the Creative Commons Attribution 4.0 License.
the Creative Commons Attribution 4.0 License.
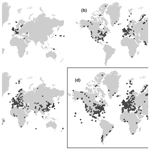
A worldwide meta-analysis (1977–2020) of sediment core dating using fallout radionuclides including 137Cs and 210Pbxs
Anthony Foucher
Pierre-Alexis Chaboche
Pierre Sabatier
Olivier Evrard
Dating recent sediment archives (< 150 years) constitutes a prerequisite for environmental and climatic reconstructions. Radiocaesium (137Cs) emitted during thermonuclear bomb testing (∼ 1950–1980) and nuclear accidents and the decrease in excess lead-210 (210Pbxs) with depth are often combined to establish sediment core chronology. Although these methods have been widely used during the last several decades, there is a lack of structured and comprehensive worldwide synthesis of fallout radionuclide analyses used for dating sediment cores in environmental and Earth sciences. The current literature overview was based on the compilation of 573 articles published between 1977 and 2020, reporting the collection of 1351 individual dating sediment cores (the dataset can be accessed at https://doi.org/10.1594/PANGAEA.931493; Foucher et al., 2021). This review was conducted in order to map the locations where 137Cs fallout events were detected. These included the thermonuclear bomb testing peak in 1963, the Chernobyl accident in 1986, the Fukushima accident in 2011, and 24 additional events identified at 112 sites that led to local or regional radioactive releases (e.g., Sellafield accidents, Chinese nuclear tests). When 210Pbxs records were used along with 137Cs data, detailed information on the 210Pbxs age–depth models were also synthesized.
With the current growing number of studies analyzing sediment cores and the increasing interest in the deployment of sediment fingerprinting techniques including radionuclides as potential discriminant properties, this spatialized synthesis provides a unique worldwide compilation for characterizing fallout radionuclide sources and levels at the global scale. This synthesis provides in particular a reference of 137Cs peak attribution for improving the sediment core dating, and it outlines the main questions that deserve attention in future research as well as the regions where additional 137Cs fallout investigations should be conducted in priority.
- Article
(4407 KB) - Full-text XML
-
Supplement
(396 KB) - BibTeX
- EndNote
Sedimentary sequences have received a growing interest as a support for conducting climatic and environmental reconstructions covering the 20th century period, which has been highly impacted by socio-environmental changes (Syvitski et al., 2020). These natural archives provide a powerful and unique tool for reconstructing the trajectory, the magnitude and the resilience of terrestrial and aquatic ecosystems facing major environmental changes, climate forcing or contamination pressures (Dearing and Jones, 2003; Jaegler et al., 2019; Sabatier et al., 2014). These recent paleo-reconstructions were carried out in various environments such as lakes, alluvial plains, lagoons, estuaries or even the open ocean. Establishing an age–depth model is the first prerequisite of any paleo-investigation based on these sediment archives. Fallout of short-lived radionuclides (caesium-137, lead-210) characterized by different half-lives () is particularly useful to derive this crucial temporal information.
Among these radionuclides, two complementary radioisotopes are classically analyzed. Caesium-137 (137Cs) is used as a discrete time marker, which provides anchoring points after 1950. In contrast, the excess of lead-210 (210Pbxs) is used as a continuous time marker to reconstruct sedimentation rates for the last 100 to 150 years (e.g., Oldfield et al., 1978; Sanchez-Cabeza et al., 2000).
The 137Cs is an artificial radionuclide (.15 years) produced by the global thermonuclear weapon tests that started in 1954 and whose fallout peaked in 1963 in the Northern Hemisphere. Furthermore, additional 137Cs releases occurred in certain regions of the world (i.e., mainly in Europe and in the Northern Hemisphere; Appleby et al., 1991) following the Chernobyl (1986) and the Fukushima Daiichi (2011) nuclear accidents. Other more local or regional sources of 137Cs releases have also been recorded. Local sources are generally found along river systems (and the radionuclides remain generally disseminated within a few dozens or hundreds of kilometers from the source), whereas the regional sources can be detected in zones situated at several thousands of kilometers from the source due to atmospheric fallout. The radioactive releases from the Mayak weapon production plant (Russia, 1949, 1957) and the Sellafield accidents (UK, 1957, 1974, 1981) provide examples of these local and regional sources, respectively (e.g., Madsen et al., 2005). To identify unambiguous 137Cs sources, complementary analyses of plutonium isotope signatures (Meusburger et al., 2020) or the detection of additional radionuclides including americium-241 (241Am) can be conducted (Appleby et al., 1991). The use and limitations of 137Cs for dating sediment sequences have been widely described in previous publications (Delaval et al., 2020; Klaminder et al., 2012; Miller and Heit, 1986).
In contrast to 137Cs, 210Pb is a natural radionuclide (.3 years), which is a decay product of the 238U chain ( years). 238U decays through a series of nuclides (e.g., 226Ra, 222Rn). 222Rn ( d) is a gas that partly remains in situ, forming “supported” 210Pb and that partly escapes to the atmosphere, forming “excess” 210Pb (210Pbxs) when falling back on the soil surface as a result of wet and dry fallout. The evolution of 210Pbxs activities with depth in sediment cores follows an exponential decrease from which it is possible to calculate the sedimentation rates for the last 100–150 years. Several dating models were established in the literature in order to infer sediment ages from 210Pbxs (Appleby and Oldfield, 1978; Bruel and Sabatier, 2020; Goldberg, 1963; Sanchez-Cabeza and Ruiz-Fernández, 2012), and several limitations to this method were also described (e.g., Binford, 1990). Sediment core inventories are often reported for both radionuclides to compare the study location with a reference site. The “reference” core must be chosen not to be affected by post-depositional disturbance processes (e.g., erosion, accumulation) and must be located in the vicinity of the studied sediment core. Comparing the radionuclide inventories calculated in the sediment cores of interest with that found at a nearby reference site may provide information on sediment dynamics (e.g., through the calculation of erosion or accumulation rates).
Although both 137Cs and 210Pbxs activities were largely used to assign ages (often in years) to the succession of layers in sedimentary sequences, there is a lack of structured and comprehensive worldwide synthesis of radionuclide fallout. Accordingly, the current literature overview was based on a Web of Science search with the objectives to (1) identify the worldwide distribution of studies using these radionuclides for sediment dating, (2) identify the presence of discrete peaks of 137Cs in these archives (attributed to global and regional fallout or to local sources) and the ages and activities associated with these peaks, and (3) characterize the 210Pbxs models used for dating sediment archives when they were applied in combination with 137Cs.
The overall goal of this synthesis was to build a worldwide reference and make it available to the scientific community sharing interests in sediment core dating or sediment source fingerprinting. It will also raise questions that should deserve attention in future research.
Journal articles using 137Cs for dating sediment cores published in English were extracted from the Thomson Reuters Web of Science database until 29 February 2020. The search words “137Cs” and “sediment core” were used in combination. A total of 910 publications were found by the automatic literature searching tool starting from 1977. Soil science studies focusing on the depth distribution of 137Cs in soils (Jagercikova et al., 2014) and publications reporting 137Cs measurements conducted on surface soil layers or deploying sediment fingerprinting approaches using 137Cs as a diagnostic property (Evrard et al., 2020) were excluded from further analysis and removed from the database.
Among the remaining references, 573 publications were selected to feed a database including a total of 1351 individual dated sediment cores (Fig. 1) – (the database is freely downloadable from https://doi.org/10.1594/PANGAEA.931493).
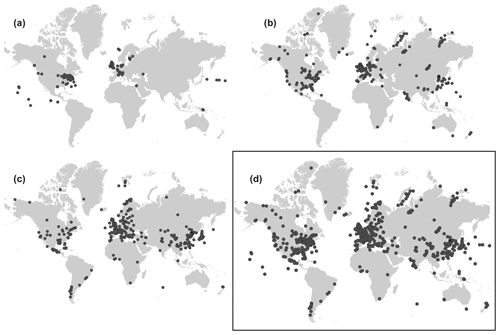
Figure 1Location of the sediment cores included in the database. (a) Sediment cores described in articles published between 1977 and 1990, (b) between 1990 and 2005, and (c) between 2005 and 2020. (d) Full database of sediment cores compiled in the current research.
The database includes the following categories of information:
-
publication metadata (site name, author information, DOI or permanent link, journal title, publication year, main processes studied, GPS coordinates, country)
-
sampling information (sampling year, core length, coring environment – e.g., lacustrine, coastal, floodplain, corer type, corer diameter, subsampling increment, sediment compaction measured during the core collection)
-
catchment and lake characteristics (name, size, main land use in the drainage catchment, slope, elevation range)
-
description of the 137Cs-related properties (surface activity; activity and sediment depth of the Fukushima, Chernobyl and 1963 peak fallout; total 137Cs inventory in the core; 137Cs inventory at a nearby reference site; sedimentation rate; detection of local releases)
-
description of the 210Pbxs-related properties (sedimentation rate, 210Pbxs analysis technique, 210Pbxs age model used, total 210Pbxs inventory in the core, 210Pbxs inventory at a nearby reference site).
