
2.2 Definitions and typology
The literature reviewed identified many sources of organic input that can be used for soil amendment. These include crop residues, agro-processing by-products, forestry and logging industry residues, manure, poultry and meat processing and fishery and aquaculture by-products. Authors have used the terms “residue”, “by-product”, “co-product” and “waste” when referring to various organic resources. Therefore, it was necessary to provide clear definitions and typologies (systematic classification) to facilitate their consistent estimation and compilation in the OMD. A clear definition could only be found in relation to an existing EU directive (European Parliament and Council, 2008; 2008/98/EC) that was adopted herein. Accordingly, a by-product is defined as a substance or object whose primary aim is not the production of that item, whereas waste is defined as any substance or object which the holder discards, intends to discard or is required to discard. According to the directive, an object or substance should be regarded as a by-product only when certain conditions are met as specified under Article 5. In this paper, this norm was followed, and the term “by-product” was used consistently to refer to side products originating from the food manufacturing stage. By-products may be products of either primary or secondary processing of crops and animals that are available at breweries, wineries, milling and refining facilities or slaughterhouses and fish processing facilities (Lopes and Ligabue-Braun, 2021). Wastes were not included in the OMD as they consist of a wide variety of materials whose disposal may be required in accordance with local legislation. Crop residues, agro-processing by-products, manure and forestry residues were included in the OMD.
2.2.1 Crop residues
Crop residues were defined as plant parts left in the field after harvest, including straw of cereals, pods and stems of legumes, tops, stalks, leaves and shoots of tuber crops, oil crops, sugar crops, vegetable crops and pruning and litter of fruit and nut trees.
2.2.2 Agro-processing by-products
Agro-processing by-products were defined as products from the food and agriculture industry (Lopes and Ligabue-Braun, 2021). According to the literature reviewed, the main sources of agro-processing by-products are cereal processing, sugar processing, breweries, the beverage industry, oil presses, bioenergy production, slaughterhouse by-products and fish processing by-products, which are defined further below.
Cereal processing by-products
Cereal processing by-products are defined here as the by-products of rice milling and the multi-stage process of flour production from cereals such as wheat, rice and maize. In the milling process of rice, the husk (hull), which is the outer cover of the grain, is removed. Further milling removes the bran, resulting in white rice. Rice husk constitutes about 20 % of the dry weight of rice grains (Singh, 2018). The bran is the part of the cereal grain that could be used in a further milling process or as a biorefinery feedstock (Caldeira et al., 2020).
Sugar industry by-products
The by-products from the sugar industry include bagasse, sugar beet pulp, molasses, and filter press mud, which are available at the milling and refining facilities. Bagasse is the fibrous residue remaining after the milling of cane stalks for juice extraction, and it constitutes roughly 27 %–28 % of the dry weight of the plant biomass (Bezerra and Ragauskas, 2016). The residue-to-product ratios (RPRs) of bagasse were reported to vary from 0.14 to 1.16 (Koopmans and Koppejan, 1998).
Brewery and winery by-products
Spent grain and grape pomace is the main by-product of the brewery and winery industries, respectively. Barley grain is the main raw material for beer, and ∼20 kg of a brewer's wet spent grains are produced per 100 L of beer produced (Gonzalez-Garcia et al., 2018). Approximately 75 % of grapes produced are intended for wine production, of which 20 %–30 % are grape pomace consisting of the skin, pulp, seeds and stalks (Antonić et al., 2020).
Beverage industry by-products
The beverage industry manufactures ready-to-drink products such as fruit juice, cocoa, coffee and tea-based products, soft drinks, energy drinks, milk products and nutritional beverages. The by-products of fruit processing include peels, skin, rind and seeds. The main by-products of cocoa processing are cocoa pod husks, cocoa bean shells and cocoa mucilage. In the initial stage of cocoa processing, 70 %–80 % of the fruit is discarded, and approximately 10 t of shells are generated for each tonne of cocoa (Dutra et al., 2023).
In making the coffee beverage, approximately 90 % dry matter of the coffee cherry is discarded in the form of husks, parchments, mucilage, silver skin and spent coffee grounds (Iriondo-DeHond et al., 2020). On a wet-weight basis, in 100 kg of mature coffee cherries, 39 kg corresponds to the skin and pulp, and 22 kg of mucilage and about 39 kg of parchment are generated (Iriondo-DeHond et al., 2020).
Oil processing by-products
The main oil crops are oil palm, coconut, groundnut, soybean and olives. By-products from palm oil mills include empty fruit bunches (EFBs), palm oil mill effluent, decanter cake, seed shells and fibre from the mesocarp. A hectare of oil palm produces 10–35 t of fresh fruit bunches (FFBs) per year on a wet-weight basis. EFBs, fibre, shells and decanter cake account for 30 %, 6 %, 3 % and 29 % of the FFBs (Embrandiri et al., 2012). EFBs form the residue left after the processing of fresh fruit bunches at the mill. Palm press fibre (PPF) or mesocarp fibre is produced after pressing fruit or the mesocarp to obtain oil. On average, for every tonne of FFBs processed, 120 kg of fibre is produced on a wet-weight basis (Embrandiri et al., 2012). Palm kernel shells (PKSs) are difficult to decompose, and they have been used as mulch. Decanter cake is another waste product used as either fertilizer or animal food. Palm oil mill effluent is the outcome of oil extraction, washing and cleaning processes in mills. On a wet-weight basis, about 3 t of oil mill effluent is produced for every tonne of oil extracted in an oil mill.
Coconut consists of husks (33 %–35 %), shells (12 %–15 %) and copra (28 %–30 %) on a wet-weight basis. According to Onwudike (1996), about 2220 kg of dry husks and 1040 kg of dry shells become available per hectare per year. Lim (1986) gives figures of 5280 kg of dry husks and 2510 kg of dry shells per hectare per year in large-scale estates. Copra production ranges from 0.5–1 t per hectare per year with traditional harvesting on smallholdings to 3–9 t per hectare for improved clonal varieties and intensive management (Lim, 1986).
The processing of groundnut oil produces a large portion of peanut meal as a by-product, together with skins and hulls. On a wet-weight basis, 1000 kg of peanuts can generate about 500–700 kg of peanut meal, depending on the procedure of oil extraction (Zhao et al., 2012). An estimated 35–45 g of skin and 230–300 g of hulls are generated per kilogram of shelled groundnut kernels (Zhao et al., 2012). Soybean curd residue is the main by-product of soybean products, and about 1.1 kg of fresh curd residue is produced from every kilogram of soybeans processed into soy milk or tofu (Khare et al., 1995). The manufacturing process of olive oil yields semi-solid wastes called olive cake (30 %) and aqueous liquor (50 %). About 10 g of olive cake is produced per kilogram of virgin olive oil processed (Masella et al., 2014).
Bioenergy by-products
The main processes in the production of bioenergy are pyrolysis, gasification and anaerobic digestion (Hamelin et al., 2019; Masoumi et al., 2021). The main bioenergy by-products with potential use in soil amendment are (1) biochar from thermochemical conversion with pyrolysis producing bio-oil and gasification producing syngas as the main product, (2) hydrochar from hydrothermal liquefaction with bio-oil as the main product, (3) digestate from anaerobic digestion with biogas as the main product and (4) molasses from lignocellulosic ethanol production with bio-ethanol as the main product (Hamelin et al., 2019; Karan and Hamelin, 2021; Masoumi et al., 2021). Conversion of agricultural residues and by-products into biochar provides an option for better waste management and reduction of the residue volume to be applied (Alkharabsheh et al., 2021). Biological methods such as digestion and composting do not reliably get rid of contaminants such as antibiotics, heavy metals and pathogens from agricultural and fishery residues. Processing these materials into biochar, however, can destroy pathogens and pollutants such as hormones and antibiotics given the high temperatures during pyrolysis. In addition, biochar has been reported to control plant diseases (de Medeiros et al., 2021; Poveda et al., 2021).
Due to the need to dry the feedstock for pyrolysis, which can be energy-intensive and costly for very wet feedstock, hydrothermal carbonization is considered an alternative to pyrolysis. Hydrothermal carbonization is carried out at relatively lower temperatures of 80–240 °C under sub-critical water pressure (Padhye et al., 2022). The solid output of this process is called hydrochar (Masoumi et al., 2021; Padhye et al., 2022).
Biogas production involves anaerobic digestion of organic wastes to produce methane (Akbar et al., 2021; Ma et al., 2022). This process produces large quantities of digestate that can be used as soil amendment. Since anaerobic digestion deactivates pathogens (Ma et al., 2022), it is also safer than direct application of bio-waste. Due to the increasing numbers of livestock feeding operations and the consequent increase in the number of large-scale biogas plants, huge quantities of digestate are produced in some regions. Digestate probably has more than 80 % moisture, whereas hydrochar can have a 20 %–50 % moisture content.
Slaughterhouse by-products
Slaughterhouse by-products consist of poultry and meat processing by-products. The inedible parts of slaughtered animals vary with the species, e.g. 22 % for turkeys, 37 % for broilers, 38 %–40 % for pigs, 47 % for sheep and goats and 49 % for cattle (Mozhiarasi and Natarajan, 2025).
Fish processing by-products
Fish processing by-products include the trimmings of fish in aquaculture or capture fisheries, e.g. heads, frames, skin and tails. These materials may constitute up to 70 % of fish and shellfish after processing. Depending on the market, some species are not processed at all, while others, especially larger fish, are often extensively transformed to fillets or parts of fillets. Fish fillet yield is species-dependent and is often in the range of 30 %–50 % of the fish on a wet-weight basis.
