the Creative Commons Attribution 4.0 License.
the Creative Commons Attribution 4.0 License.
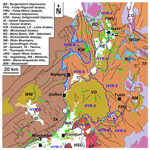
Mineral, thermal and deep groundwater of Hesse, Germany
Rafael Schäffer
Kristian Bär
Sebastian Fischer
Johann-Gerhard Fritsche
Ingo Sass
The composition of mineral, thermal or deep groundwaters is of interest for several geotechnical applications, such as drinking-water supply, spas or geothermal energy. Verified and reliable knowledge of temperature, pH, hydrochemical composition and other parameters is crucial to extract fluids with as few technical problems as possible and exploit groundwater reservoirs economically and environmentally sustainably. However, at sites where empirical data are lacking, the correct prediction of fluid properties is often difficult, resulting in considerable economic risks. Here we present the first comprehensive and publicly available database of mineral, thermal and deep groundwaters of Hesse compiled from published and our own data. Presently, it contains 1035 datasets from 560 different springs or wells sampled since 1810. A dataset consists of metadata like location; altitude; depth; rock type or stratigraphic unit; information on the water type; references; physical–chemical parameters; concentrations of major, minor and trace elements; and content of dissolved and free gases as well as isotope data. The dataset allows the evaluation of time series and distribution of groundwater properties both laterally and vertically. We show a simple statistical evaluation based on the five major hydrogeological regions of Hesse. Our database can be used to re-evaluate genesis and circulation of deep groundwaters, to estimate reservoir temperatures with a solution geothermometer, or to assess groundwater ages by means of isotope data. It can also be useful for a first conception of deep geothermal utilization. In the future, an update and extension of the database is intended.
The database of mineral, thermal and deep groundwaters of Hesse has been made available by Schäffer et al. (2020) in xlsx and csv file format at TUdatalib, https://doi.org/10.25534/tudatalib-340. The second updated version of the database can be accessed directly at https://tudatalib.ulb.tu-darmstadt.de/handle/tudatalib/2508.2 (last access: 20 October 2021).
- Article
(2935 KB) - Full-text XML
- BibTeX
- EndNote
1.1 Motivation
The physical and chemical properties of groundwater determine its suitability for drinking water, thermal water, mineral water, mineral extraction, heat extraction and so on. It also impacts largely its technical utilization since changes in temperature and pressure influence processes like scaling, corrosion or degassing, resulting in further costs for remediation measures (Wolfgramm et al., 2011). In addition, the hydrochemical composition also controls fluid properties like density, heat capacity or viscosity (Stober et al., 2013). Knowledge of the hydrochemistry is therefore imperative in order to foresee technical or economic challenges which might emerge during utilization. For this purpose, we developed the database of mineral, thermal and deep groundwaters of the federal state of Hesse, Germany. It compiles 1035 published hydrochemical datasets from 560 different measurement points in the entire Hessian territory and some adjacent areas. It has been compiled by the TU Darmstadt in close cooperation with the Hessian Agency for Nature Conservation, Environment and Geology (HLNUG) as part of the R & D project Hessen 3D 2.0 (3D modelling of the petrothermal and medium deep geothermal resources for power production, direct heat utilization and storage of the federal state of Hesse) funded by the Federal Ministry for Economic Affairs and Energy (grant number 0325944A).
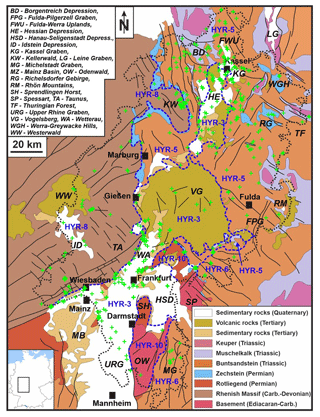
Figure 1Hydrochemical sampling points (green crosses) and hydrochemical regions in the federal state of Hesse. Generalized geological map after Schäffer et al. (2018).
The data have been collected in close cooperation with the Federal Institute for Geosciences and Natural Resources (BGR). This cooperation emerged because the BGR is setting up a comprehensive database containing quality-checked data about formation waters from the entire German territory (Fischer and May, 2019). The BGR currently reviews internal resources (databases, library and archive) and plans to collect data from various external sources including local state authorities, research institutes, universities and industry. Within the formation water database, formation water is defined as groundwater with total dissolved solids (TDS) > 1 g L−1 and/or temperature > 20 ∘C that originates from hydrogeologic entities deeper than 100 The formation water database is being implemented within the GeODin software package (GeODin 2021) and will be made publicly available in the future.
1.2 Geology and hydrogeology of Hesse
Hydrogeological maps of the whole state territory were elaborated by Diederich et al. (1985). Herrmann et al. (2012) developed a conceptual model to evaluate residence times in upper aquifers in Hesse. Fritsche et al. (2003) and Ad-hoc-Arbeitsgruppe Hydrogeologie (2016) divide Hesse into five major hydrogeological regions (HYR, Fig. 1):
-
the Upper Rhine Graben including the Mainz Basin in southern Hesse as well as Cenozoic deposits in northern Hesse with the volcanic rocks of the Vogelsberg (HYR-03),
-
parts of the central German basin range in eastern and northern Hesse, with dominantly Triassic rocks at the surface (HYR-05),
-
parts of the South German Scarplands in south-eastern Hesse (HYR-06),
-
the Rhenish Massif in western and north-western Hesse as part of the Variscan Rhenohercynian zone (HYR-08), and
-
the crystalline Odenwald and Spessart in south-eastern Hesse as part of the Variscan Mid-German Crystalline Rise (HYR-10).
1.2.1 Upper Rhine Graben, Mainz Basin, Hessian Depression and Vogelsberg (HYR-03)
The Quaternary unconsolidated sediments of the Upper Rhine Graben constitute a thick, productive, intensively used pore aquifer. Especially at the eastern margin of the graben, clayey intercalations divide the sequence of sand and gravel into separated aquifers with different pressure levels and varying water quality. In general, TDS increase with depth and some of these highly mineralized waters ascend along permeable fault zones active in the Cenozoic sediments. Groundwater-bearing Tertiary sands and gravels follow underneath, which often alternate with clay-rich layers and can therefore locally form natural gas deposits. Marls, mudstones and limestones of the deeper Tertiary strata feature little but highly mineralized groundwater. Layers of the Pechelbronn Group are oil bearing. The Upper Rhine Graben is well explored by deep drillings due to the hydrocarbon exploration and exploitation since the 1950s. However, the Permian Rotliegend, which forms the base of the Cenozoic graben deposits at approximately 2 to 3.2 km depth and has been drilled by only up to 70 wells so far but has rarely been sampled for its hydrochemistry. Even the most recent well, drilled to a depth of about 4000 m for geothermal energy production in Trebur in 2016 (Reinecker et al., 2019), has not been used for fluid sampling.
Limestone beds and gravel layers within Miocene and Oligocene marls feature fossil groundwater in the northern foothills of the Mainz Basin on the southern edge of the Taunus. To the south, the Taunus Border Fault separates the Rhenish Massif from the Upper Rhine Graben and locally acts as a vertical pathway for the rise of highly mineralized deep waters (Loges et al., 2012), reaching the highest temperatures in the west, e.g. Kochbrunnen in Wiesbaden with more than 65 ∘C (Wagner et al., 2005). The temperatures of mineral waters are at least 30 ∘C lower in the eastern direction: Bad Soden (max 32.0 ∘C), Bad Homburg (max 22.4 ∘C) and Bad Nauheim (max 32.7 ∘C) (Golwer, 2005; Schäffer and Sass, 2016).