In this meta-analysis, both 137Cs and 210Pbxs activities and inventories were systematically decay-corrected from the sampling date to 1 January 2020 to allow comparisons between cores.
2.1 Publication characteristics
Studies included in the database mainly provided dating of lacustrine sediment (47 %) and coastal–bay–estuarine sequences (29 %) and to a lesser extent, marine sediment (9 %), floodplain–riverine sediment (8 %), marsh–wetland–peatland sediment (4.5 %) and lagoon sediment (2.5 %). These studies were published in a large variety of journals (n = 153) dealing mainly with geosciences, chemistry and environmental radioactivity. The most frequently encountered journals in the database are the Journal of Environmental Radioactivity (n = 49), Science of the Total Environment (n = 45), Estuarine, Coastal and Shelf Science (n = 23), Environmental Science and Technology (n = 22) and Marine Pollution Bulletin (n = 16). The compiled articles were published between 1977 and 2020. The number of studies reporting the use of 137Cs for dating sediment cores has significantly increased from the 1970s to 2020 (Fig. 2).
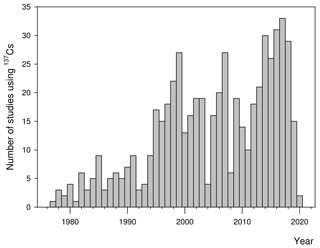
Figure 2Evolution of the number of studies using 137Cs for dating sediment sequences between 1977 and 2020.
Studies compiled in the database mainly focus on contaminant–pollution-related topics (in 31 % of the publications) followed by investigations of sedimentation rates (26 %), monitoring of radionuclide concentrations in the environment (21 %), paleo-environmental reconstructions (9 %), and core chronology methods and limitations (3.5 %). They were conducted in 63 different countries around the world. The countries with the highest number of studies are the United States of America (21 %), China (10 %), the United Kingdom (10 %), France (6 %) and Canada (5 %). Most studies were conducted in Europe during the entire 1977–2020 period (with 43 % of publications) followed by America (32 %, respectively 28.4 % for North America and 3.6 % for South America), Asia (20 %), Africa (3.5 %) and Oceania (1.5 %) – (Fig. 1). However, the proportion of publications per continent has largely changed during the 1977–2020 period. Virtually all publications were conducted at study sites located in Europe and North America during the 1977–1990 period (with 50 % and 48.3 % of studies, respectively) with only some marginal contributions from studies carried out in Asia (1.4 %). Then, between 1990 and 2005, although Europe's (46 %) and North America's (34 %) dominant positions remained, the share of studies conducted in Asia increased (13 %). Finally, among the 313 studies published between 2005 and 2020, only 35 % of studies were carried out in Europe, followed closely by Asia (30 %) and America (including 21 % and 5.5 % for North and South America, respectively) – (Fig. 1). This data compilation underlines the emergence of Asian publications during the last 2 decades, whereas North American and European publications remained stable. In the absence of a large number of studies conducted in the Southern Hemisphere, the current compilation is mainly representative of observations made in the Northern Hemisphere.
2.2 Research design
2.2.1 Metadata and core collection
As metadata are required to extend the data life cycle and promote knowledge integration by the community, a minimum reporting guideline has been recently suggested for short-lived radionuclides (Bruel and Sabatier, 2020; Courtney Mustaphi et al., 2019). Among them, the sampling year is one of the most important, and it was reported in 92 % of publications. On average, 5.5 years (standard deviation – SD: 3.5 years) occurred between the core collection in the field and the publication. Coring or site coordinates were provided in 45 % of the publications. Alternatively, the site location was indicated on a map, or the name of the location of interest (city, river, lake, area) was given in the publication text (52 %). Only 3 % of the publications did not provide any information about the sampling location.
The type of coring device used for sample collection was usually mentioned (81 % of publications). In total, 32 different corer types were reported to have been used. Gravity corer was the most commonly used device (39 %) followed by hand coring (9.6 %), piston corer (8.7 %), box-corer (8.2 %) and vibra-corer (7.3 %). Piston and gravity corers were mostly used in lacustrine studies (in 64 and 76 % of the studies, respectively), and a box corer was used in 81 % of the marine and the coastal–estuarine studies. In contrast, a vibra-corer was mainly used in floodplain–riverine environments (44 % of the studies). When the corer type was provided, the corer brand was only reported in 18.8 % of the publications (the most cited brands are Uwitec, Kajac and Eijkelkamp in respectively 40 %, 10 % and 6 % of the articles reporting this information). Technical characteristics of the corer, such as corer diameter or size, are detailed in 44 % of the studies (their mean diameter amounts to 8.6 cm (SD: 3.2 cm)).
Information about the drainage catchment (e.g., surface area), land use and lake characteristics is only reported in 20 %, 10 % and 17.5 % of the studies that investigated continental environments. When they were mentioned, the average catchment and water body (lake, pond, dam reservoir) surface areas reached on average 8330 km2 (SD: 55 400 km2, range: 0.045 to 525 000 km2 ) and 260 km2 (SD: 1130 km2, range: 0.0005 to 10 000 km2). These average values were likely overestimated because few studies provided the catchment metadata. To improve the inter-comparison between study sites in the future, we highly recommend the authors provide systematically detailed information on the study site description.
2.2.2 Core subsampling and data availability
Information about the total core length was present in 68 % of the publications. This information was given in figures, tables or directly in the text. On average, the core length reached 0.85 m (SD: 1.8 m). The subsampling mode was detailed in 72 % of the publications, with an average increment thickness of 2.1 cm (SD: 1.5 cm).
In 73 % of the publications, the analytical method used to analyze 210Pbxs was provided. Alpha spectrometry was used in 33 % of the studies, whereas gamma spectrometry was used in the rest of the publications. As shown in Fig. 3, a shift between both analytical techniques was observed around 2005, due to the analytical developments made during this period and the improvement of gamma spectrometry potential at low energies, including at 46 keV, i.e., the gamma emission peak of 210Pb.
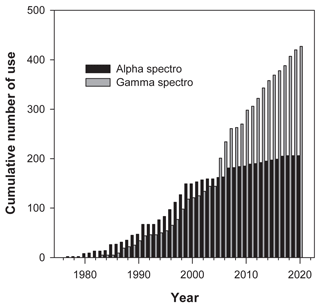
Figure 3Evolution of the cumulative number of studies using alpha spectrometry and gamma spectrometry for analyzing 210Pbxs in sediment sequences between 1977 and 2020.
In 60.5 % of the studies, radionuclide data were provided in figures only, while in 30.7 % of the articles they were reported in both figures and tables. Tables were only found in 1.3 % of the publications. In 7.5 % of the studies, radionuclide activities were not presented at all; only the dating results (i.e., estimated year of sediment deposition in the successive layers) were provided. The studies in which the detailed data were not reported could not be included to conduct study comparisons. We highly encourage future studies to include at least the peak radionuclide activities in the text or, even better, the provide a table with the comprehensive radionuclide analysis results in the sediment archives.
3.1 137Cs detection
The first appearance of 137Cs in the deepest sediment layers was discussed in 14 % of studies. In most of them, this fallout was attributed to the 1954–1955 period corresponding to the initial ubiquitous fallout of this radionuclide around the world (Evrard et al., 2020). As the 137Cs fallout activities in 1954 have remained relatively low and largely (∼ 75 %) decayed since then, the first 137Cs appearance found in depth in a sediment core likely corresponds to deposition that took place in 1955. It was detected at the average depth of 41 cm (SD: 45 cm, range: 2 to 360 cm). In some studies, conducted in both the Northern Hemisphere (e.g., Canada) and Southern Hemisphere (e.g., South Africa) the deepest fallout appearance in the core was nevertheless attributed to 1957 or 1958, without any further explanation in the text.
Fallout associated with the atmospheric nuclear tests (∼ 1950 to ∼ 1980, with a peak in 1963) was detected in 50.9 % of the sediment cores (n = 668) at a mean depth of 27.5 cm (SD: 36 cm, range: 1 to 286 cm) with an average activity of 69.8 Bq kg−1 (SD: 91 Bq kg−1, range: 0.8 to 473 Bq kg−1) in the Northern Hemisphere and 11.7 Bq kg−1 (SD: 16 Bq kg−1, range: 0.4 to 85 Bq kg−1) in the Southern Hemisphere. The year of maximal fallout was commonly attributed to 1963 in both the Northern Hemisphere and Southern Hemisphere, although studies conducted in the Southern Hemisphere attributed the year 1964 (n = 5) or 1965 (n = 4) to this peak on several occasions, to take into account the time needed for a full atmosphere homogenization. The Chernobyl accident was identified by the publication authors in 20.5 % of the sediment cores (n = 278 sediment cores). This event was mainly recorded in Europe (77.7 %), although it was also reported from cores collected in Asia (17.6 %, mostly in the western part of the continent) as well as in northern Africa (1.4 %) – (Fig. 4). Surprisingly, a peak of radiocaesium was also attributed to this accident in cores from China (n = 17), eastern Asia, North America (2.9 %, Canada (n = 4) and Mexico (n = 3)) as well as in central Africa (Ghana (n = 2)) or in Oceania (Australia (n = 1)). The average activity associated with this accidental fallout was 670 Bq kg−1 (SD: 5070 Bq kg−1, range: 2.3 to 48 000 Bq kg−1). The highest activities associated with the Chernobyl accident were detected in the vicinity of the nuclear plant, especially in Ukraine, where the highest activities were recorded, as well as in Sweden, Germany, Poland, Romania and the Alps. The lowest activities attributed to this accident were mostly located in western Europe (e.g., United Kingdom) and in southeastern Europe (Greece, Turkey). On average, this event was detected at a 30 cm depth (SD: 59 cm, range: 1 to 420 cm) in the sediment cores.