2.2.3 Livestock manure
Livestock manure is defined here as the excreta of domestic animals (e.g. poultry, cows, sheep, horses and rabbits), including the plant material used as bedding for animals. Two major categories of manure sources are recognized by the IPCC: manure management systems and manure left on pasture. Manure left on pasture is difficult to collect and therefore is largely unavailable for use as soil amendment. In management systems, manure may be found in liquid (liquid or slurry) or solid form on cattle, pig and poultry farms. In such systems, cattle produce large quantities of manure, with dairy cows producing 62 kg per day or about 10 % of the weight of an average cow on a wet-weight basis (EnviroStats, 2008). Feedlot cattle can generate manure of about 5 %–6 % of their body weight each day or a dry mass of roughly 5.5 kg per animal per day (Font-Palma, 2019). Full-grown milking cows can produce 7 %–8 % of their body weight as manure per day or roughly 7.3 kg dry mass per animal per day (Font-Palma, 2019). Bulls, beef cows, steers, heifers and calves produce 42, 37, 26, 24 and 12 kg of manure per animal per day (EnviroStats, 2008). Different categories of pigs produce 1–4 kg of manure per day, while poultry species produce less than 1 kg of manure per day.
2.2.4 Forestry residues
Forestry residues can be divided into primary and secondary residues (Karan and Hamelin, 2020). Primary residues are defined as residues that are left after logging operations (e.g. branches, stumps, treetops and bark), whereas secondary residues are by-products and co-products of industrial wood-processing operations (Karan and Hamelin, 2020). Primary residues were excluded from the OMD because they are often unavailable for agricultural use. Here, only wood residues were included. The FAOSTAT definition of wood residues covers wood that has passed through some form of processing but also constitutes the raw material of a further process such as for particle board, fibre board or energy purposes (FAO, 2022e). This excludes wood chips, made either directly in the forest from roundwood or in the wood processing industry (i.e. already counted as pulpwood or wood chips and particles), and agglomerated products such as logs, briquettes, pellets, similar forms and post-consumer wood.
2.3 Estimating the quantities produced
Crop residue production is typically estimated from grain yield using the harvest index (Smerald et al., 2023). The challenge with this approach is that the harvest index varies greatly in response to genetic, environmental and agronomic factors, and hence universally applicable harvest indices are lacking at the country level. As a result, practitioners often use RPRs to estimate residue biomass from data on production of primary products obtained from local statistics or global databases such as FAOSTAT and EUROSTAT (e.g. Bentsen et al., 2014; Bedoić et al., 2019; Karan and Hamelin, 2021; Ronzon and Piotrowski, 2017). The estimation is sometimes done assuming a mathematical relationship (e.g. linear, logarithmic, hyperbolic or exponential) between the primary crop yield and the residue yield (Bentsen et al., 2014; Ronzon and Piotrowski, 2017; Smerald et al., 2023). The disadvantage of the RPR is that it is constant over time and space for a given crop, whereas methods based on mathematical functions can be more flexible. In this work, the estimation of residues and by-products generally followed IPCC guidelines (Hergoualc'h et al., 2019) and the FAO guidelines in the Bioenergy and Food Security Rapid Appraisal user manual for crop and livestock residues (FAO, 2014).
In the case of crop residues, country-specific harvest indices or RPRs are not available for almost all countries. Therefore, the IPCC approach was used to estimate crop residues from harvested products. The IPCC provides two alternative methods for estimation of the above-ground crop residue yield (AGDM(T)) (kg ha−1) on a dry-mass basis. The first method involves multiplying the harvested crop yield by the ratio of above-ground dry matter (RAG(T)) provided in Table 11.A of the IPCC (2019). The second method involves estimation of residue yields from crop yields using the linear equations in Table 11.2 (Hergoualc'h et al., 2019). For any given crop (T), the following two methods were available based on the exact IPCC notations:
The first method always yields a constant harvest index, and most of the time it yields values larger than the typical values reported in the literature (e.g. Ludemann et al., 2023). For example, the IPCC default values of RAG(T)=1 and 1.2 for maize and barley yield harvest indices of 0.50 and 0.47, while the typical values are less than 0.47 and 0.41, respectively. As a result, the first method systematically underestimates residue production relative to the second method. The advantage of the second method is that it yields a more realistic harvest index commensurate with the grain yield achieved in a particular country and year. Therefore, the second method was chosen to estimate AGDM(T) from Crop(T) in FAOSTAT for the period 2015–2020. Then, the total annual above-ground residue production (AGR(T)) was calculated for each crop (T) by multiplying AGDM(T) by the harvested area available in FAOSTAT per country and year for maize, wheat, rice, barley, soybean and groundnut. The average values of 6 years (2015–2020) per country were summed across the countries to provide annual above-ground residue production estimates (AGR(T)) in tonnes on a dry-matter basis for each region. Then these values were added to produce a global estimate of the total residue production. The uncertainty around each estimate was expressed with 95 % confidence limits (CLs). It is not possible to generate estimates such as the standard errors or 95 % confidence limits of the sum of quantities using conventional statistical methods. Therefore, the 95 % CLs were estimated using bias-corrected bootstrapping, a non-parametric method which involves random resampling of the sample totals (sum) with replacement.
Table 2The IPCC equations used for estimation of the above-ground crop residue yield (AGDM(T)) in tonnes per hectare from the grain yield (Crop(T)) in tonnes per hectare from FAOSTAT, together with the IPCC default values for the dry-matter fraction of the harvested product and the dry-matter fraction of the above-ground crop residue.
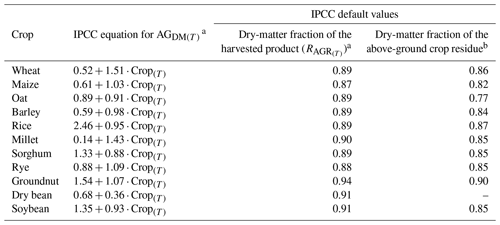
a These are all dry-matter values at grain moisture contents of 9 %–13 % or dry-matter fractions of 0.87–0.91. b Values are from Ludemann et al. (2023).
Production of agro-processing by-products is often estimated using country-specific coefficients following FAO guidelines (FAO, 2014). Whenever available, these values were defined as extraction rates and obtained from the FAO's Technical Conversion Factors for Agricultural Commodities (FAOSTAT, 2009). When not available, average values from the literature were used to estimate the various by-products from the production data in FAOSTAT. For example, the median value of 0.29 from Koopmans and Koppejan (1998) was used to estimate bagasse from sugarcane.
Poultry processing by-products were estimated using country-specific take-off rates, dressed carcass weights (percentage of live weight) and stocks (heads) as follows:
For each poultry species (chickens, ducks, geese and turkeys) in each country or territory, the take-off rate (%), average live weight (kilogram per animal) and dressed carcass wet weight (%) were obtained from the FAO's Technical Conversion Factors for Agricultural Commodities (FAOSTAT, 2009), while stocks (number of animals) were obtained from FAOSTAT Crops and livestock products (https://www.fao.org/faostat/en/#data/QCL, last access: 21 June 2023).
Similarly, meat processing by-products were estimated using country-specific take-off rates, dressed carcass weight (percentage of live weight) and stocks (heads) as follows:
The dressed carcass weight is the weight of the carcass after removal of its hide/skin, head, feet, offal, raw fats and blood, which are often not collected in the course of slaughter. For each species (buffaloes, cattle, sheep, goats, horses, camels and pigs) in each country or territory, the take-off rate (%), average live weight (kilogram per animal) and dressed carcass wet weight (%) were available in the FAO's Technical Conversion Factors for Agricultural Commodities. As with the poultry species, stocks were available in FAOSTAT Crops and livestock products for each country or territory. Carcass weight, together with edible and inedible offal, was used as defined in the FAO's Livestock statistics: Concepts, definitions and classifications (FAO, 2011). According to the definition in the FAO (2011), edible offal in most countries includes the head or head meat, throat bread, thick skirt, tongue, sweet bread, genital organs, brains, lungs, udder, feet, liver, stomach or tripes, tail meat, spleen, blood, heart and diaphragm. In this calculation, the inedible portions are assumed to be disposed of in slaughterhouses, and these were considered residues. However, under certain circumstances, parts such as the head, feet, fat and blood can be used in a variety of ways. Since data on specific uses of inedible offal are not available from slaughterhouses, we were unable to establish alternative uses.
Residues from capture fisheries and aquaculture species were estimated using country-specific conversion factors available in the Handbook of Fishery Statistical Standards (FAO, 2004) for selected species. In the fishery industry, the term “conversion factor” is used principally when converting the volume or mass (more commonly referred to as the “weight”) of a product at one stage into its volume or mass at another stage in the chain (FAO, 2004). Conversion factors for a particular state of processing vary according to species and state of processing. The state of processing is hierarchical and may consist of the following categories: (a) gutted, (b) headed and gutted, (c) dressed and (d) filleted (skin on or off). The FAO global inland and marine capture database includes catches for over 2000 species and items (including the “not elsewhere included” category). Since conversion factors are not available for all the species, the first species were ranked based on the number of countries producing and the total production in 2019. Then the top six species were selected for the present analysis because of the availability of conversion factors and the large number of countries involved in their production. Of the aquaculture species, rainbow trout (Oncorhynchus mykiss) was chosen as it was the most grown in aquaculture in 91 countries. In capture fisheries, yellow-fin tuna (Thunnus albacares), skipjack tuna (Katsuwonus pelamis), swordfish (Xiphias gladius), big-eye tuna (Thunnus obesus) and albacore (Thunnus alalunga) were chosen for the analysis. Each of these species was harvested in 96, 90, 83, 79 and 71 countries, respectively. The production quantity was then converted into residues as value − (value CF), where CF is the indicative factor for converting product weight into live weight. The FAO database of capture fishery production only covers retained catches; data on by-catches (discarded catches) are not included (Garibaldi, 2012). This means that the by-products can be severely underestimated.
Manure production (tonnes per year on a dry-matter basis) was estimated from the manure excretion rate (kilogram per head per day on a dry-weight basis) and stocks (from FAOSTAT) following the FAO guidelines for the different animal categories (FAO, 2014). The general formula for manure production is as follows:
Since there is no global database which provides country-specific data on manure production, the FAO tool uses the IPCC default values (FAO, 2014). For each species, average manure excretion rates were obtained from values compiled from the literature. For the USA, excretion rates were obtained from ASAE (American Society of Agriculture Engineers) Standards D384.1 (ASAE, 2005). Manure production was estimated separately for different management systems of cattle (non-dairy and dairy) and chicken (broilers and layers) because these are always managed as separate enterprises.
