the Creative Commons Attribution 4.0 License.
the Creative Commons Attribution 4.0 License.
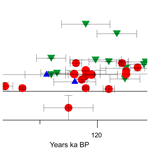
A standardized database of Marine Isotope Stage 5e sea-level proxies in southern Africa (Angola, Namibia and South Africa)
J. Andrew G. Cooper
Andrew N. Green
Evidence for sea-level change during and around Marine Isotope Stage (MIS) 5e (ca. 125 ka) in southern Africa derives from a wide variety of geomorphic and sedimentological sea-level indicators, supported in the past 2 decades by absolute chronological control, particularly on littoral deposits, some of which have a quantifiable relationship to former sea level. In addition to these proxies, data provided by both terrestrial (dune sediments and archaeological remains) and marine (lagoonal and nearshore littoral sediments) limiting points provide broad constraints on sea level. Here, we review publications describing such data points. Using the framework of the World Atlas of Last Interglacial Shorelines, we insert in a standardized database (https://doi.org/10.5281/zenodo.4459297, Cooper and Green, 2020) all the elements available to assess former palaeo-relative sea level (palaeo-RSL) and the chronological constraints associated with them (including uncertainties). Overall, we reviewed 71 studies, from which we extracted 39 sea-level indicators and 26 limiting points. As far as age attribution is concerned, early analysis of molluscs and whole-rock beachrock samples using U series allowed dating of several sea-level indicators during the 1980s, but the more widespread application of optically stimulated luminescence (OSL) dating since 2004 has yielded many more (and more accurate) sea-level indicators from several sites. This has helped resolve the nature and timing of MIS 5e shorelines and has the potential to further elucidate the apparent presence of two or more sea-level peaks at several South African sites during this interval. The standardized sea-level database presented in this paper is the first of its kind for this region. Future research should be directed to improve the stratigraphic description of last interglacial shorelines and to obtain better dating, high-accuracy elevation measurements with better palaeo-RSL interpretation.
- Article
(6122 KB) - Full-text XML
-
Supplement
(509 KB) - BibTeX
- EndNote
This paper aims to describe in detail the background information contained in the southern Africa Marine Isotope Stage (MIS) 5e sea-level database, which was compiled as a contribution to the World Atlas of Last Interglacial Shorelines (WALIS) ESSD special issue. The database was created using the WALIS interface, available at https://warmcoasts.eu/world-atlas.html (last access: 23 February 2021). The WALIS interface has been built following the lessons learned from the PALSEA (a PAGES–INQUA) working group in terms of sea-level databases, summarized in a recent paper by Düsterhus et al. (2016). In brief, the WALIS interface allows a large range of data and metadata on last interglacial relative sea-level indicators and associated ages to be inserted into a MySQL database. An export tool allows users to download their datasets as a multi-sheet XLS file. The database for southern Africa described in this study represents the output of the export tool mentioned above, it is open access (Cooper and Green, 2020, https://doi.org/10.5281/zenodo.4459297), and it is available at https://zenodo.org/record/4459297#.YAwp0MX7RQ8. Each field in our database is described by Rovere et al. (2020) at the following link: https://doi.org/10.5281/zenodo.3961544. The open access database will facilitate research on the global and regional patterns of sea-level change by the sea-level research community, including geophysical modellers, oceanographers, geologists and archaeologists.
To describe our database, and help the reader navigate through our choices in standardizing other authors' works, we first give an overview of the published literature in the region of interest (See Sect. 2). While not all the studies cited in this section contain enough data to be included in the database, they represent the historical background upon which new data were collected and include sites that provide the potential for further investigation with modern dating techniques. Then, we describe the types of sea-level indicators and elevation measurement techniques we encountered while compiling the database (see Sects. 3 and 4). In Sect. 5, we report details for each administrative province/region within the area of interest, where sea-level data were reviewed. In the final two sections, we discuss further details on other metadata on palaeo-sea-level indicators that are not included in our database but that might be useful as research on Quaternary shoreline progress in southern Africa. We discuss future research directions that may stem from this data compilation in Sect. 6.
Multiple elevated shorelines (some exceeding 100 m above sea level) occur around the coast of southern Africa (Angola, Namibia and South Africa). Many of these extend over large distances, and they have long been recognized and described in varying levels of detail (e.g. Krige, 1927; Haughton, 1931; Soares do Carvalho, 1961; Davies, 1970, 1972, 1973, 1980). These and other studies have enabled a compilation of South African sea-level data for the Plio–Pleistocene (Hearty et al., 2020). Many early descriptions of sedimentary evidence of former sea level are rather vague, with imprecise levelling, positioning and a lack of absolute (and even relative) dating control, but they drew attention to the presence and nature of many potential MIS 5e deposits. Geomorphological and occasional sedimentological description of these raised coastal deposits was often scarce or incomplete, and their relationship to former sea level was imprecisely defined. The issues regarding levelling are discussed below (Sect. 4), but in this overview, unless otherwise stated, elevations are expressed in relation to mean sea level (MSL).
Previous dating of higher-than-present shorelines in southern Africa came from the study of archaeological material with no fixed relationship to sea level. It could, however, be used to constrain the minimum age of coastal deposits. The presence of Acheulean (approx. 1.5 Ma–150 ka) tools in littoral deposits or on elevated marine terraces was frequently used to differentiate pre-last-interglacial shorelines. Davies (1980), for example, maintained that all elevated beach deposits above 4 m in Namibia were pre-MIS 5 because they contained Acheulean tools. The presence of stone tools of only the Sangoan culture (130–10 ka BP) was regarded as indicative of last interglacial shorelines: for example, on this basis Davies (1970) assigned a probable last interglacial age to a shoreline at ca. +9 m in KwaZulu-Natal, on the east coast of South Africa (Fig. 1). Archaeological investigations (e.g. Fisher et al., 2013) continue to identify sites that may hold evidence of former sea levels during former highstands.
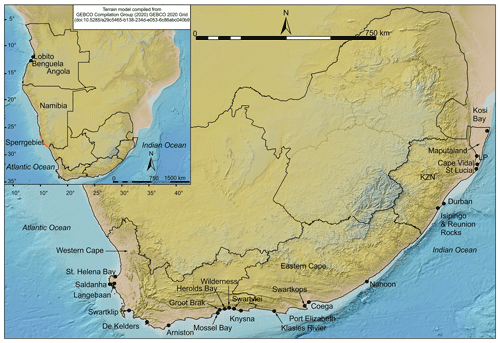
Figure 1(Inset) Locality Map of the Angolan, Namibian and South African coasts showing sites mentioned in the text. Main map shows details of sites in South Africa (KZN: KwaZulu-Natal province; LP: Lister's Point). Co-ordinates for each site are provided in the database.
The presence of Early Pleistocene and Tertiary animal macrofossils (e.g. Hendey, 1970; Kensley and Pether, 1986; Pether, 1986; Le Roux, 1990) provided the first absolute controls on some elevated shorelines, particularly in the Western Cape. These studies demonstrated that shorelines higher than ca. 10 m were Early Pleistocene or older (several high shorelines date to the Miocene and Pliocene). Le Roux (1990) used these macrofossils to correlate Neogene units (including shoreline deposits) around the entire Cape coast of South Africa.
Dating and identification of the relationship of potential last interglacial shorelines to former sea levels remained problematic and contentious through the 1970s to the 1990s. Tankard (1975a, b) noted the presence of shoreline deposits (open coast and lagoonal) associated with higher-than-present sea levels in the Western Cape (St Helena Bay area) (Fig. 1). By comparison with modern open-coast and estuarine deposits, the former sea level was calculated at +6.25 m (Tankard, 1975a), and by comparison with global occurrences of shorelines at this elevation, it was assigned a probable MIS 5e age. These deposits contained sub-fossil mollusc shells (in life position and transported) that included a cool-water open-coast assemblage and a lagoonal assemblage that included species currently confined to the tropics. These thermally anomalous molluscs (Tankard, 1975b) were taken as indicative of warmer waters during the last interglacial. Tankard (1975b) noted several sites between St Helena Bay and Knysna where these assemblages occurred in sediments associated with a former sea level of ca. +6 m (Fig. 1). In a regional review of Pleistocene shorelines, Davies (1970, 1971, 1972, 1980) presented a gazette of several potential last interglacial sites, both estuarine and open coast, in South Africa and Namibia where molluscs that are currently restricted to warmer waters of west and east Africa occur. These he termed the Swartkops fauna. Davies (1980) stated (p. 154) “In estuaries of the South Cape between Coega and Mossel Bay and apparently as far west as Arniston, beds occur with warm-water fauna at peak altitudes of +4 to +9 m. Some have probably been eroded, and all would have been laid down under water, so MSL would have been over +9 m”. A warm-water estuarine fauna from a +6–8 m estuarine terrace at Kosi Bay (Fig. 1) was also assigned to the last interglacial (Cooper et al., 1989). Subsequently, Le Roux (1990) reported that these warm-water mollusc species from estuarine/lagoonal facies were associated with the 6–8 m shoreline and are probably coincident with “the well-documented period of higher temperatures at c. 120 000 B.P. (Kilburn & Tankard, 1975).”
