the Creative Commons Attribution 4.0 License.
the Creative Commons Attribution 4.0 License.
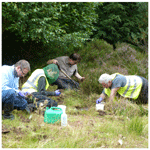
Element and radionuclide concentrations in soils and wildlife from forests in north-eastern England with a focus on species representative of the ICRP's Reference Animals and Plants
Catherine L. Barnett
Nicholas A. Beresford
Michael D. Wood
Maria Izquierdo
Lee A. Walker
Ross Fawkes
There are international recommendations that the environment (i.e. wildlife) is assessed for the potential impact of releases of ionizing radiation. The transfer of radionuclides to wildlife from media (e.g. soil, water) is usually described using the whole-organism concentration ratio (CRwo-media), and a number of assessment models use these values to estimate radiation exposure and risk to wildlife; however, there are many gaps in knowledge. This paper describes a study conducted in 2015–2016 to sample terrestrial wildlife, soil and water from two forests in north-eastern England. Sampling was targeted towards species representative of the International Commission on Radiological Protection's (ICRP) terrestrial Reference Animals and Plants (RAPs): Wild Grass (Poaceae family), Pine Tree (Pinaceae family), Earthworm (Lumbricidae family), Bee (Apidae family), Rat (Muridae family), Deer (Cervidae family) and Frog (Ranidae family); opportunistic sampling of plant and fungi species was also conducted. The dataset comprises stable-element concentrations for 30 elements, radionuclide activity concentrations for K-40 and Cs-137, and radionuclide and stable-element concentration ratios. These data have significantly increased the number of CRwo-media values available for the ICRP RAPs and will contribute to the development of the databases underpinning the ICRP's environmental protection framework. Data will be included in the international database of wildlife transfer parameters for radioecological models and hence are likely to contribute to model developments in the future.
All data and supporting documentation are freely available from the Environmental Information Data Centre (EIDC; https://eidc.ac.uk/, last access: 13 November 2020) under the terms and conditions of the Open Government Licence (Barnett et al., 2020 https://doi.org/10.5285/8f85c188-a915-46ac-966a-95fcb1491be6).
- Article
(717 KB) - Full-text XML
- BibTeX
- EndNote
Over the last 20 years there has been a step change in international radiological protection, requiring assessment of environmental (i.e. wildlife) impacts of ionizing radiation releases rather than assuming that protecting humans ensures protection of other species (ICRP, 2007; IAEA, 2014a). Consequently, various assessment models have been developed to estimate radiation exposure and risk to wildlife (e.g. Beresford et al., 2008a; Brown et al., 2016). These models require an approach to estimate radionuclide activity concentration in organisms if this is unknown (Beresford et al., 2008b). The transfer of radionuclides to wildlife is usually described using the whole-organism concentration ratio (CRwo-media), where, in the case of terrestrial organisms, CRwo-soilis estimated as follows:
Compilations of CRwo-media values have been published by the International Atomic Energy Agency (IAEA, 2014b) and the International Commission on Radiological Protection (ICRP, 2009). These data compilations were facilitated by the creation of an on-line database of CRwo-media values (Copplestone et al., 2013; http://www.wildlifetransferdatabase.org/, last access: 1 June 2020), which has continued to be updated (Brown et al., 2016). The available models and data compilations typically use a system of simplified (often called “reference”) organisms (e.g. transfer data maybe collated for generic mammals, fish, trees etc.). Even so, there are many organism–radionuclide combinations requiring CRwo-media values and large gaps in our knowledge. For instance, there were only data available for about 50 % of the 1500 CRwo-media values required to populate the most recent version of the ERICA Tool (Brown et al., 2016); data are especially sparse for the ICRP's Reference Animals and Plant (RAPs; ICRP, 2008) (ICRP, 2009) and some elements (IAEA, 2014b). As one approach to fill data gaps, the ICRP (ICRP, 2009) suggested selecting a series of sites from which samples of as many RAPs as possible could be collected; this has subsequently been referred to as the “Reference Site” concept (Thørring et al., 2016). In Barnett et al. (2014), we presented the first such study (together with the accompanying dataset, Barnett et al., 2013), which was conducted in north-western England; subsequently studies have been published for sites in Spain, Norway and the Ukrainian Chernobyl Exclusion Zone (Guillén et al., 2017, 2018; Thørring et al., 2016; Beresford et al., 2018a, 2020). In addition to radionuclides, these studies presented data for stable-element concentrations; stable elements are increasingly being used as proxies for radionuclides in parameterizing models (Beresford, 2010; IAEA, 2014b; Copplestone et al., 2013).
