the Creative Commons Attribution 4.0 License.
the Creative Commons Attribution 4.0 License.
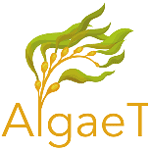
AlgaeTraits: a trait database for (European) seaweeds
Marine Robuchon
Stefanie Dekeyzer
Ignacio Bárbara
Inka Bartsch
Aurélie Blanfuné
Charles-François Boudouresque
Wim Decock
Christophe Destombe
Bruno de Reviers
Pilar Díaz-Tapia
Anne Herbst
Romain Julliard
Rolf Karez
Priit Kersen
Stacy A. Krueger-Hadfield
Ralph Kuhlenkamp
Akira F. Peters
Viviana Peña
Cristina Piñeiro-Corbeira
Fabio Rindi
Florence Rousseau
Jan Rueness
Hendrik Schubert
Kjersti Sjøtun
Marta Sansón
Dan Smale
Thierry Thibaut
Myriam Valero
Leen Vandepitte
Bart Vanhoorne
Alba Vergés
Marc Verlaque
Christophe Vieira
Line Le Gall
Frederik Leliaert
Olivier De Clerck
The analysis of biological and ecological traits has a long history in evolutionary and ecological research. However, trait data are often scattered and standardised terminology that transcends taxonomic and biogeographical context are generally missing. As part of the development of a global trait database of marine species, we collated trait information for European seaweeds and structured the data within the standardised framework of the World Register of Marine Species (WoRMS). We collected 45 175 trait records for 21 biologically and ecologically relevant traits of seaweeds. This resulted in a trait database for 1745 European seaweed species of which more than half (56 %) of the records were documented at the species level, while the remaining 44 % were documented at a higher taxonomic level and subsequently inherited at lower levels. The trait database for European seaweeds will serve as a foundation for future research on diversity and evolution of seaweeds and their responses to global changes. The data will contribute to developing detailed trait-based ecosystem models and will be an important tool to inform marine conservation policies. The data are publicly accessible through the AlgaeTraits portal, https://doi.org/10.14284/574 (AlgaeTraits, 2022).
Please read the corrigendum first before continuing.
-
Notice on corrigendum
The requested paper has a corresponding corrigendum published. Please read the corrigendum first before downloading the article.
-
Article
(5859 KB)
- Corrigendum
-
Supplement
(578 KB)
-
The requested paper has a corresponding corrigendum published. Please read the corrigendum first before downloading the article.
- Article
(5859 KB) - Full-text XML
- Corrigendum
-
Supplement
(578 KB) - BibTeX
- EndNote
Trait-based approaches, focusing on diversity of organismal characteristics rather than species diversity, are an effective data source to answer important biological questions (Costello et al., 2015; Beauchard et al., 2017; Degen et al., 2018). Traditionally, traits have been and still are of significant value for taxonomic research (Voultsiadou et al., 2017). Moreover, as they enable the description and investigation of complex ecosystems in relatively simple ways without having to laboriously study each individual component species, they are also integral to the study of the ecological and evolutionary dynamics of populations, species, communities, and ecosystems (Violle et al., 2007; Kattge et al., 2011; Degen et al., 2018; Schleuning et al., 2020). For example, the analysis of trait data can assist in estimating responses to multiple stressors, including anthropogenic and climate change impacts (Degen et al., 2018; Schleuning et al., 2020), and may provide crucial information needed to develop effective management strategies to counter negative consequences of climate change (Bremner, 2008).
Although the importance of trait-based approaches is widely recognised, there remains a general lack of accessible, standardised, and harmonised trait data for aquatic organisms (Costello et al., 2015; Beauchard et al., 2017; Degen et al., 2018; Martini et al., 2021). Recent initiatives have intended to resolve this gap and made significant progress for some marine animals, including fishes, polychaetes, copepods, and macroinvertebrates (reviewed in Martini et al., 2021), but aquatic photoautotrophs, including marine macroalgae (or seaweeds), remain underrepresented. Seaweed traits and functional forms have been used for at least one century as a tool to answer various ecological and evolutionary questions. Since the early 1900s, traits have been explored to formulate life-form classification schemes for algae which intended to reflect habitat requirements and responses to environmental fluctuations (Chapman and Chapman, 1976; Feldmann, 1966) or describe different reproductive strategies (De Wreede and Kinger, 1988; Bell, 1994) used to explain various evolutionary questions (Heesch et al., 2021). In the 1980s, Littler and Littler (1980) and Littler et al. (1983) used the adaptive value of morphological traits to categorise algal species into morpho-functional groups, which cluster species with similar ecological functioning. As the concept of morpho-functional groups is relatively easy to apply and can limit processing time and associated costs, it has been frequently applied in seaweed ecology (Veiga et al., 2013; Vélez-Rubio et al., 2021). As a result, multiple variations of the original six morpho-functional groups have been developed and applied in a wide range of contexts. For example, they have been applied to understand the distribution of communities along spatial (Steneck and Dethier, 1994; Wieters et al., 2012; Gaspar et al., 2017) or environmental scales (Balata et al., 2007; Gaspar et al., 2017; Gómez et al., 2019; Gómez and Huovinen, 2020), to understand the potential of communities to resist invasion (Arenas et al., 2006), and to evaluate the ecological status of coastal waters (Orfanidis et al., 2011). Yet, other recent research indicates that current morpho-functional groups may not capture enough variation to describe ecological functioning (Mauffrey et al., 2020; Ryznar et al., 2020) and call for further development towards a broader trait-based approach that includes non-morphological and other traits (Mauffrey et al., 2020).
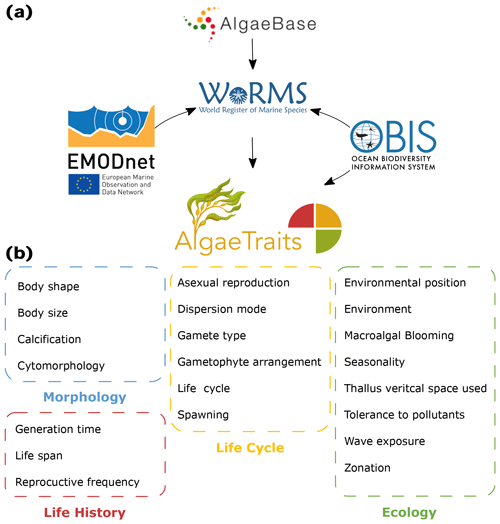
Figure 1(a) Relation of AlgaeTraits to other databases. AlgaeTraits is a subregister of the World Register of Marine Species (WoRMS), which aims to provide a comprehensive authoritative list of all published names of marine organisms and other biological information including traits. AlgaeBase, the most complete list on global algal taxonomy, is used as the main source for algal taxonomy in WoRMS. WoRMS and AlgaeTraits display OBIS occurrence data. All traits included in WoRMS and AlgaeTraits were collected under the EMODnet project. (b) All 21 traits currently included in AlgaeTraits can be categorised under morphology, life history, life cycle, and ecology.
Seaweed trait information remains largely scattered, not widely available, and not semantically standardised, which has hampered the development and application of phycological trait-based approaches over broad taxonomic, spatial, or temporal scales. To overcome such drawbacks and enhance the development of a common ontology favouring the use and comparability of trait-based approaches on seaweeds, we present AlgaeTraits, a seaweed trait database for 1745 species occurring along the Atlantic and Mediterranean coasts of Europe. We expand the spectrum of commonly used functional traits (i.e. measurable or quantifiable properties of individuals; McGill et al., 2006) to include data on habitat, seasonality, morphology, life cycle, life history, and biogeographical range (Fig. 1, Table A1). In total, we describe 21 traits linked to taxonomic information, covering 9 of the 10 previously prioritised traits in Costello et al. (2015). These data are available on the AlgaeTraits data portal https://doi.org/10.14284/574 (AlgaeTraits, 2022), which is a subregister of the World Register of Marine species (WoRMS, 2022; Marine Species Traits, 2022). Here we introduce AlgaeTraits by (i) presenting the methodologies used to collect data, (ii) highlighting trait coverage for the current and first version of the database, and (iii) exploring trait variability for a selected subset of traits.