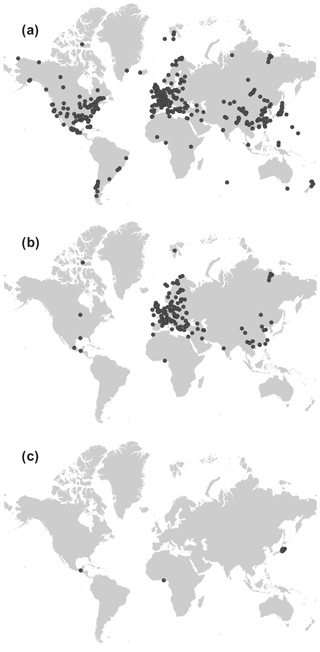
Figure 4Identification of fallout peaks attributed (a) to the atmospheric nuclear tests, (b) the Chernobyl accident and (c) the Fukushima accident in the sediment cores.
The discrimination between fallout that occurred in 1963 and after Chernobyl can be achieved through the analysis of 239+240Pu and 241Am. Both radionuclides were respectively searched for in 12.7 % and 11 % of the sedimentary sequences compiled in this database. Accordingly, 241Am was found in 30 % of studies where the 1963 fallout alone was identified.
Finally, fallout associated with the Fukushima accident was identified in 1.9 % of the sediment cores (n = 26). This level was mainly detected in mainland Japan or along the Japanese eastern coast (Fig. 4), although it was also surprisingly identified in Mexican and Ghanaian sediment sequences. The average activity associated with this fallout was 1920 Bq kg−1 (SD: 7780 Bq kg−1, range: 6 to 35 700 Bq kg−1). This level was identified at an average depth of 1.8 cm (SD: 3 cm, range: 0.25 and 11.5 cm) in the sediment sequences.
In addition to fallout attributed to the Fukushima and Chernobyl accidents and the atmospheric nuclear tests, 24 other local or regional events that have released radionuclides into the environment were identified by the authors and listed in the current database (Table 1). Among these events, emissions following the Sellafield nuclear plan accidents were observed in 40 sequences (3 % of the sediment cores covered in the database), the fallout associated with the Mayak Production Association (Russia) in 12 sediment cores (0.9 %), the dumping of radioactive waste into the Kara Sea (Russia) in 8 sediment cores (0.6 %), the Chinese nuclear test fallout in 5 sediment archives (0.4 %), the Mühlenberg nuclear power plant (Switzerland) in 5 sediment cores, the Bugey and Crey-Malville nuclear facilities (France) in 5 cores, and the Indian Point Energy Center in 4 sequences (New York State, USA). Other events included releases from the Krasnoyarsk-26 site (Russia; n = 4 cores), Rokkasho nuclear facilities (Japan; n = 3), La Hague nuclear fuel reprocessing plant (France; n = 3) and underwater nuclear weapons tests conducted in Novaya Zemlya (Russia, n = 3), (Fig. 5 and Table 1).
Table 1Details on the local and regional events that were shown to have released radionuclides into the environment (NA: not attributed; NPP: nuclear power plant; DoE: US Department of Energy).
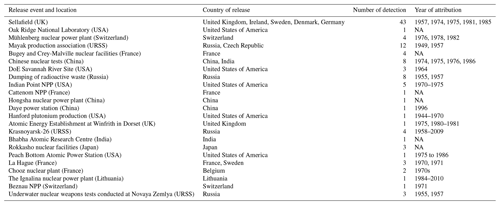
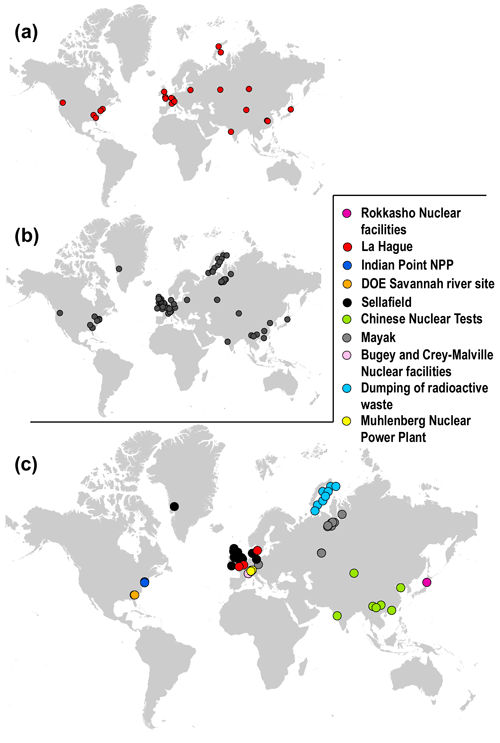
Figure 5137Cs activity levels attributed to local and regional radioactive releases. (a) Local and regional sources identified in the literature. (b) Releases associated with these events detected in sediment cores. (c) Some examples of events detected in sediment cores.
As the calculation of radionuclide inventories required an estimation of the dry bulk density which was often lacking in publications, 137Cs inventories were only reported for 26 % of the sediment cores. The average inventory in the Northern Hemisphere was 30 890 Bq m−2 (SD: 359 000 Bq m−2, range: 10 to 6 000 000 Bq m−2; the highest value was measured in Russia close to the Mayak site) compared to 990 Bq m−2 (SD: 1030 Bq m−2, range: 205 to 3180 Bq m−2) in the Southern Hemisphere. The high standard deviation observed in the Northern Hemisphere is mainly due to the high inventories found in the vicinity of Chernobyl, Krasnoyarsk and the Mayak production site (Fig. 6).
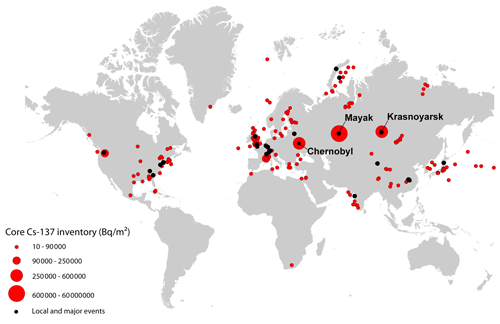
Figure 6137Cs inventory in the sediment cores covered in the database and location of some of the main sources of 137Cs.
Inventories of 137Cs at reference locations located close to the sediment core collection site were provided in 7.7 % of the publications. The average reference inventory was 1610 Bq m−2 (SD: 2810 Bq m−2, range: 268 to 24 000 Bq m−2) in the Northern Hemisphere. Only one inventory was reported in the Southern Hemisphere (360 Bq m−2).
Information about sedimentation rates based on 137Cs analyses was provided in 34.6 % of the publications. The 1963 peak level was generally used for estimating this average sedimentation rate.
3.2 Latitudinal distribution of 137Cs in sediment cores
The maximal activities associated with the atmospheric nuclear tests (activities generally attributed to 1963) were recorded between the latitudinal bands comprised between 40 and 60∘ N, with an average activity of 90 and 117 Bq kg−1, respectively. Values were not available for the bands located between 0–10∘, 50–60∘ and 80–90∘ S. This is consistent with the United Nations Scientific Committee of the Effects of Atomic Radiation (UNSCEAR, 2000) compilations recording the highest inventories between 40 and 60∘ N for this event (inventories reached respectively 1186 and 1383 Bq m2). Distribution of both databases were similar, with a strong correlation coefficient between inventories and activities (r2 = 0.87, p value < 0.05) – (Fig. 7).
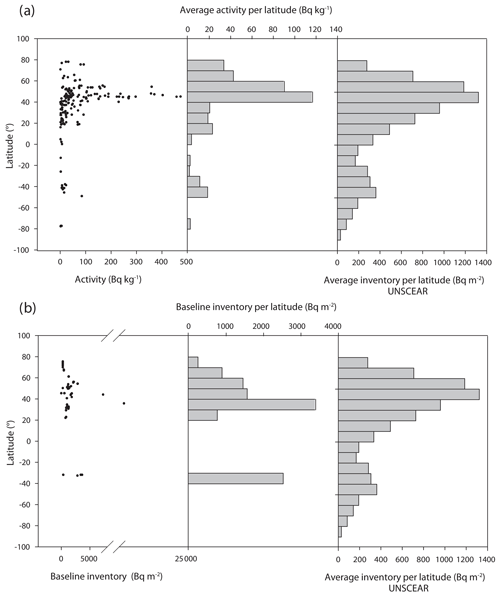
Figure 7(a) Comparison of latitudinal distribution of 137Cs activities associated with the atmospheric nuclear tests. From left to right, individual data collected in sediment cores, average activity per latitude as derived from the current database and average inventory distribution per latitudinal band as derived from the UNSCEAR database. (b) Comparison of latitudinal distribution of 137Cs baseline inventories. From left to right, individual data collected in sediment cores, average inventory per latitudinal band provided by the database and the average inventory distribution per latitudinal band as from the UNSCEAR database.