When compiling forestry residues, primary residues were excluded because of the concerns related to the environmental and economic sustainability of removing them from the forest for soil application on farmland. Therefore, the analysis focused on wood residues following the FAO definition. Country-specific data on the production quantity of wood residues (item code 1620) in FAOSTAT (https://www.fao.org/faostat/en/#data/FO, last access: 21 June 2023) were used to compile the OMD. These are reported in FAOSTAT in cubic metres solid volume, excluding bark.
A database of all the coefficients and RPRs used in the estimation of the various residues and by-products is now available in the OMD.
3.1 Crop residues
Maize had the largest global total annual above-ground residue production (∼1.28; CL: 0.43–2.33×109 t), followed by wheat (∼1.25; CL: 0.66–1.91×109 t) and rice (∼1.11; CL: 0.09–1.93×109 t) (Table 3). The estimated quantities of crop residues varied widely by continent and region. For example, the largest total annual production of maize residue was recorded in North America, including Canada and the USA ( t), followed by East Asia ( t), including China, the Democratic People's Republic of Korea, South Korea and Japan. China accounted for over 99 % of the residues produced in East Asia. The largest wheat residue production was recorded in South Asia ( t), including Afghanistan, Bhutan, India, Iran, Nepal, Pakistan and Sri Lanka, of which over 67 % was in India. Rice residue production was highest in South Asia ( t), of which over 70 % was produced in India. The global total annual residue production from soybean was t, while for groundnuts the corresponding value was t (Table 3). The largest soybean residue production was recorded in South America ( t), of which Brazil accounted for 61 % of soybean residue production in that region. This was followed by North America ( t), of which the USA accounted for 94 % of the soybean residue production there.
3.2 Agro-processing by-products
3.2.1 By-products from processing crops
Globally, maize processing yielded the highest number of by-products (0.12; CL: 0.04–0.23×109 t), followed by wheat (0.10; CL: 0.05–0.15×109 t), rice (0.09; CL: 0.03–0.16×109 t) and barley (0.04; CL: 0.03–0.06×109 t) (Table 4). The highest number of maize processing by-products was recorded in North America, followed by East Asia and South America. The highest number of wheat processing by-products was recorded in South Asia, followed by eastern Europe and East Asia (Table 4). The global sugarcane bagasse production is estimated at 548.7×106 t per annum (Table 5), including 44.8 % and 23.8 % produced in South America and South Asia, respectively. Brazil accounts for 89.1 % of the annual bagasse production in South America. Similarly, India accounts for 80.3 % of the annual bagasse production in South Asia.
The global annual production of by-products of coffee, cocoa and oil palm processing was estimated at 20.5, 5.3 and 170.1×106 t (Table 4). The largest quantity of coffee-processing by-products was recorded in South America, with Brazil producing about 6.5×106 t and accounting for over 71 % of the annual production in South America. This was followed by South-East Asia, where Viet Nam produced 3.3×106 t annually. The largest quantity of by-products from cocoa was produced in western Africa, where Côte d'Ivoire accounted for over 60 % of the production in that region. Of the 170.1×106 t of global annual oil palm by-products, Indonesia accounted for over 59 % of the total annual global production.
Table 4Estimated total annual agro-processing by-products of selected cereal and legume crops produced (1000 t on a dry-matter basis) across different regions. All values were estimated using FAOSTAT data (see the Methods section).
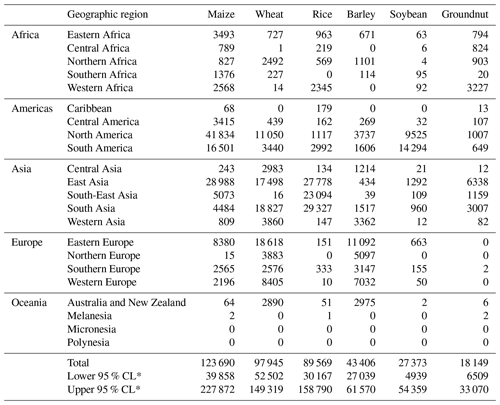
* Values represent the lower and upper 95 % confidence limits estimated using bootstrapping.
Table 5Estimated total annual production of agro-processing by-products of coffee, cocoa, oil palm and sugarcane produced (1000 t on a dry-matter basis) across different regions. All values were estimated using FAOSTAT data (see the Methods section).
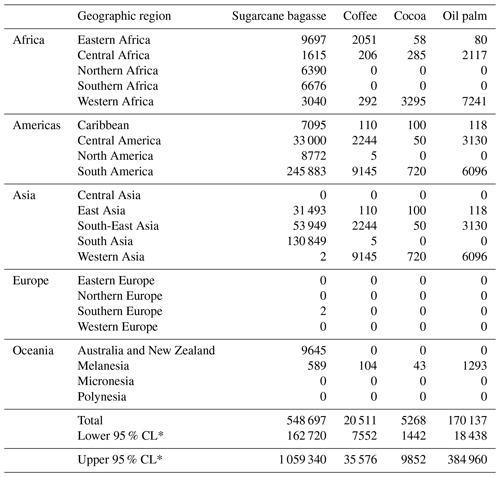
* Values represent the lower and upper 95 % confidence limits estimated using bootstrapping.
3.2.2 By-products from slaughterhouses
Globally, the largest quantity of residues produced annually was from cattle (16.5×106 t), followed by chicken (10.7×106 t) and pigs (6.2×106 t), but with wide variation among the regions (Table 6). The largest quantity of by-products from cattle was recorded in South America (5.31×106 t), of which Brazil accounted for 77 % of the by-products produced in that region. This was followed by North America (4.59×106 t, of which 94 % was in the USA) and East Asia (0.99×106 t, of which 84 % was in China). The total annual production of by-products from poultry processing was highest in North America (6.0×106 t), of which over 99 % was produced in the USA. This was followed by East Asia (0.91×106 t), of which China accounted for over 72 % of the production there.
Table 6Estimated total annual number of slaughterhouse by-products potentially produced (1000 t on a dry-matter basis) across different regions. All values were estimated using FAOSTAT data (see the Methods section).
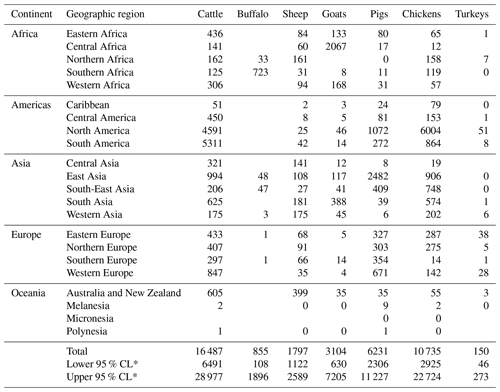
* Values represent the lower and upper 95 % confidence limits estimated using bootstrapping.
3.2.3 By-products from fisheries and aquaculture
The estimated annual number of by-products potentially produced from processing of selected fish species in aquaculture and capture fisheries is summarized in Table 6. Of the species grown in aquaculture, the largest number of by-products was produced by rainbow trout (over 0.08×106 t) across 91 countries (Table 7). The largest proportion was recorded in South Asia (predominantly in Iran and Türkiye), followed by South America (mainly in Peru and Chile) and northern Europe (mostly in Norway) (Table 7). Of the capture fishery species, the largest number of by-products was produced from skipjack tuna harvest (0.14×106 t), followed by yellow-fin tuna (0.08×106 t).
3.3 Livestock manure
Globally, cattle, buffalo and chicken produced the highest proportion of the potential annual manure produced every year (Table 8). On a dry-matter basis, non-dairy cattle produce an estimated 2.23×109 t (CL: 1.32–3.23), while dairy cattle produce about 0.82×109 t (CL: 0.50–1.21) annually. The largest quantity of non-dairy cattle manure was produced in South America (where Brazil accounts for 60 %), followed by South Asia (where India accounts for 68 %). Annual production of dairy cattle manure was highest in South Asia (where India accounts for 68 %). The highest annual manure production by buffalo occurs in East Asia (China accounts for 99 %) and South Asia (India accounts for 70 %). The largest quantity of broiler chicken manure was recorded in South-East Asia, where Indonesia accounts for 76 % in that region. The next highest production was recorded in South Asia, where Pakistan and Iran account for 42 % and 37 % in that region (Table 8).
3.4 Wood residues
Globally, an estimated 0.23×109 t (CL: 0.08–0.43) of wood residues are produced every year (Table 9), but the highest production occurs in East Asia (China producing the most), followed by South America and North America, where Brazil and the USA have the highest production. Annual wood residue production was highest in China (95.1×106 t), followed by Brazil (18.8×106 t). The values presented in Table 8 are based on countries for which data were available in FAOSTAT. Since data are not available for all countries in many regions, it was not possible to calculate the residue production per country as a proportion of the total production in the respective region. Countries in the Caribbean, Central Asia, central Africa, western Africa, northern Africa and South Asia are represented poorly (Table 9).
The preceding sections presented indicative estimates of the quantities of agricultural residues and by-products for selected crops and animals that are available in the OMD. Due to the lack of a uniform methodology and data across countries, it was not possible to accurately estimate the quantities of residues produced by all crops and agro-processing activities. We are also keenly aware that the values presented could either overestimate or underestimate the global residue production. However, the OMD is a living database that will be updated and enriched as new data and methods become available in order to build a solid reference resource for industry, researchers and decision-makers in soil health management, pollution risk reduction, bioenergy production and other sectors. The OMD is envisaged as complementing existing databases such as FAOSTAT and FishStat and organic resource quality databases such as Phyllis. The OMD may be used for various purposes, including estimation of the availability of soil amendments, animal feed, bioenergy and other agricultural activities such as mushroom production. The use of agricultural and forestry residues and by-products for soil amendment may be constrained by these competing uses (Duncan et al., 2016; Ji et al., 2018). The following sections will discuss the production and competing uses of agricultural, fishery and forestry residues as well as the opportunities and challenges for their use as soil amendments.