2.1 Trait collection
Trait data collection started with an extensive literature review in 2013, supported by the Biology project of the European Marine Observation and Data Network (EMODnet). In 2015, the data were further refined with expert opinions (Supplement S1). In total, more than 200 references were used and 33 experts (all co-authors on this article) contributed by filling out a survey. For specific traits where interspecific variation is known to be minimal, trait information was documented in the World Register of Marine Species (WoRMS, 2022) at the genus level. The database behind WoRMS (Aphia, Vandepitte et al., 2015, 2018, see further) is built in such a way that the information at the genus level is then automatically inherited to all species within this genus, if no information at the species level was provided or available. Traits that may have substantial interspecific variation, such as blooming, seasonality, wave exposure, zonation, and body size (Table A1), were not inherited from the genus to species level and were only documented at the species level. Contributions were quality-checked to verify whether they conformed to the survey and the database format.
2.2 Trait data
We included 21 traits for marine seaweeds: 8 ecological traits, 4 morphological traits, 6 life cycle traits, and 3 life history related traits (Fig. 1, Table A1). Ecological traits relate to the habitat of the seaweed with respect to environment (marine, freshwater, brackish), tidal zonation, various degrees of wave exposure, environmental position (substrate), tolerance to organic pollutants, vertical space use, and seasonality. In the few instances where seaweed species are known for their potential to form blooms, this has been indicated as well (Fig. 1, Table A1). Morphological traits describe the thallus based on body shape, body size, calcification, and cytomorphology (Fig. 1, Table A1). Body size is the only quantitative trait included in AlgaeTraits and can be documented as a mean, maximum, or minimum value to describe the thallus length or diameter. Life-history-related traits describe patterns that influence demography and population dynamics, while life cycle traits relate to the various stages an organism undergoes from one stage in its development (e.g. fertilisation) to the same stage in the next generation (Albecker et al., 2021) (Fig. 1, Table A1). In addition to these 21 traits, species distributions were documented as ecoregions defined by Spalding et al. (2007) and can be used to subset the database according to geographic region. Moreover, WoRMS also displays species' occurrences of the Ocean Biodiversity Information System (OBIS; https://www.obis.org, last access: 2 March 2022) (Fig. 1). Contrary to the other traits, environment and distribution were not uploaded as traits (i.e. attributes) in the database system (Aphia; see further) but as environment flags and distributions. This implies that environment and distribution are slightly differently visualised on the AlgaeTraits data portal (https://www.algaetraits.org, last access: 10 September 2022).
2.3 Taxonomy
The total number of described seaweeds is estimated at 9250 species on a global scale (Appeltans et al., 2012; Guiry, 2012), of which at least 1800 species occur in Europe (Costello et al., 2022). The taxonomic classification of these species is not always straightforward and is continuously updated. To provide the best possible taxonomic accuracy, the trait data were implemented in WoRMS. WoRMS aims to provide a complete taxonomic authoritative list of all currently published names of marine species and is synchronised with AlgaeBase, the most complete database on global algal taxonomic information (Guiry and Guiry, 2022).
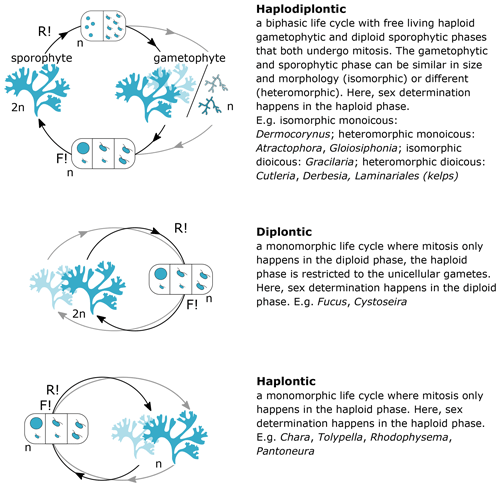
Figure 2Life cycles of seaweeds. The sexual life cycle of seaweeds can be divided in three main categories: (1) biphasic haplodiplontic life cycle, (2) monophasic haplontic life cycle, or (3) monophasic diplontic life cycle. Male and female reproductive structures (haploid gametes) can be formed on the same or separate thalli (mono- vs. di-; light blue indicates production of male and female gametes on separate individuals), and sex determination can happen in both the haploid and diploid life phase (-oicous vs. -oecious). The form of the gametes can be (1) identical in size and morphology (isogamous), (2) different in size but both motile with flagella (anisogamous), or (3) different in morphology and size with a bigger non-motile female gamete lacking a flagella and smaller motile male gametes with flagella (oogamous), except for red algae in which the male gamete is also non-motile. n – haploid, 2n – diploid, F! – fertilisation, R! – meiosis.
2.4 Metadata
Every trait value is linked to a source (expert or literature) and a note field that can be used to include relevant metadata related to specific trait values. As values for the traits asexual reproduction, macroalgal blooming, body size, and seasonality may display considerable geographic variation, we assigned a specific locality to these trait values. Localities are defined as georegions obtained from the Marine Regions platform, which provides a hierarchical, standardised list of georeferenced marine regions and areas (https://www.marineregions.org, last access: 6 April 2022) As mentioned above, life cycles of seaweeds can be complex and often consist of different life stages with radically different morphological, physiological, and ecological characteristics (Fig. 2). When relevant, trait values are documented for the specific life cycle stage they apply to (i.e. sporophyte, gametophyte, microthallus, macrothallus).
2.5 Database structure and management
The AlgaeTraits database is a thematic subregister of WoRMS, part of the Aphia platform. The Aphia platform is an MS SQL (Microsoft Structured Query Language) database specifically built to include taxonomic data and related information such as biological traits. In total, Aphia contains more than 400 data fields, which are maintained by more than 500 experts under guidance of the WoRMS steering committee, which takes the lead on setting priorities and future directions and coordination of the experts (Vandepitte et al., 2015, 2018). Both editors and database users are supported by a data management team that includes technical and scientific staff hosted at the Flanders Marine Institute (VLIZ) and can be contacted through info@marinespecies.org. This data management team is committed to safeguarding the integrity and online access of the database.
Twenty-one traits were documented resulting in 45 195 entries for 2830 accepted taxa according to AlgaeBase, among which 681 were at the genus level and 1742 at the species level. Fifty-six percent of the trait entries for species were inherited from the genus level. Environment and cytomorphology were the traits most documented for species, while the least information was provided for the traits that show substantial variation within genera and that were not inherited from genus to species level (Fig. 3).
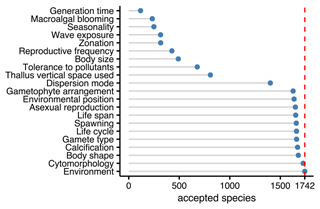
Figure 3Number of taxonomically accepted species from Europe with trait information by trait. The dashed red line indicates the total number of accepted species (1742) according to AlgaeBase.
3.1 Morphology-related traits
3.1.1 Body shape
Seaweeds are known for their wide variation in body shapes, as illustrated in Fig. 4. For example, seaweeds can exist as filaments (filamentous) or be leaf-like (foliose) or cushion-like (saccate). They can trail closely along the surface (prostrate), be upright (erect), or take many other shapes (Table A1, Fig. 4). Body shape had a high data coverage of 96 % (1678 species) at the species level (Figs. 3, 5–7).
