the Creative Commons Attribution 4.0 License.
the Creative Commons Attribution 4.0 License.
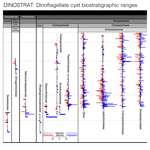
DINOSTRAT version 2.1-GTS2020
DINOSTRAT version 2.1-GTS2020 is now available (https://doi.org/10.5281/zenodo.10506652, Bijl et al., 2024b). This version updates DINOSTRAT to the Geologic Time Scale 2020, and new publications are added into the database. The resulting database now contains over 9450 entries from 209 sites. This update has not led to major and profound changes in the conclusions made previously. DINOSTRAT allows full presentation of the first and last stratigraphic occurrences of dinoflagellate cyst subfamilies and families, as well as the evolutionary turnover throughout geologic history, as a reliable representation of dinoflagellate evolution. Although the picture of dinoflagellate evolution from DINOSTRAT is broadly consistent with that in previous publications, with DINOSTRAT the underlying data are openly available, reproducible and up to date. This release of DINOSTRAT allows calibration of stratigraphic records to the Geologic Time Scale 2020 using dinoflagellate cysts as a biostratigraphic tool.
- Article
(2324 KB) - Full-text XML
- Companion paper
- BibTeX
- EndNote
DINOSTRAT version 1.1 (Bijl, 2022) offers a comprehensive open-access database of organic-walled dinoflagellate cyst (dinocyst from hereon) first and last occurrences (hereafter referred to as “events”). The dinocyst events in DINOSTRAT were calibrated to the Geologic Time Scale 2012 (GTS2012; Gradstein et al., 2012). In 2020, a new geologic timescale, the Geologic Time Scale 2020 (GTS2020), was published (Gradstein et al., 2020). I here release DINOSTRAT version 2.1-GTS2020, in which stratigraphic ranges of dinocysts are calibrated to the GTS2020 of Gradstein et al. (2020).
In the release of version 2.1 of DINOSTRAT, I updated stratigraphic calibrations of the dinocyst first and last occurrences to the GTS2020 (Gradstein et al., 2020). New papers that meet the criteria as laid out in DINOSTRAT version 1.1 (see Bijl, 2022, for more information about the approach) were added (see Table 1 for the new additions). The approach of data entry remained the same in this new release. Published dinocyst ranges were included when they could be calibrated to a chrono-, bio- or magnetostratigraphic zonation, whereby five reliability tiers were distinguished: dinocyst events were calibrated (1) against reliable magnetostratigraphy, (2) against compromised magnetostratigraphy, (3) against biostratigraphic zonations (nannofossils, foraminifer zones, ammonites), (4) indirectly against biostratigraphic zones (whereby the origin of those zones is not exactly clear, or these zones were not derived from the same material), and (5) from unclear chronostratigraphic constraints (Bijl, 2022). I had the palaeolatitude evolution of the “stable” sites (i.e. away from major plate boundaries or tectonically active regions) recalculated based on the upgraded https://paleolatitude.org (last access: 12 January 2024) calculator, using the framework of Vaes et al. (2023). I upgraded the plotting tools with extra features: the stages from the GTS2020 (Gradstein et al., 2020) are added to visually aid the interpretation of the plots.
The database is released as version 2.1-GTS2020 on GitHub and Zenodo (Bijl, 2024b, https://doi.org/10.5281/zenodo.10506652), on which the new datasets and plotting tools can be found. The supplements (Bijl, 2024a) contain all the new figures for families, genera, species and sites in DINOSTRAT. The calibration of some entries in DINOSTRAT version 1.1 had to be adjusted for DINOSTRAT version 2.1-GTS2020 because of a revision of the Cretaceous ammonite zonations in the GTS2020 (see Gradstein et al., 2020; Chap. Cretaceous). An overview of the changes induced by this can be found on GitHub (Bijl, 2024b). This update has not led to any major changes in the database. For most events, this update caused only minor adjustments in the calculated absolute age. The adjusted ammonite zonation scheme in the Cretaceous that the GTS2020 proposed did lead to a lot of recalibrations of dinocyst events. Yet, their absolute age should in principle not have changed much, since the ammonite zonation adjustment was mostly about redefining the index species and zone boundaries. With this update of DINOSTRAT, dinocyst events can now be used as a chronostratigraphic indicator on the GTS2020.