Several sedimentological and geomorphological observations suggested the presence of more than one last interglacial stillstand of sea level at South African sites. Hobday (1976) recognized three last interglacial shorelines from Lake St Lucia (+8, +3.4–5.3 and +4.5 m), and Tankard (1976) found three last interglacial shorelines around Saldanha Bay (+6.3, +2–3.5 and 0 m) (Fig. 1). At least the lowest of these three in each case may now be tentatively assigned a Late Holocene age (Cooper et al., 2018). Davies (1971, 1972, 1980) noted the repeated occurrence around the entire South African coast of a +6 m terrace incised into a +9 m terrace, implying two highstands separated by a regression. Barwis and Tankard (1983) also recorded two shorelines separated by a regression at Swartklip near Cape Town (Fig. 1). Cooper and Flores (1991) described the sedimentary facies of an outcrop at Isipingo near Durban (Fig. 1) and demonstrated that sediments from two former high sea levels were preserved between +5 and +6 m. Sedimentological work at Nahoon near East London (Jacobs and Roberts, 2009) (Fig. 1) also pointed to two sea-level highstands during the 5e interglacial, separated by a period of regression and dune building.
From the 1970s until early 2000s, in the absence of direct dating, it was widely accepted that the MIS 5e shoreline(s) in South Africa was associated with sea level in the approximate +6–8 m range (e.g. Hobday, 1976; Hobday and Jackson, 1979; Barwis and Tankard, 1983; Cooper and Flores, 1991). The lack of dating control beyond old (contaminated) C14 dates limited further comment on the time of deposition of these units.
Hendey and Cooke (1985) and Hendey and Volman (1986) mounted a challenge to this view after they found (on the basis of vertebrate fossil evidence) that deposits at Saldanha Bay associated with a +6–8 m shoreline (that had previously been correlated with MIS 5e) were actually of Early Pleistocene (1–1.5 Ma) age. Building on this, they then challenged the admittedly tenuous reported links between sea level and human occupation of important archaeological deposits in a cave at the Klasies River mouth (Fig. 1). This evidence, they maintained, supported an Early Pleistocene age for formation of the +6–8 m erosional terrace in the cave. They asserted that occupation of the cave (supported by U Th dates of 98 and 110 ka) was contemporaneous with formation of a lower (supposed MIS 5e) erosional terrace associated with a +4 m sea level. Based on observations at this and two other cave sites (at De Kelders and Herolds Bay, Fig. 1), they contended that the +6–8 m shoreline in South Africa was Early Pleistocene and the MIS 5e sea level did not exceed +4 m. Subsequent OSL dating (see below) shows that their contention, based on universal extrapolation from a few sites, was incorrect.
Barwis and Tankard (1983) reported undated observations of shoreline deposits related to two closely spaced highstands at Swartklip, near Cape Town. The sedimentary deposits were interpreted as beachrock capped by calcrete, topped by estuarine sediments and washover fans. These in turn were overlain by aeolian dunes. The washover deposits were tentatively linked to a 135 ka sea-level high and have been widely cited as examples of last interglacial shorelines. Subsequent sedimentological and fossil analysis (John Pether, personal communication, 2020), however, reveals that the estuarine and washover deposits represent inter-dune wetlands and aeolian deposits, respectively, and that both form part of a major MIS 7 aeolian dune deposit (Roberts et al., 2009). MIS 5 shorelines are, however, represented in the immediate surroundings by marine erosional surfaces and littoral deposits that require further study (John Pether, personal communication, 2020).
Apart from several old and contaminated radiocarbon dates, no absolute dates existed for supposed MIS 5e shoreline deposits in South Africa until Davies (1980, p. 162) reported the results of amino acid racemization dating of molluscs from three sites near Port Elizabeth (Fig. 1). The results, although inconclusive, suggested that two shells from a deposit at +6.5 m but “contemporary with the +8 m beds upstream” in the Swartkops estuary “may be 130 000 B.P. or perhaps in the range 160 000–220 000”. The first published compilation of late Quaternary to recent sea-level data (Ramsay and Cooper, 2002) included only four dates from the late Quaternary, all of them based on uranium series dating. Since then, detailed studies in the Wilderness and Mossel Bay areas of the Western Cape (Fig. 1) (Carr et al., 2010; Bateman et al., 2008, 2011) and the Maputaland coastal plain in KwaZulu-Natal (Porat and Botha, 2008) applied OSL dating to aeolianite and occasional littoral facies to investigate the timing of major coastal barrier and dune-building episodes. These were subsequently extended by offshore investigations that applied optically stimulated luminescence (OSL and, in one instance, paired OSL and U series) dating to submerged deposits (Bosman, 2012; Cawthra et al., 2018, 2019, 2020). Data from these studies are reported below in a regional report of data availability.
In southern Namibia, diamond mining provided the impetus for investigation of former shoreline deposits both onshore and offshore (e.g. Spaggiari et al., 2006; Spaggiari, 2011; Runds, 2017; Runds et al., 2019; Kirkpatrick et al., 2019). The several higher-than-present “beaches” of the Sperrgebiet, or forbidden zone (Fig. 1), rest on erosional terraces and were labelled A–F (with increasing elevation) by Corvinus (1983) (Fig. 2). This terminology has since been widely applied. Shorelines D, E and F range in bedrock platform height from +10 to +30 m a.m.s.l. (Fig. 2). They contain a warm-water marine zone fossil Donax rogersi and are Tertiary in age (SACS, 1980; Apollus, 1995; Jacob, 2001; Roberts and Brink, 2002). Shorelines A, B and C are characterized by modern cold-water faunas, particularly the infaunal bivalve Donax serra (Pickford and Senut, 2000; Pether, 2000; Jacob, 2001; Miller, 2008). These littoral deposits truncate calcrete sandstones and underlying sands bearing the fossil Equus capensis and Acheulean artefacts and are therefore younger than Middle Pleistocene (Pickford and Senut, 2000). Fossil and Middle Stone Age archaeological remains suggest that these lower shorelines (A–C) are younger than 200 000 years. The supposed Middle Pleistocene (C beach) is located at +8 m, and the Late Pleistocene (MIS 5e) (B beach) is at +4 m (Hallam, 1964; Corvinus, 1983; Pether, 1986; Schneider and Miller, 1992; Ward, 2000; Pether et al., 2000). The A beach at +2–3 m is likely of Holocene age, but no absolute dating control has been established on shorelines A, B or C.
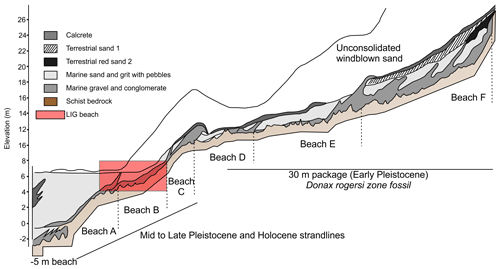
Figure 2Schematic east–west cross section through the raised beach deposits of southern Namibia (modified from Runds, 2017). Subtidal to +10 m elevations represent the A, B and C beaches, and the +30 m package represents the D, E and F beaches). Data were compiled originally from Hallam (1964) and Jacob (2001). Beach “B” is commonly ascribed to MIS 5e but has not been dated definitively.
In Angola, Soares do Carvalho (1961) first identified several marine terraces at various elevations above present sea level. These had littoral deposits resting upon them, and as in Namibia, terraces and overlying deposits were considered to be broadly coeval. Giresse at al. (1984) reported U-series dates on a number of these terraces at elevations between 0 and 55 m near Benguela and Lobito (Fig. 1). Of these, three dates, deemed by the authors to be acceptable, were in the MIS 5 range. Mollusc samples from a +10–12 m terrace dated to 91 and 136 ka, and those from a +20 m terrace dated to 103 ka. These provided little additional insight into the chronology of the Angolan shorelines, which remained enigmatic. Walker et al. (2016) identified a widespread terrace at +25 m elevation that dated to 45 ka (based on eight OSL dates). This demonstrated Late Pleistocene dynamic uplift of the Angolan coast by ca. 300 m (ca. 2 mm/yr) via a mantle done with diameter of ca. 1000 km, centred on Benguela. This finding of substantial tectonic deformation along the Angolan coast effectively requires a reappraisal of the ages and elevations of all shorelines in Angola, which has yet to be undertaken.