South-east of Frankfurt to Aschaffenburg, the Hanau-Seligenstadt Depression, filled by Pleistocene gravels and sands of the river Main and Tertiary unconsolidated rocks, forms an up to 180 m thick sequence of pore aquifers, divided by clayey aquicludes above the Rotliegend. Similar sequences, formed by limnic–fluviatile sands and gravels as well as clays of the Tertiary, exist in the Wetterau, situated in the transition area to the Hessian Depression. The thickness and depth of aquifers and aquicludes can vary greatly as a result of the local block faulting. In deeper Tertiary aquifers, highly mineralized waters with elevated sodium and chloride concentrations occur, rising particularly in the western Wetterau along the eastern, partly covered, extension of the southern Taunus Border Fault (Scharpff et al., 1974). The important mineral water deposits of Bad Vilbel, Rosbach vor der Höhe and Bad Nauheim are located along this fault zone and conclude the line of thermal spas associated with this major fault (Chelius, 1905; Scharpff, 1976; Golwer, 2009).
In central Hesse, the thick sequence of Tertiary volcanic rocks of the Vogelsberg (e.g. basalts, interbedded pyroclastic materials and their weathering products) and the tectonic overprinting of the rock sequence cause a fractured, multi-layered aquifer system (Ehrenberg et al., 1981). Hydrogeologically, the Vogelsberg is subdivided into the zone of suspended aquifers and the zone of continuous groundwater saturation (Leßmann et al., 2001). The Vogelsberg is considered a supra-regional water extraction area and thus of great significance for water management. The volcanic rocks are underlain by Triassic Buntsandstein. The 1000 m deep thermal water well in Herbstein taps mineral water from Zechstein and Rotliegend with a temperature of up to 33 ∘C (Hölting, 1979; Käß and Käß, 2008).
The Hessian Depression is a Cenozoic graben structure filled with thick deposits of Tertiary sediments, which cover the palaeosurface of the Buntsandstein. Usually, the Tertiary strata are rather unproductive aquifers. Poorly permeable rocks of the Upper Buntsandstein (Röt) with thicknesses between 100 and 200 m form the base of this aquifer. Below follows the Middle Buntsandstein aquifer, which is used for drinking-water production. Groundwater recharge mainly occurs through inflow from catchment areas in the west and east outside the Hessian Depression. Tertiary basalts, which cut along fault lines through the sedimentary sequence, form fractured aquifers of low extent and yield. Two circa 1300 m deep boreholes tapped Na–Cl waters in dolomites of the Permian Zechstein with temperatures of nearly 42 ∘C in Kassel-Wilhelmshöhe at the beginning of the 20th century (Udluft, 1969).
1.2.2 Central German basin range (HYR-05)
The central German basin range includes several hydrogeological subareas. Two groundwater levels are distinguished in the Borgentreich Depression and Kassel Graben in northern Hesse. One aquifer exists in the Triassic Muschelkalk and another in the Middle Buntsandstein, and they are largely separated from each other by the aquiclude of the Upper Buntsandstein. The north–south-oriented Leine Graben in the most north-eastern part of Hesse consists of a mosaic of faulted blocks composed by Triassic sediments, largely undisturbed internally. In the Werra-Greywacke Hills between Witzenhausen and Eschwege, shallow groundwater from Palaeozoic rocks already has a high hardness and sulfate concentration due to overlaying carbonate rocks of the Permian Zechstein (Finkenwirth, 1970; Hölting, 1972, 1981). The same accounts for groundwater from siliceous fracture aquifers of the Rotliegend within the Richelsdorfer Gebirge (Richelsdorf Hills) (Fritsche et al., 2001).
The Lower and Middle Buntsandstein dominantly crops out in large parts of north-eastern and eastern Hesse. In this hydrogeological subarea known as the Fulda-Werra Uplands, hydraulically separated groundwater levels are formed locally in individual faulted blocks within the Buntsandstein aquifer (Brinkmann et al., 2014). However, seen on a large scale, these blocks are hydraulically connected. Several roughly north-east–south-west and perpendicularly oriented grabens with fault displacements of several hundred metres interrupt the faulted blocks of the Buntsandstein. In the Rhön Mountains, younger Triassic sediments and Tertiary basaltic rocks partly cover the Buntsandstein. The Zechstein contains salt deposits in stratiform bedding in depths between 200 and 1000 m between Bad Hersfeld and the Thüringer Wald (Thuringian Forest) as well as south of the Fulda–Pilgerzell Graben near Neuhof (Dietz, 1929; Trusheim, 1964; Käding, 2001). The fault tectonics are less pronounced in this area due to the plastic behaviour of the salt deposits. This causes a lower hydraulic permeability of the overlying Triassic strata. Over a large area, clayey sequences within the Zechstein protect these salt deposits from dissolution. Leaching leads to a disintegration of the caprocks only at the edges of the salt slope (the inclined rim of the salt deposit exposed to leaching) or in areas of irregular subrosion depressions, increasing the rise of deep saline groundwater from dolomites of the Leine Formation (Plattendolomit). The Plattendolomit is a well-permeable fracture and/or karst aquifer that bears confined, partly artesian and highly mineralized groundwater of the Na–Cl type (Hölting, 1981; Schäffer et al., 2018). Since the 1920s, it has also served as a horizon for saline wastewater injection from the potash industry in the Werra and Fulda area (Skowronek et al., 1999). Higher mineralized groundwater with saline wastewater components rises from the Plattendolomit and the Lower Buntsandstein to the surface particularly on the salt slope and at shallow depths of the Plattendolomit in river valleys (Skowronek et al., 1999).
1.2.3 South German Scarplands (HYR-06)
In south-eastern Hesse, clastic rocks of the Buntsandstein form a fractured multilevel aquifer divided by clayey intercalations which is used for drinking-water production (Ludwig et al., 2011). In the eastern Odenwald, the Buntsandstein overlies the Zechstein and the basement with increasing thickness towards the east. Muschelkalk is preserved within the Michelstadt Graben and forms a shallow karst aquifer (Becht et al., 2017).
1.2.4 Rhenish Massif (HYR-08)
The Rhenish Massif forms north-western Hesse. It consists of strongly folded, imbricated and widely over-thrusted low-grade metamorphic rock sequences which often dip steeply and are faulted narrowly. The Carboniferous slates in the northern Rhenish Massif have low hydraulic permeability. Of higher permeability are Devonian slates, silicified rocks, and especially greywackes and limestones. The latter can be karstified to the point of forming caves. Zechstein sediments crop out at the eastern rim of the Rhenish Massif, northwards and eastwards of the Kellerwald. The karstified carbonate and anhydrite rocks, which also comprise clastic sediments, form fracture and karst aquifers. The marginal Zechstein facies consisting of siltstones and mudstones dominates south of the Kellerwald. Fracture aquifers of the Buntsandstein dominate further towards the Hessian Depression, containing medium- to coarse-grained sandstones with a few thin layers of mudstone.
Over a large area, the rock sequences in the southern Rhenish Massif produce even less groundwater and are less permeable than in the north. Only the well-fractured Taunus quartzite, constituting the ridge of the Taunus, is used here for large-scale drinking-water extraction, often by long tunnels. Locally, in the area of the Idstein Depression, young extensional faults have also formed a highly permeable fractured aquifer. Especially at the edges of the graben, it has higher permeabilities and storage volumes compared to adjacent areas, although the fractured aquifer consists of the same schists and quartzites. Highly mineralized deep waters rise by CO2 gas lift and form natural mineral springs in fault zones surrounding Oberselters and Niederselters (Carlé, 1975). While Na–Cl-dominated mineral waters rise along the southern Taunus Border Fault, the mineral waters within the Rhenish Massif contain higher proportions of Ca, Mg and HCO3 and are classified as local formations (Carlé, 1958; Thews, 1972; Kirnbauer et al., 2012). The acidulous waters of Selters in the Taunus or the rather low mineralized healing waters of Bad Schwalbach or Schlangenbad are among these (Carlé, 1975; Käß and Käß, 2008). Further north, e.g. in Biskirchen, Leun, waters occur that contain a lot of Ca and Mg. In nearby Löhnberg-Selters, however, Na–Ca–Cl water is extracted.