This paper describes a study conducted in 2015–2016 to sample terrestrial wildlife and associated soil samples from two forest sites in north-eastern England (Fig. 1) sampled using a similar protocol to that described in Barnett et al. (2014) and subsequently adopted by Guillén et al. (2018) and Beresford et al. (2020) for samplings in Spain and Ukraine. Samples were analysed to determine concentrations of stable elements (Ag Al, As, Ba, Be, Ca, Cd, Co, Cr, Cs, Cu, Fe, I, K, Li, Mg, Mn, Mo, Na, Ni, P, Pb, Rb, Se, Sr, Ti, Tl, U, V and Zn), the majority of which have radioisotopes that need to be considered in radiological assessments, and the gamma-emitting radionuclides 40K and 137Cs. Whole-organism concentration ratios (CRwo-media) have been estimated from these data. The complete dataset associated with this study is available from https://doi.org/10.5285/8f85c188-a915-46ac-966a-95fcb1491be6 (Barnett et al., 2020).
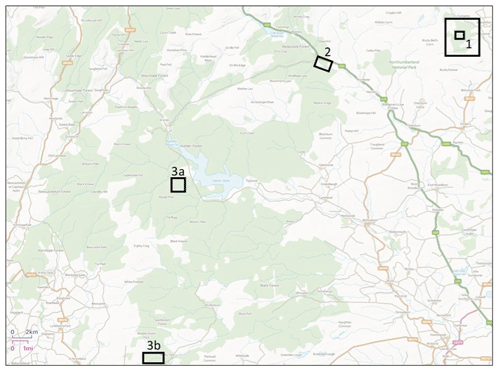
Figure 1Location of sampling sites. Site 1 (inner square) identifies the Holystone main sampling area, and Site 1 (outer square) identifies the Holystone wider sampling area (from which roe deer, fungal fruiting body, berry and some vegetation samples were collected). Site 2 identifies the Kielder main sampling area. Sites 3a and 3b identify the Kielder deer sampling areas (and those of the associated fungal fruiting body and some vegetation samples); note that site 3b is referred to as Spadeadam in Barnett et al. (2020). Contains Ordnance Survey data © Crown copyright and database right 2020.
2.1 Site descriptions
An overview of the sampling sites “Holystone Woods” and “Kielder Forest”, located in north-eastern England (Fig. 1), is given below.
2.1.1 Holystone Woods
Holystone Woods (Ordinance Survey National Grid Reference 3940 6030, total area ca. 8.7 km2, altitude ca. 130 to 360 m) is located within the Northumberland National Park; it borders the Ministry of Defence Otterburn training area (Forestry Commission England 2016) (Site 1 in Fig. 1). Three Sites of Special Scientific Interest (SSSIs) surround the area (Holystone Burn Wood, Holystone North Wood and Harbottle Moors). It is a coniferous forest plantation mainly established between 1954 and 1974 (Forestry Commission England, 2016); the dominant tree species are Pinus sylvestris and Pinus contorta, with some Picea spp. and Larix spp. and some other coniferous and broadleaf species (e.g. Betula spp.) present. Soil types vary and are a complex mixture dominated by peaty surface water gleys and podzolic ironpans. There is an abundance of Pteridium spp. in open places, and the understorey is dominated by Deschampsia flexuosa and Vaccinium myrtillus with some Calluna vulgaris and Sphagnum species.
Our main sampling area (ca. 0.06 km2 in area) at Holystone Woods was within a mixed coniferous plantation, which also contained a few small broadleaf trees with an understorey of grasses (predominantly Molinia caerulea) and some sedge species and shrubs (e.g. Vaccinium spp.). Additional samples were also collected from the surrounding forest (marked in Fig. 1 as “Holystone wider sampling area”).