We note that our database contains information on palaeo-relative sea levels. The relative term highlights the fact that every palaeo-sea level we report is uncorrected for potential post-depositional uplift or subsidence due to, for example, tectonics or glacial isostatic adjustment. Although the South African coast is regarded as tectonically stable during the Quaternary, the potential impact of neotectonics on raised shoreline elevation has also been raised in northwestern South Africa (Namaqualand) and Namibia. Roberts and Brink (2002) reported deformation of Miocene and Pliocene shorelines on the NW coast of South Africa involving ca. 50 m vertical displacement. Raised beaches in the southern Sperrgebiet (southern Namibia, Fig. 1) said to be of Plio–Pleistocene age appear to diminish in altitude from south to north (Stocken, 1978; Dingle et al., 1983) and may imply recent deformation. Pickford and Senut (2000) note, however, that some of the Sperrgebiet shorelines may be as old as Miocene and the appearance of tilting could be an artefact.
In reviewing existing studies, we identified several types of last interglacial sea-level indicators in the region (Table 1). All are sedimentological (based on distinctive sedimentary facies that are diagnostic of particular marine, coastal and terrestrial environments), while one (beachrock) has an additional diagenetic component. Their indicative meaning (i.e. the relationship between the elevation of the indicator and the palaeo-relative sea level it represents) can be inferred by comparison with modern equivalents within certain error limits (Shennan et al., 2015; Rovere et al., 2016), but this was not always undertaken in the original study. In such instances, in the database we have sought to retrofit this interpretation to the reported observations. Several other data points are simply limiting dates. These occur an unquantifiable distance above (terrestrial limiting) or below (marine limiting) sea level. Most of these included in the dataset are derived from aeolianite that accumulated above (Mean High Water) (MHW).
Table 1Sea-level indicators used in this study and their relationship to sea level at the time of deposition. MHHW: mean higher high water; MLLW: mean lower low water (chart datum); MLW: mean low water.
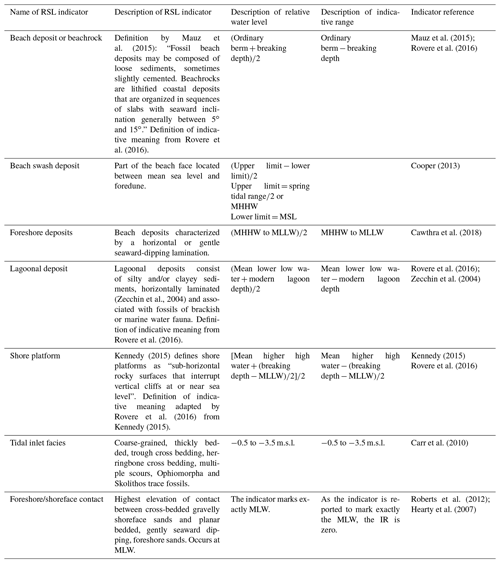
The most widespread sea-level indicators in southern Africa are shore platforms (Kennedy, 2015; Rovere, 2016) that sometimes have associated littoral deposits resting upon them. Although they can be related to former sea levels by comparison with modern regional equivalents (Smith et al., 2010; Dixon et al., 2015; Cooper and Green, 2016), few of these documented occurrences have been dated. Notable exceptions are the raised beaches overlying shore platforms in Angola (Walker et al., 2016) that proved not to be of MIS 5 age but which establish the utility of this sea-level indicator.
Contemporary tidal inlet (Cooper, 1990, 2001) and foreshore facies (Smith et al., 2010) extend over a vertical range of a few metres on the microtidal (ca. 2 m) and high-energy coast of southern Africa, and no systematic report of their relationship to contemporary sea-level datums exists. Consequently, the former sea level associated with these deposits can only be constrained to within a few metres through comparison with their modern equivalents (Carr et al., 2010; Cawthra et al., 2018). Identification of swash zone sediments can potentially provide somewhat better constraint on former sea level (Cooper, 2014) as the swash zone typically extends from the low water mark to the beach berm. However, wave run-up on beaches depends on many factors including the beach slope and grain size and can be significantly higher during storms. Wave run-up on South African beaches ranged from 2 to 9 m during a storm in 2007 (Mather et al., 2010).
Following Hearty et al. (2007), the contact between shoreface and foreshore sediments was used as a sea-level indicator in South Africa by Roberts et al. (2012) and Cawthra et al. (2018). This occurs in outcrop as a planar, conformable contact between cross-bedded gravelly sands (shoreface) and planar bedded, gently dipping sands (foreshore) and is correlated with mean low tide.
Beachrock is defined by a unique combination of sedimentary texture and cement (Vousdoukas et al., 2007; Mauz et al., 2015). The distinctive bedding (near-horizontal plane lamination, symmetrical ripples, and/or planar and trough cross beds) derives from deposition on the lower intertidal beach and the cement is diagnostic of cementation in the intertidal zone. Several generations of cement can be present, and these may reflect changes in porewater chemistry that result from changes in sea level and beach morphology (Kelly et al., 2014; Wiles et al., 2018). Careful identification of particular beachrock sub-facies can provide sub-metre constraints on former sea level (Mauz et al., 2015).
Many South African estuaries and lagoons contain brackish water back-barrier sediments with distinctive molluscan faunas, of which several are known to exist in a quantifiable relationship to sea level (Kilburn and Rippey, 1982). No indicators of this type, have, however, been reported for MIS 5 in the study area. Sediments in contemporary back-barrier locations extend from MHW to a maximum of −3 m, although during fluvial floods, water levels can extend to 3–4 m higher (Cooper et al., 1990). In South African perched lagoons (Cooper, 2001), which lack a surface connection to the ocean for extended periods, the enclosed water level and associated sediments may reach higher levels (seldom > 1 m above MHW). No systematic morphological measurements are available for southern African back-barrier systems (Harrison et al., 2000).
The database also includes some broad indicators that simply record whether sea level was higher or lower than the dated sample. These include terrestrial limiting dates set by aeolianites (now-cemented aeolian dunes that formed by wind action on dry land) or marine limiting dates set by undifferentiated shoreface sediments that formed at an unknown depth below mean low water in the marine environment.
The reviewed studies report elevations measured by either barometric altimeter (limited to early studies), levelling, or echo-sounding (multibeam echo-sounding in more recent studies) or do not report the elevation measurement method. As a consequence, the sea-level datum to which the data are referred is usually not reported but has been assumed to be mean sea level. The spring tidal range around southern Africa is generally less than 2 m, but more precise recording and reporting of the relationship of former littoral deposits to contemporary sea level (and preferably a fixed datum with a known relationship to modern sea level) are desirable in future studies in order to reduce vertical uncertainties. Roberts et al. (2012) present a model for future investigations in which all elevations are reported to orthometric zero, which is linked in turn to land levelling datum and the WGS84 horizontal and vertical datums. Measurements were undertaken using total station and/or differential GPS with a reported vertical measurement error of ±1.5 cm.
The sea-level information reviewed for South Africa was extracted from sources that are described hereafter, with details reported in the Supplement annexed to this paper, as exported from the WALIS data insertion. All site names are the same as those reported in the database.
The database includes 54 data points from stratigraphy. Chronological control is based on luminescence dating (49 data points), luminescence dating (12 data points), and amino acid racemization (AAR) (1 data point), and 1 data point relies on chronostratigraphic control. The highest reported accuracy is associated with the luminescence dates, whereas the AAR data point is extremely uncertain. Elevations cited in the following text are stated in relation to MSL unless there is explicit information to relate them to another datum.
5.1 Western Cape
In the Western Cape, important work on last interglacial shorelines has been conducted by Carr et al. (2010), who provided a detailed analysis from sites at three locations (Swartvlei, Great Brak estuary and Cape Agulhas) on the south coast (Fig. 1). This involved several OSL dates for sea-level indicators (tidal inlet, beach berm and foreshore) and several terrestrial limiting data from overlying terrestrial dune (aeolianite) deposits. Collectively, these define a sequence of sea-level indicators recording transgression to a peak of ca. +8.5 m at ca. 127 ka followed by regression. At Swartvlei and Great Brak tidal inlet facies overlain by shoreface or aeolian facies indicate a highstand 6.0–8.5 m above modern sea level. At Cape Agulhas, a gravel beach (ca. 3.8 m a.m.s.l.) and an overlying sandy shoreface facies (up to 7.5 m a.m.s.l.) were deposited between 138 ± 7 ka and 118 ± 7 ka. The highstand was followed by accumulation of aeolian dunes (dating to 122 ± 7 ka and 113 ± 6 ka).