Lower Carboniferous schists form low-permeability fractured aquifers in the region of the rivers Lahn and Dill in the central Hessian part of the Rhenish Massif. A special characteristic is the use of groundwater collected from old drainage tunnels of abandoned hematite ore mines. They tap large-scale catchment areas where diabases (metavolcanites), greenstones, greywackes and slates are predominant. Of greater hydrogeological and water management importance are the Middle Devonian massive reef limestones, which represent highly permeable fractured and karst aquifers with high storage volumes, e.g. in the Limburg area.
The relatively well permeable Tertiary volcanic (basaltic) rocks of the Westerwald lie in the west of the Lahn and Dill area on top of the Palaeozoic or its Tertiary clayey weathering surface.
1.2.5 Crystalline Odenwald (HYR-10)
The crystalline Odenwald in southern Hesse consists of plutonic igneous and metamorphic rocks – namely granites, diorites, amphibolites, gneisses – which are referred to as fracture aquifers. Significant permeabilities generally only occur in near-surface weathering zones up to a depth of a few tens of metres. Otherwise, the basement is very low to extremely low permeable (Becht et al., 2017). This also applies to the narrow Hessian strip of the crystalline Spessart. In some areas, a weathering layer of rubble is deposited over the bedrock in which a pore aquifer of medium to moderate permeability develops. In the north, Permian Rotliegend of the Sprendlinger Horst covers the crystalline Odenwald. It contains conglomerates, arkoses, sandstones, mudstones, and subordinated limestones and embedded volcanites. Small-scale isolated fracture aquifers, developed mainly in the sandstones and volcanites, are low to very low permeable only.
2.1 Criteria for input data
The HLNUG runs a public database on groundwater and drinking-water protection called GruSchu (GruSchu, 2021). GruSchu includes data of groundwater, raw water, drinking water and curative water provided by waterworks as well as the public measuring station network. Therefore, GruSchu contains information mainly on shallow aquifers. For deeper aquifers it is thus necessary to compile a complementary database on natural mineral, thermal and deep groundwaters. To be included, datasets had to comprise the concentration of major elements (Na+, Ca2+, Mg2+, K+, Cl−, and ) and meet at least one of the following criteria:
-
water temperature of more than 20 ∘C (German definition of thermal water; von Himstedt et al., 1907; Michel, 1997)
-
concentration of total dissolved solids or free carbon dioxide of at least 1 g L−1 (traditional German definition of mineral water; von Himstedt et al., 1907; Michel, 1997)
-
groundwater origin at least 100 m below ground level (definition of the formation water database of the BGR).
Gas composition and isotope data, mainly Δ13C, 14C and Δ34S, were included even without concentrations of major elements. Nine analyses (nos. 439, 440, 463, 464, 479, 480, 781, 928, 975 in the database) located a few kilometres beyond the federal border of Hesse were included to improve the spatial data distribution. Additionally, three datasets are added although they do not meet the criteria mentioned above. The Sossenheimer Sprudel (no. 544) is included due to its location within Frankfurt and its historical significance. Wells Groß-Umstadt V (no. 615) and Mörlenbach Weihrich IV (no. 772) are nearly 100 m deep and represent the crystalline basement of the Odenwald, where data are generally sparse.
The datasets mentioned above are further supplemented by 18 datasets with mean values for rock units occurring in Hesse from Ludwig (2013). Furthermore, 37 datasets from the Baden-Württemberg, Rhineland-Palatinate or French part of the Upper Rhine Graben (URG) from Stober and Jodocy (2011), Stober and Bucher (2015), and Sanjuan et al. (2016) were included since only sparse data from the literature are available in Hesse for the hydrogeological units of the graben fill of the URG and the Mesozoic to Paleozoic units below.
2.2 Literature survey
The database is based almost exclusively on published data archived in the libraries of the TU Darmstadt as well as the BGR. Major sources were the official explanations of geological maps 1:25 000 and periodicals of the HLNUG and its precursor organizations (Geologisches Jahrbuch Hessen, Geologische Abhandlungen Hessen, Abhandlungen des hessischen Landesamtes für Bodenforschung and Notizblatt des Hessischen Landesamtes für Bodenforschung). Of those, the official hydrogeological map series of Hesse 1:300 000 by Diederich et al. (1991) is the one which provided the most data by far. Other main sources were three standard works on mineral and thermal waters of Germany and central Europe (von Himstedt et al., 1907; Carlé, 1975; Käß and Käß, 2008). Other books or journal articles, published technical reports, theses, our own measurements, personal communications, and information on municipal web pages served as secondary data sources. Some data were taken from GruSchu, especially to complement hydrochemical analyses with isotope data.
2.3 Old analyses
Analyses from the 19th century and the beginning of the 20th century are integrated if the analysis is of interest for longer time series; no younger analytical data are available; or they appear trustworthy due to the experience or reputation of a laboratory or an author, e.g. Justus von Liebig (von Liebig, 1839a, c) or Carl Remigius Fresenius (Fresenius, 1886, 1887). Unfortunately, some old analyses had to be excluded because a conversion into contemporary units or species used today (Table 1; cf. Käß and Käß, 2008) could not be carried out correctly or the datasets were too incomplete (e.g. von Liebig, 1839b; Jochheim, 1858; Chelius, 1905). Concentrations in old analyses were often given as salts or acids and not as ions or elements like today. Some authors presented both (e.g. von Himstedt et al., 1907), but normally salt or acid concentrations had to be converted using molar masses. Since that time, approved molar masses have shifted slightly causing a mistake in our recalculation which might be in the range of or below the measurement uncertainty in former times and thus poses no problem here.
2.4 Explanation of database structure
The database is structured in 11 domains: metadata, references; physical–chemical (sum) parameters; chemical parameters, divided into major elements, minor elements and trace elements; dissolved gases; free gases; and isotopes, as well as evaluation and electrical balance, available in the Excel version only (Schäffer et al., 2020).
Table 2Rock types, local stratigraphic units and geological periods after HLUG (2007) and DSK (2016) used for classification in the hydrochemical database. Fm is a contraction of Formation.
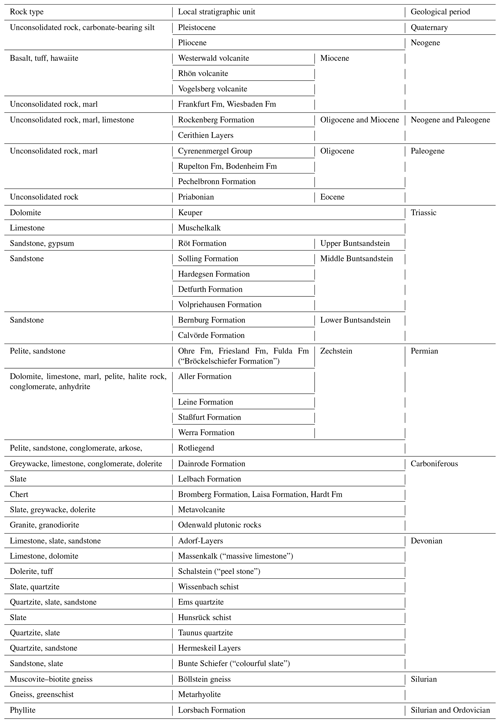
The domain metadata contains information on the location (place), name, position (coordinates), altitude, water-catchment system, geology and water type. Light-grey-shaded fields in the columns referring to coordinates, altitude, final depth and geology mark our own additions of the dataset which were not given in the original reference. Coordinates are given in both the traditional German Gauss–Krüger coordinate system (transverse Mercator projection, DHDN3) and the modern Universal Transverse Mercator (UTM, WGS84) coordinate system. Table 2 summarizes generalized rock types of the aquifer units, which were chosen in accordance with the PetroPhysical Property Database P3 (Bär et al., 2020). Local stratigraphic units and their geological period are chosen in accordance with the stratigraphic table of Germany (DSK, 2016) and the geological overview map of Hesse 1:300 000 (HLUG, 2007). Entries in the field of water type (own information) provide a quick overview of the groundwater chemistry. T, M, B and A stand for thermal water, mineral water, brine and acidulous water, classified in accordance with the definition mentioned in Sect. 2.1. Brines are defined here using the common balneological definition in Germany of at least 14 g L−1 of dissolved sodium and chloride (Fricke and Michel, 1974; Adam et al., 2000). Cations and anions are listed with descending ratios, if their equivalent concentration reaches at least 20 % (Fricke and Michel, 1974; Michel, 1997).