Comparison between reference inventories of 137Cs compiled in the current research and those from UNSCEAR showed a lower correlation (r2 = 0.4, p value < 0.05). This poorer correlation was mostly due to the absence of inventories reported between 10∘ N and 30∘ S and between 30 and 90∘ S. It can also be explained by the high inventories recorded between 30∘ and 40∘ N, which were impacted by the high values measured in the vicinity of the Mayak production site (Fig. 7).
3.3 210Pbxs detection and use
This natural radionuclide was often used in combination with 137Cs in this database (in 64 % of the studies). In these publications, the core chronology based on 210Pbxs was estimated with seven different models: constant rate of supply (CRS) (Appleby and Oldfield, 1978), constant initial concentration (CIC) (Pennington et al., 1976), constant flux constant sedimentation (CFCS) (Krishnaswamy et al., 1971), periodic flux model (PF) (Sanchez-Cabeza et al., 2000), constant initial concentration and constant sedimentation rate (CICCS) (He and Walling, 1996), constant rate of accumulation (CRA) and constant initial activity (CIA) (Robbins and Herche, 1993). Among the most employed tools, the CRS model (also referred to as the CRSMV model in some publications) was applied in 54 % of the studies, the CIC model (also designated as CA model) in 23 % of the articles and the CFCS model (also called the CF, CSM, CFS or RSSM model with some of them including limited adaptations) in 20 % of the publications. Sedimentation rate was detailed in 56.7 % of publications using the 210Pbxs for establishing core chronology.
The 210Pbxs inventories were given in 11.7 % of the studies, and the inventories of this natural radionuclide at references sites were presented in only 4.1 % of the studies. The average inventory in the Northern Hemisphere amounted to 6510 Bq m−2 (SD: 10 640 Bq m−2, range: 20 to 96 000 Bq m−2). Only one value was available in the Southern Hemisphere (2560 Bq m−2). The average inventory at reference sites in the Northern Hemisphere was 1730 Bq m−2 (SD: 760 Bq m−2) with an average flux of 90 Bq m−2 yr−1 (SD: 100 Bq m−2 yr−1). Corresponding inventories at reference sites were not reported for the Southern Hemisphere and only one value of 210Pbxs flux was provided (39 Bq m−2 yr−1).
3.4 Other radionuclides
In addition to 137Cs and 210Pbxs, other radionuclides were frequently detected in the sediment cores. Most of these additional radionuclides were analyzed in order to provide a discrimination between the different 137Cs sources including plutonium, americium, cobalt and strontium or to provide information on sediment dynamics over shorter periods (e.g., 7Be, 228Th). Among the 28 radionuclides frequently identified, the 239+240Pu ratio and 241Am activities were the most widely reported. The other reported radionuclides were beryllium isotopes (7Be, 1.7 %; 8Be, 0.07 %; 9Be, 0.07 %; 10Be, 0.22 %; 11Be, 0.07 %), bismuth (207Bi, 0.22 %; 214Bi, 0.59 %), caesium (134Cs, 4.9 %), cobalt (60Co, 1.4 %), neptunium (237Np, 0.15 %), plutonium (238Pu, 2.5 %; 241Pu, 0.3 %; 242Pu, 0.07 %; 243Pu, 0.07 %), potassium (40K, 1.5 %), radium (226Ra, 0.96 %; 228Ra, 0.14 %), strontium (90Sr, 2.8 %), technetium (99Tc, 0.07 %), thorium (228Th, 0.3 %; 232Th, 0.3 %; 234Th, 0.37 %) and uranium (236U, 0.07 %; 238U, 0.3 %). The 14C was analyzed in 4.5 % of the sediment cores. This isotope was exclusively analyzed by accelerator mass spectrometry (AMS) in the articles included in the database for providing long-term chronology.
4.1 General patterns of 137Cs fallout
According to UNSCEAR (2000), 77 % of the total worldwide fallout of 137Cs is estimated to have occurred in the Northern Hemisphere. Previous studies have identified two main sources of 137Cs in Europe, including the fallout associated with the thermonuclear weapon tests and that emitted by the Chernobyl accident (Meusburger et al., 2020). In the current database, 137Cs deposition associated with above-ground nuclear tests was largely detected across the European continent with a peak systematically assigned to 1963 (Fig. 4). This peak was described in the literature as the most reliable time marker worldwide (He and Walling, 1997). In addition, no technical problem was reported to detect the occurrence of fallout associated with the Chernobyl accident. This fallout was identified in sediment cores across Europe from north to south (from Norway to Spain) and from west to east (from Ireland to Belarus). In certain areas as in western France, southwestern England, southern Spain, Portugal or Iceland, this fallout was not detected (Fig. 4). This observation is in agreement with our previous knowledge on the spatial distribution of Chernobyl fallout across Europe (Evangeliou et al., 2016). In some regions (e.g., Alps), the Chernobyl fallout appears to be highly heterogeneous and reflects the radioactive cloud pathway and the heterogeneous rainfall pattern after the catastrophe (Alric et al., 2013). In addition to the peaks associated with the Chernobyl accident and the global fallout, the current research has allowed us to identify nine local or regional events in Europe that led to radionuclide releases and peaks in sediment sequences. The Sellafield–Windscale releases (1957, 1974, 1975, 1981, 1985) were the most often detected in European sequences (identified in the United Kingdom, along Greenland coasts and in the Baltic Sea) – (e.g., Perner et al., 2010).
In North America, the 1963 peak was often detected in sediment cores (e.g., Van Metre et al., 2004; Corcoran et al., 2018) – (Fig. 4). Five local events were also identified in addition to this peak with a limited spatial extent as in the vicinity of the Savannah River site (1964) (Lewis et al., 2000), Indian Point Energy Center (1971–1975) (Bopp et al., 1982), Oak Ridge National Laboratory (Sobocinski, 1990), the Peach Bottom Atomic Power Station (McLean and Summers, 1990) and the Hanford Plutonium Production Center (1944–1970) (Beasley and Jennings, 1984).
Asia and more specifically China proved to be the most complex region of the world for identifying the sources of the discrete peaks of 137Cs. The 137Cs peaks identified in parts of China (e.g., Xinjiang, Guangdong, Guizhou provinces – Bai et al., 2002; Chen et al., 2014) were associated with Chinese aboveground nuclear tests conducted during the 1960s–1970s. In addition, several studies conducted in the northwest and the south of China (Xinjiang, Yunnan and adjacent areas) proposed that the uppermost 137Cs peak found in lacustrine sediment cores may be attributed to the Chernobyl fallout in 1986. In Chinese cores, fallout associated with the Chernobyl accident was even detected more frequently than the Chinese nuclear tests. It was detected in the Tibetan Plateau (e.g., Lake Ngoring – Zhang et al., 2014), in the headwaters of the Yellow River and in cores collected in lower reaches of this river, in its delta (Wu et al., 2015) and along the Yellow Sea coastline (Sun et al., 2012). This event was also often identified in several lacustrine deposits of southwestern China (e.g., Yunnan Province) – (Zeng and Wu, 2009; Wang et al., 2018). Conversely, it was almost never detected in the central and northeastern parts of China (it was only reported in the Miyun reservoir, north of Beijing; Xia et al., 2015). However, whether this/these peak(s) should be attributed to the Chinese nuclear tests, Russian tests or the Chernobyl accident remains debated in the scientific community. Nevertheless, the current literature review shows that a 137Cs peak in a lacustrine sediment core was never attributed to the Chernobyl accident in a vast region comprised between the eastern part of Turkey and China. Accordingly, it is very unlikely that the attribution of a 137Cs peak to the Chernobyl fallout in Chinese cores is meaningful. As these events took place ∼ 10 years apart, future research should incorporate the use of additional tracers that may unambiguously discriminate both sources of radionuclides in this region. Plutonium (240Pu 239Pu) or caesium (135Cs 137Cs) atom ratios may provide good candidates to achieve this type of discrimination (Bu et al., 2015; Lan et al., 2020; Zhu et al., 2020).
Further to the east, lacustrine and marine sedimentary sequences studied in Japan allowed us to identify three main sources of 137Cs. The first local 137Cs source detected in Japan was attributed to the atomic bomb peak in 1945 in the Nagasaki area (Mahara and Kudo, 1995). The peak associated with the atmospheric nuclear tests in 1963 was then largely recorded across the entire Japanese peninsula (e.g., Lu and Matsumoto, 2009; Hosono et al., 2016) whereas the fallout associated with the Fukushima accident was mainly identified in the vicinity of the Fukushima NPP, in particular in lacustrine sediments of the Miyagi, Fukushima and Ibaraki prefectures (Cao et al., 2017; Jaegler et al., 2019; Minoura et al., 2014), as well as in sediment samples collected along the Pacific coast off the Fukushima prefecture (e.g., Black and Buesseler, 2014) – (Fig. 4).