2.1.2 Kielder forest
Kielder Forest is also located in Northumberland; it is the largest man-made woodland in England (ca. 650 km2). Soils within Kielder vary considerably, with peat often being a major constituent (Forestry Commission England, 2016). Our main sampling area (ca. 0.16 km2 in size; Site 2 in Fig. 1) was situated in the northern section of Kielder in an area referred to as Redesdale Forest (Ordinance Survey National Grid Reference, NGR, 3760 6010; 50 km2, altitude 60–550 m) (Forestry Commission, 1951). Redesdale Forest, and Kielder Forest as a whole, is dominated by Picea sitchensis with a few other coniferous species, including Larix spp., and some broadleaf species (e.g. Betula spp.); the majority of coniferous trees were planted between 1930 and 1960 (Forestry Commission England, 2011). The understorey is mainly Molinia caerulea and Calluna vulgaris. As for Holystone, additional samples were also collected from outside of the main sampling area; these sites are identified in Fig. 1 as 3a and 3b (site 3b is referred to as Spadeadam – the name of the forest “block” – in Barnett et al., 2020).
2.2 Sample collection and preparation
Sampling was targeted towards species representative of the ICRP's terrestrial RAPs: Wild Grass (Poaceae family), Pine Tree (Pinaceae family), Earthworm (Lumbricidae family), Bee (Apidae family), Rat (Muridae family), Deer (Cervidae family) and Frog (Ranidae family). It was not possible to sample species representative of the Duck (Anatidae family). By-catch of Myodes glareolus and Bufo bufo were also retained for analyses. Additional plant and fungi species were collected when it was thought that these may contribute to the diet of the sampled deer.
Table 1Species sampled and analysed during this study.
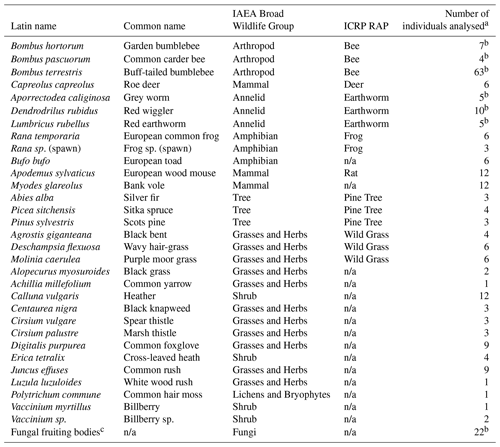
a For these species further samples were collected but were not analysed; information (e.g. mass and dimensions) is available from Barnett et al. (2020). b Some samples were analysed as composite samples. c See Sect. 2.2.13 for a list of species; some species were analysed as composite samples; n/a stands for not applicable; soil (n=47) and water (n=3) samples were also collected.
The species analysed together with their relationship to both the relevant ICRP RAP (ICRP, 2009) and also the IAEA broad wildlife group (IAEA, 2014b) are listed in Table 1. Appendix Table A1 summarizes the elements and radionuclides determined in each sample type; the accompanying dataset (Barnett et al., 2020) provides details of all samples (including masses and dimensions where appropriate) including those collected and retained but not analysed.
At Holystone Woods the majority of samples were collected from the 0.06 km2 study area with the exception of the three Capreolus capreolus which were collected at distances of less than 2 km to the north and east of the main study site within the Holystone wider sampling area in Fig. 1. Samples of vegetation species and also fungal fruit bodies which may be ingested by deer (see Sect. 4) were collected from the wider sampling area as identified in Fig. 1. As for Holystone, the majority of samples from Kielder Forest were collected from the main sampling area. Three C. capreolus were collected from elsewhere within Kielder Forest (see Sites 3a and 3b in Fig. 1). Samples of species which may contribute to the diet of deer (see Sect. 4) were also collected from these areas.
Details of the sampling methods for each sample type are given in Sect. 2.2.1–2.2.13; locations (NGRs) of all individual sampling sites were recorded using a hand-held GPS (accuracy approximately 5 m).
2.2.1 Soil
A total of 16 soil samples (approximately 15 cm × 15 cm × 10 cm deep (as specified in ICRP, 2009) were collected in August 2015 from both the main Kielder and main Holystone sampling sites; locations corresponded to the sampling sites of the animals and plants collected. A further 15 soils were collected in January 2016 from areas where C. capreolus were sampled from within ca. 0.5 km2 of where the deer were shot (0.5 km2 is the typical home range of a female C. capreolus, Barnett et al., 2014); these comprised three samples from Site 3a, three from Site 3b and nine from the wider Holystone sampling area. Although collected by spade, the soil samples were trimmed by knife such that a consistent profile was obtained. Prior to processing, surface vegetation and detritus were removed, with the root mat (where present) being retained in the sample.