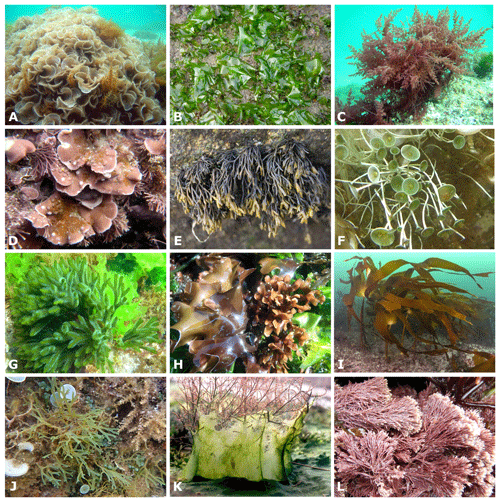
Figure 4Morphological body shape diversity of seaweeds. (a) Flabellate and calcified Padina, (b) foliose Ulva, (c) branched Asparagopsis, (d) crustose and calcified Mesophyllum, (e) branched Pelvetia, (f) siphonous and calcified Acetabularia, and (g) branched and siphonous Codium. (h) Chondrus (left) and Mastocarpus (right) (i) erect Laminaria, (j) dichotomously branched Dictyota, (k) filamentous Rhizoclonium, and (l) calcified and articulated Jania. Photo credits: (a–d), (f–h), (k–l): Ignacio Bárbara; (e, j): Olivier De Clerck; (i) Cristina Piñeiro-Corbeira.
3.1.2 Body size
Seaweed body size was described in several dimensions (thallus length, diameter, width, thickness, height) and varied from a few micrometres in thickness or width (e.g. filamentous, turf forming species such as Hapalospongidion macrocarpum) to several metres long (e.g. kelp such as Saccharina latissima). Compared to other traits, body size had a relatively low data coverage of 28 % (490 species) for European accepted seaweed species (Figs. 3, 5–7).
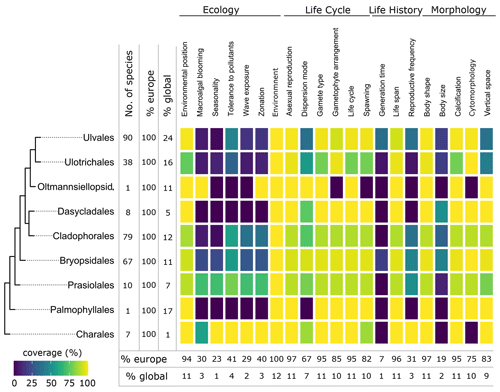
Figure 5Data coverage for green seaweeds. The taxonomic coverage is restricted to marine representatives. The order Charales is included to accommodate a limited number of species that occur in brackish habitats. The left side shows a phylogenetic tree at the ordinal level. Next to the tree the number of species with trait information available in the database is indicated as the total number (no. of species), the percentage relative to the total number of European species (% Europe), and the percentage relative to the total number of global species (% global). The heatmap indicates the percentage of trait coverage relative to the total number of European species per order per trait included in the database. At the bottom of the figure, average species coverage per trait is presented as a percentage of the total number of European (% Europe) and global (% global) species. Oltmannsiellopsid. refers to Oltmannsiellopsidales.
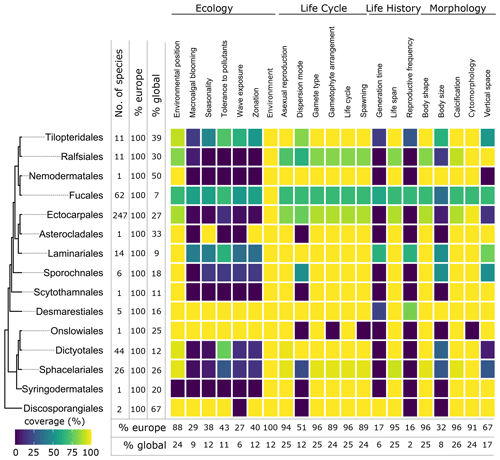
Figure 6Data coverage for brown seaweeds (Phaeophyceae). The left side shows a phylogenetic tree at the ordinal level. Next to the tree the number of species with trait information available in the database is indicated as the total number (no. of species), the percentage relative to the total number of European species (% Europe), and the percentage relative to the total number of global species (% global). The heatmap indicates the percentage of trait coverage relative to the total number of European species per order per trait included in the database. At the bottom of the figure, average species coverage per trait is presented as a percentage of the total number of European (% Europe) and global (% global) species.
3.1.3 Calcification
Several species in different taxonomic groups have calcified thalli (Figs. 8–10). In the green seaweed orders Dasycladales and Bryopsidales, calcification can occur as intra- or extracellular aragonite deposition, and both orders contain calcified articulated and calcified non-articulated species (Fig. 8). In European brown seaweeds, calcification is restricted to Padina (Dictyotales), where the surface of several species is covered with aragonite crystals (Benita et al., 2018) (Figs. 4a, 9). Calcification in various forms is present in four European orders of red seaweeds: in the Corallinales and Sporolithales as calcite in the cell walls (Figs. 4d, 10), whereas the Peyssonneliales and Nemaliales deposit aragonite (Pentecost, 1980). Calcification had a high data coverage of 96 % (1670 species) on a European level (Figs. 3, 5–7).
3.1.4 Cytomorphology
Cytomorphology was the trait best documented on a European level and reached a data coverage of 99 % (1726 species) (Figs. 4, 5–7). Although the trait which distinguishes unicellular from multicellular organisms and therefore might be perceived as trivial, in a seaweed context, it does set apart macroscopic multicellular thalli from a series of exotic cytomorphologies, including coenocytic and siphonal growth forms. Especially in green seaweeds (e.g. Bryopsidales and Dasycladales), the morphology of the thallus is decoupled from the formation of cells, which may result in thalli tens of centimetres tall and differentiated in blade-like structures, stolons and rhizoids while still being essentially unicellular.
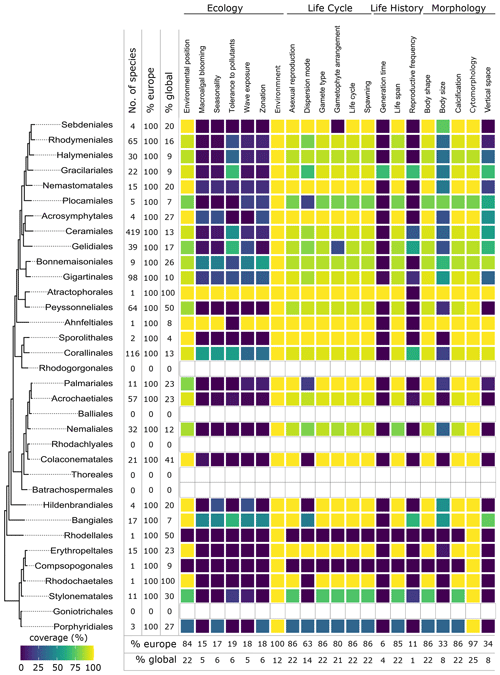
Figure 7Data coverage for red seaweeds (Rhodophyta). The left side shows a phylogenetic tree at the ordinal level. Next to the tree the number of species with trait information available in the database is indicated as the total number (no. of species), the percentage relative to the total number of European species (% Europe), and the percentage relative to the total number of global species (% global). The heatmap indicates the percentage of trait coverage relative to the total number of European species per order per trait included in the database. At the bottom of the figure, average species coverage per trait is presented as a percentage of the total number of European (% Europe) and global (% global) species. Orders with blank bars are not present in European marine waters.
3.2 Ecology-related traits
3.2.1 Environmental position
Environmental position was well documented on the European level with 94 % (1636 species) (Figs. 3, 5–7). Most seaweeds grow attached to rock (epilithic) or other macrophytes (epiphytic), while some other species grow on animals (epizoic) or within rocks (endolithic), macrophytes (endophytic), or animals (endozoic) (Table A1). Many seaweeds are not very specific with respect to the substrate onto which they are attached. The same species may grow epilithically or epiphytically, but some species show high substrate specificity. Several diminutive algal species (e.g. Acrochaete, Acrochaetium, Laminariocolax and Myrionema) grow exclusively epiphytically, endophytically, or even endozoically on a variety of hosts. At least for some species (e.g. Vertebrata lanosa being associated with Ascophyllum nodosum), high substrate specificity has been demonstrated (Garbary, 2017).