The domain references contains information on the date of analysis, the laboratory or person who executed the analysis, and a citation. If a dataset was found several times in the literature, often with complementary information, multiple citations are given sorted from oldest to youngest. The number of analyses for each spring or well is also given sorted from oldest to youngest. Dark-grey-shaded fields indicate comments or explanations available within the observation column, for example, additional information, contradicting values in different literature sources or missing data.
The domains physical–chemical (sum) parameters and chemical parameters contain the most important parameters for classifying groundwaters. If authors stated that certain ions or elements were measured but found to be below the detection limit they specified, this information is included in the database. Light-grey-shaded fields in columns concerning the electrical balance and major elements mark our own calculations, e.g. bicarbonate from the carbonate hardness or single ions from the electrical balance itself. Total dissolved solids (TDS) are calculated from the sum of all stated concentrations for major, minor and trace elements. The electrical balance considers the concentrations of Na+, K+, Mg2+, Ca2+, Mn, Fe, , , and Cl−, and it is calculated in accordance with the German standard DIN 38402-62 (2014), given in Eq. (1). This includes a factor of 0.5 in the denominator resulting in a twice-as-high deviation of the electrical balance in comparison to the international common formula (e.g. Appelo and Postma, 2007). The electrical balances of 24 analyses deviate more than ± 5 % and are marked with a red filling.
where ΔIB is deviation of the electrical balance, ceq(Kat) is equivalent concentration of the cations and ceq(An) is equivalent concentration of the anions.
The database includes 1035 datasets from 560 different measurement points in the entire Hessian territory and adjacent areas (Fig. 1). Of these, 890 datasets include concentrations of major elements and therefore TDS and electrical balance. The database covers a period of more than 200 years. An analysis of the Schwefelquelle (“Sulfur spring”, no. 520) in Flörsheim from 1810 is the oldest dataset (Thews, 1969). Figure 2 shows the data distribution with respect to depth, temperature, TDS and carbon dioxide content. The depth is classified into four groups (Fig. 2a): 24.6 % (n=259) of the datasets have a depth of 0 to 10 m and are springs, shafts or very shallow boreholes tapping the uppermost aquifer. They are in many cases related to fault zones and therefore releasing water of a deeper origin. Another 30.6 % (n=313) of the datasets belong to the second group (depth > 10 to 100 m), most of them being wells used for drinking-water supply. A limit of 100 m is defined here in accordance with the formation water database of the BGR. The third group (depth > 100 to 400 m) is with 37.3 % (n=386) the largest one. These are mainly wells to supply drinking water, thermal baths or spas, where the limit of 400 m follows the definition of shallow geothermal systems (e.g. PK Tiefe Geothermie, 2007; Sass et al., 2016). The fourth group (deeper than 400 m) consists of 6.9 % (n=71) of the datasets and includes mostly deep spa wells, research drillings or boreholes from hydrocarbon exploration. Borehole Pfungstadt I (no. 853), south-west of Darmstadt, is the well with the deepest hydrochemical analysis (2291 m). In 0.6 % (n=7) of the datasets no information on the depth is available.
In total, 12.7 % (n=131) of the datasets are thermal waters and 70.6 % (n=734) are below 20 ∘C (Fig. 2b). The remaining 16.7 % (n=170) do not have an entry for temperature. A dataset from Kochbrunnen (“Cooking well”) in Wiesbaden has a temperature of 67.3 ∘C and represents the hottest natural thermal water of Hesse. It has 12 entries from 1849 to 2011 (nos. 997–1008) and therefore has the largest number of analyses for one sampling location within the database.
The majority of the datasets, 53.8 % (n=557), fulfil the definition of mineral waters due to their TDS; another 11.3 % (n=117) have sufficient sodium and chloride concentration to be classified as brine in a balneological sense (Fig. 2c). Solebohrung III (“Brine wellbore”) in Bad Karlshafen (no. 59), in the northernmost part of Hesse north of Kassel, shows by far the highest TDS with 301 g L−1. This extreme high concentration is caused by its origin where the brine leaches halite rocks of the Zechstein. Note that 14.0 % (n=145) cannot be defined due to missing hydrochemical data.
In total, 36.5 % (n=378) of the datasets fulfil the definition of mineral waters due to their free carbon dioxide content, also called acidulous waters (Fig. 2d). Georg-Viktor-Quelle B (no. 345) in Bad Wildungen, located between Marburg and Kassel, shows the highest content of free carbon dioxide (< 21.5 mg L−1). Of the datasets, 18.8 % (n=194) have no entries for the carbon dioxide content.
The first step of the statistical evaluation was to match datasets with the individual hydrogeological units of Hesse (see Sect. 1.2) based on their location (i.e. coordinates). The second step was to prepare and condition the bulk database itself. The conditioning included the following steps:
-
Delete datasets with incomplete entries (e.g. missing hydrochemical data).
-
Reduce multiple datasets to only one measurement per location (no time series considered).
-
Delete measurements with electrical balance exceeding an error greater than ± 5 %.
After the conditioning, the database comprised 544 individual datasets. For the statistical evaluation the parameters temperature, pH and TDS as well as major ions (Na+, K+, Ca2+, Mg2+, Cl−, and ) were considered.
Table 3Specific statistical values of major ions and TDS separately listed for the individual hydrogeological regions of Hesse.
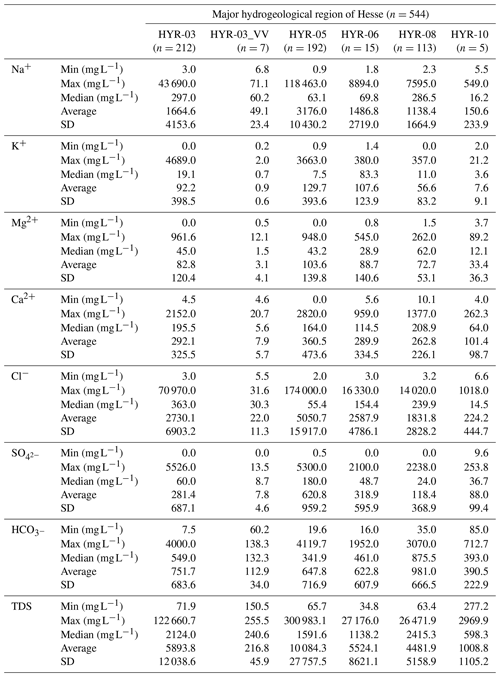
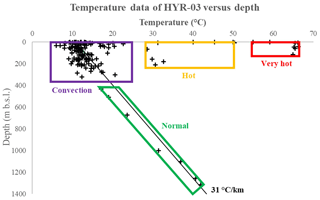
Figure 3Temperature data of HYR-03 plotted against depth. While the majority of data are characterized by convection and/or follow the typical Hessian geothermal gradient (normal), two data clusters of hot and very hot groundwaters are also apparent. Note that the field convection has been constrained rather arbitrarily and is not based on firm scientific evidence.