Subsamples were removed (ensuring each was representative of the complete soil profile) from each fresh soil sample for determination of pH, loss on ignition (LOI) and dry matter content. The remaining sample was then divided into two subsamples, which were air-dried at 20 ∘C to avoid loss of volatile elements. Once dry, one subsample was ground in an agate mill, prior to ICP-MS analysis; the second sample was ground in a Christie and Norris mill or soil sieve (depending upon organic matter content) to approximately 2 mm for subsequent gamma analysis.
The methods described by Allen (1989) were used to determine the pH (in distilled water) and LOI (ashing at 450 ∘C for 4 h) of all 47 soil samples collected.
2.2.2 Water
Three water samples (each of approximately 1 L) were collected from seasonal ponds in the Kielder main sampling site on 16 March 2015. The samples were collected from three areas within the amphibian trapping area (Sect. 2.2.6); Rana sp. spawn samples were also collected at the same time. All water samples were stored at 4 ∘C following collection; once in the laboratory all samples were filtered through Whatman 541 filters. A 50 mL aliquot of each of the filtered water samples was adjusted to 1 % HNO3 (Baker ultrex II) prior to ICP-MS analyses; the remaining filtered samples underwent gamma analyses.
2.2.3 Bombus spp. (bee spp.)
Bees belonging to the Bombus genus were collected from both main sampling sites between 17 and 21 August 2015 using pan traps (21 cm diameter plastic bowls), which were coloured white, fluorescent yellow or fluorescent blue (see Westphal et al., 2008, for a description of the trapping method). At both the Holystone and Kielder sites, traps were placed on the ground surface close to small mammal trapping points (see Sect. 2.2.8). The pan traps were filled with deionized water to which a small amount of non-perfumed washing-up liquid was added (to prevent evaporation) and covered with a wire cage (a plasticized hanging plant basket) to prevent, e.g. amphibian access; pan traps were emptied every day over the 5 d sampling period. After sieving, individual bees were separated from other invertebrates into pre-weighed scintillation vials and stored frozen (−20 ∘C). Species from each site were identified by an expert (Claire Carvell, UKCEH, personal communication, 2015) (see Table 1). The fresh masses (FM) of a subset of individual bees, from each species, were recorded and the samples then freeze-dried with subsequent dry mass (DM) being recorded. Dried samples were homogenized in an agate mortar to avoid contamination by metals. The majority of the bees were acid digested and analysed by ICP-MS as bulked samples of individuals of the same species from a given site. The only exceptions were a large B. terrestris queen and a single B. hortorum collected from Holystone that were analysed as individuals.
2.2.4 Capreolus capreolus (roe deer)
Three female adult C. capreolus were obtained via the culling scheme operated by the Forestry Commission from within the wider Holystone sampling area (see Fig. 1) on 3 November 2015; one male animal was obtained from Site 3a within Kielder Forest and two animals (one male and one female) were obtained on 5 November 2017 from Site 3b.
The live mass of all the deer was recorded by the Forestry Commission upon sampling. All animals were collected from site promptly and dissected at UKCEH. The following organs and tissues were removed: liver, kidney, spleen, lung, thyroid gland, a hind leg (dissected to obtain separate muscle and bone samples), and the gastrointestinal tract and its contents. The carcass from one animal (identified as Deer 6 in the accompanying dataset) was also divided down the spine, and all muscle, fat and bone was removed from one-half of the carcass; the data obtained were used to estimate the total body mass of each of these components. Bone samples were cleaned of any residual soft tissue by placing in a beetle (Dermestes maculatus) colony.
All samples had their FM recorded and, where appropriate, were diced into approximately 1 cm pieces. They were retained frozen (−20 ∘C) prior to freeze-drying and subsequent analysis. The freeze-dried kidney, liver, gonad, muscle and bone samples were homogenized and analysed by ICP-MS. Gamma analyses were performed on fresh rumen contents and fresh muscle samples.