3.2.2 Macroalgal blooming
Macroalgal blooming indicates the demonstrated capacity of a species to produce blooms. This trait had relatively low data coverage of 13 % (233 species) on a European level (Figs. 3, 5–7). Notorious examples of blooming species include Ulva prolifera, Cladophora glomerata, Caulerpa cylindracea, and holopelagic Sargassum and Rugulopteryx okamurae, and events have been often linked to eutrophication (Charlier et al., 2008; Pierucci et al., 2019; Smetacek and Zingone, 2013). The trait, however, shows considerable variation with geographic location. This is exemplified by several non-native species, which bloom in the invaded region while showing non such behaviour in their regions of origin. This variation was incorporated in the database by linking entries for macroalgal blooming to geographic localities.
3.2.3 Seasonality
Seasonality had a relatively low data coverage of 14 % on a European level (249 species) (Figs. 3, 5–7). Seaweeds with large geographical ranges adapt to local seasonal conditions by adjusting their phenology (Lüning, 1991). It is therefore possible for a species to be present in one season in one part of its range while being absent elsewhere.
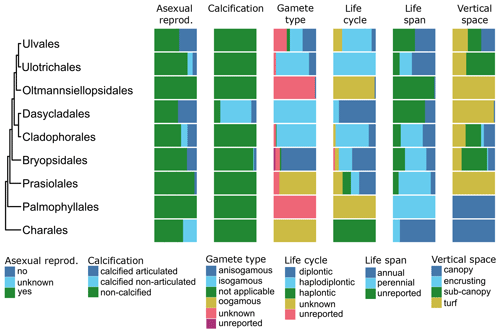
Figure 8Trait variability for a selected set of traits (asexual reproduction, calcification, gamete type, life cycle, life span, vertical space) for European green seaweeds. The left side shows a phylogenetic tree at the ordinal level. On the right stacked bar plots indicate trait variability as the proportion of species exhibiting a certain trait value by order and by trait.
3.2.4 Vertical space
All types of vertical space (encrusting, turf, sub-canopy, canopy; Table A1) occur and vary among and within orders of the green (Fig. 8), brown (Fig. 9), and red seaweeds (Fig. 10). Vertical space had a moderate coverage of 46 % on European level (808 species) (Figs. 3, 5–7).
3.2.5 Tolerance to organic pollutants
Some seaweeds have a lower tolerance to turbidity or nutrient concentrations than others (Table A1). For example, Choristocarpus tenellus is mainly observed in waters with low nutrient levels and high visibility (oligotrophic, clear water; Table A1), while Ulva spp. are mainly observed from waters with mid to high nutrient concentration (mesotrophic, eutrophic; Table A1). Tolerance to organic pollutants was covered for 39 % of European accepted seaweeds (678 species) (Figs. 3, 5–7).
3.2.6 Wave exposure
Some seaweed species are solely known from habitats with low-energy wave forces (sheltered; Table A1) such as Chaetomorpha adrianii or from habitats with solely high-energy wave forces (exposed; Table A1) such as Valonia utricularis. Other seaweeds can occur in a variety of wave exposures including sheltered semi-exposed and exposed such as the non-native Sargassum muticum. Wave exposure had a relatively low coverage of 18 % on the European level (315 species) (Figs. 3, 5–7).
3.2.7 Zonation
Many species are physiologically adapted to endure the stress of tidal differences and daily changes between desiccation and submersion (e.g. Pelvetia canaliculata). Other species will only occur below the low water mark and do not endure such high variation in desiccation, salinity, temperature, or other stressors under normal conditions (e.g. Alaria esculenta). Zonation had a relatively low data coverage of 18 % on a European level (316 species) (Figs. 3, 5–7).
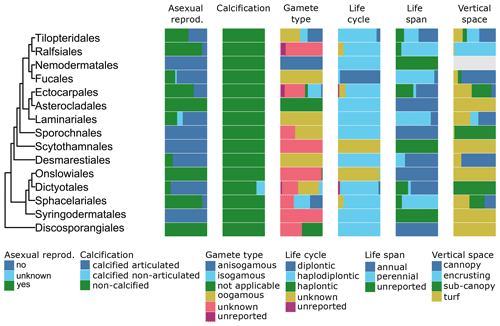
Figure 9Trait variability for a selected set of traits (asexual reproduction, calcification, gamete type, life cycle, life span, vertical space) for European brown seaweeds (Phaeophyceae). The left side shows a phylogenetic tree at the ordinal level. On the right stacked bar plots indicate trait variability as the proportion of species exhibiting a certain trait value by order and by trait. Grey bars indicate missing data.
3.3 Life-cycle-related traits
3.3.1 Life cycle
Information on life cycle was available for 95 % of European seaweed species (1660 species) (Figs. 3, 5–7). Many seaweeds have a biphasic or haplodiplontic life cycle in which a diploid sporophytic and a haploid gametophytic life phase alternate. These can have a similar (isomorphic; e.g. Chondrus crispus) or distinct (heteromorphic) body shape (e.g. Laminariales) (Fig. 2). Haplodiplontic life cycles are the dominant type of life cycle in the brown (Fig. 9) and red (Fig. 10) seaweeds. Other seaweeds have a monophasic life cycle that is either diploid (diplontic) or haploid (haplontic) (Fig. 2). Variation in the life cycle has been documented in several species either in the lab (e.g. in reds, Maggs, 1988; in Ectocarpus, Coelho et al., 2012) or in the field (e.g. in Gracilaria, Destombe et al., 1989). However, the knowledge about the processes driving this variation (e.g. epigenetic (plastic) or genetic bases) is not known.
3.3.2 Asexual reproduction
In many seaweeds, sexual reproduction occurs alongside asexual reproduction (i.e. partial clonality) that does not involve fusion of gametes or meiosis and usually results in progeny with an identical genetic constitution to the parent and to each other (Table A1). Asexual reproduction is widely spread in green, brown, and red seaweeds (Figs. 8–10) and can happen through fragmentation, direct development of spores, parthenogenesis, or other mechanisms. Asexual reproduction had a high data coverage of 95 % on a European level (1649 species) (Figs. 3, 5–7).
3.3.3 Dispersion mode
Seaweed dispersal is heavily influenced by ocean currents and water motion (but in red seaweeds, animal mediated transport of male gametes could be important; Lavaut et al., 2022) and is in most cases limited in spatial scale. But dispersal over longer distances is also possible when the species can for example drift, or the dispersal is mediated by vectors such as boat hulls (Table 1A). Dispersion mode has a moderate data coverage of 80 % on the European level (1401 species) (Figs. 3, 5–7).
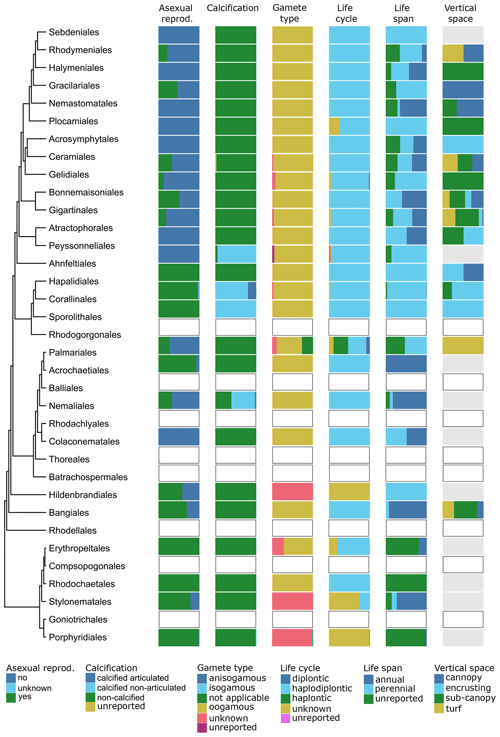
Figure 10Trait variability for a selected set of traits (asexual reproduction, calcification, gamete type, life cycle, life span, vertical space) for European red seaweeds. The left side shows a phylogenetic tree at the ordinal level. On the right stacked bar plots indicate trait variability as the proportion of species exhibiting a certain trait value by order and by trait. Orders with blank bars are not present in European marine waters, and grey bars indicate missing data.