According to UNSCEAR (2000), only 23 % of the total 137Cs fallout is estimated to have occurred in the Southern Hemisphere. In contrast to what was reported for the Northern Hemisphere, few studies using both 137Cs and 210Pbxs for dating sediment archives were found in this part of the world. This is mainly the case for Africa and Oceania. Studies available in the Southern Hemisphere reported the occurrence of a single peak attributed to the thermonuclear tests. However, no consensus was shared in the literature to assign a single year to this peak. Several authors have attributed the year 1963 (e.g., Chile, Antarctica – Urrutia et al., 2007; Christ et al., 2015) to this peak as in the Northern Hemisphere, whereas other authors dated this peak to 1964 (e.g., Chile – Elbert et al., 2013) or even 1965 (e.g., in Chile, Argentina, South Africa or in the Kerguelen Island – Arnaud et al., 2006; Kastner et al., 2010; Boardman and Foster, 2011; Ficetola et al., 2018). This shift of 1 to 2 years in the assigned year for the thermonuclear bomb fallout peak between the Northern Hemisphere and Southern Hemisphere can be attributed to the time required for the mixing of air masses from both hemispheres and the occurrence of significant 137Cs fallout transfer to the Southern Hemisphere. However, this question remains debated, and additional sedimentary sequences should be collected in the future to improve the assignment of this peak (Guevara and Arribére, 2007) and sediment core dating in the Southern Hemisphere.
4.2 Limitations of the currently published 137Cs research
This worldwide database also underlines the unexpected detection of 137Cs sources in certain regions of the world. In China, a large number of studies claim to have detected Chernobyl fallout, whereas more regional sources of 137Cs attributed to the Chinese and Russian nuclear tests conducted in Lop Nor and Semipalatinsk, respectively, are not considered a potential origin (Huang et al., 2019; Zhao et al., 2020). The potential impact of these tests should be investigated more thoroughly. Conversely, the attribution of a peak to the Chernobyl fallout should be avoided without clear isotopic evidence (e.g., plutonium isotopes), as the associated fallout was not observed in a vast region that extends from the Ural Mountains/eastern Turkey to China. For example, in a lacustrine sediment core collected in Mexico, the occurrence of 137Cs was attributed to both the Chernobyl (Méndez-García et al., 2014) and Fukushima accidents (Caballero et al., 2020). This remains to be confirmed as other studies conducted nearby did not record these 137Cs sources. They only recorded fallout associated with the global bomb peak in 1963 (e.g., Hansen, 2012). A similar finding was made in Ghana where both fallout sources associated with Fukushima and Chernobyl accidents were recorded in the Amisa and Sakumo River estuaries (Mahu et al., 2016), whereas no single 137Cs peak could be identified in sediment collected in the nearby Volta and Pra River estuaries (Klubi et al., 2017). In North America, 137Cs fallout attributed to Chernobyl was identified in Canada, in Vermilion Lake (Schindler and Kamber, 2013), as well as in North Lake in the United States (Hardaway et al. 1998). The attribution of 137Cs peaks to Chernobyl in Mexico, North America and Africa should be made with great caution in view of the observations made in the current literature compilation and our knowledge of the fallout dispersion (Evangeliou et al., 2016).
These very likely incorrect assignments could instead have been induced by the occurrence of some local and regional 137Cs sources or by various processes that may affect the distribution of 137Cs in sediment cores. For example, soil erosion was detected in various studies as one of the major processes complicating the 137Cs peak identification in the core. This process may have induced an incorrect assignment through the sudden delivery of sediment with higher 137Cs levels (Foucher et al., 2019; Swales et al., 2002) or in contrast lower levels of 137Cs in some sediment layers that may be delivered by subsoil sources, sheltered from atmospheric fallout and depleted in this radioisotope (Foster et al., 2005). Furthermore, earlier deposits of 137Cs on ice and snow were reported to have increased the 137Cs activity in a sediment sequence of Antarctica after ice melting (Sanders et al., 2010). Conversely, certain storm events and the associated flood deposits can lead to the dilution of the 137Cs signal (Gu et al., 2011). In addition to these natural and anthropogenic processes that may lead to an increase or a decrease in the 137Cs activity along sedimentary sequences, a large number of studies included in the current database reported the occurrence of post-depositional processes. Among them, bioturbation and post-depositional mobility/diffusion may disturb the temporal profile of radionuclides with depth along the core and dilute their concentrations (Benninger et al., 1998; Larsen et al., 2010; Matter et al., 2010). Finally, in some studies, this intense sediment mixing led to the impossibility to establish a chronology, typically when no discrete peak was clearly detected (Eades et al., 2002) or when inconsistent peaks were observed. The frequency of the occurrence of these mixing processes in sediment sequences is difficult to estimate as the analyses of cores that cannot be dated often remain unpublished.
4.3 Main recommendations for future studies
Overall, this literature review has demonstrated the frequent lack of metadata in publications dealing with sediment core dating and analysis. Accordingly, we highly recommend the authors of future studies to provide detailed information about the reservoir/coring site characteristics, the catchment properties (when relevant) and the coring methodology. In the current synthesis, raw radionuclide data were only provided in tables or in a reusable format in 30.7 % of the publications. To extend the short-lived radionuclide data life cycle (Bruel and Sabatier, 2020; Courtney Mustaphi et al., 2019; Wilkinson et al., 2016) and allow more frequent inter-comparisons between sites and methodologies, we strongly encourage the authors to systematically provide the detailed radionuclide analysis results in tables associated with the publication or in open-access data repositories.
Most of the studies covered in the current compilation were conducted in the Northern Hemisphere. This synthesis therefore highlights a massive lack of studies in the Southern Hemisphere in general, and in Africa in particular. As these regions of the world have been undergoing major anthropogenic changes and pressures since the mid-20th century, this should be further explored and investigated through the analysis of sediment cores. The achievement of more studies in the Southern Hemisphere will contribute to improve our knowledge of nuclear-fallout-related events in the southern half of the world where no consensus has been reached at this stage regarding the attribution of a single year to the 137Cs fallout peak.
Finally, the current meta-analysis suggests the potential occurrence of errors in peak attributions in certain regions of the world. To avoid any dating and subsequent interpretation errors in the reconstructions (e.g., contamination) based on the analysis of these archives, we strongly recommend the analysis of complementary tracers that may discriminate 137Cs sources unambiguously (e.g., 241Am, 90Sr, plutonium (240Pu 239Pu) or caesium (135Cs 137Cs) atom ratios).
Data are available in the PANGAEA repository at https://doi.org/10.1594/PANGAEA.931493 (Foucher et al., 2021).
A unique meta-analysis of the sediment core dating research using radionuclides was conducted for the 1977–2020 period. This literature overview provides a worldwide reference to help the scientific community reach a consensus for dating recent sedimentary archives by identifying the 137Cs peak(s) that may be found in various regions and environments across the globe. This study documents the occurrence of three main sources of 137Cs that are the most widely detected in sediment cores (nuclear weapon tests, Chernobyl and Fukushima accidents), as well as 24 additional local and regional releases of 137Cs, which have been synthetized here. The correct attribution of these sources may improve the establishment of core chronology or the validation of 210Pbxs age models. Nevertheless, this meta-analysis outlines the complexity to discriminate between some of these 137Cs sources and the necessity to use additional tools such as plutonium and caesium isotopic ratios to provide an unambiguous distinction between potential sources and avoid any dating errors. This review also highlights the low proportion of paleo-reconstructions conducted in the Southern Hemisphere in general, and in Africa and Oceania in particular, compared to what has been published for the Northern Hemisphere. It also underlines the necessity to improve the identification of the maximal period of 137Cs emissions after the thermonuclear tests in the Southern Hemisphere by collecting a larger number of sediment archives across wider areas.
Overall, this meta-analysis demonstrates the effectiveness of using the 137Cs and 210Pbxs activities for dating sediment cores and reconstructing environmental changes and their impacts over the 20th century. In the future, authors are encouraged to share their data including the radionuclide activities measured in the successive sediment layers and the associated metadata in their publications.
The supplement related to this article is available online at: https://doi.org/10.5194/essd-13-4951-2021-supplement.
AF and OE contributed to the dataset compilation. AF, PAC, PS and OE participated in the results discussion and drafted the main manuscript.
The contact author has declared that neither they nor their co-authors have any competing interests.
Publisher's note: Copernicus Publications remains neutral with regard to jurisdictional claims in published maps and institutional affiliations.
The authors are very grateful to the scientific community using fallout radionuclides and especially to those colleagues who published their raw data for dating sediment cores without whom the current overview would not have been possible. The lockdown periods associated with the worldwide COVID-19 pandemic also allowed the authors to find time to focus on this demanding literature review.
This article benefited from support of the INTERPOL project (INTERcomparison of sediment POLlution on the main French rivers) funded by OFB (French Agency of Biodiversity) and multiple discussions in the framework of the TRAJECTOIRE project funded by the French National Research Agency (ANR-19-CE03-0009).