Table 1New papers added to DINOSTRAT version 2.1-GTS2020: reference, geography, age base and age top (in Ma), tier (see DINOSTRAT version 1), and means of calibration to the Geologic Time Scale. For the meaning of the abbreviations of the microplankton zones indicated in the column “Calibrated to”, the reader is referred to the GTS2020 (Gradstein et al., 2020).
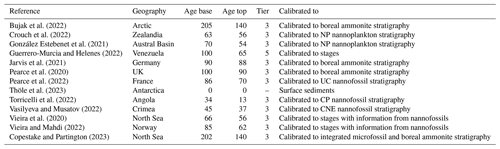
All the conclusions and interpretations made in the release paper of DINOSTRAT still stand with the addition of data and recalibration to the GTS2020. The North Atlantic Ocean is still strongly overrepresented in DINOSTRAT and the Pacific Ocean underrepresented in the geographical distribution of sites used to calibrate dinocyst assemblages (Fig. 1), and equatorial regions and the Southern Hemisphere remain underexplored, despite these being large ocean regions (Fig. 2). Bujak et al. (2022) added crucial calibrated dinocyst events from the Jurassic Arctic Basin. The addition of the new Antarctica-proximal surface sediment data of Thöle et al. (2023) adds geographic distribution information for modern dinocysts in the Southern Hemisphere, but the stratigraphic first occurrence of many modern species remains largely unknown (see supplements).
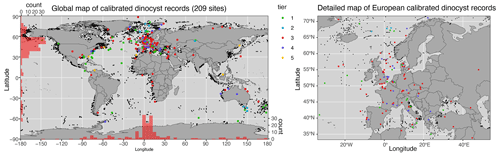
Figure 1Map of sites included in DINOSTRAT version 2.1-GTS2020. Maps produced using ggplot in R (Wickham, 2016).
Further exploration of DINOSTRAT has allowed the presentation of the stratigraphic ranges of dinocyst subfamilies and families (Fig. 3). As in Fig. 2b, Fig. 3 presents counts of the oldest first occurrences and youngest last occurrences of dinocyst genera and species but per subfamily or family, which yields the calibrated age of origination and final extinction of dinocyst subfamilies. This is a robust indication of the minimal stratigraphic range of these dinocyst subfamilies. It must be noted however that the suprageneric classification will remain in a state of flux for the coming decades, as many uncertainties remain. This may change the ranges of families and subfamilies described herein.
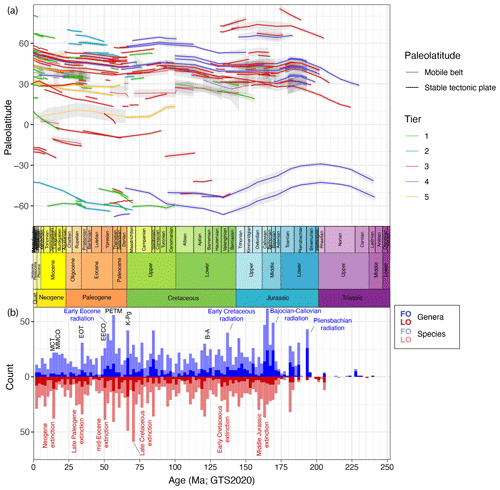
Figure 2(a) Palaeolatitude through time of sites used in DINOSTRAT version 2.1-GTS2020. (b) The number of the oldest first occurrences and youngest last occurrences of dinocyst species, plotted in 2 Myr bins. Plot produced using ggplot in R (Wickham, 2016).