In total, 219 datasets describe the Upper Rhine Graben and Mainz Basin (HYR-03) including Vogelsberg volcanites (HYR-03_VV; n=7). The pH values of water samples from this group range from 5.7 to 8.2. The average is 6.9 with a standard deviation of 0.6. At 18 locations, the groundwater pH is below 6. These originate from Silurian gneisses and phyllites, Devonian limestones and quartzites, and unconsolidated rocks of the Quaternary. Temperatures within HYR-03 are between 6.0 and 66.3 ∘C (average is 17.6; standard deviation is 13.8). Note that as many as 50 datasets do not have an entry for temperature. Twenty-six datasets have groundwater temperatures above 20.0 ∘C. All but one of these groundwaters show Na+ as the dominating cation, most often in combination with Cl− (pure Na–Cl water type at 19 locations; one location has a Ca–Na–SO4 water type). Within HYR-03, the highest groundwater temperatures above 40 ∘C mainly occur in Silurian gneisses and phyllites of Wiesbaden. By plotting groundwater temperature versus depth (Fig. 3), several distinct subgroups can be differentiated. The largest subgroup is characterized by mixed groundwater due to convection. Convection occurs when hydraulically permeable subsurface pathways, e.g. faults or fractures, allow deeper, connate groundwater to migrate upwards. This groundwater is relatively warm and highly mineralized. Typically, it mixes with colder groundwater of meteoric origin in the shallower subsurface (up to approximately 250 m b.s.l.) and is therefore diluted (e.g. Schäffer et al., 2018). The subgroup “normal” represents groundwater with temperatures roughly following the typical Hessian geothermal gradient (green). One subgroup shows increased groundwater temperatures at or close to the surface (hot, yellow), and the subgroup “very hot” shows groundwater temperatures ≥ 55 ∘C. Groundwater mineralizations (i.e. TDS) of HYR-03 range from 72 to as much as 122 661 mg L−1 (Table 3). The groundwater from location no. 673 (see above) has the maximum TDS with a pronounced Na–Cl water type. This is noteworthy as from this site no halite deposits are known that may explain the high TDS and Na–Cl domination. Because this value is approximately twice as high as the second highest (64 396 mg L−1), it is considered an outlier and hence excluded from further statistics. Groundwater with TDS concentrations above 10 000 mg L−1 (n=29) show a pronounced Na–Cl dominance. These high mineralizations mainly occur in unconsolidated rocks of the Neogene (n=10) as well as in Permian Zechstein (n=5) and Devonian limestones (n=4). TDS concentrations below 1000 mg L−1 are dominated by Ca2+ and . These mainly occur in groundwater from Triassic Buntsandstein, Quaternary and Tertiary aquifers. No depth–TDS correlation is apparent.
Groundwater from the Vogelsberg volcanites (HYR-03_VV; n=7) are characterized by increased pH values between 8.0 and 9.9 and low mineralizations between 151 and 256 mg L−1 (Table 3). Chemically, Na+ and are the major dissolved ions, and the groundwater of HYR-03_VV represents Na–HCO3–Cl water types (one Ca–Mg–HCO3).
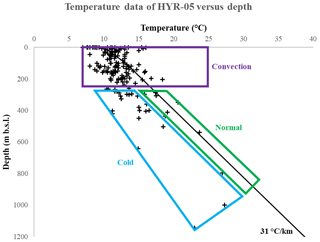
Figure 4Temperature data of HYR-05 plotted against depth. Similarly to HYR-03 (Fig. 3) the majority of data from HYR-05 are also characterized by convection and/or follow the typical Hessian geothermal gradient. However, as opposed to HYR-03, neither hot nor very hot groundwater clusters are apparent. Instead, HYR-05 shows a data cluster of cold groundwaters with temperatures below the typical Hessian geothermal gradient. Note that the field convection has been constrained rather arbitrarily and is not based on firm scientific evidence.
The central German basin range (HYR-05) comprises 192 datasets. Groundwater temperatures are between 7.0 and 27.3 ∘C. Note that 21 datasets have no temperature entries. At five locations groundwater temperatures are above 20 ∘C. These originate from depths greater than 100 and mainly occur in Permian formations. In Fig. 4 depth–temperature dependence for the groundwater of HYR-05 is displayed. While a large subgroup of groundwater is characterized by convection (purple) and another subgroup roughly follows the typical Hessian geothermal gradient (normal, green) similarly to groundwater of HYR-03, one new features is apparent. There is one subgroup with groundwater temperatures below the geothermal gradient (cold, blue).
The pH values of samples from HYR-05 are between 5.2 and 9.3 (average is 6.8; standard deviation is 0.7). Increased pH values above eight (n=4) are found in groundwater from Permian Rotliegend and Rhön volcanite. These datasets are dominated by Na+ and . Lower pH values below 6 typically occur in Triassic sandstones and Permian rock series. Note that Schlossquelle in Bad Wildungen (no. 361) has a pH of 6.0 and a Mg–Ca–Na–HCO3 water type reflecting Mg2+ domination. A total number of 67 datasets do not have an entry for pH. Mineralization ranges from 65.7 to 300 983 mg L−1 (Table 3). The average is 10 084 mg L−1 (note the median of 1592 here) with a standard deviation of 27 758 mg L−1. Hence, HYR-05 has the highest TDS average of the five major hydrogeological regions of Hesse. Groundwater with TDS contents above 10 000 mg L−1 (n=40) are all characterized by Na–Cl water types. At TDS values above 50 000 mg L−1 (n=9) groundwater mainly occur in Zechstein formations. Lower mineralization below 1000 mg L−1 (n=76) relates to groundwater from sandstone of the Buntsandstein. These groundwaters are characterized by Ca–Mg–HCO3 water types. A correlation of TDS with depth is not evident for HYR-05, whereas in northern and eastern Hesse, deep waters from the Buntsandstein are usually mixed by ascending leachate waters of the Zechstein, especially next to the salt slope.
The South German Scarplands in south-eastern Hesse (HYR-06) comprise 15 groundwater samples mainly from Buntsandstein and Zechstein formations. Temperatures are between 10.0 and 20.5 ∘C (four datasets do not have temperature values). Only one groundwater has a temperature above 20.0 ∘C; the remainder are between 10.0 and 14.0 ∘C. pH values range from 6.0 to 7.3, with seven samples having no pH entry. Mineralization ranges from 34.8 to 27 176.0 mg L−1 (Table 3). The average TDS value is 5524 mg L−1, and the standard deviation is 8621 mg L−1. The lowest mineralization occurs in groundwater from formations of the Middle Buntsandstein. These typically have a Ca–HCO3(–Cl) water type. TDS values above 10 000 mg L−1 are Na–Cl dominated; the Martinusquelle in Bad Orb additionally shows a significant influence of 2100 mg L−1. Here it is noteworthy that in Bad König three samples show a Ca–K–HCO3–Cl water type. These are the only datasets of the entire database with K+ as the co-dominating cation. Among the thermal waters of Hesse, Bad König is the only location where thermal water from granites is extracted. These granites – presumably mica (i.e. muscovite) – may explain the high content of dissolved K+.
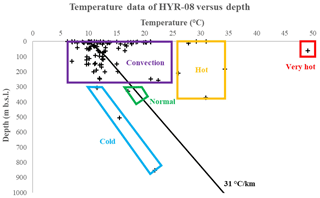
Figure 5Temperature data of HYR-08 plotted against depth. Next to the clusters hot and very hot HYR-08 also shows the cluster cold. Only one groundwater shows a normal temperature in accordance with the typical Hessian geothermal gradient. Note that the field convection has been constrained rather arbitrarily and is not based on firm scientific evidence.