4.1 Crop residues
The estimates provided for the selected crops (Table 3) reveal that large quantities of crop residue biomass are produced annually. However, there are large differences in the share of residues of the different crops between countries and regions. For example, the highest total annual production of maize residue was recorded in North America, whereas the highest wheat and rice residue production was recorded in South Asia. Our estimates are based on uniform application of a single equation for each crop across the countries due to a lack of country-specific conversion coefficients. We are keenly aware that this can affect the accuracy of estimates in the database. The use of country-specific harvest indices or equations could have provided more accurate data.
The competing uses of residues may vary between regions, countries and even farming systems within countries. We were unable to disaggregate the total residue into different categories due to the lack of country-specific data on the proportion fed to animals, burnt or left on the ground. According to estimates by Smerald et al. (2023), about 44 % of cereal residues are left on the field, 33 % are used for animal feed and bedding, 16 % are used for other purposes and 6 % are burnt globally. In China, which is one of the largest producers of crop residues, 82.3 % are currently collected and used as fertilizer (62.3 %), feed (16.0 %), energy (9.6 %), cultural substrate (0.8 %) or raw material (1.1 %) (Zhao et al., 2024). In many parts of the world, crop residues are widely used as soil amendments or as mulch to protect the soil from erosion. Farmers also remove residues to feed animals or use them as beddings. For example, about 16 % of the collectible crop residues are used as animal bedding in Europe (Monforti et al., 2013). In the EU member states alone, around 28×106 t of crop residues are used for animal bedding annually (Scarlat et al., 2010). Crop residues are also used as fuel in industrial and domestic set-ups. For example, in rural areas in Africa and Asia, crop residues are used for cooking. There is also growing interest in the use of crop residues for the generation of biofuels as alternatives to fossil fuels and industrial applications that include textiles, natural fibres, polymers, bio-sorbents and reinforcement material in composites (Siqueira et al., 2022). However, country-specific data are virtually lacking for producing a database of the competing uses.
The estimated total annual crop residues produced by the top cereal and legume crops across the different regions indicate great potential for their use in soil amendments and contributions to bio-economy processes. Depending on the availability of technology for recovery, some of the crop residues produced may be used for recycling in bioenergy production and as soil amendments. Raw crop residues such as straw can be incorporated into the soil or applied to the soil surface as mulch, and this can reduce erosion, maintain soil moisture and add carbon and nutrients to the soil. A growing body of meta-analyses has provided compelling evidence that residue retention significantly increases crop yields, soil nutrient stocks, water use efficiency, carbon sequestration, microbial diversity and functionality (Shu et al., 2022; Wang et al., 2020).
While crop residues can contribute to enhancing soil organic carbon stocks and nutrient availability for crops and can contribute to reducing soil erosion, not all crop residues produced are readily available as a soil amendment. Some crop residue is burnt in the field or used as fuel for domestic purposes, animal feed and/or bedding, mushroom production, construction and industrial applications (FAO, 2022a; Ji et al., 2018). In some cropping systems and regions, residues are burnt in the field during land preparation because this is the easiest option for farmers. For example, the intensification of rice cropping with high-yielding and short-duration varieties in Asia has resulted in larger volumes of rice straw, which must be managed over a very short time of between two or three cropping rounds per year (Van Hung et al., 2020). In such systems, soil application of residues poses challenges due to the insufficient time for decomposition of straw, which hinders crop establishment. This has led to an increase in open-field burning of rice straw in some Asian countries (Lin and Begho, 2022; Van Hung et al., 2020).
Of the residues produced annually, only a small fraction may be recovered because the collection, storage and transportation of raw residues pose challenges to their use outside their production area. One way to reduce their transportation costs and increase their use is to convert bulky residues and by-products into briquettes, pellets, biochar or anaerobic digestate that can be handled and transported more easily than raw residues (Bora et al., 2020). In some regions, the short time frame between two cropping seasons may not allow collection of available residues (FAO, 2014). Even when collection is feasible, the cost of transportation may limit soil application to far from the farm where the residues were produced. This may be overcome by mechanized collection, high-density compaction, briquetting, pelletizing or on-site processing (e.g. composting or anaerobic digestion). High-density compaction can reduce the volume of crop residues, thus making them easier to store and transport over a long distance. For example, the volumetric weight of mechanically compacted rice straw bales is 50 %–100 % higher than that of loose straw. Briquetting and pelletizing can increase the volumetric weight of baled straw further by up to 700 % and reduce transportation costs by more than 60 % (Balingbing et al., 2020).
The quality of residues may play a critical role in the build-up of carbon and nutrients in the soil (Cotrufo et al., 2013) against the backdrop of the importance of the soil ecosystem (Schmidt et al., 2011). The carbon content of residues is about 30 %–50 % (Table 1). The nitrogen content of various cereal straws ranges between 0.3 % and 2.8 %, and only pulse straws are relatively nitrogen-rich (Table 1). With low C : N ratios (Table 1), residues from legumes are likely to decompose more rapidly than cereals. The phosphorus and potassium contents of most residues are 0.05 %–0.3 % and 0.2 %–2 %, respectively (Table 1). As such, crop residues represent a substantial store of carbon and nutrients that can be used as inputs for soil amendment. A role of crop residue incorporation that has remained less appreciated is their contribution to soil micronutrient stocks, especially sulfur, calcium, magnesium, zinc and silicon, which are often not part of the recommended fertilizers. Where straw is incorporated, reserves of soil nitrogen, phosphorus, potassium and silicon have also been known to be maintained at acceptable levels (Dobermann and Fairhurst, 2002).
4.2 Agro-processing by-products
Our estimates indicate that substantial quantities of by-products are produced every year but with a great deal of variability across the regions. Unlike crop residues, most of the by-products are produced in localized processing plants, which makes their collection more convenient. However, some of the by-products may not be available for soil amendment as they have various other uses. For example, husks of rice are mostly used as fuel in rice mills (Petersen et al., 2015). Rice husk is also used as an insulating material. In crops such as oil palm, cocoa and coffee, the processing also occurs in a few countries where the commodities are grown on a commercial scale.
Although oil palm is widely cultivated in plantations across the humid tropics of Asia, Africa and the Americas, over 90 % of the global palm oil production occurs in just five countries, i.e. Indonesia (58.8 %), Malaysia (25.6 %), Thailand (3.9 %), Colombia (2.9 %) and Nigeria (1.4 %) (Murphy et al., 2021). Although the oil palm industry is one of the best sources of organic inputs for agricultural use (Adu et al., 2022; Embrandiri et al., 2012), the residues may not be available for direct soil application in areas far from processing plants. However, this can be circumvented through conversion into compost or digestates, which are easier to handle and transport.
Our global estimate of sugarcane bagasse production (548.7×106 t) is very close to the 540×106 t reported in Bezerra and Ragauskas (2016). Unlike other crop residues, bagasse is not readily available for soil amendment. This is because much of the bagasse produced is used for steam generation in sugar mills, and the remainder is burnt as dry bagasse is known to be a fire hazard. Bagasse roughly consists of 20 %–30 % lignin, 40 %–45 % cellulose and 30 %–35 % hemicellulose, making it a promising feedstock for second-generation biofuel production (Bezerra and Ragauskas, 2016; Petersen et al., 2015). In some countries bagasse is also used as a raw material for the paper and board industry.
While slaughterhouse operations produce large quantities of by-products, some are processed by the rendering industry for conversion into animal feed, pet food, poultry meal and animal fats (Mozhiarasi and Natarajan, 2025). There are challenges with the availability of by-products from slaughterhouse and fish processing facilities for soil application. Different parts of animals, such as the head, feet, fat and blood, can be used in a variety of ways and therefore may not be readily available for soil amendment. Some fish parts, especially the viscera, deteriorate very rapidly, and therefore they require preservation as soon as possible after being processed. This is not always possible due to inadequate processing facilities or limited volumes that make recovery of by-products unprofitable. When fish are processed into fillets at sea, the viscera, head and frames are often discarded since refrigeration facilities are used for the most valuable products (Olsen et al., 2014).
4.3 Livestock manure
Our estimates in Table 7 show that large quantities of manure are produced annually albeit with high variability across regions. These estimates include both manure management systems and manure left on pasture. Only one-fifth of the livestock manure produced is applied to cropland due to various constraints. For example, much of the manure produced may not be available for application as soil amendment on cropland because over 70 % is directly deposited onto pasture (FAO, 2018). Manure applied to soil can be a significant source of macronutrients and micronutrients (FAO, 2018; Sileshi et al., 2019). In addition, manure is a significant source of organic matter, which is a key determinant of soil health (FAO, 2018). For example, globally, manure applied to soil was estimated to contribute 24 and 31×106 t of nitrogen per annum based on the IPCC Tier 1 and Tier 2 approaches, respectively (FAO, 2018). According to van Dijk et al. (2016), manure application to soil constitutes approximately 53 % of the P and 33 % of the N applied annually to agricultural land in the EU27.
Even if manure is available in abundance, its application may be constrained by environmental quality and economic considerations in some jurisdictions. For example, in the USA, Environmental Protection Agency regulation requires large animal feeding operations to meet nutrient planning requirements for land application of manure. Similarly, according to EU Council Directive 91/676/EEC, the amount of livestock manure applied to land each year shall not exceed 170 kg N per hectare. Legislation may also forbid manure application during certain periods (e.g. in non-cropping seasons) or on land that would otherwise lead to environmental impacts through runoff or nutrient leaching (Loyon, 2018).
The bulky nature of manure limits the areas over which it can be applied economically. According to Paudel et al. (2009), the economically optimal distances for dairy manure application are 30 km for nitrogen and 15 km for phosphorus and potassium in order to meet the recommended N, P2O5 and K2O needs on cropland. Conversion of manure into anaerobic digestate or compost can circumvent the handling, storage and transportation costs of raw manure from intensive animal production units. When efficiently managed and recycled within agricultural systems, livestock manure represents a large source of plant nutrients that can reduce the need for synthetic fertilizer inputs and reduce GHG emissions (FAO, 2018). Manure may be applied by injection, band application, surface spreading or incorporation (Emmerling et al., 2020). Injection has been cited as the best application method for reducing NH3 emissions, while surface application using splash plates has been banned in most European countries because of its strong impact on NH3 emissions (Emmerling et al., 2020).