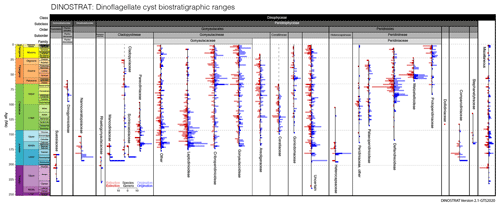
Figure 3Number of dinocyst species and genera with the oldest first occurrence and youngest last occurrence, plotted per dinocyst subfamily or family, in 2 Myr bins.
Peridiniphycideae have their first occurrence in the Ladinian (Middle Triassic), followed by Rhaetogonyaulacaceae, Suessiaceae and several miscellaneous Dinophyceae in the Carnian (Upper Triassic). The Heterocapsaceae, as first representatives of modern Peridiniales, and the Mancodiniaceae as first representatives of the modern (but now non-cyst-forming) Cladopyxiineae, Nannoceratopsiaceae and Comparodiniaceae first occur in the Sinemurian (Lower Jurassic). Scriniocassiaceae and Cladopyxiaceae (both part of the suborder of Cladopyxiineae) first occur in the Pliensbachian, which sees the last occurrence of the Shublikodiniaceae, the first of the cyst-forming dinocyst families to go extinct. The Toarcian sees the last occurrence of the Suessiales, as well as the first occurrence of the Pareodiniaceae and Leptodinioideae of the family Gonyaulacaceae, with many modern representatives. The Aalenian (Middle Jurassic) sees the first occurrence of the Cribroperidinioideae. The Gonyaulacoideae first occur in the Bajocian, during which also many other Gonyaulacaceae taxa have their first occurrences. The Scriniocassiceae have their last occurrence in this stage. The Stephanelytraceae and Areoligeraceae first occur in the Bathonian, while the Mancodiniaceae have their last occurrence. The Peridiniaceae first occur in the Callovian. The Deflandreoideae first occur in the Oxfordian (Upper Jurassic). The Nannoceratopsiaceae have their last occurrence and the Ceratiaceae their first occurrence in the Kimmeridgian. The Goniodomaceae have their first occurrence in the Tithonian. Palaeoperidinioideae first occur and Dollidiniaceae and Comparodiniaceae have their last occurrence in the Valanginian (Lower Cretaceous). Stephanelytraceae have their last occurrence in the Aptian. Heterocapsaceae last occur in the Albian; however, motile cells still live in the modern ocean (Fensome et al., 1993). In the Cenomanian (Upper Cretaceous) Dinogymnioideae first occur and Pareodiniaceae have their last occurrence. The Santonian sees the first occurrence of the Protoperidinioideae. The Ceratiaceae have their last occurrence at the end of the Maastrichtian. In the Danian (Paleocene), Wetzelielloideae have their first occurrence, the youngest subfamily to appear in the cyst record. Dinogymnioideae have their last occurrence in the Selandian; however motile cells of this subfamily still occur in the modern ocean (Fensome et al., 1993). The Palaeoperidinioideae, Cladopyxiaceae and Wetzelielloideae have their last occurrence in the Chattian (Oligocene). The Areoligeraceae have their last occurrence in the Burdigalian (Miocene). The Tortonian sees the last occurrence of the Leptodinioideae and the Messinian the last occurrence of the Deflandreoideae.