Cawthra et al. (2014) broadly confirmed these observations but identified a second sea-level peak from the Great Brak River sequence based on a lower foreshore deposit overlain by aeolianite in a regressive succession, which is in turn overlain by a higher foreshore deposit heralding renewed transgression. The two foreshore units separated by aeolianite suggest two sea-level highstands separated by regression. The second sea-level peak is represented by an ∼2 m thick coarse sandy tabular deposit with an orthometric height of 6.8 m a.m.s.l. It was not dated but is younger than the underlying foreshore sediments (111.2 ± 7 ka).
In a study primarily of MIS 11 deposits at Dana Bay, Roberts et al. (2012) also described and dated a regressive MIS 5 sequence comprising shoreface, foreshore and aeolian units. The shoreface–foreshore contact, marked by a transition from cross-bedded gravelly sand to gently seaward dipping planar bedded sands, was invoked as a palaeo-shoreline indicator as this contact occurs in contemporary beaches at mean low water. Using this approach in conjunction with detailed and accurate levelling tied to fixed absolute levels, Roberts et al. (2012) established the former low-tide level at +5.2 m. MSL is 1 m higher. Sea-level index points were derived by OSL dating of the foreshore sands (125 ± 9 and 116 ± 9 ka), and a limiting date (125 ± 9 ka) was set by the overlying aeolianite.
At Langebaan several dated samples yielded a largely concordant series of thermoluminescence (TL), infrared stimulated thermoluminescence (IRSL) and U-series ages. The hominid footprints horizon was dated to ∼ 108 ka, (corrected to ∼117 ka based on global sea-level curves and the conformable contact with the underlying strata) (Roberts, 2008). A discordant (older) TL date was attributed to incomplete bleaching of quartz grains (Roberts and Berger, 1997). The Langebaan footprints were interpreted to date from initial regression from the younger of two MIS 5e highstands identified at Nahoon (see below) at ∼ 120 ka (Roberts, 2008).
5.2 Eastern Cape
Jacobs and Roberts (2009) undertook a detailed study to clarify apparently conflicting ages of hominid footprint-bearing aeolianite at Nahoon (Fig. 3). They dated aeolianite and associated beach facies, reporting limiting ages for the aeolianite and an index point for the beach facies. The analysis also revealed the presence of two MIS 5e beaches, only the younger of which was OSL dated (117 ± 6 ka). Roberts (2008) noted “already fully lithified footprint-bearing [a]eolianites of the Nahoon Formation in the area had been planed off and gullied by a later sea level which rose to ∼ 6 m a.m.s.l. This suggested a Marine Isotope Stage 5e (MIS 5e) event. The Nahoon Formation [a]eolianites rest on shallow marine deposits at ∼ 1 m a.m.s.l., representing an older sea-level highstand. Thus the geological evidence from Nahoon points to two highstands, an older event at ∼ 2 m a.m.s.l. and a younger counterpart at ∼ 6 m a.m.s.l.” Subsequent sedimentary analysis (Morrissey et al., 2020, p. 1) delivered an alternative interpretation in which two phases of aeolianite deposition were separated by “a single stepped sea-level transgression phase up to +7.82 ± 0.82 m a.s.l., evidenced by intraformational beachrock facies and marine benches”. No further dates were provided.
At Blind River, East London, Wang et al. (2008) provide two OSL dates on “estuarine calcarenite” that is conformably overlain by a “storm beach gravel” between +8 and +10 m m.s.l. The stratigraphically conformable dates from the estuarine deposit (119 ± 9 and 118 ± 7 ka) were from +5 and +6.5 m, respectively.
Although many potential MIS 5 deposits have been reported from the open coast and estuaries of the Eastern Cape, the only other dated evidence comes from sites at Coffee Bay (Fig. 1), where an oyster shell from a beach whose base is at +4.5 m yielded a U Th age of 104.9 ± 9 ka (Ramsay and Cooper, 2002)
5.3 KwaZulu-Natal
Porat and Botha (2008), in a comprehensive study of dune development on the Maputaland coastal plain, established a chronology for the many aeolian depositional units. This enabled a reappraisal of the regional stratigraphy (Botha, 2018). They, however, recorded only one MIS 5e date (132.7 ka, MP-22) from the crest of a 50 m high coastal dune at Cape Vidal (Fig. 1), which gave no clear indication of the associated sea level. A cluster of U Th ages from 95.7 to 117 ka (Ramsay et al., 1993), however, derived from marine shells, beachrock and an elephant tusk associated with a solution pothole on an erosional terrace cut into aeolianite near Durban, identify a sea level at about +6 m. This was correlated with the second of two supposed (but undated) MIS 5 shorelines described at adjacent sites at Isipingo by Cooper and Flores (1991). Similarly, supposed last interglacial shoreline deposits were described from adjacent sites at Durban (Cawthra et al., 2012). At Phinda Game Reserve near St Lucia (Fig. 1), an oyster shell in a palaeo-shoreline yielded a U Th age of 95 ± 4 ka (Ramsay and Cooper, 2002). Several beachrock and aeolianite outcrops on the continental shelf (Ramsay, 1994; Bosman, 2012) have yielded sea-level index points and limiting dates on either side of MIS 5e (Table 1).
5.4 Namibia
The geological and sedimentological literature on the Namibian coastal deposits is quite extensive, but none of the literature surveyed meets the minimum database standards for MIS 5e sea-level quantification. The potential 5e sites reported in the literature lack absolute dating control; to our knowledge, and despite the extent of diamond mining from raised shorelines north (downdrift) of the Orange River, no MIS 5e dates have been presented for raised shorelines in Namibia. It has been supposed on molluscan fossil evidence that a shoreline complex at +8 m (the C beach of Corvinus, 1983) is of Middle Pleistocene and a +4 m (B beach) is of last interglacial age. These deposits require further investigation, especially to establish their ages.
5.5 Angola
Early U-series dates presented by Giresse et al. (1984) included three potential MS5e ages for raised shorelines at +12 and +20 m. These require reappraisal, however, in the light of the subsequent presentation of multiple OSL dates for a +25 m shoreline dating to 45 ka (Walker et al., 2016), which implies large-scale Quaternary uplift along the Angolan coast. The Giresse et al. (1984) data are included in the database, but their reliability and actual significance is uncertain.
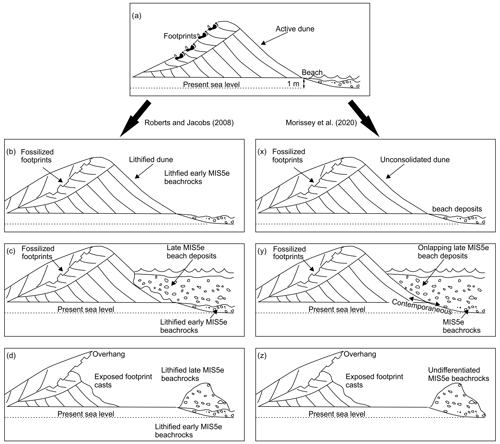
Figure 3Diagrammatic sequence of depositional events and sea-level change associated with recorded hominid footprints on a fossil dune surface at Nahoon, East London. Two interpretations of the sequence are compared. In each, the starting point (a) is the same, but in the interpretation of Jacobs and Roberts (2009) (b–d), the footprints are buried by further aeolian sedimentation, and the (undated) but supposed early MIS 5e dune/beach succession is lithified (b), indicating that sea level had fallen by an unknown magnitude below the present level. In (c) a later (higher) MIS 5e highstand (to about ±6 m) partly erodes the earlier MIS 5e marine and aeolian deposits, and in panel (d) further erosion of the MIS 5e dune/beach succession occurs during the Holocene highstand, resulting in the development of an overhang, the underside of which bears the (natural) casts of the footprints. In the interpretation (x–z) of Morrissey et al. (2020), littoral sedimentation deposits an onlapping and conformable littoral unit during a continuously rising MIS 5e sea level.
6.1 Last interglacial sea-level fluctuations
The collated data from South Africa for the period 140–80 ka BP are shown in Fig. 4 superimposed on a continuous record from the Red Sea to provide temporal context. Data from 90 to 140 ka are shown in detail in Fig. 5. The record is largely internally consistent, although some OSL dates on submerged aeolianites plot well under the sea level inferred by emergent sea-level indicators. These may be the result of ex situ sampling of loose boulders on the seabed. Carr et al. (2010) presented the earliest dated point for sea level above present ca. 138 ka. Data from Cawthra et al. (2018) suggest a subsequent period of lower-than-present sea levels around 130 ka. Carr et al. (2010) provide several data points for an MIS 5e sea-level highstand of ca. +8 m centred on ca. 125 ka, and there is a cluster of dates for a highstand between 115 and 120 ka. Taken together, however, the collated regional data are inconclusive regarding the presence of more than one sea-level peak during MIS 5e (Fig. 5); the paucity of data points and overlaps in the age ranges preclude a definitive statement. In the lack of full dating control it is not possible to argue for the presence (Hearty et al., 2007) or absence (Mauz et al., 2018) of two sea-level peaks in MIS 5e.