This paper was edited by Attila Demény and reviewed by two anonymous referees.
Alric, B., Jenny, J. P., Berthon, V., Arnaud, F., Pignol, C., Reyss, J. L., Sabatier, P., and Perga, M. E.: Local forcings affect lake zooplankton vulnerability and response to climate warming, Ecology, 94, 2767–2780, https://doi.org/10.1890/12-1903.1, 2013.
Appleby, P. G. and Oldfield, F.: The calculation of lead-210 dates assuming a constant rate of supply of unsupported210Pb to the sediment, Catena, 5, 1–8, https://doi.org/10.1016/S0341-8162(78)80002-2, 1978.
Appleby, P. G., Richardson, N., and Nolan, P. J.: 241Am dating of lake sediments, Hydrobiologia, 214, 35–42, https://doi.org/10.1007/BF00050929, 1991.
Arnaud, F., Magand, O., Chapron, E., Bertrand, S., Boës, X., Charlet, F., and Mélières, M. A.: Radionuclide dating (210Pb, 137Cs, 241Am) of recent lake sediments in a highly active geodynamic setting (Lakes Puyehue and Icalma-Chilean Lake District), Sci. Total Environ., 366, 837–850, https://doi.org/10.1016/j.scitotenv.2005.08.013, 2006.
Bai, Z. G., Wan, G. J., Huang, R. G., and Liu, T. S.: A comparison on the accumulation characteristics of 7Be and 137Cs in lake sediments and surface soils in western Yunnan and central Guizhou, China, Catena, 49, 253–270, https://doi.org/10.1016/S0341-8162(02)00004-8, 2002.
Beasley, T. M. and Jennings, C. D.: The inventories of plutonium-239, -240, americium-241, cesium-137, and cobalt-60 in Columbia River sediments from Hanford to the Columbia River Estuary, Environ. Sci. Technol., 18, 207–212, https://doi.org/10.1021/es00121a014, 1984.
Benninger, L. K., Suayah, I. B., and Stanley, D. J.: Manzala lagoon, Nile delta, Egypt: Modern sediment accumulation based on radioactive tracers, Environ. Geol., 34, 183–193, https://doi.org/10.1007/s002540050270, 1998.
Binford, M. W.: Calculation and uncertainty analysis of 210Pb dates for PIRLA project lake sediment cores, J. Paleolimnol., 3, 253–267, https://doi.org/10.1007/BF00219461, 1990.
Black, E. E. and Buesseler, K. O.: Spatial variability and the fate of cesium in coastal sediments near Fukushima, Japan, Biogeosciences, 11, 5123–5137, https://doi.org/10.5194/bg-11-5123-2014, 2014.
Boardman, J. and Foster, I. D. L.: The potential significance of the breaching of small farm dams in the Sneeuberg region, South Africa, J. Soil. Sediment., 11, 1456–1465, https://doi.org/10.1007/s11368-011-0425-5, 2011.
Bopp, R. F., Simpson, H. J., Olsen, C. R., Trier, R. M., and Kostyk, N.: Chlorinated hydrocarbons and radionuclide chronologies in sediments of the Hudson River and estuary, New York, Environ. Sci. Technol., 16, 666–676, https://doi.org/10.1021/es00104a008, 1982.
Bruel, R. and Sabatier, P.: serac: an R package for ShortlivEd RAdionuclide chronology of recent sediment cores, J. Environ. Radioact., 225, 106449, https://doi.org/10.1016/j.jenvrad.2020.106449, 2020.
Bu, W., Ni, Y., Guo, Q., Zheng, J., and Uchida, S.: Pu isotopes in soils collected downwind from Lop Nor: Regional fallout vs. global fallout, Sci. Rep., 5, 12262, https://doi.org/10.1038/srep12262, 2015.
Caballero, M., Mora, L., Muñoz, E., Escolero, O., Bonifaz, R., Ruiz, C., and Prado, B.: Anthropogenic influence on the sediment chemistry and diatom assemblages of Balamtetik Lake, Chiapas, Mexico, Environ. Sci. Pollut. Res., 27, 15935–15943, https://doi.org/10.1007/s11356-019-04581-9, 2020.
Cao, L., Ishii, N., Zheng, J., Kagami, M., Pan, S., Tagami, K., and Uchida, S.: Vertical distributions of Pu and radiocesium isotopes in sediments from Lake Inba after the Fukushima Daiichi Nuclear Power Plant accident: Source identification and accumulation, Appl. Geochem., 78, 287–294, https://doi.org/10.1016/j.apgeochem.2017.01.012, 2017.
Chen, B., Zheng, Z., Huang, K., Zheng, Y., Zhang, G., Zhang, Q., and Huang, X.: Radionuclide dating of recent sediment and the validation of pollen-environment reconstruction in a small watershed reservoir in southeastern China, Catena, 115, 29–38, https://doi.org/10.1016/j.catena.2013.11.010, 2014.
Christ, A. J., Talaia-Murray, M., Elking, N., Domack, E. W., Leventer, A., Lavoie, C., Brachfeld, S., Yoo, K. C., Gilbert, R., Jeong, S. M., Petrushak, S., Wellner, J., Balco, G., Brachfeld, S., de Batist, M., Domack, E., Gordon, A., Haran, A., Henriet, J. P., Huber, B., Ishman, S., Jeong, S., King, M., Lavoie, C., Leventer, A., McCormick, M., Mosley-Thompson, E., Pettit, E., Scambos, T., Smith, C., Thompson, L., Truffer, M., van Dover, C., Vernet, M., Wellner, J., Yu, K., and Zagorodnov, V.: Late Holocene glacial advance and ice shelf growth in Barilari Bay, Graham Land, West Antarctic Peninsula, Bull. Geol. Soc. Am., 127, 297–315, https://doi.org/10.1130/B31035.1, 2015.
Corcoran, M., Sherif, M. I., Smalley, C., Li, A., Rockne, K. J., Giesy, J. P., and Sturchio, N. C.: Accumulation rates, focusing factors, and chronologies from depth profiles of 210Pb and 137Cs in sediments of the Laurentian Great Lakes, J. Great Lakes Res., 44, 693–704, https://doi.org/10.1016/j.jglr.2018.05.013, 2018.
Courtney Mustaphi, C. J., Brahney, J., Aquino-López, M. A., Goring, S., Orton, K., Noronha, A., Czaplewski, J., Asena, Q., Paton, S., and Panga Brushworth, J.: Guidelines for reporting and archiving 210Pb sediment chronologies to improve fidelity and extend data lifecycle, Quat. Geochronol., 52, 77–87, https://doi.org/10.1016/j.quageo.2019.04.003, 2019.
Dearing, J. A. and Jones, R. T.: Coupling temporal and spatial dimensions of global sediment flux through lake and marine sediment records, Global Planet. Change, 39, 147–168, https://doi.org/10.1016/S0921-8181(03)00022-5, 2003.
Delaval, A., Duffa, C., and Radakovitch, O.: A review on cesium desorption at the freshwater-seawater interface, J. Environ. Radioact., 218, 106255, https://doi.org/10.1016/j.jenvrad.2020.106255, 2020.
Eades, L. J., Farmer, J. G., MacKenzie, A. B., Kirika, A., and Bailey-Watts, A. E.: Stable lead isotopic characterisation of the historical record of environmental lead contamination in dated freshwater lake sediment cores from northern and central Scotland, Sci. Total Environ., 292, 55–67, https://doi.org/10.1016/S0048-9697(02)00026-8, 2002.
Elbert, J., Wartenburger, R., von Gunten, L., Urrutia, R., Fischer, D., Fujak, M., Hamann, Y., Greber, N. D., and Grosjean, M.: Late Holocene air temperature variability reconstructed from the sediments of Laguna Escondida, Patagonia, Chile (45∘ N30'S), Palaeogeogr. Palaeoclim., 369, 482–492, https://doi.org/10.1016/j.palaeo.2012.11.013, 2013.
Evangeliou, N., Hamburger, T., Talerko, N., Zibtsev, S., Bondar, Y., Stohl, A., Balkanski, Y., Mousseau, T. A., and Møller, A. P.: Reconstructing the Chernobyl Nuclear Power Plant (CNPP) accident 30 years after. A unique database of air concentration and deposition measurements over Europe, Environ. Pollut., 216, 408–418, https://doi.org/10.1016/j.envpol.2016.05.030, 2016.
Evrard, O., Chaboche, P.-A., Ramon, R., Foucher, A., and Laceby, J. P.: A global review of sediment source fingerprinting research incorporating fallout radiocesium (137Cs), Geomorphology, 362, 107103, https://doi.org/10.1016/j.geomorph.2020.107103, 2020.
Ficetola, G. F., Poulenard, J., Sabatier, P., Messager, E., Gielly, L., Leloup, A., Etienne, D., Bakke, J., Malet, E., Fanget, B., Støren, E., Reyss, J.-L., Taberlet, P., and Arnaud, F.: DNA from lake sediments reveals long-term ecosystem changes after a biological invasion, Sci. Adv., 4, eaar4292, https://doi.org/10.1126/sciadv.aar4292, 2018.