The counts of the oldest first occurrences and youngest last occurrences through time (Fig. 3) provide a rough indication of the amount of turnover in subfamilies. Yet, for several reasons I dictate caution not to overinterpret Fig. 3, particularly the implications of cyst species turnover for biological diversity and evolution. The first practical reason is that many entries in DINOSTRAT have only a first occurrence or last occurrence logged, not both (because of their long range or because of calibration limitations), which prevents assessing the full stratigraphic range of many species and, therefore, prevents the assessment of total diversity through time. The second reason for this caution is the chance of absence bias or false negatives in the fossil cyst record, for instance the absence of inocyst species because the corresponding dinoflagellate ceased to make cysts and not because it went extinct. Only about 10 % of modern dinoflagellates include a cyst phase in their life cycle (Bravo and Figueroa, 2014). It is impossible to assess whether this fraction of cyst-producing dinoflagellates has changed through time. Yet, the representation of many dinoflagellate families in the fossil record of dinocysts (Fig. 3) does suggest that a cyst phase was more common in a more diverse group of dinoflagellate taxa in the past. Interpreting what inocyst turnover would mean for the palaeobiology of dinoflagellates remains complex due to the apparent ability of a few inocyst families to switch cyst formation on and off: the Cladopyxiaceae and Ceratiaceae are known as modern motile cells (Fensome et al., 1993), yet their stratigraphic occurrence as cysts in the fossil record ended long before modern times (Fig. 3). This demonstrates that dinocyst diversity likely underestimates past dinoflagellate diversity, by an unknown amount that is likely not stable through time. Finally, dinocyst taxonomy is a morphological taxonomy and is not defined on genetic grounds. On a cyst genus level and higher, the defining morphological differences focus on plate tabulation (Fensome et al., 1993), a feature so fundamental to cell structure that it probably does reflect biological diversity. The defining features of many cyst species, however, are focused on ornament rather than tabulation, and for some of those features, it remains a question as to what extent these defining features reflect biological diversity, eco-phenotypical variability or effects of bio-provincialism. Examples of this complexity include a study whereby one strain of Gonyaulax spinifera produced cysts of Spiniferites and Nematosphaeropsis spp., with a full suite of morphological intermediates (Rochon et al., 2009). Such examples call into question the biological significance of cyst species also in the fossil record. Although surely there is a signal of biological diversity captured in fossil dinocyst species assemblages, the total species diversity needs to be treated with some caution.
The stratigraphic ranges of dinocyst subfamilies or families (Fig. 3) from DINOSTRAT match closely those in the dinocyst diversity plot of Fensome et al. (1996). This was expected, as both syntheses probably use overlapping literature resources as a basis for the inferred dinoflagellate evolution. DINOSTRAT delivers a verifiable, reproducible database that underpins dinoflagellate evolution and that is updated to the most recent geologic timescale, the GTS2020. It provides a platform that allows iterative improvement of the communities' collective knowledge of dinocyst biostratigraphy. The similarity to other overviews of evolution (Fensome et al., 1996) demonstrates the completeness of the DINOSTRAT database.
DINOSTRAT version 2.1-GTS2020, including R code to make the figures, is available on GitHub and Zenodo (Bijl, 2024b, https://doi.org/10.5281/zenodo.10506652).
Supplementary figures are available in Bijl (2024a, https://doi.org/10.5281/zenodo.10501273) for those not familiar with R programming:
-
palaeolatitude distributions of dinocyst subfamilies and species (2066 plots)
-
palaeolatitude distributions, stratigraphic ranges and distribution maps of dinocyst genera (471 plots)
-
palaeolatitude distributions, stratigraphic ranges and distribution maps of dinocyst families (27 plots)
-
palaeolatitude distributions of modern dinocyst species (96 plots)
-
stratigraphic ranges of dinocysts per site (211 plots).
The author has declared that there are no competing interests.
Publisher's note: Copernicus Publications remains neutral with regard to jurisdictional claims made in the text, published maps, institutional affiliations, or any other geographical representation in this paper. While Copernicus Publications makes every effort to include appropriate place names, the final responsibility lies with the authors.
The author thanks the LPP Foundation and the department of Earth Sciences of Utrecht University for supporting this work. Thanks go to Appy Sluijs, Henk Brinkhuis, Francesca Sangiorgi, Denise Kulhanek, Jeremy Young and Paul Bown for fruitful discussions. I thank the editor Giuseppe Manzella for helpful comments on this paper. Jan Hennissen, Rob Fensome and the anonymous reviewer are thanked for their constructive review of the paper.