6.2 Other interglacials
Tertiary and Early Pleistocene shoreline deposits are widely developed on the east and west coasts of South Africa, Namibia and Angola. These were recently reviewed by Hearty et al. (2020) in the context of global climate change.
Well-preserved littoral deposits of the prolonged MIS 11 highstand containing diagnostic sea-level information were described by Roberts et al. (2012) from near Mossel Bay in the Western Cape. These dated to ∼ 390 ka and yielded a precise maximum elevation of +14 m (correction for minor crustal uplift, and glacial isostatic adjustment (GIA) indicated eustatic sea level of +13 m ± 2 m). The sequence also revealed sea-level fluctuations during MIS 11, including an early highstand close to present sea level.
In both the Western Cape and KwaZulu-Natal, coastal dunes of MIS 7 have been dated, but no sea-level indicators have been reported. Ramsay and Cooper (2002) reported a U Th age of 182 ± 18 ka (Pta-U430) from aeolianite from Reunion Rocks near Durban, which overlaps with an OSL date of 203 ± 13 ka (MP-33) from the same location reported by Porat and Botha (2008). Bateman et al. (2004) similarly recorded three OSL dates in the range of 176–283 ka from a landward dune ridge at Wilderness (Shfd02132, 02133 and 02134). The widespread occurrence of aeolianites that yield MIS 7 OSL dates in the Western Cape has been highlighted by Roberts et al. (2009, 2014).
6.3 Holocene sea-level indicators
Shorelines associated with a Holocene sea-level highstand are well developed around the coast of South Africa (Ramsay, 1996; Compton, 2001) and Namibia (Compton, 2006). A recent regional review (Cooper et al., 2018) identified a Middle Holocene highstand of +2 to +4 m between 7.3 and 6 ka BP, with potential Late Holocene oscillations of < 1 m amplitude around the present sea level in the subsequent interval.
6.4 Controversies
The past controversy around the age of the +6–8 m shoreline, created by Hendey and Volman (1986), was an artefact of limited chronological control and a lack of detailed sedimentological analysis and reporting. OSL dating has since proved that last interglacial shorelines do extend between +6 and +8 m. Their work does, however, point to the need for caution in making broad generalizations about the age of shorelines at specific elevations, and it is clear that shoreline deposits of various ages can occur at similar levels if the right conditions exist for preservation. The widely cited Swartklip section (Barwis and Tankard, 1983) does not contain MIS 5 sea-level indicators and is now established as dating to MIS 7 (Roberts et al., 2014). The question of potential tectonism in Angola and Namibia is a relatively recent one. The evidence of uplift of Quaternary shorelines presented by Walker et al. (2016) from central Angola is persuasive, but the spatial and temporal extent of tectonic influence on raised shorelines on the coast of Angola, Namibia and possibly Namaqualand in NW South Africa requires further investigation. De Beer (2012), for example, provided evidence of Plio–Pleistocene reactivation of Mesozoic faults in Namaqualand, possibly as recently as the latest Pleistocene. This was attributed to local seismogenic activity rather than regional uplift.
6.5 Uncertainties and data quality
The data reported are subject to a variety of uncertainties related to the original elevation and associated datum. In few studies are these described adequately. In addition, regional data on the relationship of contemporary sedimentary facies and geomorphic units to any tidal datum are lacking. This adds to the uncertainty in determining the indicative meaning of the sedimentary facies.
Elevation and datum uncertainties in the sampled material must be considered, especially in pre-2004 studies (the advent of OSL dating). Very many studies do not explicitly report the elevation measurement technique or the measurement error. In the database, these errors have been estimated via the authors' own experience. In most instances, elevation measurements are not reported to a specified datum, whether chart datum (marine) or ordnance datum (terrestrial), or a tidal level. This reduces the vertical resolution of most data points, which have been referred to MSL in the database.
Palaeo-RSL calculations from modern analogues rely on detailed knowledge of contemporary coastal environments and associated sedimentary facies. Southern Africa has a high-energy, wave-dominated coast within which distinctive sedimentary facies have a large vertical range. Our quantification of modern analogues is based on a combined analysis of the global literature on sea-level indicators (Rovere et al., 2016), especially on high-energy coasts (e.g. Cooper, 2013; Mauz et al., 2015), descriptions of the contemporary South African littoral geomorphology and sedimentology (Smith et al., 2010; Bond et al., 2013; Kelly et al., 2014; Dixon et al., 2015; Cooper and Green, 2016; Wiles et al., 2018; Green et al., 2019), and our own experience. More direct measurement (especially in relation to a fixed datum) and reporting of the distribution and variability of ranges of open-coast sedimentary facies in the region would help constrain comparisons with modern analogues. Tidal-inlet-associated units are particularly difficult to constrain because of the marked variability in geomorphology of estuaries and their inlets around the South African coast (Cooper, 2001) and the paucity of direct measurements of inlet depth. The ranges listed in the database are based on the authors' own experience.
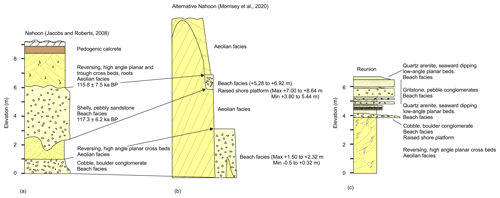
Figure 6Key sites in South Africa with sedimentary but not fully dated records of sea-level variability during MIS 5e. Locations on Fig. 1. (a) Sequence at Nahoon (after Jacobs and Roberts, 2009). A similar lowermost beach facies represents a possible early MIS 5e highstand at 1 m, with a regression represented by the aeolian facies, followed by a second transgression. (b) Alternative interpretation of the Nahoon sequence (from Morrissey et al., 2020), in which the two beach units are regarded as coeval. (c) An undated sequence of littoral sediments overlying an aeolianite at Reunion, Durban, adjacent to the site at Isipingo described by Cooper and Flores (1991). The lowermost beach facies overlies the platform from which Ramsay et al. (1993) described an elephant tusk found in a solution pothole. The sequence holds the potential to investigate the relationship between platform formation and beach deposition in relation to former sea levels.
Uncertainties related to dating refer mainly to U-series dates. These are sometimes at odds with other dating methods and suggest issues with the source material (not from closed systems, for example). OSL dating, in contrast, seems to have given more consistent results, although even here there are some inconsistencies between aeolianite and other dates. The OSL dates are, however, on sandy sediments from the high-energy coast where vertical uncertainties (see above) are comparatively high. Many of the U-series dates are from estuarine sediments where vertical uncertainties on sea-level indicators could potentially be better constrained due to the lower-energy conditions in which they accumulate and the specific vertical ranges of certain organisms.
A mandatory evaluation of data quality is included with each RSL data point. This was undertaken following the WALIS guidelines (Rovere et al., 2020).
The southern Africa database (Cooper and Green, 2020, https://doi.org/10.5281/zenodo.4459297) is open access. The files at this link were exported from the WALIS database interface on 23 January 2021. A description of each field in the database is contained at https://doi.org/10.5281/zenodo.4459297, which is readily accessible and searchable at https://walis-help.readthedocs.io/en/latest/ (Rovere, 2021a). More information on the World Atlas of Last Interglacial Shorelines can be found at https://warmcoasts.eu/world-atlas.html (Rovere, 2021b). Users of our database are encouraged to cite the original sources alongside with our database and this article.
Our newly compiled database provides a means to investigate the record of sea-level variability around southern Africa and to identify data gaps and precise questions for further investigation. In this regard, and in light of recent developments in dating, several sites that were reported in earlier studies as likely MIS 5e shorelines merit further investigation as they may help refine the detailed pattern of sea-level change during that interval. Sites with particularly well preserved littoral sedimentary facies that record sea-level variability merit fresh investigation. Particularly high-priority sites (Fig. 6) include the poorly age constrained sites at Isipingo and Reunion (KwaZulu-Natal) (Cooper and Flores, 1991) and Nahoon Point (Eastern Cape), where two contrasting interpretations have been presented (Jacobs and Roberts, 2009; Morrissey et al., 2020) (Fig. 3). The added potential to date submerged littoral sediments, as has been carried out at several sites in South Africa, holds the possibility of elucidating the timing and magnitude of sea-level fluctuations between sea-level highstands.