4.4 Wood residues
Wood residues are obviously underestimated for many regions because data were unavailable for some countries. Of the countries for which data exist, annual wood residue production was highest in China and Brazil, representing 42 % and 8.3 % of the annual global wood residue production. Wood log production in Brazil generates about 50.8×106 m3 of lignocellulosic residue yearly (Domingues et al., 2017). Assuming a wood density of ∼ 450 kg m3, this value is approximately 22.9×106 t, which is slightly higher than the 18.8×106 t in our database. The competing uses of wood residues include use as wood fuel for domestic purposes (Flammini et al., 2023), bioenergy generation (Karan and Hamelin, 2020) and raw materials for the manufacture of agglomerated products such as pulp, particle board and fibre board (FAO, 2022e). Although wood residues could potentially be used for soil amendment after processing (e.g. wood ash, biochar and compost), the proportion actually available may be small due to their various competing uses. Agroforestry trees and plantation crops such as coconut, oil palm and rubber generate considerable amounts of woody and leafy biomass from pruning and lopping. A large proportion of such residues can be used for soil amendment directly or after processing into compost or biochar (Bluhm and Lehmann, 2023). However, data were not readily available for these residues, and therefore it was not possible to collate their quantities in the OMD.
One of the key limitations of this work is the lack of country-specific data on the quantities of some categories of residues, such as crop residues and manure. Country-specific conversion factors or equations are also lacking for converting production statistics into residue data. As a result, we were forced to use a single conversion factor or a single equation in some cases. A second limitation of this work is our inability to provide global estimates of all residues from agriculture, fisheries and forestry. The effort to compile estimates of all residues and by-products was hampered by the lack of methods for conversion of primary products into residues and industry standards for collection and aggregation of such data. For example, we did not include the quantities of residues produced by minor crops, fruit trees and other trees in agroforestry and forestry. A third limitation of this work is that we were unable to account for situations where products are processed in a country different from their origin, as this would entail additional data on export and import. This could be considered an important future endeavour in the development of new versions of the OMD.
Also, the OMD does not contain the quantities of by-products such as biochar, compost and digestate produced in each country due to a lack of data on their production. By-products of secondary processing that occurs in breweries and the beverage industry could not be obtained either due to a lack of mechanisms for capturing them at the country level. By-products from capture fisheries were estimated only for a few species because conversion factors were unavailable for the majority of the species. Even for those species where conversion factors were available, residues from capture fisheries were probably underestimated by a large margin because recovery of inedible parts is challenging. This is because fish are processed at sea, and inedible parts may be discarded in the sea (Olsen et al., 2014). Commercial fish products are often directly processed on board vessels and, by the time these have landed, the fish have been frozen, gutted, headed and/or processed, leading to a considerable change from their original weight. This leaves a great deal of uncertainty about estimation of fishery by-products. We were also unable to provide uncertainties associated with estimates of the quantities presented at national or sub-national levels due to a lack of data. Therefore, we strongly recommend investment in the inventory of agricultural, fishery and forestry residues as well as by-products and wastes at the national and sub-national levels for use in a circular bio-economy.
This work only provides an inventory of the various residues at the country level, which is valuable in its own right. However, further work needs to be done to produce a global map of carbon and nutrients from residues at a much greater spatial distribution and finer resolution than individual countries in order to inform policy and good practice for more efficient allocation of biomass resources. There is also an urgent need to document alternative and competing uses of the various categories of residue estimation of the different uses of each residue and unused or wasted residues at the national and local levels. This requires further work deemed outside the scope of this publication.
Due to a lack of basic data, this work was unable to determine the proportion of the residues in each category that are actually available for use as soil amendment. Even where data were available, legislative and regulatory issues may have limited their use as soil amendments. For example, environmental concerns about pollution by antibiotics, heavy metals and pathogens have led to regulations regarding the direct spread of manure on land (Font-Palma, 2019). Strict regulations such as those under EU Nitrates Directive 91/676/EEC (EEC, 1991) mean that only a small proportion of the total volume of manure produced can be used for soil amendment. It is also forbidden to apply manure or anaerobic digestate at particular times of the year or on certain types of land (Loyon, 2018). In some jurisdictions, organic matter that has been designated as waste may be subject to regulatory restrictions on how it can be used or managed subsequently (Loyon, 2018). In this analysis, it was not possible to evaluate the extent to which national policies and regulatory frameworks governing the classification of organic matter streams as wastes or by-products and governing waste management can provide incentives or not for the use of organic inputs for soil amendment. Legislation banning residue burning and incentives for farmers to adopt good agricultural practices can also incentivise appropriate use of agricultural residues. For example, EU Regulation No. 1307/2013 has established rules for direct payments to farmers under support schemes within the framework of the common agricultural policy. To receive full payments, farmers in the member states have to comply with statutory management requirements and standards for good agricultural and environmental conditions as well as the requirement of “greening” (Heyl et al., 2021). Quantitative targets are used to incentivise the implementation of agricultural practices that increase soil organic carbon stocks (Bruni et al., 2022). For example, the EU Mission Board for Soil Health and Food proposed a series of quantitative targets for soils to become healthier. Of these, the current SOC losses of about 0.5 % per year at the 20 cm soil depth of croplands should be reversed to an increase of 0.1 %–0.4 % per year by 2030 (Bruni et al., 2022). Such targets and related regulations will have implications for how and where agricultural residues can be used for soil amendment.
Transport costs may also hinder the use in other regions of the excess volume produced in one region. In some regions, anaerobic digestate is produced in excess of its agricultural assimilation potential (Torrijos, 2016). For example, in the EU, digestate production reached 56×106 t per annum by 2010, of which 80 % could be recycled back into agriculture (Kizito et al., 2019). Similarly, in China, the annual digestate production is approximately 2.3×106 t, of which less than 70 % is recycled back into agriculture due to land limitations (Kizito et al., 2019). These observations highlight the need to explore opportunities for the use of residues and by-products outside the country where they are produced.
The OMD data are available at https://doi.org/10.5281/zenodo.10450921 (Sileshi et al., 2024).
This work has provided typologies, definitions and quantities of various agricultural residues and by-products, which can be useful for the inventory and estimation of various residue streams that are potentially available for recycling in the agricultural, bioenergy and other sectors. The OMD is the first of its kind to consolidate biomass estimates of residues and by-products from the agricultural, fishery, forestry and related industries globally. The OMD will be updated continuously as new production data are published in FAOSTAT and will be publicly available for use by different decision-makers. It will hopefully contribute to the Better Production and Better Environment dimensions of the FAO's Strategic Framework 2022–2031 in support of the 2030 agenda. The OMD is also expected to contribute to evidence-based policies and actions in support of the transition towards a circular economy and more sustainable agriculture and food systems. Currently, estimates of crop residues and manure in the OMD are only available at the national level based on a single equation applied uniformly due to the lack of country-specific conversion factors. Therefore, finer-scale data and country-specific conversion factors and/or equations are urgently needed for spatial targeting of residues and by-products for various applications. A detailed site-specific inventory of various categories of residues and their local uses is highly recommended. An inventory of the competing uses and fates of the various residues and wastes is also urgently needed for each country.
EB, GWS and FNT conceptualized and designed the study. GWS and JL developed the methodology, and GWS conducted the data curation and formal analysis. GWS and EB wrote and edited the manuscript, while JL and FNT reviewed and edited the manuscript. EB acquired the funding. All the authors read and approved the final version of the manuscript.
At least one of the (co-)authors is a member of the editorial board of Earth System Science Data. The peer-review process was guided by an independent editor, and the authors also have no other competing interests to declare.
The views expressed in this paper are the authors' only and do not represent FAO's positions and policies on the subject matter.
Publisher's note: Copernicus Publications remains neutral with regard to jurisdictional claims made in the text, published maps, institutional affiliations, or any other geographical representation in this paper. While Copernicus Publications makes every effort to include appropriate place names, the final responsibility lies with the authors.
FAOSTAT is funded by the FAO's regular budget, with data received by national experts serving as focal points. We acknowledge the efforts of national experts who provided the statistics on food, agriculture and energy use, which form the basis of this effort. This work was supported financially by the McKnight Foundation (grant no. 15-113) through the Strengthening Multistakeholder Cooperation on Agroecological Approaches for Sustainable Agriculture programme.
This research has been supported by the McKnight Foundation (grant no. 15-113).
This paper was edited by Chaoqun Lu and reviewed by two anonymous referees.
Adu, M. O., Atia, K., Arthur, E., Asare, P. A., Obour, P. B., Danso, E. O., Frimpong, K. A., Sanleri, K. A., Asare-Larbi, S., Adjei, R., Mensah, G., and Andersen, M. N.: The use of oil palm empty fruit bunches as a soil amendment to improve growth and yield of crops, A meta-analysis, Agron. Sust. Dev., 42, 13, https://doi.org/10.1007/s13593-022-00753-z, 2022.
Akbar, S., Ahmed, S., Khan, S., and Badshah, M.: Anaerobic digestate: a sustainable source of bio-fertilizer, in: Sustainable Intensification for Agroecosystem Services and Management, edited by: Jhariya M. K., Banerjee, A., Meena, R. S., Kumar, S., and, Raj, A., Springer, Singapore, https://doi.org/10.1007/978-981-16-3207-5_15, 2021.
Al-Gheethi, A., Ma, N. L., Rupani, P. F., Sultana, Z., Azrina, M., Yaakob, M., Mohamed, R. M. S. R., and Soon, C. F.: Biowastes of slaughterhouses and wet markets: an overview of waste management for disease prevention, Environ. Sci. Pollut. Res., 30, 71780–71793, https://doi.org/10.1007/s11356-021-16629-w, 2021.
Alkharabsheh, H. M., Seleiman, M. F., Battaglia, M. L., Shami, A., Jalal, R. S., Alhammad, B. A., Almutairi, K. F., and Al-Saif, A. M.: Biochar and its broad impacts in soil quality and fertility, nutrient leaching and crop productivity: A Review, Agronomy, 11, 993, https://doi.org/10.3390/agronomy11050993, 2021.