This paper was edited by Giuseppe M. R. Manzella and reviewed by Jan Hennissen, Rob Fensome, and one anonymous referee.
Bijl, P. K.: DINOSTRAT: a global database of the stratigraphic and paleolatitudinal distribution of Mesozoic–Cenozoic organic-walled dinoflagellate cysts, Earth Syst. Sci. Data, 14, 579–617, https://doi.org/10.5194/essd-14-579-2022, 2022.
Bijl, P. K.: DINOSTRAT version 2.1-GTS2020 Supplements, Zenodo [code], https://doi.org/10.5281/zenodo.10501273, 2024a.
Bijl, P. K.: DINOSTRAT Version2.1-GTS2020 (2.1), Zenodo [data set], https://doi.org/10.5281/zenodo.10506652, 2024b.
Bravo, I. and Figueroa, R. I.: Towards an Ecological Understanding of Dinoflagellate Cyst Functions, Microorganisms, 2, 11–32, https://doi.org/10.3390/microorganisms2010011, 2014.
Bujak, J., Bringué, M., Goryacheva, A. A., Lebedeva, N. K., Pestchevitskaya, E. B., Riding, J. B., and Smelror, M.: Jurassic palynoevents in the circum-Arctic region, Atlantic Geoscience, 58, 55–98, https://doi.org/10.4138/atlgeo.2022.003, 2022.
Copestake, P. and Partington Mark, A.: Chapter 13, Biozonation of the Jurassic–lowermost Cretaceous of the North Sea region, Geological Society, London, Memoirs, 59, 387–436, https://doi.org/10.1144/M59-2022-61, 2023.
Crouch, E. M., Clowes, C. D., Raine, J. I., Alegret, L., Cramwinckel, M. J., and Sutherland, R.: Latest Cretaceous and Paleocene biostratigraphy and paleogeography of northern Zealandia, IODP Site U1509, New Caledonia Trough, southwest Pacific, New Zeal. J. Geol. Geop., 67, 20–44, https://doi.org/10.1080/00288306.2022.2090386, 2022.
Fensome, R. A., Taylor, F. J. R., Norris, G., Sarjeant, W. A. S., Wharton, D. I., and Williams, G. L.: A Classification of Modern and Fossil Dinoflagellates, Book, Whole, Micropalaeontology, Special Paper, Salem, Sheridan Press, Hanover, Pennsylvania, USA, 351 pp., 1993.
Fensome, R. A., MacRae, R. A., Moldowan, J. M., Taylor, F. J. R., and Williams, G. L.: The Early Mesozoic Radiation of Dinoflagellates, Paleobiology, 22, 329–338, 1996.
González Estebenet, M. S., Guler, M. V., and Pérez Panera, J. P.: Late Maastrichtian to Danian organic-walled dinoflagellate cysts and calcareous nannofossils from eastern Austral Basin, Patagonia, Argentina, Rev. Palaeobot. Palyno., 285, 104342, https://doi.org/10.1016/j.revpalbo.2020.104342, 2021.
Gradstein, F. M., Ogg, J. G., Schmitz, M. D., and Ogg, G. M.: The Geologic Time Scale 2012, ISBN 9780444594488, 2012.
Gradstein, F. M., Ogg, J. G., Schmitz, M. D., and Ogg, G. M.: Geologic Time Scale 2020, edited by: Gradstein, F. M., Ogg, J. G., Schmitz, M. D., and Ogg, G. M., Elsevier, https://doi.org/10.1016/B978-0-12-824360-2.00032-2, 2020.
Guerrero-Murcia, L. A. and Helenes, J.: Statistic biostratigraphy and paleoecology of tropical Upper Cretaceous dinoflagellate cysts, J. S. Am. Earth Sci., 115, 103730, https://doi.org/10.1016/j.jsames.2022.103730, 2022.