The Rhenish Massif in western and north-western Hesse (HYR-08) comprises 113 datasets in total. The depth interval is between 0 and 853 Groundwater temperatures within HYR-08 range from 6.2 to 49.1 ∘C; the average is 13.9 ∘C with a standard deviation of 7.2 (eight cases of no data). By far the highest groundwater temperature of 49.1 ∘C is from Schützenhofquelle (no. 1019) in Wiesbaden (Fig. 5, subgroup very hot). Increased temperatures between 20.0 and 34.3 ∘C are mainly found both in Silurian–Ordovician phyllites and gneisses (n=9) and in Devonian rocks (n=4). Temperatures below 10.0 ∘C are typical of Devonian schists (e.g. Hunsrück and Wissenbach schist). While the majority of groundwaters belong to the subgroup “convection”, one subgroup shows increased groundwater temperatures partially at or close to the surface (“hot”). Analogous to HYR-05, a subgroup with groundwater temperatures below the geothermal gradient (“cold”) is apparent in Fig. 5.
The pH values of HYR-08 are between 5.4 and 8.0; the average is 6.2. Note that 51 datasets do not have a pH entry. As many as 35 datasets show a pH value below 6. These mainly belong to Silurian–Ordovician gneisses and phyllites (n=18) and Devonian schists (n=11).
Groundwater mineralization within HYR-08 ranges from 53.0 to 26 471.9 mg L−1. The average TDS value is 4479.0 mg L−1 with a standard deviation of 5161.6 mg L−1 (Table 3). It is noteworthy here that location no. 509 has the lowest mineralization of 53.0 mg L−1 of which 16.8 mg L−1 is H2SiO3. The highest mineralization above 10 000 mg L−1 (n=16) mainly occurs in groundwater from Silurian metavolcanites (n=6) as well as Silurian–Ordovician phyllites (n=7). These groundwaters show a pronounced Na–Cl water type. The lowest mineralization below 1000 mg L−1 are Ca–Mg–HCO3 dominated. These low TDS are typical of groundwater of Devonian schists and Triassic–Devonian sandstones. Two datasets of HYR-08 show groundwater with as the main anion. These are from the Permian (Zechstein) lithologies and show Ca–SO4 and Ca–Mg–SO4 water types. No correlation with depth is apparent for TDS.
The crystalline Odenwald in south-eastern Hesse as part of the south-western German basement (HYR-10) comprises five datasets: one each from Rotliegend (arkose), Zechstein (?) and Paleogene (marl), as well as two from sandstones of the Buntsandstein (S3 and S3–S6). Groundwater temperatures range from 10.9 to 18.0 ∘C (Tmax from Rotliegend). pH values are between 6.7 and 7.3 (two datasets without entry). Compared with the other major hydrogeological regions of Hesse, mineralization of HYR-10 is rather low, ranging from 277 to 2970 mg L−1 (Table 3). It is noteworthy that all the five groundwaters have different water types.
The database of mineral, thermal and deep groundwaters of Hesse has been made available by Schäffer et al. (2020) in xlsx and csv file format at TUdatalib, https://doi.org/10.25534/tudatalib-340. The second updated version of the database can be accessed directly at https://tudatalib.ulb.tu-darmstadt.de/handle/tudatalib/2508.2 (Schäffer et al., 2021).
The hydrochemical composition of groundwaters and related fluid properties are of great importance for the planning and trouble-free operation of geothermal power plants or direct heat utilization as well as for understanding hydrochemical processes in aquifers. Here, we describe the first publicly available data compilation of thermal, mineral and deep groundwater of Hesse. We compiled and evaluated hydrochemical data in a database for all geological units that are assessed as suitable for deep geothermal use. Through extensive literature research, it was possible to compile a dataset that thoroughly covers the Hessian state territory, both spatially and chronologically. In the near future, a major improvement of data accessibility is expected after implementation of the Geologiedatengesetz (Geological Data Act) passed in 2020. It transforms private into public data and allows state authorities to publish data which have been rated as classified so far. The HLNUG has thousands of documents, reports, statements, etc., which could not be evaluated and used up to now due to confidentiality and property rights. Thus, an update and extension of the database is planned, and contributions of other people are welcome.
The database can serve as a basis or tool for other projects or scientific questions. It can be used to estimate reservoir temperatures with solution geothermometers. In the best case, geothermometers can be used to determine reservoir temperatures for individual aquifers of the entire Hessian territory. The database can be also used to re-evaluate the genesis and circulation of deep groundwaters. In addition, the isotope data can be used for calculating groundwater ages or evaluating residence times, which in turn are criteria for the search of a repository for radioactive waste (StandAG, 2017). Furthermore, the hydrochemical database will be integrated by the BGR into the formation water database covering the entire German territory.
IS was involved in the conceptualization and funding acquisition. JGF organized data from the HLNUG and wrote Sect. 1.2. KB was responsible for conceptualization, funding acquisition and project administration and wrote Sect. 1.1. RS collected data from the library of the TU Darmstadt; compiled the data and developed the database; wrote Sects. 2, 4 and 5; and contributed to Sect. 3. SF collected data from the library of the BGR, performed the formal analysis and wrote Sect. 3 as well as contributed to Sect. 1.1. All authors conducted an internal review of the manuscript.
The contact author has declared that neither they nor their co-authors have any competing interests.
Publisher's note: Copernicus Publications remains neutral with regard to jurisdictional claims in published maps and institutional affiliations.
This publication is part of the research project “3D modelling of the petrothermal and medium deep geothermal resources for power production, direct heat utilization and storage of the federal state of Hesse” funded by the Federal Ministry for Economic Affairs and Energy (BMWi).
Lena Muhl, Sofia Nalbadi and Petra Kraft helped to collect and review the literature, transferred the data or our own sources into the database, and suggested restructures of the database where needed. Renate Senner (HLNUG) gave useful advice on the groundwater database of Hesse (GruSchu). Christoph Kludt (HLNUG) provided a data collection of selected mineral and medicinal waters of Hesse. We also appreciate the help and support of Marion Homann and the library team at Geozentrum Hannover (i.e. BGR) during the data search. We thank all contributors and also our external cooperation partners for their support and work to fill the database with valuable data or for providing valuable reports or publications to be included in the compilation. We thank Mirko Mälicke and the two anonymous reviewers for the constructive and helpful comments which improved this paper substantially.
This research has been supported by the Bundesministerium für Wirtschaft und Energie (grant no. 0325944).
This paper was edited by Giulio G. R. Iovine and reviewed by Mirko Mälicke and two anonymous referees.
Ad-hoc-Arbeitsgruppe Hydrogeologie (ed.): Regional Hydrogeology of Germany: Aquifers in Germany, their distribution and properties, Geologisches Jahrbuch Reihe A, 163, 1–452, 2016.
Adam, C., Gläßer, W., and Hölting, B.: Hydrogeologisches Wörterbuch, Enke, Stuttgart, Germany, 311 pp., 2000.
Appelo, C. A. J. and Postma, D.: Geochemistry, groundwater and pollution, 2nd edn., Balkema Publishers, Leiden, 649 pp., 2007.
Bär, K., Reinsch, T., and Bott, J.: The PetroPhysical Property Database (P3) – a global compilation of lab-measured rock properties, Earth Syst. Sci. Data, 12, 2485–2515, https://doi.org/10.5194/essd-12-2485-2020, 2020.
Becht, A., Diehl, M., Friedrich, R., Fritsche, J.-G., Hergesell, M., Hoselmann, C., Hug-Diegel, N., Kämmerer, D., Kött, A., Leßmann, B., Lorenz-Hefner, C., Ludwig, F., Marold, U., Nesbor, H.-D., von Pape, W.-P., Pöschl, W., Prein, A., Rückert, H., Schneider, M. W., Senner, R., and Vero, G.: Hydrogeologie von Hessen – Odenwald und Sprendlinger Horst, Grundwasser in Hessen, 2, 1–136, 2017.
Brinkmann, P. J., Leßmann, B., and Matzer, S.: “Typical” description of a hydrogeological system in the Bunter Sandstone, Grundwasser, 19, 181–188, https://doi.org/10.1007/s00767-014-0256-6, 2014.