3.3.4 Gamete type
Gamete type varies among and within orders of green and brown seaweeds (Figs. 8, 9). In red seaweeds, gamete type is more conserved: male gamete is unflagellated (spermatia), and fertilisation takes place in the female organ called the carpogonium. This type of reproduction is considered oogamous when information is available. This trait was documented for 95 % of the European seaweeds (1661 species) (Figs. 3, 5–7).
3.3.5 Gametophyte arrangements
In seaweeds, the male and female gametes can be formed on the same or separate thalli (mono- vs. di-); sex determination can happen in both the haploid and diploid life phase depending on the taxon (-oicous vs. -oecious) (Fig. 3, Table A1). For example, in fucoids, sex determination occurs in the diploid-dominant stage, whereas, in all red seaweeds, sex determination occurs in the haploid stage. Gametophyte arrangement was documented for 93 % of the European seaweed species (1626 species) (Figs. 3, 5–7).
3.3.6 Spawning
For the majority of the brown and green seaweeds, fertilisation occurs in the water column, but in red seaweeds, male gametes are not flagellated and fertilisation occurs on the female gametophyte and gives rise to the “third phase” of the life cycle called the carposporophyte. Spawning has been documented for 95 % of European species (1658 species) (Figs. 3, 5–7).
3.4 Life history-related traits
3.4.1 Generation time
Of all traits, least information was collected for the trait generation time (Fig. 4b). In seaweeds, generation time can vary from just a few weeks in Ulva (Wichard et al., 2015) to multiple decades such as in Gracilaria (Engel et al., 2001) or Ascophyllum (Åberg, 1992). Generation time was documented for only 7 % of the European seaweed (117 species) (Figs. 3, 5–7).
3.4.2 Life span
Life span varies within and among European green, brown, and red seaweeds (Figs. 8–10). Perennial macroalgae can live up to multiple years such as for several years. Many kelp species such as Laminariales or Gracilaria gracilis can live for more than 50 years (Engel et al., 2001); Ascophyllum nodosum can live for more than 120 years (Åberg, 1992). In contrast, annuals live only a few months, such as several small filamentous species (e.g. Chaetomorpha). At the European level, data coverage was high for life span, 95 % (1652 species) (Figs. 3, 5–7).
3.4.3 Reproductive frequency
Data coverage was limited for reproductive frequency, with data available for only 25 % for the European species (427 species) (Figs. 3, 5–7).
AlgaeTraits includes 21 traits and 1742 European seaweed species, and it is structured within a general framework and ontology aiming to describe all marine species (Costello et al., 2015). With a mean coverage of ∼60 % per trait, this database will be a solid tool for a variety of biological research and related fields, including marine conservation, nature-based solutions, and aquaculture. For example, traits can be used to monitor community and ecosystem changes (McGill et al., 2006; Vélez-Rubio et al., 2021) or to identify conservation priorities (Albouy et al., 2017; Cardeccia et al., 2018; Esmaeili et al., 2022). In addition, they can be incorporated into predictive modelling to assess eco-evolutionary consequences of climate change (Schleuning et al., 2020), can contribute to predicting the invasiveness of species (Nyberg and Wallentinus, 2005; Quell et al., 2021), can be used in research aiming to better understand the driving forces of evolutionary trait history (Heesch et al., 2021) or the mechanisms of community assembly (Weiss and Ray, 2019), and can even help to assess ecosystem service vulnerability (Díaz et al., 2013; Stevenson, 2014).
The AlgaeTraits database complements recent efforts to collect and publicly provide well-structured and organised seaweed trait data. Just as MarLIN (2006) and SeaTraIn (2022) we focussed on biologically important traits. But contrary to these other initiatives, AlgaeTraits includes all European seaweed species and presents a different, yet overlapping, set of traits. MarLIN (2006) covers over 40 traits, of which 12 overlap with AlgaeTraits, but it is limited to only 30 seaweed species. SeaTrain (2022) covers around 10 traits, of which 2 traits overlap with AlgaeTraits, and includes 96 seaweed species. As to taxonomic coverage (1742 species) and inclusion of functional important traits as prioritised by Costello et al. (2015), AlgaeTraits is the most extensive seaweed trait database published so far.
In this first version of AlgaeTraits, not all traits are available for all species yet, and the current list of traits is not yet complete. For example, other key eco-evolutionary traits that might be considered to be included in the database are tolerance to light, tolerance to temperature, tolerance to grazing, tolerance to sedimentation, or tolerance to epiphytism. There is currently no possibility to describe parasitic algal life forms in the database, ignoring a relatively diverse group of red algae. Completion and further refining and expanding the database will be an ongoing effort of the AlgaeTraits editor community. AlgaeTraits currently has 30 trained thematic editors who actively update the trait information on a voluntary basis through the online editing interface (https://www.algaetraits.org, last access: 10/09/2022) and gradually work on the expansion of this European database to a global database. The AlgaeTraits editorial community will also need to report to the WoRMS steering committee. As the database expands, adding new traits or trait values can be considered under thorough consideration and discussion with the WoRMS steering committee and data management team.
For now, traits are included at the species level in AlgaeTraits. However, multiple traits can exhibit substantial intraspecific variation (Kattge et al., 2011). For example, morphological seaweed traits can differ among populations depending on underlying genetic patterns (Serisawa et al., 2003) or depending on environmental conditions such as wave exposure with rather smaller and more slender individuals in exposed than sheltered localities (Ruuskanen et al., 1999; Fowler-Walker et al., 2006; Kim et al., 2022). But also habitat-preference, life-history (Araújo et al., 2011), or life-cycle traits can vary intraspecifically. Some seaweeds reproduce sexually under normal conditions but change to asexual reproduction under specific environmental conditions (Demes and Graham, 2011; Murúa et al., 2017) or at the limits of their distribution (i.e. geographic parthenogenesis: Oppliger et al., 2014; Hoshino et al., 2021). Intraspecific trait variation is thus caused by both genetic variation and phenotypic plasticity and can be a response to improve performance under specific environmental biotic or abiotic conditions (Kattge et al., 2011). At the moment, we considered intraspecific variation by assigning localities (or life stages) to the trait values when possible or specifying detailed information in the note field. However, complementary approaches measuring trait information at specimen level (e.g. Mauffrey et al., 2020; Cappelatti et al., 2019) are useful, especially for quantitative traits, to fully capture intraspecific trait variability and allow more in-depth analysis.
The data can be accessed from the AlgaeTraits portal, https://doi.org/10.14284/574 (AlgaeTraits, 2022).
We provided a consolidated database of important traits of European seaweeds that is distinct in its completeness and taxonomic coverage. Because the database is standardised and fits within the broader framework of WoRMS that aims to include all taxa described, it serves not only as a significant resource for phycological research focusing on trait-based ecology or evolution, but also for general macro-ecological and macroevolutionary research in general. The database can help to explore ecological questions about relations among traits or help to unravel taxonomic and evolutionary patterns of traits in seaweeds. Because some trait values vary geographically and some traits values were documented at the genus level, the database may not always provide the highest variation at the species level. However, an active thematic editorial community is currently committed to further updating and improving the quality of this European database and expanding it to a global level to facilitate thorough and broad-scale trait-based analysis.
The supplement related to this article is available online at: https://doi.org/10.5194/essd-15-2711-2023-supplement.