In addition, the widespread palaeo-lagoonal/estuarine deposits that occur in many estuaries from KwaZulu-Natal to the Western Cape that have been noted (Davies, 1970, 1971, 1972, 1980; Cooper, 1996, 1999) but little investigated, remain a valuable resource for detailed sea -level reconstruction around the MIS 5e interglacial. In particular, the Lister's Point outcrop in Lake St Lucia (KwaZulu-Natal) (Fig. 1), which has been the subject of controversy due to poorly resolved stratigraphy, is worthy of further detailed investigation. Part of the confusion (and unnecessarily strongly held opinions) that surrounds the site arises from poorly detailed stratigraphical investigations. Cooper et al. (2013) established that at least 5 highstand deposits are preserved, including two separate coral-bearing units, the uppermost of which is probably from the last interglacial. The site is also adjacent to other deposits that have yielded U Th ages suggestive of MIS 5e, although they are somewhat younger (Ramsay and Cooper, 2002).
The supplement related to this article is available online at: https://doi.org/10.5194/essd-13-953-2021-supplement.
JAGC and ANG both reviewed the literature, compiled the data, and discussed and assigned indicative meanings. Both wrote and edited the manuscript, designed and prepared the figures, and addressed referee comments.
The authors declare that they have no conflict of interest.
This article is part of the special issue “WALIS – the World Atlas of Last Interglacial Shorelines”. It is not associated with a conference.
The data used in this study were compiled in WALIS, a sea-level database interface developed by the ERC Starting Grant WARMCOASTS (ERC-StG-802414), in collaboration with the PALSEA (PAGES–INQUA) working group. The database structure was designed by Alessio Rovere, Deirdre Ryan, Thomas Lorscheid, Andrea Dutton, Peter Chutcharavan, Dominik Brill, Nathan Jankowski, Daniela Mueller, Melanie Bartz, Evan Gowan and Kim Cohen. The data points used in this study were compiled and contributed to WALIS by Andrew Cooper and Andrew Green. We are grateful to Alessio Rovere for guidance and assistance in the preparation of this paper. Reviews by Hayley Cawthra and Natasha Barlow and comments by Barbara Mauz greatly helped in preparation of the final paper. John Pether kindly provided comments and unpublished observations on the Swartklip and adjacent sections.
This paper was edited by Alessio Rovere and reviewed by Natasha Barlow and one anonymous referee.
Apollus, L.: The distribution of diamonds on a late Cainozoic gravel beach, southwestern Namibia, MS thesis, unpublished, Department of Geology and Applied Geology, University of Glasgow, Glasgow, United Kingdom, 170 pp., 1995.
Barwis, J. H. and Tankard, A. J.: Pleistocene shoreline deposition and sea-level history at Swartklip, South Africa, J. Sediment. Res.,, 53, 1281–1294, 1983.
Bateman, M. D., Holmes, P. J., Carr, A. S., Horton, B. P., and Jaiswal, M. K.: Aeolianite and barrier dune construction spanning the last two glacial–interglacial cycles from the southern Cape coast, South Africa, Quaternary Sci. Rev., 23, 1681–1698, 2004.
Bateman, M. D., Carr, A. S., Murray-Wallace, C. V., Roberts, D. L., and Holmes, P. J.: A dating intercomparison study on Late Stone Age coastal midden deposits, South Africa, Geoarchaeology, 23, 715–741, 2008.
Bateman, M. D., Carr, A. S., Dunajko, A. C., Holmes, P. J., Roberts, D. L., McLaren, S. J., Bryant, R. G., Marker, M. E., and Murray-Wallace, C. V.: The evolution of coastal barrier systems: a case study of the Middle-Late Pleistocene Wilderness barriers, South Africa, Quaternary Sci. Rev., 30, 63–81, 2011.
Bond, J., Green, A. N., Cooper, J. A. G., and Humphries, M. S.: Seasonal and episodic variability in the morphodynamics of an ephemeral inlet, Zinkwazi Estuary, South Africa, J. Coastal Res., 65, 446–451, 2013.
Bosman, C.: The marine geology of the Aliwal Shoal, Scottburgh, South Africa, PhD Thesis, unpublished, University of KwaZulu-Natal, Durban, South Africa, 2012.
Botha, G. A.: Lithostratigraphy of the late Cenozoic Maputaland Group, S. Afr. J. Geol., 121, 95–108, 2018.
Carr, A. S., Bateman, M. D., Roberts, D. L., Murray-Wallace, C. V., Jacobs, Z., and Holmes, P. J.: The last interglacial sea-level high stand on the southern Cape coastline of South Africa, Quaternary Res., 73, 351–363, 2010.
Cawthra, H. C., Uken, R., and Ovechkina, M. N.: New insights into the geological evolution of the Durban Bluff and adjacent Blood Reef, South Africa, S. Afr. J. Geol., 115, 291–308, 2012.
Cawthra, H. C., Bateman, M. D., Carr, A. S., Compton, J. S., and Holmes, P. J.: Understanding Late Quaternary change at the land–ocean interface: a synthesis of the evolution of the Wilderness coastline, South Africa, Quaternary Sci. Rev., 99, 210–223, 2014.
Cawthra, H. C., Jacobs, Z., Compton, J. S., Fisher, E. C., Karkanas, P., and Marean, C. W.: Depositional and sea-level history from MIS 6 (Termination II) to MIS 3 on the southern continental shelf of South Africa, Quaternary Sci. Rev., 181, 156–172, 2018.
Cawthra, H. C., Anderson, R. J., De Vynck, J. C., Jacobs, Z., Jerardino, A., Kyriacou, K., and Marean, C. W.: Migration of Pleistocene shorelines across the Palaeo-Agulhas Plain: Evidence from dated sub-bottom profiles and archaeological shellfish assemblages, Quaternary Sci. Rev., 235, 106107, 2019.
Cawthra, H. C., Frenzel, P., Hahn, A., Compton, J. S., Gander, L., and Zabel, M.: Seismic stratigraphy of the inner to mid Agulhas Bank, South Africa, Quaternary Sci. Rev. 235, 105979, https://doi.org/10.1016/j.quascirev.2019.105979, 2020.
Compton, J. S.: Holocene sea-level fluctuations inferred from the evolution of depositional environments of the southern Langebaan Lagoon salt marsh, South Africa, Holocene, 11, 395–405, 2001.
Compton, J. S.: The mid-Holocene sea-level highstand at Bogenfels Pan on the southwest coast of Namibia, Quaternary Res., 66, 303–310, 2006.
Cooper, J. A. G.: Ephemeral stream-mouth bars at flood-breach river mouths on a wave-dominated coast: Comparison with ebb-tidal deltas at barrier inlets, Mar. Geol., 95, 57–70, 1990.
Cooper, J. A. G.: Geomorphological variability among microtidal estuaries from the wave-dominated South African coast, Geomorphology, 40, 99–122, 2001.
Cooper, J. A. G.: Sedimentary indicators of relative sea-level change–high energy, Encyclopedia of Quaternary Science, 4, 385–395, 2013.
Cooper, J. A. G. and Green, A. N.: Geomorphology and preservation potential of coastal and submerged aeolianite: examples from KwaZulu-Natal, South Africa, Geomorphology, 271, 1–12, 2016.
Cooper, J. A. G., and Green, A. N.: Database of Last Interglacial sea levels in Angola, Namibia and South Africa, Zenodo, https://doi.org/10.5281/zenodo.4459297, 2020.
Cooper, J. A. G. and Flores, R. M.: Shoreline deposits and diagenesis resulting from two Late Pleistocene highstands near +5 and +6 metres, Durban, South Africa, Mar. Geol., 97, 325–343, 1991.
Cooper, J. A. G, Kilburn, R. N., and Kyle, R.: A late Pleistocene molluscan assemblage from Lake Nhlange, Zululand, and its palaeoenvironmental implications, S. Afr. J. Geol., 92, 73–83, 1989.
Cooper, J. A. G., Mason, T. R., Reddering, J. S. V., and Illenberger, W. K.: Geomorphological effects of catastrophic flooding on a small subtropical estuary, Earth Surf. Proc. Land., 15, 25–41, 1990.
Cooper, J. A. G., Green, A. N., and Smith, A. M.: Vertical stacking of multiple highstand shoreline deposits from the Cretaceous to the present: facies development and preservation, J. Coastal Res., 65, 1904–1908, 2013.
Cooper, J. A. G., Green, A. N., and Compton, J. S.: Sea-level change in southern Africa since the Last Glacial Maximum, Quaternary Sci. Rev., 201, 303–318, 2018.
Cooper, M. R.: The Cainozoic palaeontology and stratigraphy of KwaZulu-Natal: Part 1. The Mtunzini Formation. Stratigraphy and fauna, Durban Museum Novitates, 21, 1–10, 1996.
Cooper, M. R.: The Cainozoic palaeontology and stratigraphy of KwaZulu-Natal. Part 3. The Mduku Formation. Stratigraphy and fauna, Durban Museum Novitates, 24, 48–56, 1999.