American Society of Agriculture Engineers (ASAE): Manure production and characteristics ASAE Standards D384.1, https://elibrary.asabe.org/abstract.asp?aid=32018&t=2&redir=&redirType= (last access: 31 January 2025), 2005.
Andrews, E. M., Kassama, S., Smith, E. E., Brown, P. H., and Khalsa, S. D. S.: A review of potassium-rich crop residues used as organic matter amendments in tree crop agroecosystems, Agriculture, 11, 580, https://doi.org/10.3390/agriculture11070580, 2021.
Antonić, B., Jančiková, S., Dordević, D., and Tremlová, B.: Grape pomace valorization: A systematic review and meta-analysis, Foods, 9, 1627, https://doi.org/10.3390/foods9111627, 2020.
Bai, S. H., Omidvar, N., Gallart, M., Kämper, W., Tahmasbian, I., Farrar, M. B., Singh, S., Zhou, G., Muqadass, B., Xu, C-Y., Koech, R., Li, Y., Nguyen, T. T. N., and van Zwieten, L.: Combined effects of biochar and fertilizer applications on yield: A review and meta-analysis, Sci. Total Environ., 808, 152073, https://doi.org/10.1016/j.scitotenv.2021.152073, 2022.
Balingbing, C., Van Hung, N., Nghi, N. T., Van Hieu, N., Roxas, A. P., Tado, C. J., Bautista, E., and Gummert, M.: Mechanized collection and densification of rice straw, in: Sustainable Rice Straw Management, edited by: Gummert, M., Hung, N., Chivenge, P., and Douthwaite, B., Springer, 15–32, https://doi.org/10.1007/978-3-030-32373-8_2, 2020.
Bedoić, R., Ćosić, B., and Duić, N.: Technical potential and geographic distribution of agricultural residues, co-products and by-products in the European Union, Sci. Total Environ., 686, 568–579, https://doi.org/10.1016/j.scitotenv.2019.05.219, 2019.
Behnassi, M. and El Haiba, M.: Implications of the Russia–Ukraine war for global food security, Nat. Hum. Behav., 6, 754–755, https://doi.org/10.1038/s41562-022-01391-x, 2022.
Bentsen, N. S., Felby, C., and Thorsen, B. J.: Agricultural residue production and potentials for energy and materials services, Prog. Energy Combust. Sci., 40, 59–73, https://doi.org/10.1016/j.pecs.2013.09.003, 2014.
Bezerra, T. L. and Ragauskas, A. J.: A review of sugarcane bagasse for second-generation bioethanol and biopower production, Biofuels, Bioprod. Bioref., 10, 634–647, https://doi.org/10.1002/bbb.1662, 2016.
Bluhm, D. and Lehmann, J.: Biochar-based recycling of biomass and nutrients at multiple scales, in: Biological Approaches to Regenerative Soil Systems, edited by: Uphoff, N. and Thies, J., Taylor and Francis, London, 324–331, https://doi.org/10.1201/9781003093718-31, 2023.
Bora, R., Tao, Y., Lehmann, J., Tester, J., Richardson, R., and You, F.: Techno-economic feasibility and spatial analysis of thermochemical conversion pathways for regional poultry waste valorization, ACS Sust. Chem. Eng., 8, 5763–5775, https://doi.org/10.1021/acssuschemeng.0c01229, 2020.
Bruni, E., Guenet, B., Clivot, H., Kätterer, T., Martin, M., Virto, I., and Chenu, C.: Defining quantitative targets for topsoil organic carbon stock increase in European croplands: case studies with exogenous organic matter inputs, Front. Environ. Sci., 10, 824724, https://doi.org/10.3389/fenvs.2022.824724, 2022.
Caldeira, C., Vlysidis, A., Fiore, G., De Laurentiis, V., Vignali, G., and Sala, S. Sustainability of food waste biorefinery: A review on valorisation pathways, techno-economic constraints, and environmental assessment, Bioresour. Technol., 312, 123575, https://doi.org/10.1016/j.biortech.2020.123575, 2020.
Chivenge, P., Vanlauwe, B., and Six, J.: Does the combined application of organic and mineral nutrient sources influence maize productivity? A meta-analysis, Plant Soil, 342, 1–30, https://doi.org/10.1007/s11104-010-0626-5, 2011.
Cobo, J. G., Barrios, E., Kass, D., and Thomas, R. J.: Decomposition and nutrient release by green manures in a tropical hillside agroecosystem, Plant Soil, 240, 331–342, https://doi.org/10.1023/A:1015720324392, 2002.
Cotrufo, M. F., Wallenstein, M. D., Boot, C. M., Denef, K., and Paul, E.: The Microbial Efficiency-Matrix Stabilization (MEMS) framework integrates plant litter decomposition with soil organic matter stabilization: Do labile plant inputs form stable soil organic matter?, Glob. Change Biol., 19, 988–995, https://doi.org/10.1111/gcb.12113, 2013.
de Medeiros, E. V., Lima, N. T., de Sousa Lima, J. R., Pinto, K. M. S., da Costa, D. P., Junior, C. L. F., Souza, R. M. S., and Hammecker, C.: Biochar as a strategy to manage plant diseases caused by pathogens inhabiting the soil: a critical review, Phytoparasitica, 49, 713–726, https://doi.org/10.1007/s12600-021-00887-y, 2021.
Dobermann, A. and Fairhurst, T. H.: Rice Straw Management, Better Crops Int. 16, Special Supplement, https://www.researchgate.net/publication/228850474_Rice_straw_management (last access: 18 May 2023), 2002.
Domingues, R. R., Trugilho, P. F., Silva, C. A., Melo, L. C. A., Magriotis, Z. M., and Sánchez-Monedero, M. A.: Properties of biochar derived from wood and high-nutrient biomasses with the aim of agronomic and environmental benefits, PLoS ONE, 12, e0176884, https://doi.org/10.1371/journal.pone.0176884, 2017.
Duncan, A. J., Bachewe, F., Mekonnen, F., Valbuena, D., Rachier, G., Lule, D., Bahta, M., and Erenstein, O.: Crop residue allocation to livestock feed, soil improvement and other uses along a productivity gradient in Eastern Africa, Agric. Ecosys. Environ., 228, 101–110, https://doi.org/10.1016/j.agee.2016.05.011, 2016.
Dutra, J. C. F., Passos, M. F., García, G. J. Y., Gomes, R. F., Magalhães, T. A., Freitas, A. S., Laguna, J. G., da Costa, F. M. R., da Silva, T. F., Rodrigues, L. S., Américo, M. F., Campos, G. M., Pereira, G., Vandenbeghe, L., Soccol, C. R., Góes-Neto, A., and Azevedo, V. A. C.: Anaerobic digestion using cocoa residues as substrate: Systematic review and meta-analysis, Energy Sust. Dev., 72, 265–277, https://doi.org/10.1016/j.esd.2022.12.007, 2023.
ECN: Phyllis2 – Database for biomass and waste, https://www.ecn.nl/phyllis2/ (last access: 17 May 2023), 2018.
EEC: European Union Council. Directive 91/676/EEC of 12 December 1991 concerning the protection of waters against pollution caused by nitrates from agricultural sources, Off. J. Eur. Union, L 375, 1–8, 1991.
Embrandiri, A., Singh, R. P., Ibrahim, H. M., and Ramli, A. A.: Land application of biomass residue generated from palm oil processing: its potential benefits and threats, Environmentalist, 32, 111–117, https://doi.org/10.1007/s10669-011-9367-0, 2012.
Emmerling, C., Krein, A., and Junk, J.: Meta-analysis of strategies to reduce NH3 emissions from slurries in European agriculture and consequences for greenhouse gas emissions, Agronomy, 10, 1633, https://doi.org/10.3390/agronomy10111633, 2020.
EnviroStats: A geographical profile of livestock manure production in Canada, EnviroStats, vol. 2, https://www150.statcan.gc.ca/n1/en/pub/16-002-x/16-002-x2008004-eng.pdf?st=8hSAiqPk (last access: 20 June 2023), 2008.
European Parliament and Council: DIRECTIVE 2008/98/EC of the European Parliament and of the Council of 19 November 2008 on waste and repealing certain Directives, https://eur-lex.europa.eu/eli/dir/2008/98/oj/eng (last access: 29 January 2025), 2008.
Fan, X., Chen, Z., Niu, Z., Zeng, R., Ou, J., Liu, X., and Wang, X.: Replacing synthetic nitrogen fertilizer with different types of organic materials improves grain yield in China: A meta-analysis, Agronomy, 11, 2429, https://doi.org/10.3390/agronomy11122429, 2021.
FAO: CWP Handbook of Fishery Statistical Standards. Coordinating Working Party on Fishery Statistics, FAO, Rome, https://www.fao.org/3/j4000e/j4000e.pdf (last access: 9 May 2023), 2004.
FAO: Livestock statistics: Concepts, definitions and classifications, https://www.fao.org/economic/the-statistics-division-ess/methodology/methodology-systems/livestock-statistics-concepts-definitions-and-classifications/en/ (last access: 9 May 2023), 2011.
FAO: Bioenergy and Food Security Rapid Appraisal (BEFS RA) User Manual: Crop Residues and Livestock Residues, FAO, Rome, 2014.
FAO: Nitrogen inputs to agricultural soils from livestock manure: New statistics, Integrated Crop Management, 24, 1–41, FAO, Rome, 2018.
FAO: Establishing residue supply chains to reduce open burning. The case of rice straw and renewable energy in Punjab, India, Environment and Natural Resources Management Working Paper No. 95, Rome, https://doi.org/10.4060/cb9570en, 2022a.
FAO: Inorganic fertilizers 1990–2020, https://www.fao.org/food-agriculture-statistics/data-release/data-release-detail/en/c/1599852/ (last access: 9 May 2023), 2022b.
FAO: The importance of Ukraine and the Russian federation for global agricultural markets and the risks associated with the war in Ukraine, https://www.fao.org/3/cb9013en/cb9013en.pdf (last access: 9 May 2023), 2022c.