SV, MR, IBD, IB, AB, C-FB, CD, BDR, PD-T, AH, RJ, RK. PK, SAKH, RK, AFP, VP, CP-C, FB, FR, JR, HS, IKS, MS, DS, TT, MVa, AV, MVe, CV, LL, FL, and ODC contributed data. SV and MR collated the data and performed quality control. SD, WD, BV, and LV supported data curation. SV performed analysis and wrote the original draft with help from MR, LV, LL, FL, and ODC. All authors approved and contributed to the final draft for submission. LLG, FL, and ODC contributed equally as last authors.
The contact author has declared that none of the authors has any competing interests.
Publisher's note: Copernicus Publications remains neutral with regard to jurisdictional claims in published maps and institutional affiliations.
This research has been supported by the Fonds Wetenschappelijk Onderzoek (grant nos. GOH3817N, I001621N, I000819N, and I002021N) and the European Union (grant no. SI2.657705).
This paper was edited by Giuseppe M.R. Manzella and reviewed by Annalisa Minelli and one anonymous referee.
Åberg, P.: A demographic study of two populations of the seaweed Ascophyllum nodosum, Ecology, 73, 1473–1487, https://doi.org/10.2307/1940691, 1992.
Albecker, M. A., Wilkins, L. G., Krueger-Hadfield, S. A., Bashevkin, S. M., Hahn, M .W., Hare, M. P., Kindsvater, H. K., Sewell, M. A., Lotterhos, K. E., and Reitzel, A. M.: Does a complex life cycle affect adaptation to environmental change? Genome-informed insights for characterizing selection across complex life cycle, P. R. Soc. B, 288, 20212122, https://doi.org/10.1098/rspb.2021.2122, 2021.
Albouy, C., Delattre, V. L., Mérigot, B., Meynard, C. N., and Leprieur, F.: Multifaceted biodiversity hotspots of marine mammals for conservation priorities, Divers. Distrib., 23, 615–626, https://doi.org/10.1111/ddi.12556, 2017.
AlgaeTraits: A trait database for European seaweeds, AlgaeTraits [data set], https://doi.org/10.14284/574, 2022.
Appeltans, W., Ahyong, S. T., Anderson, G., Angel, M. V., Artois, T., Bailly, N., Bamber, R., Barber, A., Bartsch, I., Berta, A., and Błażewicz-Paszkowycz, M.: The magnitude of global marine species diversity, Curr. Biol., 22, 2189–2202, https://doi.org/10.1016/j.cub.2012.09.036, 2012.
Arenas, F., Sánchez, I., Hawkins, S. J., and Jenkins, S. R.: The invasibility of marine algal assemblages: role of functional diversity and identity, Ecology, 87, 2851–2861, https://doi.org/10.1890/0012-9658(2006)87[2851:tiomaa]2.0.co;2, 2006.
Araújo, R., Serrão, E.A., Sousa-Pinto, I., and Åberg, P.: Phenotypic differentiation at southern limit borders: the case study of two fucoid macroalgal species with different life-history traits, J. Phycol., 47, 451–462, https://doi.org/10.1111/j.1529-8817.2011.00986.x, 2011.
Balata, D., Piazzi, L., and Cinelli, F.: Increase of sedimentation in a subtidal system: effects on the structure and diversity of macroalgal assemblages, J. Exp. Mar. Biol. Ecol., 351, 73–82, https://doi.org/10.1016/j.jembe.2007.06.019, 2007.
Beauchard, O., Veríssimo, H., Queirós, A. M., and Herman, P. M. J.: The use of multiple biological traits in marine community ecology and its potential in ecological indicator development, Ecol. Indic., 76, 81–96, https://doi.org/10.1016/j.ecolind.2017.01.011, 2017.
Bell, G.: The comparative biology of the alternation of generations, in: Lectures on mathematics in life sciences: The evolution of haplo-diploid life cycles, edited by: Kirpatrick M., American Mathematical Society, Providence, Rhode Island, 1–26, ISBN 0-8218-1176-2, 1994.
Benita, M., Dubinsky, Z., and Iluz, D.: Padina pavonica: morphology and calcification functions and mechanism, Am. J. Plant Sci., 9, 1156–1168, https://doi.org/10.4236/ajps.2018.96087, 2018.
Beukeboom, L. W. and Perrin, N.: The evolution of sex determination, Oxford University Press, USA, ISBN 978-0-19-965714-8, 2014.
Bremner, J.: Species' traits and ecological functioning in marine conservation and management, J. Exp. Mar. Biol. Ecol., 366, 37–47, https://doi.org/10.1016/j.jembe.2008.07.007, 2008.
Brodie, J., Maggs, C. A., and John, D. M.: Green Seaweeds of Britain and Ireland, British Phycological Society, The United Kingdom, ISBN 0952711532, 2007.
Cappelatti, L., Mauffrey, A. R., and Griffin, J. N.: Applying continuous functional traits to large brown macroalgae: variation across tidal emersion and wave exposure gradients, Mar. Biol., 166, 1–12, https://doi.org/10.1007/s00227-019-3574-5, 2019.
Cardeccia, A., Marchini, A., Occhipinti-Ambrogu, A., Gali, l. B., Gollasch, S., Minchin, D., Narščius, A., Olenin, S., and Ojaveer, H.: Assessing biological invasions in European Seas: Biological traits of the most widespread non-indigenous species, Estuar. Coast. Shelf S., 201, 17–28, https://doi.org/10.1016/j.ecss.2016.02.014, 2018.
Chapman, V. J. and Chapman, D. J.: Life forms in the algae, Bot. Mar., 19, 65–74, https://doi.org/10.1515/botm.1976.19.2.65, 1976.
Charlier, R. H., Morand, P., and Finkl, C. W.: How Brittany and Florida coasts cope with green tides, Int. J. Environ. Sci., 65, 191–208, https://doi.org/10.1080/00207230701791448, 2008.
Coelho, S. M., Scornet, D., Rousvoal, S., Peters, N. T., Dartevelle, L., Peters, A. F., and Cock, J. M.: Ectocarpus: A model organism for the brown algae, Cold Spring Harb. Protoc., 2, pdb.Emo065821, https://doi.org/10.1101/pdb.emo065821, 2012.
Costello, M. J., Claus, S., Dekeyzer, S., Vandepitte, L., Tuama, E. O., Lear, D., and Tyler-Walters, H.: Biological and ecological traits of marine species, PeerJ, 3, e1201, https://doi.org/10.7717/peerj.1201, 2015.
Costello, M. J., Bouchet, P., Boxshall, G., Arvanitidis, C., and Appeltans, W.: European Register of Marine Species, http://www.marbef.org/data/erms.php, last access: 29 April 2022.
Degen, R., Aune, M., Bluhm, B. A., Cassidy, C., Kędra, M., Kraan, C., Vandepitte, L., Włodarska-Kowalczuk, M., Zhulay, I., and Albano, P. G.: Trait-based approaches in rapidly changing ecosystems: A roadmap to the future polar oceans, Ecol. Indic., 91, 722–736, https://doi.org/10.1016/j.ecolind.2018.04.050, 2018.
Demes, K. W. and Graham, M. H.: Abiotic regulation of investment in sexual versus vegetative reproduction in the clonal kelp Laminaria Sinclairii (laminariales, Phaeophyceae), J. Phycol., 47, 463–470, https://doi.org/10.1111/j.1529-8817.2011.00981.x, 2011.
Destombe, C., Valero, M., Vernet, P., and, Couvet, D.: What controls haploid-diploid ratio in the red alga, Gracilaria verrucosa?, J. Evol. Biol., 2, 317–338, https://doi.org/10.1046/j.1420-9101.1989.2050317.x, 1989.
De Wreede, R. E. and Klinger, T.: Reproductive strategies in algae, in: Plant reproductive ecology: patterns and strategies, edited by: Doust, J. L. and Doust, J. J., Oxford University Press, New York, 267–284, ISBN 0-19-505175-0, 1988.