Carlé, W.: Rezente und fossile Mineral- und Thermalwässer im Oberrheintal-Graben und seiner weiteren Umgebung, Jahresberichte und Mitteilungen des Oberrheinischen Geologischen Vereines NF, 40, 77–105, 1958.
Carlé, W.: Die Mineral- und Thermalwässer von Mitteleuropa – Geologie, Chemismus, Genese, Wissenschaftliche Verlagsgesellschaft, Stuttgart, 643 pp., 1975.
Chelius, C.: Geologischer Führer durch den Vogelsberg, seine Bäder und Mineralquellen – Roths illustrierte Führer Nr. 6, Roth, Gießen, 122 pp., 1905.
Diederich, G., Finkenwirth, A., Hölting, B., Kaufmann, E., Rambow, D., Scharpff, H.-J., Stengel-Rutkowksi, W., and Wiegand, K.: Erläuterungen zu den Übersichtskarten 1:300 000 der Grundwasserergiebigkeit, der Grundwasserbeschaffenheit und der Verschmutzungsempfindlichkeit des Grundwassers von Hessen, Geologische Abhandlungen Hessen, 87, 1–51, 1985.
Diederich, G., Finkenwirth, A., Hölting, B., Kaufmann, E., Rambow, D., Scharpff, H.-J., Stengel-Rutkowksi, W., and Wiegand, K.: Hydrogeologisches Kartenwerk Hessen 1:300 000, Geologische Abhandlungen Hessen, 95, 1–83, 1991.
Dietz, C.: Überblick über die Salzlagerstätte des Werra-Kalireviers und Beschreibung der Schächte “Sachsen-Weimar“ und “Hattorf“, German J. Geol., 80, 68–93, 1929.
DIN 38402-62: German standard methods for the examination of water, waste water and sludge – General information (group A) – Part 62: Plausibility check of analytical data by performing an ion balance (A 62), Beuth, Berlin, 10 pp., 2014.
DSK: Stratigraphic Table of Germany 2016, 2nd edn., German Stratigraphic Commission, Potsdam, available at: http://www.stratigraphie.de/std/Bilder/5_2.pdf (last access: 26 November 2020), 2016.
Ehrenberg, K.-H., Fromm, K., Grubbe, K., Harre, W., Hentschel, G., Hölting, B., Holtz, S., Kreutzer, H., Meisl, S., Nöring, F., Plaumann, S., Pucher, R., Strecker, G., Sušić, M., and Zschau, H.-J.: Forschungsbohrung im Hohen Vogelsberg (Hessen) – Bohrung 1 (Flösser-Schneise) – Bohrung 2/2A (Hasselborn), Geologische Abhandlungen Hessen, 81, 1–166, 1981.
Finkenwirth, A.: Hydrogeologische Neuerkenntnisse in Nordhessen, Notizblatt des hessischen Landesamtes für Bodenforschung, 98, 212–233, 1970.
Fischer, S. and May, F.: Die Formationswasser-Datenbank der BGR: Datengrundlage für geotechnische Nutzungen des tieferen Untergrunds, Tagungsband der 81. Tagung der Norddeutschen Geologen, 11–14 June 2019, Sangerhausen, Germany, in: Geowissenschaftliche Mitteilungen von Thüringen, Beiheft 12, Jena, Germany, 22–23, 2019.
Fresenius, R.: Neue chemische Untersuchung des Kochbrunnens zu Wiesbaden und Vergleichung der Resultate mit den 1849 von mir erhaltenen, Jahrbücher des Nassauischen Vereins für Naturkunde, 39, 1–20, 1886.
Fresenius, R.: Chemische Untersuchungen der kleinen Schützenhofquelle zu Wiesbaden, Jahrbücher des Nassauischen Vereins für Naturkunde, 40, 14–27, 1887.
Fricke, K. and Michel, G.: Mineral- und Thermalwässer der Bundesrepublik Deutschland, Der Mineralbrunnen, 24, 70–89, 1974.
Fritsche, H.-G., Becker, F., and Heggemann, H.: Die Tiefbohrung Rotenburg HKZ 1001 – Geologie des Deckgebirges und hydrogeologische Ergebnisse, Geologisches Jahrbuch Hessen, 128, 5–17, 2001.
Fritsche, H.-G., Hemfler, M., Kämmerer, D., Leßmann, B., Mittelbach, G., Peters, A., Pöschl, W., Rumohr, S., and Schlösser-Kluger, I.: Beschreibung der hydrogeologischen Teilräume von Hessen gemäß EU-Wasserrahmenrichtlinie (EU-WRRL), Geologisches Jahrbuch Hessen, 130, 5–19, 2003.
GeODin: https://www.geodin.com/english/, last access: 8 September 2021.
Golwer, A.: Die Heilquellen von Bad Soden am Taunus, Geologisches Jahrbuch Hessen, 132, 5–32, 2005.
Golwer, A.: Hydrogeologie, in: Erläuterungen zur Geologischen Karte von Hessen 1:25 000, Blatt 5817 Frankfurt am Main West, edited by: Kümmerle, E. and Seidenschwann, G., Hessisches Landesamt für Umwelt und Geologie, Wiesbaden, 109–192, 2009.
GruSchu: http://gruschu.hessen.de/mapapps/resources/apps/gruschu/index.html?lang=de, last access: 20 October 2021.
Herrmann, F., Berthold, G., Fritsche, J.-G., Kunkel, R., Voigt, H.-J., and Wendland, F.: Development of a conceptual hydrogeological model for the evaluation of residence times of water in soil and groundwater: the state of Hesse case study, Germany, Environ. Earth Sci., 67, 2239–2250, https://doi.org/10.1007/s12665-012-1665-4, 2012.
HLUG: Geologische Übersichtskarte von Hessen 1:300 000, 5th edn., Hessisches Landesamt für Umwelt und Geologie, Wiesbaden, 2007.
Hölting, B.: Ein in Wetter/Krs. Marburg (Hessen) erschlossenes Na2-SO4-haltiges Mineralwasser und die Genese solcher Wässer, Notizblatt des hessischen Landesamtes für Bodenforschung, 100, 260–283, 1972.
Hölting, B.: Neue Mineralwasservorkommen in Hessen, Heilbad und Kurort, 31, 84–93, 1979.
Hölting, B.: Vorkommen und Verbreitung von tiefen Grundwässern des Na2-SO4-Typs in Hessen, Geologisches Jahrbuch Hessen, 109, 139–146, 1981.
Jochheim, P.: Die Mineralquellen des Grossherzogthums Hessen, seiner Enclaven und der Landgrafschaft Hessen-Homburg, Ferdinand Enke Verlag, Erlangen, 139 pp., 1858.
Käding, K.-C.: Die Werra-Fulda-Kalilagerstätte – 100 Jahre Förderung, Kali und Steinsalz, 14, 23–28, 2001.
Käß, W. and Käß, H.: Deutsches Bäderbuch – Zweite Auflage, Schweizerbart, Stuttgart, 1232 pp., 2008.
Kirnbauer, T., Wagner, T., Taubald, H., and Bode, M.: Post-Variscan hydrothermal vein mineralization, Taunus, Rhenish Massif (Germany): Constraints from stable and radiogenic isotope data, Ore Geol. Rev., 48, 239–257, https://doi.org/10.1016/j.oregeorev.2012.03.010, 2012.
Leßmann, B., Wiegand, K., and Scharpff, H.-J.: Die Hydrogeologie des vulkanischen Vogelsberges, Geologische Abhandlungen Hessen, 108, 3–144, 2001.
Loges, A., Wagner, T., Kirnbauer, T., Göb, S., Bau, M., Berner, Z., and Markl, G.: Source and origin of active and fossil thermal spring systems, northern Upper Rhine Graben, Germany, Appl. Geochem., 27, 1153–1169, https://doi.org/10.1016/j.apgeochem.2012.02.024, 2012.