2.2.5 Lumbricidae (earthworms)
Earthworms, belonging to the Lumbricidae family, were collected at both the Kielder and Holystone main sampling sites from various locations between 20 and 21 August 2015 by digging to approximately 30 cm. After rinsing the earthworms in deionized water they were placed in aerated containers with damp tissue paper to allow for gut evacuation for 48 h. Individuals from each site were identified by species, and adults, sub-adults and juveniles were separated. Composite samples were then created, two from Holystone and one from Kielder, each of these contained 5–10 adult individuals from the same species. The dimensions, FM and DM (after freeze-drying) for the individuals were recorded prior to bulking. Freeze-dried composite samples were homogenized in an agate mortar prior to analyses by ICP-MS.
2.2.6 Rana temporaria (European common frog) and Rana sp. spawn
Within the Kielder main sampling area, an amphibian trapping site (approx. 400 m2) was erected in a “boggy” area. A 1 m tall drift fence was constructed using plastic sheeting and wooden poles. The drift fence provides a physical barrier to amphibian movement within an area, and amphibians then tend to follow the fence line. On each sampling visit, the full length of the drift fence was searched by four people, with the aim of hand-capturing any amphibians present; however, searching by torchlight along the forestry tracks during darkness was more successful. In total four frogs were collected between 13 and 18 August 2015 from the Kielder main sampling area and three from Holystone (one of which was not analysed). After humane dispatch the animals were stored frozen (−20 ∘C) until further preparation.
The frogs had their FM recorded and then their gastrointestinal tract removed. The following organs and tissues were removed from each animal: gonads; liver; fat bodies; “tissue containing the thyroid gland”, where possible (this approach was taken due to the small size of the thyroid, see Guillén et al., 2018); a hind leg (dissected to obtain both muscle and bone samples); and a composite sample comprising kidney, spleen, and lung. Each carcass and hind-leg bone were placed in a beetle (D. maculatus) colony to clean the bone of all soft tissue. All the tissue samples had their FM recorded, were diced into small pieces (as appropriate) and retained frozen (−20 ∘C) prior to freeze-drying (DM was recorded in most cases) and ICP-MS analysis.
Three spawn samples were collected on 16 March 2015 at the Kielder main sampling site from the area of boggy ground where the amphibian trapping sheet was subsequently located. Each spawn sample was washed in deionized water and the sample FM was recorded. Each sample was then freeze-dried and the DM recorded. Following freeze-drying, samples were homogenized in an agate mortar prior to ICP-MS and gamma analyses.
2.2.7 Bufo bufo (European toad)
A total of 16 toads were collected as described above for frogs on the 13 August (n=12) and 19 August (n=4) 2015. All except one (which was collected from the Holystone main sampling area) were collected from the Kielder main sampling area; they were all stored frozen (−20 ∘C) upon capture and a whole-body FM was recorded. Subsequently, six toads were randomly selected and prepared for analysis. Their gastrointestinal tract (together with its contents) was removed and the FM of the carcass (less the gastrointestinal tract) was recorded. The carcass was then washed in deionized water, freeze-dried (DM recorded) and ashed in a muffle furnace for approximately 24 h (using a stepped heating programme up to 400 ∘C). The six ash samples had their ash mass (AM) recorded and were subsequently analysed by ICP-MS. Toads (and voles; see below) were ashed as they were by-catch and were outside the scope of the core study; ashing gave a simple sample preparation technique as there was no need to analyse individual tissues.
2.2.8 Apodemus sylvaticus (wood mouse)
At both the Holystone and Kielder main sampling sites, 60 “Longworth” traps were placed along wall lines, beside fallen trees or along obvious small mammal tracks; they were placed in these positions as mice prefer to move along linear features (Spellerberg and Gaywood, 1993). At Holystone, the traps were placed at up to four separate locations within the main sampling area, and at Kielder they were placed at six locations within the main sampling area. Depending upon the location, traps were placed either in blocks of 12 or in short runs containing 4–12 traps.