Cooper, M. R.: The Cainozoic palaeontology and stratigraphy of KwaZulu-Natal. Part 5. The False Bay coral limestone Formation. Stratigraphy and fauna, Durban Museum Novitates, 37, 7–24, 2014.
Corvinus, G.: The raised beaches of the west coast of South West Africa/Namibia: an interpretation of their archaeological and palaeontological data, C.H. Beck, Munich, 108 pp., 1983.
Davies, O.: Pleistocene beaches of Natal, Annals of the Natal Museum, 20, 403–442, 1970.
Davies, O.: Pleistocene Shorelines in the Southern and South-eastern Cape Province (Part 1), Annals of the Natal Museum, 21, 181–223, 1971.
Davies, O.: Pleistocene shorelines in the southern and south-eastern Cape Province (Part 2), Annals of the Natal Museum, 21, 225–279, 1972.
Davies, O.: Pleistocene shorelines in the southwestern Cape and South-West Africa, Annals of the Natal Museum, 21, 719–765, 1973.
Davies, O.: Last interglacial shorelines in the South Cape, Palaeontographica Africa, 23, 153–171, 1980.
De Beer, C. H.: Evidence of Neogene to Quaternary faulting and seismogenic deformation along the Namaqualand coast, South Africa, S. Afr. J. Geol., 115, 117–136, 2012.
Dixon, S., Green, A., and Cooper, J. A. G.: Storm swash deposition on an embayed rock coastline: Facies, formative mechanisms, and preservation, J. Sediment. Res., 85, 1155–1165, 2015.
Dingle, R. V., Siesser, W. G., and Newton, A. R.: Mesozoic and Tertiary Geology of southern Africa, A.A. Balkema, Rotterdam, 1983.
Düsterhus, A., Rovere, A., Carlson, A. E., Horton, B. P., Klemann, V., Tarasov, L., Barlow, N. L. M., Bradwell, T., Clark, J., Dutton, A., Gehrels, W. R., Hibbert, F. D., Hijma, M. P., Khan, N., Kopp, R. E., Sivan, D., and Törnqvist, T. E.: Palaeo-sea-level and palaeo-ice-sheet databases: problems, strategies, and perspectives, Clim. Past, 12, 911–921, https://doi.org/10.5194/cp-12-911-2016, 2016.
Fisher, E. C., Albert, R. M., Botha, G., Cawthra, H. C., Esteban, I., Harris, J., Jacobs, Z., Jerardino, A., Marean, C. W., Neumann, F. H., and Pargeter, J.: Archaeological reconnaissance for middle stone age sites along the Pondoland Coast, South Africa, PaleoAnthropology, 104–137, 2013.
Giresse, P., Hoang, C. T., and Kouyoumontzakis, G.: Analysis of vertical movements deduced from a geochronological study of marine Pleistocene deposits, southern coast of Angola, J. Afr. Earth Sci., 2, 177–187, 1984.
Grant, K. M., Rohling, E. J., Ramsey, C. B., Cheng, H., Edwards, R. L., Florindo, F., Heslop, D., Marra, F., Roberts, A. P., Tamisiea, M. E., and Williams, F.: Sea-level variability over five glacial cycles, Nat. Commun., 5, 1–9, 2014.
Green, A. N., Pillay, T., Cooper, J. A. G., and Guisado-Pintado, E.: Overwash-dominated stratigraphy of barriers with intermittent inlets, Earth Surf. Proc. Land., 44, 2097–2111, 2019.
Hallam, C. D.: The geology of the coastal diamond deposits of southern Africa, in: The Geology of Some Ore Deposits in Southern Africa, edited by: Haughton, S. H., Vol. 2, Geological Society of South Africa, 671–729, 1964.
Harrison, T. D., Cooper, J. A. G., and Ramm, A. E. L.: State of South African Estuaries. Department of Environmental Affairs and Tourism, State of the Environment Series, 2. Pretoria, South Africa, 127 pp., 2000.
Haughton, S. H.: Late Tertiary and recent deposits of the west coast of South Africa, Trans. Geol. Soc. S. Afr., 34, 19–57, 1931.
Hearty, P. J., Hollin, J. T., Neumann, A. C., O'Leary, M. J., and McCulloch, M.: Global sea-level fluctuations during the Last Interglaciation (MIS 5e), Quaternary Sci. Rev., 26, 2090–2112, 2007.
Hearty, P. J., Rovere, A., Sandstrom, M. R., O'Leary, M. J., Roberts, D., and Raymo, M. E.: Pliocene-Pleistocene stratigraphy and sea-level estimates, Republic of South Africa with implications for a 400 ppmv CO2 world, Paleoceanogr. Paleocl., 35, e2019PA003835, https://doi.org/10.1029/2019PA003835, 2020.
Hendey, Q. B.: The age of the fossiliferous deposits at Langebaanweg, Cape Province, Annals of the South African Museum, 56, 119–131, 1970.
Hendey, Q. B. and Cooke, H. B. S.: Kolpocheorus paiceae (Mammalia, Suidae) from Skurwerug, near Saldanha, South Africa, and its palaeoenvironmental implications, Annals of the South African Museum, 97, 9–56, 1985.
Hendey, Q. and Volman, T.: Last interglacial sea levels and coastal caves in the Cape Province, South Africa, Quaternary Res., 25, 189–198, 1986.
Hobday, D. K.: Quaternary sedimentation and development of the lagoonal complex, Lake St Lucia, Zululand, Annals of the South African Museum, 71, 93–113, 1976.
Hobday, D. K. and Jackson, M. P. A.: Transgressive shore zone sedimentation and syndepositional deformation in the Pleistocene of Zululand, South Africa, J. Sediment. Res., 49, 145–158, 1979.
Jacob, J.: Late Proterozoic bedrock geology and its influence on Neogene littoral marine diamondiferous trap sites, MA1–Sperrgebiet, Namibia, MS, thesis, unpublished, Department of Geological Science, University of Cape Town, 140 pp., 2001.
Jacobs, Z. and Roberts, D. L.: Last Interglacial Age for aeolian and marine deposits and the Nahoon fossil human footprints, Southeast Coast of South Africa, Quat. Geochronol., 4, 160–169, 2009.
Kensley, B. and Pether, J.: Late Tertiary and Early Quaternary fossil mollusca of the Hondeklip Area, Cape Province, South Africa, Annals of the South African Museum, 97, 141–225, 1986.
Kelly, C. S., Green, A. N., Cooper, J. A. G., and Wiles, E.: Beachrock facies variability and sea level implications: a preliminary study, J. Coastal Res., 70, 736–742, 2014.
Kennedy, D. M.: Where is the seaward edge? A review and definition of shore platform morphology, Earth-Sci. Rev., 147, 99–108, 2015.
Krige, A. V.: Examination of the Tertiary and Quaternary changes of sea-level in South Africa, Ann. Univ. Stellenbosch, 5, 81 pp., 1927.
Kilburn, R. and Rippey, E.: Sea shells of southern Africa, MacMillan, Cape Town, 1982.
Kilburn, R. N. and Tankard, A. J.: Pleistocene molluscs from the west and south coasts of the Cape Province, South Africa, Annals of the South African Museum, 67, 183–226, 1975.
Kirkpatrick, L., Jacob, J., and Green, A. N.: Beaches and bedrock: How geological framework controls coastal morphology and the relative grade of a Southern Namibian diamond placer deposit, Ore Geol. Rev., 107, 853–862, 2019.
Le Roux, F. G.: Palaeontological correlation of Cenozoic marine deposits of the southeastern, southern and western coasts, Cape Province, South African J. Geol., 93, 514–518, 1990.
Pether, J.: Late Tertiary and Early Quaternary marine deposits of the Namaqualand coast, Cape Province: new perspectives, S. Afr. J. Sci., 82, 464–470, 1986.
Pether, J., Roberts, D. L., and Ward, J. D.: Deposits of the west coast, Oxford Monographs on Geology and Geophysics, 40, 33–54, 2000.
Mather, A. A., Stretch, D., and Garland, G.: Wave runup on natural beaches, Coast Eng. Proc., 32, 1–6, 2010.
Mauz, B., Vacchi, M., Green, A., Hoffmann, G., and Cooper, J. A. G.: Beachrock: a tool for reconstructing relative sea level in the far-field, Mar. Geol., 362, 1–16, 2015.
Mauz, B., Shen, Z., Elmejdoub, N., and Spada, G.: No evidence from the eastern Mediterranean for a MIS 5e double peak sea-level highstand, Quaternary Res., 89, 505–510, 2018.
Miller, R. M.: The Geology of Namibia, Vol. 1, Ministry of Mines and Energy, Geological Survey, Windhoek, 2008.