Foster, I. D. L., Boardman, J., Keay-Bright, J., and Meadows, M. E.: Land degradation and sediment dynamics in the South African Karoo, in: IAHS-AISH Publication, 207–213, Proceedings of symposium S1 held during the Seventh IAHS Scientific Assembly at Foz do Iguaçu, Brazil, April 2005, 2005.
Foucher, A., Evrard, O., Chabert, C., Cerdan, O., Lefèvre, I., Vandromme, R., and Salvador-Blanes, S.: Erosional response to land abandonment in rural areas of Western Europe during the Anthropocene: A case study in the Massif-Central, France, Agr. Ecosyst. Environ., 284, 106582, https://doi.org/10.1016/j.agee.2019.106582, 2019.
Foucher, A., Chaboche, P.-A., Sabatier, P., and Evrard, O.: Global review of 137Cs and 210Pbxs fallout used for dating sediment cores, PANGAEA [data set], https://doi.org/10.1594/PANGAEA.931493, 2021.
Goldberg, E.: Proceeding of a symposium on radioactive dating, in Geochronology with Pb-210, Proceedings of the Symposium on Radioactive Dating Held by the International Atomic Energy Agency in Co-operation with the Joint Commission on Applied Radioactivity, Athens, 1963.
Gu, J., Chen, Z., and Salem, A.: Post-Aswan dam sedimentation rate of lagoons of the Nile Delta, Egypt, Environ. Earth Sci., 64, 1807–1813, https://doi.org/10.1007/s12665-011-0983-2, 2011.
Guevara, S. R. and Arribére, M.: Comment on the article “Radionuclide dating (Pb-210, Cs-137, Am-241) of recent lake sediments in a highly active geodynamic setting (Lakes Puyehue and Icalma-Chilean Lake District)”, Sci. Total Environ., 385, 310–311 https://doi.org/10.1016/j.scitotenv.2007.05.006, 2007.
Hansen, A. M.: Lake sediment cores as indicators of historical metal(loid) accumulation – A case study in Mexico, Appl. Geochem., 27, 1745–1752, https://doi.org/10.1016/j.apgeochem.2012.02.010, 2012.
He, Q. and Walling, D. E.: Use of fallout Pb-210 measurements to investigate longer-term rates and patterns of overbank sediment deposition on the floodplains of lowland rivers, Earth Surf. Proc. Land., 21, 141–154, https://doi.org/10.1002/(SICI)1096-9837(199602)21:2<141::AID-ESP572>3.0.CO;2-9, 1996.
He, Q. and Walling, D. E.: The distribution of fallout Cs-137 and Pb-210 in undisturbed and cultivated soils, Appl. Radiat. Isot., 48, 677–690, 1997.
Hosono, T., Alvarez, K., and Kuwae, M.: Lead isotope ratios in six lake sediment cores from Japan Archipelago: Historical record of trans-boundary pollution sources, Sci. Total Environ., 559, 24–37, https://doi.org/10.1016/j.scitotenv.2016.03.138, 2016.
Huang, Y., Tims, S. G., Froehlich, M. B., Pan, S., Fifield, L. K., Pavetich, S., and Koll, D.: The 240Pu/239Pu atom ratio in Chinese soils, Sci. Total Environ., 678, 603–610, https://doi.org/10.1016/j.scitotenv.2019.04.251, 2019.
Jaegler, H., Pointurier, F., Diez-Fernández, S., Gourgiotis, A., Isnard, H., Hayashi, S., Tsuji, H., Onda, Y., Hubert, A., Laceby, J. P., and Evrard, O.: Reconstruction of uranium and plutonium isotopic signatures in sediment accumulated in the Mano Dam reservoir, Japan, before and after the Fukushima nuclear accident, Chemosphere, 225, 849–858, https://doi.org/10.1016/j.chemosphere.2019.03.064, 2019.
Jagercikova, M., Cornu, S., Le Bas, C., and Evrard, O.: Vertical distributions of 137Cs in soils: a meta-analysis, J. Soil. Sediment., 15, 81–95, https://doi.org/10.1007/s11368-014-0982-5, 2014.
Kastner, S., Enters, D., Ohlendorf, C., Haberzettl, T., Kuhn, G., Lücke, A., Mayr, C., Reyss, J. L., Wastegård, S., and Zolitschka, B.: Reconstructing 2000years of hydrological variation derived from laminated proglacial sediments of Lago del Desierto at the eastern margin of the South Patagonian Ice Field, Argentina, Global Planet. Change, 72, 201–214, https://doi.org/10.1016/j.gloplacha.2010.04.007, 2010.
Klaminder, J., Appleby, P., Crook, P., and Renberg, I.: Post-deposition diffusion of 137Cs in lake sediment: Implications for radiocaesium dating, Sedimentology, 59, 2259–2267, https://doi.org/10.1111/j.1365-3091.2012.01343.x, 2012.
Klubi, E., Abril, J. M., Nyarko, E., Laissaoui, A., and Benmansour, M.: Radioecological assessment and radiometric dating of sediment cores from dynamic sedimentary systems of Pra and Volta estuaries (Ghana) along the Equatorial Atlantic, J. Environ. Radioact., 178–179, 116–126, https://doi.org/10.1016/j.jenvrad.2017.08.001, 2017.
Krishnaswamy, S., Lal, D., Martin, J. M., and Meybeck, M.: Geochronology of lake sediments, Earth Planet. Sci. Lett., 11, 407–414, https://doi.org/10.1016/0012-821X(71)90202-0, 1971.
Lan, J., Wang, T., Chawchai, S., Cheng, P., Zhou, K., Yu, K., Yan, D., Wang, Y., Zang, J., Liu, Y., Tan, L., Ai, L., and Xu, H.: Time marker of 137Cs fallout maximum in lake sediments of Northwest China, Quaternary Sci. Rev., 241, 106413, https://doi.org/10.1016/j.quascirev.2020.106413, 2020.
Larsen, J., Appleby, P. G., Christensen, G. N., Berg, T., and Eide, I.: Historical and geographical trends in sediment chronology from lakes and marine sites along the Norwegian Coast, Water. Air. Soil Pollut., 206, 237–250, https://doi.org/10.1007/s11270-009-0099-4, 2010.
Lewis, G. P., Taylor, B. E., Pinder, J. E., and Dixon, P. M.: Apparent decline of the sediment 137Cs inventory of an abandoned reactor cooling reservoir: Export or uncertainty?, J. Environ. Radioact., 49, 293–306, https://doi.org/10.1016/S0265-931X(99)00127-7, 2000.
Lu, X. and Matsumoto, E.: Implications of excess 210Pb and 137Cs in sediment cores from Mikawa Bay, Japan, J. Environ. Sci., 21, 707–712, https://doi.org/10.1016/S1001-0742(08)62328-1, 2009.
Madsen, A. T., Murray, A. S., Andersen, T. J., Pejrup, M., and Breuning-Madsen, H.: Optically stimulated luminescence dating of young estuarine sediments: a comparison with 210Pb and 137Cs dating, Mar. Geol., 214, 251–268, https://doi.org/10.1016/j.margeo.2004.10.034, 2005.
Mahara, Y. and Kudo, A.: Plutonium released by the Nagasaki A-bomb: Mobility in the environment, Appl. Radiat. Isot., 46, 1191–1201, https://doi.org/10.1016/0969-8043(95)00161-6, 1995.
Mahu, E., Nyarko, E., Hulme, S., Swarzenski, P., Asiedu, D. K., and Coale, K. H.: Geochronology and historical deposition of trace metals in three tropical estuaries in the Gulf of Guinea, Estuar. Coast. Shelf S., 177, 31–40, https://doi.org/10.1016/j.ecss.2016.05.007, 2016.
Matter, M., Anselmetti, F. S., Jordanoska, B., Wagner, B., Wessels, M., and Wüest, A.: Carbonate sedimentation and effects of eutrophication observed at the Kališta subaquatic springs in Lake Ohrid (Macedonia), Biogeosciences, 7, 3755–3767, https://doi.org/10.5194/bg-7-3755-2010, 2010.
McLean, R. I. and Summers, J. K.: Evaluation of transport and storage of 60Co, 134Cs, 137Cs and 65Zn by river sediments in the lower susquehanna river, Environ. Pollut., 63, 137–153, https://doi.org/10.1016/0269-7491(90)90064-J, 1990.
Méndez-García, C., Renteria-Villalobos, M., García-Tenorio, R., and Montero-Cabrera, M. E.: Uranium series isotopes concentration in sediments at San Marcos and Luis L. Leon reservoirs, Chihuahua, Mexico, 1607, 83–92, https://doi.org/10.1063/1.4890707, 2014.
Van Metre, P., Wilson, J., Fuller, C., Callender, E., and Mahler, B.: Collection, Analysis, and Age-Dating of Sediment Cores From 56 U.S. Lakes and Reservoirs Sampled by the U.S. Geological Survey, 1992–2001, U.S. Geol. Surv. Sci. Investig. Rep. 2004–5184, 2004.