Díaz, S., Purvis, A., Cornelissen, J. H., Mace, G. M., Donoghue, M. J., Ewers, R. M., Jordano, P., and Pearse, W. D.: Functional traits, the phylogeny of function, and ecosystem service vulnerability, Ecol. Evol., 3, 2958–2975, https://doi.org/10.1002/ece3.601, 2013.
Engel, C., Åberg, P., Gaggiotti, O. E., Destombe, C., and Valero, M.: Population dynamics and stage structure in a haploid-diploid red seaweed, Gracilaria gracilis, J. Ecol., 436–450, https://doi.org/10.1046/j.1365-2745.2001.00567.x, 2001.
Esmaeili, Y. S., Corte, G. N., Checon, H. H., Bilatto, C. G., Lefcheck, J. S., Zacagnini Amaral, A. C., and Turra, A.: Revealing the drivers of taxonomic and functional diversity of nearshore fish assemblages: Implications for conservation priorities, Divers. Distrib., 28, 1597–1609, https://doi.org/10.1111/ddi.13453, 2022.
Feldmann, J.: Les types biologiques d'algues marines benthiques, B. Soc. Bot. Fr., 113, 45–60, https://doi.org/10.1080/00378941.1966.10838473, 1966.
Fowler-Walker, M. J., Wernberg, T., and Connell S. D.: Differences in kelp morphology between wave sheltered and exposed localities: morphologically plastic or fixed traits?, Mar. Biol., 148, 755–767, https://doi.org/10.1007/s00227-005-0125-z, 2006.
Garbary, D. J.: Harvesting Ascophyllum nodosum (Phaeophyceae) reduces the abundance of its host-specific epiphyte Vertebrata lanosa (Rhodophyta), Bot. Mar., 60, 297–301, https://doi.org/10.1515/bot-2016-0074, 2017.
Gaspar, R., Pereira, L., and Neto, J.M.: Intertidal zonation and latitudinal gradients on macroalgal assemblages: Species, functional groups and thallus morphology approaches, Ecol. Indic., 81, 90–103, https://doi.org/10.1016/j.ecolind.2017.05.060, 2017.
Gómez, I. and Huovinen, P.: Form and Function in Antarctic Seaweeds: Photobiological Adaptations, Zonation Patterns, and Ecosystem Feedbacks, in: Antarctic Seaweeds, edited by: Gómez, I. and Huovinen, P., Springer, Cham, 217–237, https://doi.org/10.1007/978-3-030-39448-6_11, 2020.
Gómez, I., Navarro, N. P., and Huovinen, P.: Bio-optical and physiological patterns in Antarctic seaweeds: a functional trait based approach to characterize vertical zonation, Prog. Oceanogr., 174, 17–27, https://doi.org/10.1016/j.pocean.2018.03.013, 2019.
Guiry, M. D.: How many species of algae are there?, J. Phycol., 48, 1057–1063, https://doi.org/10.1111/j.1529-8817.2012.01222.x, 2012.
Guiry, M. D. and Guiry, G. M.: AlgaeBase, https://www.algaebase.org/, last access: 2 May 2022.
Heesch, S., Serrano-Serrano, M., Barrera-Redondo, J., Luthringer, R., Peters, A. F., Destombe, C., Cock, J. M., Valero, M., Roze, D., Salamin, N., and Coelho, S. M.: Evolution of life cycles and reproductive traits: insights from the brown algae, J. Evol. Biol., 34, 992–1009, https://doi.org/10.1111/jeb.13880, 2021.
Hoshino, M., Hiruta, S. F., Croce, M. E., Kamiya, M., Jomori, T., Wakimoto, T., and Kogame, K.: Geographical parthenogenesis in the brown alga Scytosiphon lomentaria (Scytosiphonaceae): Sexuals in warm waters and parthenogens in cold waters, Mol. Ecol., 30, 5814–5830, https://doi.org/10.1111/mec.16152, 2021.
Kattge, J., Ogle, K., Bönisch, G., Díaz, S., Lavorel, S., Madin, J., Nadrowski, K., Nöllert, S., Sartor, K., and Wirth, C.: A generic structure for plant trait databases, Methods Ecol. Evol., 2, 202–213, https://doi.org/10.1111/j.2041-210X.2010.00067.x, 2011.
Kim, S., Choi, S. K., Van, S., Kim, S. T., Kang, Y. H., and Park, S. R.: Geographic differentiation of morphological characteristics in the brown seaweed Sargassum thunbergii along the Korean coast: A Response to Local Environmental Conditions, J. Mar. Sci. Eng., 10, 2–15, https://doi.org/10.3390/jmse10040549, 2022.
Lavaut, E., Guillemin, M.-L., Colin, S., Faure, A., Coudret, J., Destombe, C., and Valero, M.: Pollinators of the sea: A discovery of animal-mediated fertilization in seaweed, Science, 377, 528–530, https://doi.org/10.1126/science.abo6661, 2022.
Lawrence, E.: Henderson's dictionary of Biology, 13th edition, Pearson Education Limited, London, UK, ISBN 9780131273849, 2005.
Lincoln, R. J., Boxshall, G. A., and Clark, P. F.: Dictionary of ecology, evolution and systematics, 2nd ed, Cambridge, Cambridge University Press, ISBN 9780521438421, 1998.
Littler, M. M. and Littler, D. S.: The evolution of thallus form and survival strategies in benthic marine macroalgae: field and laboratory tests of functional form model, Am. Nat., 116, 25–44, https://doi.org/10.1086/283610, 1980.
Littler, M. M., Littler, D. S., and Taylor P. R.: Evolutionary strategies in a tropical barrier reef system: functionale from groups of marine macroalgae, J. Phycol., 19, 229–237, https://doi.org/10.1111/j.0022-3646.1983.00229.x, 1983.
Lüning, K.: Seaweeds: their environment, biogeography, and ecophysiology, John Wiley & Sons, https://doi.org/10.1002/aqc.327001020, 1991.
Nyberg, C. and Wallentinus, I.: Can species traits be used to predict macroalgal introductions?, Biol. Invasions, 7, 265–279, https://doi.org/10.1007/s10530-004-0738-z, 2005.
Maggs, C. A.: Intraspecific life history variability in the Florideophycidae (Rhodophyta), Bot. Mar., 31, 465–490, https://doi.org/10.1515/botm.1988.31.6.465, 1988.
Maggs, C. A. and Hommersand, M. H.: Seaweeds of the British Isles Volume 1 Rhodophyta Part 3A Ceramiales, The Natural History Museum, London, UK, ISBN 0113100450, 1993.
Marine Species Traits: Marine Species Traits, https://www.marinespecies.org/traits, last access: 25 August 2022.
MarLIN: BIOTIC – Biological Traits Information Catalogue, Marine Life Information Network, Plymouth, Marine Biological Association of the United Kingdom, https://www.marlin.ac.uk/biotic, last access: 31 August 2022, 2006.
Martini, S., Larras, F., Boyé, A., Faure, E., Aberle, N., Archambault, P., Bacouillard, L., Beisner, B.E., Bittner, L., Castella, E., Danger, M., Gauthier, O., Karp-Boss, L., Lombard, F., Maps, F., Stemmann, L., Thiébaut, E., Usseglio-Polatera, P., Vogt, M., Laviale, M., and Ayata, S.-D.: Functional trait-based approaches as a common framework for aquatic ecologists, Limnol. Oceanogr., 66, 965–994, https://doi.org/10.1002/lno.11655, 2021.
Mauffrey, A. R. L., Cappelatti, L., and Griffin, J. N.: Seaweed functional diversity revisited: confronting traditional groups with quantitative traits, J. Ecol., 3, 2390–2405, https://doi.org/10.1111/1365-2745.13460, 2020.
McGill, B. J., Enquist, B. J., Weiher, E., and Westoby, M.: Rebuilding community ecology from functional traits, Trends Ecol. Evol., 21, 178–185, https://doi.org/10.1016/j.tree.2006.02.002, 2006.