Morrissey, P., Knight, J., and Stratford, D. J.: Early Marine Isotope Stage 5 sea levels, coastal dune palaeoenvironments, and human occupation on the southeast coast of South Africa, Quaternary Sci. Rev., 245, 106504, https://doi.org/10.1016/j.quascirev.2020.106504, 2020.
Pickford, M. and Senut, B.: Geology and Palaeobiology of the Central and Southern Namib Desert, Southwestern Africa: Geology and History of Study, 18, Geological Survey of Namibia, Windhoek, 2000.
Porat, N. and Botha, G. A.: The luminescence chronology of dune development on the Maputaland coastal plain, southeast Africa, Quaternary Sci. Rev., 27, 1024–1046, 2008.
Ramsay, P. J.: Marine geology of the Sodwana Bay shelf, southeast Africa, Mar. Geol., 120, 225–247, 1994.
Ramsay, P. J.: 9000 years of sea-level change along the southern African coastline, Quatern. Int., 31, 71–75, 1996.
Ramsay, P. J. and Cooper, J. A. G.: Late Quaternary sea-level change in South Africa, Quaternary Res., 57, 82–90, 2002.
Ramsay, P. J., Smith. A. M., Lee-Thorp, J. C., Vogel, J. C., Tyldsley, M., and Kidwell, W.: 130,000 year-old fossil elephant found near Durban: Preliminary report, S. Afr. J. Sci., 89, 165–166, 1993.
Roberts, D. and Berger, L. R.: Last interglacial (c. 117 kyr) human footprints from South Africa, S. Afr. J. Sci., 93, 349–350, 1997.
Roberts, D. L.: Last interglacial hominid and associated vertebrate fossil trackways in coastal eolianites, South Africa, Ichnos, 15, 190–207, 2008.
Roberts, D. L. and Brink, J. S.: Dating and correlation of Neogene coastal deposits in the Western Cape (South Africa): Implications for Neotectonism, S. Afr. J. Geol., 105, 337–352, 2002.
Roberts, D. L., Bateman, M. D., Murray-Wallace, C. V., Carr, A. S., and Holmes, P. J.: West coast dune plumes: climate driven contrasts in dunefield morphogenesis along the western and southern South African coasts, Palaeogeogr. Palaeocl., 271, 24–38, 2009.
Roberts, D. L., Karkanas, P., Jacobs, Z., Marean, C. W., and Roberts, R. G.: Melting ice sheets 400,000 yr ago raised sea level by 13 m: Past analogue for future trends, Earth Planet. Sc. Lett., 357, 226–237, 2012.
Roberts, D., Cawthra, H., and Musekiwa, C.: Dynamics of late Cenozoic aeolian deposition along the South African coast: a record of evolving climate and ecosystems, Geological Society, London, Special Publications 388, 353–387, 2014.
Rovere, A.: Welcome to WALIS, available at: https://walis-help.readthedocs.io/en/latest/, last access: 1 March 2021a.
Rovere, A.: The World Atlas of Last interglacial shorelines (WALIS), available at: https://warmcoasts.eu/world-atlas.html, last access: 1 March 2021b.
Rovere, A., Raymo, M. E., Vacchi, M., Lorscheid, T., Stocchi, P., Gomez-Pujol, L., Harris, D. L., Casella, E., O'Leary, M. J., and Hearty, P. J.: The analysis of Last Interglacial (MIS 5e) relative sea-level indicators: Reconstructing sea-level in a warmer world, Earth-Sci. Rev., 159, 404–427, 2016.
Rovere, A., Ryan, D., Murray-Wallace, C., Simms, A., Vacchi, M., Dutton, A., Lorscheid, T., Chutcharavan, P., Brill, D., Bartz, M., Jankowski, N., Mueller, D., Cohen, K., and Gowan, E.: Descriptions of database fields for the World Atlas of Last Interglacial Shorelines (WALIS), Zenodo, https://doi.org/10.5281/zenodo.3961544, 2020.
Runds, M. J.: Sedimentology and Depositional Environment of a Marine Target, Southern Namibia: 3D Stratigraphic Architecture and Diamond Mineralisation Potential, PhD thesis, unpublished, University of Cape Town, 2017.
Runds, M. J., Bordy, E. M., and Pether, J.: Late Quaternary sedimentological history of a submerged gravel barrier beach complex, southern Namibia, Geo-Mar. Lett., 39, 469–491, 2019.
SACS (South African Committee for Stratigraphy): Stratigraphy of Southern Africa. Part 1. Lithostratigraphy of South Africa, South West Africa/Namibia, and the Republics of Boputhatswana, Transkei, and Venda, Geological Survey of South Africa Handbook, 8, p. 690, 1980.
Schneider, G. I. C. and Miller, R. McG.: Diamonds, in: The Mineral Resources of Namibia, Ministry of Mines and Energy, Windhoek, Namibia, 5.1-1–5.1-32, 1992.
Shennan, I., Long, A. J., and Horton, B. P. (Eds.): Handbook of Sea-Level Research, John Wiley & Sons, Chichester, 2015.
Smith, A. M., Mather, A. A., Bundy, S. C., Cooper, J. A. G., Guastella, L. A., Ramsay, P. J., and Theron, A.: Contrasting styles of swell-driven coastal erosion: examples from KwaZulu-Natal, South Africa, Geol. Mag., 147, 940–953, 2010.
Soares do Carvalho, G.: Alguna problemas dos terracos quaternaries de littoral de Angola, Bol. Serv. Geol. Min. Angola, 2, 5–15, 1961.
Spaggiari, R. I.: Sedimentology of Plio-Pleistocene Gravel Barrier Deposits in the Palaeo-Orange River Mouth, Namibia: Depositional History and Diamond Mineralisation, PhD Thesis, unpublished, Rhodes University, Grahamstown, 2011.
Spaggiari, R. I., Bluck, B. J., and Ward, J. D.: Characteristics of diamondiferous Plio-Pleistocene littoral deposits within the palaeo-Orange River mouth, Namibia, Ore Geol. Rev., 28, 475–492, 2006.
Stocken, C. G.: A review of the Later Mesozoic and Cenozoic deposits of the Sperrgebiet, COM Geological Department internal report, Namdeb Diamond Corporation (Pty) Ltd., Oranjemund, Namibia, 33 pp., 1978.
Tankard, A. J. T.: Late Cenozoic history and palaeoenvironments of the south-western Cape province, South Africa, PhD thesis, unpublished, Rhodes University, Grahamstown, 1975a.
Tankard, A. J. T.: Thermally anomalous Late Pleistocene molluscs from the south-western Cape Province, South Africa, Annals of the South African Museum, 69, 17–45, 1975b.
Tankard, A. J. T.: Pleistocene history and coastal morphology of the Ysterfontein-Elands Bay area, Cape Province, Annals of the South African Museum, 69, 73–119, 1976.
Vousdoukas, M. I., Velegrakis, A. F., and Plomaritis, T. A.: Beachrock occurrence, characteristics, formation mechanisms and impacts, Earth-Sci. Rev., 85, 23–46, 2007.
Walker, R. T., Telfer, M., Kahle, R. L., Dee, M. W., Kahle, B., Schwenninger, J. L., Sloan, R. A., and Watts, A. B.: Rapid mantle-driven uplift along the Angolan margin in the late Quaternary, Nat. Geosci., 9, 909–914, 2016.
Wang, Q., Tobias, P., Roberts, D., and Jacobs, Z.: A re-examination of a human femur found at the Blind River site, East London, South Africa: Its age, morphology, and breakage pattern, Anthropol. Rev., 71, 43–61, 2008.
Ward, J. D.: Deposits of the Namibian Coast, in: The Cenozoic of Southern Africa, in: Oxford Monographs on Geology and Geophysics, edited by: Partridge, T. C. and Maud, R. R., vol. 40, 46–54, 2000.
Wiles, E., Green, A. N., and Cooper, J. A. G.: Rapid beachrock cementation on a South African beach: Linking morphodynamics and cement style, Sediment. Geol., 378, 13–18, 2018.
Zecchin, M., Nalin, R., and Roda, C.: Raised Pleistocene marine terraces of the Crotone peninsula (Calabria, southern Italy): facies analysis and organization of their deposits, Sediment. Geol., 172, 165–185, 2004.
- Abstract
- Introduction
- Literature overview
- Sea-level indicators
- Elevation measurements
- Overview of data points inserted in the database
- Further details
- Data availability
- Future research directions
- Author contributions
- Competing interests
- Special issue statement
- Acknowledgements
- Review statement
- References
- Supplement
- Abstract
- Introduction
- Literature overview
- Sea-level indicators
- Elevation measurements
- Overview of data points inserted in the database
- Further details
- Data availability
- Future research directions
- Author contributions
- Competing interests
- Special issue statement
- Acknowledgements
- Review statement
- References
- Supplement