FAO: Greenhouse gas emissions from agrifood systems. Global, regional and country trends, 2000–2020, https://www.fao.org/food-agriculture-statistics/data-release/data-release-detail/en/c/1616127/ (last access: 9 May 2023), 2022d.
FAO: Classification of forest products 2022, Rome, https://doi.org/10.4060/cb8216en, 2022e.
FAOSTAT: Technical conversion factors for agricultural commodities, Technical report, Food and Agriculture Organization of the United Nations, https://www.fao.org/fileadmin/templates/ess/documents/methodology/tcf.pdf (last access: 9 May 2023), 2009.
FAOSTAT: Forestry Production and Trade, FAO, Rome, https://www.fao.org/faostat/en/#data/FO (last access: 18 July 2023), 2023.
Flammini, A., Adzmir, H., Karl, K., and Tubiello, F. N.: Quantifying greenhouse gas emissions from wood fuel use by households, Earth Syst. Sci. Data, 15, 2179–2187, https://doi.org/10.5194/essd-15-2179-2023, 2023.
Font-Palma, C.: Methods for the treatment of cattle manure – A Review, J. Carbon Res., 5, 27, https://doi.org/10.3390/c5020027, 2019.
Garibaldi, L.: The FAO global capture production database: A six-decade effort to catch the trend, Mar. Policy, 36, 760–768, https://doi.org/10.1016/j.marpol.2011.10.024, 2012.
Gontard, N., Sonesson, U., Birkved, M., Majone, M, Bolzonella, D., Celli, A., Angellier-Coussy, H., Jang, G. W., Verniquet, A., Broeze, J., Schaer, B., Batista, A. P., and Sebok, A.: A research challenge vision regarding management of agricultural waste in a circular bio-based economy, Crit. Rev. Environ. Sci. Technol., 48, 614–654, https://doi.org/10.1080/10643389.2018.1471957, 2018.
Gonzalez-Garcia, S., Morales, P. C., and Gullon, B.: Estimating the environmental impacts of a brewery waste–based biorefinery: bio-ethanol and xylooligosaccharides joint production case study, Ind. Crops Prod., 123, 331–340, https://doi.org/10.1016/j.indcrop.2018.07.003, 2018.
Hamelin, L., Borzęcka, M., Kozak, M., and Pudełko, R.: A spatial approach to bioeconomy: Quantifying the residual biomass potential in the EU-27, Renew. Sust. Energ. Rev., 100, 127–142, https://doi.org/10.1016/j.rser.2018.10.017, 2019.
Heyl, K., Döring, T., Garske, B., Stubenrauch, J., and Ekardt, F.: The Common Agricultural Policy beyond 2020: A critical review in light of global environmental goals, Rev. Eur. Compar. Int. Environ. Law, 30, 95–106, https://doi.org/10.1111/reel.12351, 2021.
Huang, S., Zeng, Y., Wu, J., Shi, Q., and Pan, X.: Effect of crop residue retention on rice yield in China: A meta-analysis, Field Crop Res., 154, 188–194, https://doi.org/10.1016/j.fcr.2013.08.013, 2013.
Hergoualc'h, K., Akiyama, H., Bernoux, M., Chirinda, N., del Prado, A., Kasimir, A., MacDonald, J. D., Ogle, S. M., Regina, K., van der Weerden, T. J.: N2O Emissions from Managed Soils, and CO2Emissions from Lime and Urea Application. In: 2019 Refinement to the 2006 IPCC Guidelines for National Greenhouse Gas Inventories, edited by: Buendia, E. C., Tanabe, K., Kranjc, A., Baasansuren, J., Fukuda, M., Ngarize, S., Osako, A., Pyrozhenko, Y., Shermanau, P., and Federici, S., Volume 4: Agriculture, Forestry and Other Land Use Switzerland: Intergovernmental Panel on Climate Change (IPCC), https://www.ipcc-nggip.iges.or.jp/public/2006gl/pdf/4_Volume4/V4_11_Ch11_N2O&CO2.pdf, (last access: 29 January 2025), 2019.
Iriondo-DeHond, I., Iriondo-DeHond, M., and del Castillo, M. D.: Applications of compounds from coffee processing by-products, Biomolecules, 10, 1219, https://doi.org/10.3390/biom10091219, 2020.
Ji, C., Cheng, K., Nayak, D., and Pan, G.: Environmental and economic assessment of crop residue competitive utilization for biochar, briquette fuel and combined heat and power generation, J. Cleaner Prod., 192, 916–923, https://doi.org/10.1016/j.jclepro.2018.05.026, 2018.
Karan, S. K. and Hamelin, L.: Towards local bioeconomy: A stepwise framework for high-resolution spatial quantification of forestry residues, Renew. Sust. Energ. Rev., 134, 110350, https://doi.org/10.1016/j.rser.2020.110350, 2020.
Karan, S. K. and Hamelin, L.: Crop residues may be a key feedstock to bioeconomy but how reliable are current estimation methods?, Resour. Conserv. Recycl., 164, 105211, https://doi.org/10.1016/j.resconrec.2020.105211, 2021.
Khare, S. K., Jha, K., and Gandhi, A. P.: Citric acid production from okara (soy-residue) by solid-state fermentation, Biores. Technol., 54, 323–325, 1995.
Kizito, S., Luo, H., Lu, J., Bah, H., Dong, R., and Wu, S.: Role of nutrient-enriched biochar as a soil amendment during maize growth: Exploring practical alternatives to recycle agricultural residuals and to reduce chemical fertilizer demand, Sustainability, 11, 3211, https://doi.org/10.3390/su11113211, 2019.
Koopmans, A. and Koppejan, J.: Agricultural and forest residues -generation, utilization and availability. Paper presented at the Regional Consultation on Modern Applications of Biomass Energy, 6–10 January 1997, Kuala Lumpur, Malaysia, 1998.
Lim, K. O.: The energy potential and current utilization of agriculture and logging wastes in Malaysia, Renewable Energy Review Journal, 8, RERIC-AIT, Bangkok, 1986.
Lin, M. and Begho, T.: Crop residue burning in South Asia: A review of the scale, effect, and solutions with a focus on reducing reactive nitrogen losses, J. Environ. Manage., 314, 115104, https://doi.org/10.1016/j.jenvman.2022.115104, 2022.
Lopes, F. C. and Ligabue-Braun, R.: Agro-industrial residues: Eco-friendly and inexpensive substrates for microbial pigments production, Front. Sustain. Food Syst., 5, 589414, https://doi.org/10.3389/fsufs.2021.589414, 2021.
Loyon, L.: Overview of animal manure management for beef, pig, and poultry farms in France, Front. Sustain. Food Syst., 2, 36, https://doi.org/10.3389/fsufs.2018.00036, 2018.
Lu, F.: How can straw incorporation management impact on soil carbon storage? A meta-analysis, Mitig. Adapt. Strateg. Glob. Change, 20, 1545–1568, https://doi.org/10.1007/s11027-014-9564-5, 2015.
Ludemann, C. I., Hijbeek, R., van Loon, M., Murrell, T. S., Dobermann, A., and van Ittersum, M.: Global data on crop nutrient concentration and harvest indices, Dryad [data set], https://doi.org/10.5061/dryad.n2z34tn0x, 2023.
Ma, G., Chen, Y., and Ndegwa, P.: Anaerobic digestion process deactivates major pathogens in biowaste: A meta-analysis, Renew. Sust. Energ. Rev., 153, 111752, https://doi.org/10.1016/j.rser.2021.111752, 2022.
Marin, S., Andarge, T., and Foltz, J.: Effectiveness of local regulations on nonpoint source pollution: evidence from Wisconsin dairy farms, Amer. J. Agric. Econ., 105, 1333–1364, https://doi.org/10.1111/ajae.12388, 2023.
Masella, P., Guerrini, L., and Parenti, A.: The spent cake from olive oil filtration as biomass feedstock, Agric. Eng. Int., CIGR J., 16, 156–160, 2014.
Masoumi, S., Borugadda, V. B., Nanda, and Dalai, A. K.: Hydrochar: A review on its production technologies and applications, Catalysts, 11, 939, https://doi.org/10.3390/catal11080939, 2021.
Melo, L. C. A., Lehmann, J., Carneiro, J. S., and Camps-Arbestain, M.: Biochar-based fertilizer effects on crop productivity: a meta-analysis, Plant Soil, 472, 45–58, https://doi.org/10.1007/s11104-021-05276-2, 2022.
Millati, R., Cahyono, R. B., Ariyanto, T., Azzahrani, I. N., Putri, R. U., and Taherzadeh, M. J.: Agricultural, industrial, municipal, and forest wastes, in: Sustainable Resource Recovery and Zero Waste Approaches, edited by: Taherzadeh, M. J. Bolton, K., Wong, J., and Pandey, A., Elsevier, 1–22, https://doi.org/10.1016/B978-0-444-64200-4.00001-3, 2019.
Monforti, F., Bodis, K., Scarlat, N., and Dallemand, J. F.: The possible contribution of agricultural crop residues to renewable energy targets in Europe: A spatially explicit study, Renew. Sustain. Energy Rev., 19, 666–677, https://doi.org/10.1016/j.rser.2012.11.060, 2013.
Mozhiarasi, V. and Natarajan, T. S.: Slaughterhouse and poultry wastes: management practices, feedstocks for renewable energy production, and recovery of value added products, Biomass Conv. Bioref., 15, 1705–1728, https://doi.org/10.1007/s13399-022-02352-0, 2025.
Murphy, D. J., Goggin, K., and Paterson, R. R. M.: Oil palm in the 2020s and beyond: challenges and solutions, CABI Agric. Biosci., 2, 39, https://doi.org/10.1186/s43170-021-00058-3, 2021.
Oanh, N. T. K., Permadi, D. A., Hopke, P. K., Smith, K. R., Dong, N. P., and Dang, A. N.: Annual emissions of air toxics emitted from crop residue open burning in Southeast Asia over the period of 2010–2015, Atmos. Environ., 187, 163–173, https://doi.org/10.1016/j.atmosenv.2018.05.061, 2018.