Murúa, P., Müller, D. G., Patiño, D. J., and Westermeier, R.: Giant kelp vegetative propagation: Adventitious holdfast elements rejuvenate senescent individuals of the Macrocystis pyrifera “integrifolia” ecomorph, J. Phycol., 53, 230–234, https://doi.org/10.1111/jpy.12493, 2017.
Oppliger, L. V., Von Dassow, P., Bouchemousse, S., Robuchon, M., Valero, M., Correa, J. A., Mauger, S., and Destombe, C.: Alteration of sexual reproduction and genetic diversity in the kelp species Laminaria digitata at the southern limit of its range, PLoS One, 9, e102518, https://doi.org/10.1371/journal.pone.0102518, 2014.
Orfanidis, S., Panayotidis, P., and Ugland, K. I.: Ecological Evaluation Index continuous formula (EEI-c) application: a step forward for functional groups, the formula and reference condition values, Mediterr. Mar. Sci., 12, 199–231, https://doi.org/10.12681/mms.60, 2011.
Pentecost, A.: Calcification in plants, in: International Review of Cytology, Academic Press, 62, 1–27, https://doi.org/10.1016/S0074-7696(08)61897-5, 1980.
Pierucci, A., De La Fuente, G., Cannas, R., and Chiantore, M.: A new record of the invasive seaweed Caulerpa cylindracea Sonder in the South Adriatic Sea, Heliyon, 5, e02449, https://doi.org/10.1016/j.heliyon.2019.e02449, 2019.
Quell, F., Schratzberger, M., Beauchard, O., Bruggeman, J., and Webb, T.: Biological trait profiles discriminate between native and non-indigenous marine invertebrates, Aquat. Invasions, 16, 571–600, https://doi.org/10.3391/ai.2021.16.4.01, 2021.
Ruuskanen, A., Bäck, S., and Reitalu, T.: A comparison of two cartographic exposure methods using Fucus vesiculosus as an indicator, Mar. Biol., 134, 139–145, https://doi.org/10.1007/s002270050532, 1999.
Ryznar, E. R., Fong, P., and Fong, C. R.: When form does not predict function: Empirical evidence violates functional form hypotheses for marine macroalgae, J. Ecol., 109, 833–846, https://doi.org/10.1111/1365-2745.13509, 2020.
SeaTraIn: SeaTraIn, https://seaweedtraits.github.io/, last access: 31 August 2022.
Serisawa, Y., Aoki, M., Hirata, T., Bellgrove, A., Kurashima, A., Tsuchiya, Y., Sato, T., Ueda, H., and Yokohama, Y.: Growth and survival rates of large-type sporophytes of Ecklonia cava transplanted to a growth environment with small-type sporophytes, J. Appl. Phycol., 15, 311–318, https://doi.org/10.1023/A:1025183100958, 2003.
Smetacek, V. and Zingone, A.: Green and golden seaweed tides on the rise, Nature, 504, 84–88, https://doi.org/10.1038/nature12860, 2013.
Spalding, M. D., Fox, H. E., Allen, G. R., Davidson, N., Ferdaña, Z. A., Finlayson, M. A. X., Halpern, B. S., Jorge, M. A., Lombana, A. L., Lourie, S. A., and Martin, K. D.: Marine ecoregions of the world: a bioregionalization of coastal and shelf areas, BioScience, 57, 573–583, https://doi.org/10.1641/B570707, 2007.
Steneck, R. S. and Dethier, M. N.: A functional group approach to the structure of algal-dominated communities, Oikos, 69, 476–498, https://doi.org/10.2307/3545860, 1994.
Stevenson, J.: Ecological assessments with algae: a review and synthesis, J. Phycol., 50, 437–461, https://doi.org/10.1111/jpy.12189, 2014.
Schleuning, M., Neuschulz, E. L., Albrecht, J., Bender, I. M., Bowler, D. E., Dehling, D. M., Fritz, S. A., Hof, C., Mueller, T., Nowak, L., and Sorensen, M. C.: Trait-based assessments of climate-change impacts on interacting species, Trends Ecol. Evol., 35, 319–328, https://doi.org/10.1016/j.tree.2019.12.010, 2020.
Vandepitte, L., Vanhoorne, B., Decock, W., Dekeyzer, S., Trias Verbeeck, A., Bovit, L., Hernandez, F., and Mees, J.: How Aphia – the platform behind several online and taxonomically oriented databases – can serve both the taxonomic community and the field of biodiversity informatics, J. Mar. Sci. Eng., 3, 1448–1473, https://hdl.handle.net/10.3390/jmse3041448, 2015.
Vandepitte, L., Vanhoorne, B., Decock, W., Vranken, S., Lanssens, T., Dekeyzer, S., Verfaille, K., Horton, T., Kroh, A., Hernandez, F., and Mees, J.: A decade of the World Register of Marine Species–General insights and experiences from the Data Management Team: Where are we, what have we learned and how can we continue?, PLoS One, 13, e0194599, https://doi.org/10.1371/journal.pone.0194599, 2018.
Veiga, P., Rubal, M., Vieira, R., Arenas, F., and Sousa-Pinto, I.: Spatial variability in intertidal macroalgal assemblages on the North Portuguese coast: consistence between species and functional group approaches, Helgoland Mar. Res., 67, 191–201, https://doi.org/10.1007/s10152-012-0315-2, 2013.
Vélez-Rubio, G. M., González-Etchebehere, L., Scarabino, F., Trinchin, R., Manta, G., Laporta, M., Zabaleta, M., Vidal, V., de Leon-Mackey, A., and Kruk, C.: Macroalgae morpho-functional groups in Southern marine ecosystems: rocky intertidal in the Southwestern Atlantic (33∘–35∘ S), Mar. Biol., 168, 1–21, https://doi.org/10.1007/s00227-021-03960-6, 2021.
Violle, C., Navas, M. L., Vile, D., Kazakou, E., Fortunel, C., Hummel, I., and Garnier, E.: Let the concept of trait be functional!, Oikos, 116, 882–892, https://doi.org/10.1111/j.0030-1299.2007.15559.x, 2007.
Voultsiadou, E., Gerovasileiou, V., Vandepitte, L., Ganias, K., and Arvanitidis, C.: Aristotle's scientific contributions to the classification, nomenclature and distribution of marine organisms, Mediterr. Mar. Sci., 18, 468–478, https://doi.org/10.12681/mms.13874, 2017.
Weiss, K. C. and Ray, C. A.: Unifying functional trait approaches to understand the assemblage of ecological communities: synthesizing taxonomic divides, Ecography, 42, 2012–2020, https://doi.org/10.1111/ecog.04387, 2019.
Wichard, T., Charrier, B., Mineur, F., Bothwell, J. H., Clerck, O. D., and Coates, J. C.: The green seaweed Ulva: a model system to study morphogenesis, Front. Plant Sci., 6, 72, https://doi.org/10.3389/fpls.2015.00072, 2015.
Wieters, E. A., McQuaid, C., Palomo, G., Pappalardo, P., and Navarrete, S. A.: Biogeographical boundaries, functional group structure and diversity of rocky shore communities along the Argentinean coast, PLoS One, 7, e49725, https://doi.org/10.1371/journal.pone.0049725, 2012.
Womersley, H. B. S.: The marine benthic flora of southern Australia. Part I: Chlorophyta, Woolman, Government Printer, Adelaide, South Australia, ISBN 0724345523, 1984.
Womersley, H. B. S.: The marine benthic flora of southern Australia, Part II, Australian Government Printing Division, Adelaide, South Australia, 481 pp., ISBN 072436501X, 1987.
WoRMS: World Register of Marine Species: https://www.marinespecies.org, last access: 25 August 2022.
The requested paper has a corresponding corrigendum published. Please read the corrigendum first before downloading the article.
- Article
(5859 KB) - Full-text XML
- Corrigendum
-
Supplement
(578 KB) - BibTeX
- EndNote