Ludwig, F.: Geogene Hintergrundwerte der Hauptbestandteile und Spurenstoffe in hessischen Grundwässern, Geologische Abhandlungen Hessen, 118, 1–165, 2013.
Ludwig, F., Stober, I., and Bucher, K.: Hydrochemical Groundwater Evolution in the Bunter Sandstone Sequence of the Odenwald Mountain Range, Germany: A Laboratory and Field Study, Aquat. Geochem., 17, 165–193, https://doi.org/10.1007/s10498-010-9118-8, 2011.
Michel, G.: Mineral- und Thermalwässer – Allgemeine Balneologie, Lehrbuch der Hydrogeologie, vol. 7, edited by: Matthess, G., Gebrüder Borntraeger, Berlin/Stuttgart, 398 pp., 1997.
PK Tiefe Geothermie: https://www.infogeo.de/Infogeo/DE/Downloads/tiefe_geothermie_definition_08022007.pdf (last access: 8 September 2021), 2007.
Reinecker, J., Hochschild, T., Kraml, M., Löschan, G., and Kreuter, H.: Experiences and challenges in geothermal exploration in the Upper Rhine Graben, in: Proceedings of the European Geothermal Congress, The Hague, The Netherlands, 11–14 June 2019, 1–8, 2019.
Sanjuan, B., Millot, R., Innocent, C., Dezayes, C., Scheiber, J., and Brach, M.: Major geochemical characteristics of geothermal brines from the Upper Rhine Graben granitic basement with constraints on temperature and circulation, Chem. Geol., 428, 27–47. https://doi.org/10.1016/j.chemgeo.2016.02.021, 2016.
Sass, I., Brehm, D., Coldewey, W. G., Dietrich, J., Klein, R., Kellner, T., Kirschbaum, B., Lehr, C., Marek, A., Mielke, P., Müller, L., Panteleit, B., Pohl, S., Porada, J., Schiessl, S., Wedewardt, M., and Wesche, D.: Shallow geothermal systems – recommendations on design, construction, operation and monitoring, Ernst & Sohn, Berlin, 312 pp., 2016.
Schäffer, R. and Sass, I.: Migration and mixing of a carbon dioxide bearing thermal brine in shallow aquifers, Bad Nauheim, Germany, Grundwasser, 21, 305–319, https://doi.org/10.1007/s00767-016-0341-0, 2016.
Schäffer, R., Bär, K., and Sass, I.: Multimethod exploration of the hydrothermal reservoir in Bad Soden-Salmünster, Germany, German J. Geol., 169, 311–333, https://doi.org/10.1127/zdgg/2018/0147, 2018.
Schäffer, R., Bär, K., Fischer, S., Fritsche, J.-G., and Sass, I.: Database of Mineral, Thermal and Deep Groundwaters of Hesse, Germany, Technical University of Darmstadt, Darmstadt, Germany [data set], https://doi.org/10.25534/tudatalib-340, 2020.
Schäffer, R., Bär, K., Fischer, S., Fritsche, J.-G., and Sass, I.: Database of Mineral, Thermal and Deep Groundwaters of Hesse, Germany, version 1.1, Technical University of Darmstadt, Darmstadt, Germany [data set], available at: https://tudatalib.ulb.tu-darmstadt.de/handle/tudatalib/2508.2, last access: 20 October 2021.
Scharpff, H.-J.: Verbreitung und Dynamik der mineralwasserbeeinflußten Grundwässer der Wetterau/Hessen, Abhandlungen des hessischen Landesamtes für Bodenforschung, 70, 103–158, 1974.
Scharpff, H.-J.: Hydrogeologie, in: Erläuterungen zur Geologischen Karte von Hessen 1:25 000 Blatt 5618 Friedberg, edited by: Kümmerle, E., Hessisches Landesamt für Bodenforschung, Wiesbaden, 115–150, 1976.
Skowronek, F., Fritsche, J.-G., Aragon, U., and Rambow, D.: Die Versenkung und Ausbreitung von Salzabwasser im Untergrund des Werra-Kaligebietes, Geologische Abhandlungen Hessen, 105, 1–83, 1999.
StandAG: Standortauswahlgesetz vom 5. Mai 2017 (BGBl. I S. 1074), das zuletzt durch Artikel 1 des Gesetzes vom 7. Dezember 2020 (BGBl. I S. 2760) geändert worden ist, Deutscher Bundestag, Bonn, Germany, 2017.
Stober, I. and Bucher, K.: Hydraulic and hydrochemical properties of deep sedimentary reservoirs of the Upper Rhine Graben, Europe, Geofluids, 15, 464–482, https://doi.org/10.1111/gfl.12122, 2015.
Stober, I. and Jodocy, M.: Hydrochemical characteristics of deep seated waters in the Upper Rhine Graben – Basic information for geothermal energy, J. Geol. Sci., 39, 39–57, 2011.
Stober, I., Jodocy, M., and Burisch, M.: Consequences of the physical properties of deep waters for the thermal capacity of geothermal facilities and for aquifer properties, J. Geol. Sci., 41, 9–20, 2013.
Thews, J.-D.: Hydrogeologie, in: Erläuterungen zur Geologischen Karte von Hessen 1:25 000 Blatt 5916 Hochheim am Main, edited by: Kümmerle, E. and Semmel, A., Hessisches Landesamt für Bodenforschung, Wiesbaden, 109–144, 1969.
Thews, J.-D.: Zur Typologie der Grundwasserbeschaffenheit im Taunus und Taunusvorland, Abhandlungen des hessischen Landesamtes für Bodenforschung, 63, 1–42, 1972.
Trusheim, F.: Über den Untergrund Frankens – Ergebnisse von Tiefbohrungen in Franken und Nachbargebieten 1953–1960, Geol. Bavarica, 54, 1–92, 1964.
Udluft, H.: Hydrogeologie, in: Erläuterungen zur Geologischen Karte von Hessen 1:25 000 Blatt 4622 Kassel-West, edited by: Rösing, F., Hessisches Landesamt für Bodenforschung, Wiesbaden, 140–148, 1969.
von Himstedt, F., Hintz, E., Grünhut, L., Jacobj, C., Kauffmann, H., Keilhack, K., Kionka, H., Kraus, F., Kremser, V., Nicolas, P., Paul, T., Röchling, F., Scherrer, A., Schütze, C., Winckler, A., Rost, E., Sonntag, G., and Auerbach, F.: Deutsches Bäderbuch, edited by: Kaiserliches Gesundheitsamt, Verlag von J. J. Weber, Leipzig, 535 pp., 1907.
von Liebig, J.: Untersuchungen der Mineralquellen zu Soden und Bemerkungen über die Wirkung der Salze auf den Organismus, Annalen der Pharmacie, 30, 4–19, https://doi.org/10.1002/jlac.18390300103, 1839a.
von Liebig, J.: Chemische Untersuchung der Mineralquelle zu Schwalheim bei Friedberg, Annalen der Pharmacie, 31, 59–61, https://doi.org/10.1002/jlac.18390310106, 1839b.
von Liebig, J.: Zusammensetzung der Mineralquelle No. XIX in Soden, Annalen der Pharmacie, 31, 61–62. https://doi.org/10.1002/jlac.18621230310, 1839c.
Wagner, T., Kirnbauer, T., Boyce, A. J., and Fallick, A. E.: Barite–pyrite mineralization of the Wiesbaden thermal spring system, Germany: a 500-kyr record of geochemical evolution, Geofluids, 5, 124–139, 2005.
Wolfgramm, M., Rauppach, K., and Thorwart, K.: New Mineral Formations and Transport of Particles in the Thermal Water Loop of Geothermal Plants in Germany, J. Geol. Sci., 39, 213–239, 2011.