Meusburger, K., Evrard, O., Alewell, C., Borrelli, P., Cinelli, G., Ketterer, M., Mabit, L., Panagos, P., van Oost, K. and Ballabio, C.: Plutonium aided reconstruction of caesium atmospheric fallout in European topsoils, Sci. Rep., 10, 11858, https://doi.org/10.1038/s41598-020-68736-2, 2020.
Miller, K. M. and Heit, M.: A time resolution methodology for assessing the quality of lake sediment cores that are dated by 137Cs, Limnol. Oceanogr., 31, 1292–1300, https://doi.org/10.4319/lo.1986.31.6.1292, 1986.
Minoura, K., Yamada, T., Hirano, S., and Sugihara, S.: Movement of radiocaesium fallout released by the 2011 Fukushima nuclear accident, Nat. Hazards, 73, 1843–1862, https://doi.org/10.1007/s11069-014-1171-y, 2014.
Oldfield, F., Appleby, P. G., and Battarbee, R. W.: Alternative 210Pb dating: results from the New Guinea Highlands and Lough Erne, Nature, 271, 339–342, https://doi.org/10.1038/271339a0, 1978.
Pennington, W., Cambray, R. S., Eakins, J. D. and Harkness, D. D.: Radionuclide dating of the recent sediments of Blelham Tarn, Freshw. Biol., 6, 317–331, https://doi.org/10.1111/j.1365-2427.1976.tb01617.x, 1976.
Perner, K., Leipe, T., Dellwig, O., Kuijpers, A., Mikkelsen, N., Andersen, T. J., and Harff, J.: Contamination of arctic Fjord sediments by Pb-Zn mining at Maarmorilik in central West Greenland, Mar. Pollut. Bull., 60, 1065–1073, https://doi.org/10.1016/j.marpolbul.2010.01.019, 2010.
Robbins, J. A. and Herche, L. R.: Models and uncertainty in 210 Pb dating of sediments, SIL Proceedings, 1922–2010, https://doi.org/10.1080/03680770.1992.11900096, 1993.
Sabatier, P., Poulenard, J., Fanget, B., Reyss, J.-L., Develle, A.-L., Wilhelm, B., Ployon, E., Pignol, C., Naffrechoux, E., Dorioz, J.-M., Montuelle, B., and Arnaud, F.: Long-term relationships among pesticide applications, mobility, and soil erosion in a vineyard watershed, P. Natl. Acad. Sci. USA, 111, 15647–15652, https://doi.org/10.1073/pnas.1411512111, 2014.
Sanchez-Cabeza, J. A. and Ruiz-Fernández, A. C.: 210Pb sediment radiochronology: An integrated formulation and classification of dating models, Geochim. Cosmochim. Acta, 82, 183–200, https://doi.org/10.1016/j.gca.2010.12.024, 2012.
Sanchez-Cabeza, J. A., Ani-Ragolta, I., and Masqué, P.: Some considerations of the 210Pb constant rate of supply (CRS) dating model, Limnol. Oceanogr., 45, 990–995, https://doi.org/10.4319/lo.2000.45.4.0990, 2000.
Sanders, C. J., Santos, I. R., Patchineelam, S. R., Schaefer, C., and Silva-Filho, E. V.: Recent 137Cs deposition in sediments of Admiralty Bay, Antarctica, J. Environ. Radioact., 101, 421–424, https://doi.org/10.1016/j.jenvrad.2010.02.006, 2010.
Schindler, M. and Kamber, B. S.: High-resolution lake sediment reconstruction of industrial impact in a world-class mining and smelting center, Sudbury, Ontario, Canada, Appl. Geochem., 37, 102–116, https://doi.org/10.1016/j.apgeochem.2013.07.014, 2013.
Sobocinski, R. W.: Sediment transport in a small stream based on 137 Cs inventories of the bedload fraction, Water Resour. Res., 26, 1177–1187, https://doi.org/10.1029/90WR00239, 1990.
Sun, Q., Liu, D., Liu, T., Di, B., and Wu, F.: Temporal and spatial distribution of trace metals in sediments from the northern Yellow Sea coast, China: Implications for regional anthropogenic processes, Environ. Earth Sci., 66, 697–705, https://doi.org/10.1007/s12665-011-1277-4, 2012.
Swales, A., Williamson, R. B., Van Dam, L. F., Stroud, M. J., and McGlone, M. S.: Reconstruction of urban stormwater contamination of an estuary using catchment history and sediment profile dating, Estuaries, 25, 43–56, https://doi.org/10.1007/BF02696048, 2002.
Syvitski, J., Waters, C. N., Day, J., Milliman, J. D., Summerhayes, C., Steffen, W., Zalasiewicz, J., Cearreta, A., Gałuszka, A., Hajdas, I., Head, M. J., Leinfelder, R., McNeill, J. R., Poirier, C., Rose, N. L., Shotyk, W., Wagreich, M., and Williams, M.: Extraordinary human energy consumption and resultant geological impacts beginning around 1950 CE initiated the proposed Anthropocene Epoch, Commun. Earth Environ., 1, 32, https://doi.org/10.1038/s43247-020-00029-y, 2020.
UNSCEAR: Sources and Effects of Ionizing Radiation, United Nations Scientific Committee on the Effects of Atomic Radiation UNSCEAR 2000 Report to the General Assembly, with Scientific Annexes, 2000.
Urrutia, R., Araneda, A., Cruces, F., Torres, L., Chirinos, L., Treutler, H. C., Fagel, N., Bertrand, S., Alvial, I., Barra, R., and Chapron, E.: Changes in diatom, pollen, and chironomid assemblages in response to a recent volcanic event in Lake Galletué (Chilean Andes), Limnologica, 37, 49–62, https://doi.org/10.1016/j.limno.2006.09.003, 2007.
Wang, X., Yang, H., Gu, Z., Zhang, M., and Yang, B.: A century of change in sediment accumulation and trophic status in Lake Fuxian, a deep plateau lake of Southwestern China, J. Soil. Sediment., 18, 1133–1146, https://doi.org/10.1007/s11368-017-1871-5, 2018.
Wilkinson, M. D., Dumontier, M., Aalbersberg, Ij. J., Appleton, G., Axton, M., Baak, A., Blomberg, N., Boiten, J.-W., da Silva Santos, L. B., Bourne, P. E., Bouwman, J., Brookes, A. J., Clark, T., Crosas, M., Dillo, I., Dumon, O., Edmunds, S., Evelo, C. T., Finkers, R., Gonzalez-Beltran, A., Gray, A. J. G., Groth, P., Goble, C., Grethe, J. S., Heringa, J., 't Hoen, P. A. ., Hooft, R., Kuhn, T., Kok, R., Kok, J., Lusher, S. J., Martone, M. E., Mons, A., Packer, A. L., Persson, B., Rocca-Serra, P., Roos, M., van Schaik, R., Sansone, S.-A., Schultes, E., Sengstag, T., Slater, T., Strawn, G., Swertz, M. A., Thompson, M., van der Lei, J., van Mulligen, E., Velterop, J., Waagmeester, A., Wittenburg, P., Wolstencroft, K., Zhao, J., and Mons, B.: The FAIR Guiding Principles for scientific data management and stewardship, Sci. Data, 3, 160018, https://doi.org/10.1038/sdata.2016.18, 2016.
Wu, X., Bi, N., Kanai, Y., Saito, Y., Zhang, Y., Yang, Z., Fan, D., and Wang, H.: Sedimentary records off the modern Huanghe (Yellow River) delta and their response to deltaic river channel shifts over the last 200 years, J. Asian Earth Sci., 108, 68–80, https://doi.org/10.1016/j.jseaes.2015.04.028, 2015.
Xia, X., Wu, Q., Zhu, B., Zhao, P., Zhang, S., and Yang, L.: Analyzing the contribution of climate change to long-term variations in sediment nitrogen sources for reservoirs/lakes, Sci. Total Environ., 523, 64–73, https://doi.org/10.1016/j.scitotenv.2015.03.140, 2015.
Zeng, H. A. and Wu, J. L.: Sedimentary Records of Heavy Metal Pollution in Fuxian Lake, Yunnan Province, China: Intensity, History, and Sources, Pedosphere, 19, 562–569, https://doi.org/10.1016/S1002-0160(09)60150-8, 2009.
Zhang, H., Shan, B., Ao, L., Tang, W., and Wen, S.: Past atmospheric trace metal deposition in a remote lake (Lake Ngoring) at the headwater areas of Yellow River, Tibetan Plateau, Environ. Earth Sci., 72, 399–406, https://doi.org/10.1007/s12665-013-2960-4, 2014.
Zhao, X., Qiao, J., and Hou, X.: Plutonium isotopes in Northern Xinjiang, China: Level, distribution, sources and their contributions, Environ. Pollut., 265, 114929, https://doi.org/10.1016/j.envpol.2020.114929, 2020.
Zhu, L., Xu, C., Hou, X., Qiao, J., Zhao, Y., and Liu, G.: Determination of Ultratrace Level 135Cs and 135Cs/137Cs Ratio in Small Volume Seawater by Chemical Separation and Thermal Ionization Mass Spectrometry, Anal. Chem., 92, 6709–6718 https://doi.org/10.1021/acs.analchem.0c00688, 2020.