Olsen, R. L., Toppe, J., and Karunasagar, I.: Challenges and realistic opportunities in the use of by-products from processing of fish and shellfish, Trends Food Sci. Technol., 36, 144–151, https://doi.org/10.1016/j.tifs.2014.01.007, 2014.
Onwudike, O. C.: Coconut (Cocos nucifera L.) kernel, oil and meal, in: Food and Feed from Legumes and Oilseeds, edited by: Nwokolo, E. and Smartt, J., Springer, Boston, MA, https://doi.org/10.1007/978-1-4613-0433-3_33, 1996.
Palm, C. A., Gachengo, C. N., Delve, R. J., Cadisch, G., and Giller, K. E.: Organic inputs for soil fertility management in tropical agroecosystems: application of an organic resource database, Agric. Ecosyst. Environ., 83, 27–42, https://doi.org/10.1016/S0167-8809(00)00267-X, 2001.
Padhye, L. P., Bandala, E. R., Wijesiri, B., Goonetilleke, A., and Bolan, N.: Hydrochar: A promising step towards achieving a circular economy and sustainable development goals, Front. Chem. Eng., 4, 867228, https://doi.org/10.3389/fceng.2022.867228, 2022.
Paudel, K. P., Bhattarai, K., Gauthier, W. M., and Hall, L. M.: Geographic information systems (GIS) based model of dairy manure transportation and application with environmental quality consideration, Waste Manage., 29, 1634–1643, https://doi.org/10.1016/j.wasman.2008.11.028, 2009.
Petersen, A. M., Melamu, R., Knoetze, J. H., and Görgens, J. F.: Comparison of second-generation processes for the conversion of sugarcane bagasse to liquid biofuels in terms of energy efficiency, pinch point analysis and life cycle analysis, Energy Convers Manage., 91, 292–301, https://doi.org/10.1016/j.enconman.2014.12.002, 2015.
Poveda, J., Martínez-Gómez, A., Fenoll, C., and Escobar, C.: The use of biochar for plant pathogen control, Phytopathol., 111, 1490–1499, https://doi.org/10.1094/PHYTO-06-20-0248-RVW, 2021.
Ronzon, T. and Piotrowski, S.: Are primary agricultural residues promising feedstock for the European bioeconomy?, Ind. Biotechnol., 13, 113–127, https://doi.org/10.1089/ind.2017.29078.tro, 2017.
Scarlat, N., Martinov, M., and Dallemand, J. F.: Assessment of the availability of agricultural crop residues in the European Union: Potential and limitations for bioenergy use, Waste Manag., 30, 1889–1897, https://doi.org/10.1016/j.wasman.2010.04.016, 2010.
Schmidt, M. W. I., Torn, M. S., Abiven, S., Dittmar, T., Guggenberger, G., Janssens, I. A., Kleber, M., Kögel-Knabner, I., Lehmann, J., Manning, D. A. C., Nannipieri, P., Rasse, D. P., Weiner, S., and Trumbore, S. E.: Persistence of soil organic matter as an ecosystem property, Nature, 478, 49–56, https://doi.org/10.1038/nature10386, 2011.
Shen, X., Huang, G., Yang, Z., and Han, L.: Compositional characteristics and energy potential of Chinese animal manure by type and as a whole, Appl. Energy, 160, 108–119, https://doi.org/10.1016/j.apenergy.2015.09.034, 2015.
Shepherd, K. D., Palm, C. A., Gachengo, C. N., and Vanlauwe, B.: Rapid characterization of organic resource quality for soil and livestock management in tropical agroecosystems using near-infrared spectroscopy, Agron. J., 95, 1314–1322, https://doi.org/10.2134/agronj2003.1314, 2003.
Shu, X, He, J., Zhou, Z., Xia, L., Hu, Y., Zhang, Y., Zhang, Y., Luo, Y., Chu, H., Liu, W., Yuan, S., Gao, X., and Wang, C.: Organic amendments enhance soil microbial diversity, microbial functionality and crop yields: A meta-analysis, Sci. Total Environ., 829, 154627, https://doi.org/10.1016/j.scitotenv.2022.154627, 2022.
Sileshi, G. W., Nhamo, N., Mafongoya, P. L., and Tanimu, J.: The stoichiometry of animal manure and its implications for nutrient cycling and agriculture in sub-Saharan Africa, Nutr. Cycl. Agroecosyst., 107, 91–105, https://doi.org/10.1007/s10705-016-9817-7, 2017.
Sileshi, G. W., Jama, B., Vanlauwe, B., Negassa, W., and Harawa, R.: Nutrient use efficiency and crop yield response to the combined application of cattle manure and inorganic fertilizer in sub-Saharan Africa, Nutr. Cycl. Agroecosyst., 113, 181–199, https://doi.org/10.1007/s10705-019-09974-3, 2019.
Sileshi, G. W., Barrios, E., Lehmann, J., and Tubiello, F. N.: Organic Matter Database (OMD), Zenodo [data set], https://doi.org/10.5281/zenodo.10450921, 2024.
Singh, B.: Rice husk ash, in: Woodhead Publishing Series in Civil and Structural Engineering, Waste and Supplementary Cementitious Materials in Concrete, edited by: Siddique, R. and Cachim, P., Woodhead Publishing, 417–460, https://doi.org/10.1016/B978-0-08-102156-9.00013-4, 2018.
Singh, B. and Craswell, E.: Fertilizers and nitrate pollution of surface and ground water: an increasingly pervasive global problem, SN Appl. Sci., 3, 518, https://doi.org/10.1007/s42452-021-04521-8, 2021.
Siqueira, M. U., Contin, B., Fernandes, P. R. B., Ruschel-Soares, R., Siqueira, P. U., and Baruque-Ramos, J.: Brazilian agro-industrial wastes as potential textile and other raw materials: a sustainable approach, Mater. Circ. Econ., 4, 9, https://doi.org/10.1007/s42824-021-00050-2, 2022.
Smerald, A., Rahimi, J., and Scheer, C.: A global dataset for the production and usage of cereal residues in the period 1997–2021, Sci. Data, 10, 685, https://doi.org/10.1038/s41597-023-02587-0, 2023.
Torrijos, M.: State of Development of Biogas Production in Europe, Procedia Environ. Sci., 35, 881–889, https://doi.org/10.1016/j.proenv.2016.07.043, 2016.
Tubiello, F. N., Karl, K., Flammini, A., Gütschow, J., Obli-Laryea, G., Conchedda, G., Pan, X., Qi, S. Y., Halldórudóttir Heiðarsdóttir, H., Wanner, N., Quadrelli, R., Rocha Souza, L., Benoit, P., Hayek, M., Sandalow, D., Mencos Contreras, E., Rosenzweig, C., Rosero Moncayo, J., Conforti, P., and Torero, M.: Pre- and post-production processes increasingly dominate greenhouse gas emissions from agri-food systems, Earth Syst. Sci. Data, 14, 1795–1809, https://doi.org/10.5194/essd-14-1795-2022, 2022.
van Dijk, K. C., Lesschen, J. P., and Oenema, O.: Phosphorus flows and balances of the European Union Member States, Sci. Total Environ., 542, 1078–1093, https://doi.org/10.1016/j.scitotenv.2015.08.048, 2016.
Van Hung, N., Maguyon-Detras, M. C., Migo, M. V., Quilloy, R., Balingbing, C., Chivenge, P., and Gummert, M.: Rice straw overview: availability, properties, and management practices, in: Sustainable Rice Straw Management, edited by: Gummert, M., Hung, N., Chivenge, P., and Douthwaite, B., Springer, 1–14, https://doi.org/10.1007/978-3-030-32373-8_1, 2020.
Vanlauwe, B., Gachengo, C., Shepherd, K., Barrios, E., Cadisch, G., and Palm, C. A.: Laboratory validation of a resource quality-based conceptual framework for organic matter management, Soil Sci. Soc. Amer. J., 69, 1135–1145, https://doi.org/10.2136/sssaj2004.0089, 2005.
Venkatramanan, V., Shah, S., Rai, A. K., and Prasad, R.: Nexus between crop residue burning, bioeconomy and Sustainable Development Goals over North-Western India, Front. Energy Res., 8, 614212, https://doi.org/10.3389/fenrg.2020.614212, 2021.
Wang, Y., Sun, J., and Lin, H.: Environmental pollution of livestock and poultry raising in rural areas and control measures: Taking Hebei province in China as an example, Nat. Env. Poll. Technol., 16, 849–855, 2017.
Wang, X., He, C., Liu, B., Zhao, X., Liu, Y., Wang, Q., and Zhang, H.: Effects of residue returning on soil organic carbon storage and sequestration rate in China's croplands: A meta-analysis, Agronomy, 10, 691, https://doi.org/10.3390/agronomy10050691, 2020.
Woolf, D.: Review of organic-matter resource databases in the agriculture sector and related industries. Report prepared on behalf of FAO, McKnight Foundation funded project (MTF/GLO/664/MKF), 2020.
Zhang, X., Fang, Q., Zhang, T., Ma, W., Velthof, G.L., Hou, Y., Oenema, O., and Zhang, F.: Benefits and trade-offs of replacing synthetic fertilizers by animal manures in crop production in China: A meta-analysis, Glob. Chang. Biol., 26, 888–900, https://doi.org/10.1111/gcb.14826, 2020.
Zhao, X., Chen, J., and Du, F.: Potential use of peanut by-products in food processing: a review, J. Food Sci. Technol., 49, 521–529, https://doi.org/10.1007/s13197-011-0449-2, 2012.
Zhao, X., Li, R. C., Liu, W. X., Liu, W. S., Xue, Y. H., Sun, R. H., Wei, Y. X., Chen, Z., Lal, R., Dang, Y. P., Xu, Z. Y., and Zhang, H. L.: Estimation of crop residue production and its contribution to carbon neutrality in China, Res. Conserv. Recycl., 203, 107450, https://doi.org/10.1016/j.resconrec.2024.107450, 2024.