Jarvis, I., Pearce, M., Püttmann, T., Voigt, S., and Walaszczyk, I.: Palynology and calcareous nannofossil biostratigraphy of the Turonian – Coniacian boundary: The proposed boundary stratotype at Salzgitter-Salder, Germany and its correlation in NW Europe, Cretaceous Res., 123, 104782, https://doi.org/10.1016/j.cretres.2021.104782, 2021.
Pearce, M. A., Jarvis, I., Ball, P. J., and Laurin, J.: Palynology of the Cenomanian to lowermost Campanian (Upper Cretaceous) Chalk of the Trunch Borehole (Norfolk, UK) and a new dinoflagellate cyst bioevent stratigraphy for NW Europe, Rev. Palaeobot. Palyno., 278, 104188, https://doi.org/10.1016/j.revpalbo.2020.104188, 2020.
Pearce, M. A., Jarvis, I., Monkenbusch, J., Thibault, N., Ullmann, C. V., and Martinez, M.: Coniacian-Campanian palynology, carbon isotopes and clay mineralogy of the Poigny borehole (Paris Basin) and its correlation in NW Europe, C. R. Geosci., 354, 54–65, https://doi.org/10.5802/crgeos.118, 2022.
Rochon, A., Lewis, J., Ellegaard, M., and Harding, I. C.: The Gonyaulax spinifera (Dinophyceae) “complex”: Perpetuating the paradox?, Rev. Palaeobot. Palyno., 155, 52–60, https://doi.org/10.1016/j.revpalbo.2008.12.017, 2009.
Thöle, L. M., Nooteboom, P. D., Hou, S., Wang, R., Nie, S., Michel, E., Sauermilch, I., Marret, F., Sangiorgi, F., and Bijl, P. K.: An expanded database of Southern Hemisphere surface sediment dinoflagellate cyst assemblages and their oceanographic affinities, J. Micropalaeontol., 42, 35–56, https://doi.org/10.5194/jm-42-35-2023, 2023.
Torricelli, S., Menichetti, E., and Knezaurek, G.: Climate-driven dinoflagellate cyst stratigraphy of the Oligocene-lower Miocene turbidite succession of the Lower Congo Basin (offshore NW Angola), J. Afr. Earth Sci., 192, 104536, https://doi.org/10.1016/j.jafrearsci.2022.104536, 2022.
Vaes, B., van Hinsbergen, D. J. J., van de Lagemaat, S. H. A., van der Wiel, E., Lom, N., Advokaat, E. L., Boschman, L. M., Gallo, L. C., Greve, A., Guilmette, C., Li, S., Lippert, P. C., Montheil, L., Qayyum, A., and Langereis, C. G.: A global apparent polar wander path for the last 320 Ma calculated from site-level paleomagnetic data, Earth-Sci. Rev., 245, 104547, https://doi.org/10.1016/j.earscirev.2023.104547, 2023.
Vasilyeva, O. N. and Musatov, V. A.: Dinoflagellate cyst and nannoplankton assemblages from the Middle Eocene Kuma Formation of Crimean Peninsula – biostratigraphy and palynofacies, Palaeoworld, 32, 523–546, https://doi.org/10.1016/j.palwor.2022.11.006, 2022.
Vieira, M. and Mahdi, S.: New Late Cretaceous to earliest Paleogene (Campanian–Danian) dinoflagellate cysts from the Møre Basin, offshore Norway, Palynology, 46, 1–19, https://doi.org/10.1080/01916122.2021.2006817, 2022.
Vieira, M., Mahdi, S., and Holmes, N.: High resolution biostratigraphic zonation for the UK central North Sea Paleocene, Mar. Petrol. Geol., 117, 104400, https://doi.org/10.1016/j.marpetgeo.2020.104400, 2020.
Wickham, H.: ggplot2: Elegant Graphics for Data Analysis, Springer-Verlag, New York, ISBN 331924275X, 9783319242750, 2016.