Traps were baited overnight with oats, carrot and insect pupae; grass hay was added as bedding material. At both sites baited traps were left open for 3 d before trapping began to familiarize the animals to them. Once trapping began all traps were inspected each morning and then closed, they were re-baited and reset early each evening. Trapping at the Holystone main sampling site took place between 17 and 21 August 2015. At Kielder, trapping was only conducted at the main sampling site on 17 August 2015; subsequent trapping was unnecessary due to the high number of animals caught. Trapping was conducted in accordance with Natural England general licence WML-GL01 (Natural England, 2017). Mice (and voles, Sect. 2.2.9) were euthanized immediately upon being found in a trap using the appropriate humane method given in Schedule 1 of the Animals (Scientific Procedures) Act (1986) (UK Parliament, 1994). Any shrews (Sorex sp.) trapped were released.
A total of 9 male and 5 female mice were trapped at Holystone, and 8 male and 10 female mice were trapped at Kielder (2 additional mice were caught, but their sex was not recorded). Whole-body FM (including gastrointestinal tract and contents) and dimensions were recorded for all mice. Randomly selected mice (n=12, 6 from Holystone and 6 from Kielder) had their pelt and gastrointestinal tract (including contents) removed and the FM of the remaining carcass recorded; this was then washed in deionized water. The liver; gonads; a sample of tissue containing the thyroid gland (see Guillén et al., 2018); a hind leg (dissected for separate muscle and bone samples); and a composite sample made up of the spleen, kidney, and lung were removed. Individual tissue FM was recorded and the samples were then stored frozen (−20 ∘C) prior to freeze-drying after which their DM was recorded. The remaining carcass (and hind-leg bone samples) was placed in a beetle (D. maculatus) colony to clean the bone of remaining soft tissue; once clean the FM of the remaining bone from both the carcass and hind leg was recorded and samples stored frozen (−20 ∘C) prior to freeze-drying, after which their DM was recorded. The liver, tissue containing thyroid, muscle and bone samples were homogenized. The tissue samples from the 12 randomly selected mice were analysed by ICP-MS.
2.2.9 Myodes glareolus (bank vole)
Voles were by-catch from the mice-trapping activities. A total of 13 voles were captured from the Holystone main sampling site and 14 from the Kielder main sampling site; they were stored frozen (−20 ∘C) after being euthanized as described in Sect. 2.2.8. All voles had their whole-body FM recorded; the pelt and gastrointestinal tract (including contents) was then removed and the FM of the remaining carcass was recorded for each vole; they were then washed in deionized water. Of the 27 animals captured, 6 (3 male and 3 female) from each main sampling site were randomly selected for ICP-MS analysis. These 12 carcasses were then freeze-dried (DM recorded) and ashed in a muffle furnace for approximately 24 h, using a stepped heating programme up to 400 ∘C; the AM was then recorded prior to analysis by ICP-MS.
2.2.10 Abies alba, Pinus sylvestris and Picea sitchensis (silver fir, Scots pine and sitka spruce)
Species selected for sampling were those that were dominant at each site. Samples of heartwood core (taken using an increment borer), small branches (approximately 1 cm in diameter) and needles were collected from pine trees located at both the Kielder (three P. sitchensis) and Holystone (two P. sylvestris and one A. alba) main sampling areas on 20 August 2015 and from one tree (P. sitchensis) at Site 3b on 21 January 2016. Needles (and cones, if present) were removed from the collected branches, the branches were then cut into small pieces (ca. 1 cm length). The FM was recorded for all sampled tree components, which were then air-dried (approximately 20 ∘C) prior to DM being determined. Branch, needle and cone samples were then finely ground using an agate mill or mortar (dependent upon size) and the heartwood core samples were manually size-reduced to approximately 5 mm in diameter. ICP-MS analysis was conducted on all the needle and heartwood core samples; all the branch samples and two cone samples also underwent gamma analysis.
2.2.11 Molina caerulea, Agrostis gigantea and Deschampsia flexuosa (purple moor grass, black bent and wavy hair-grass)
A total of 16 samples were collected from Kielder (n=4 A. gigantea, n=3 D. flexuosa and n=3 M. caerulea) and Holystone (n=3 D. flexuosa and n=3 M. caerulea) main sampling areas on 20 August 2015; both leaf and flower stems were collected to within ca. 1 cm of the soil surface. Species selected were those that were abundant at each site. All the samples were stored cool (4 ∘C) prior to transport to the laboratory where their FM was recorded; they were then air-dried (approximately 20 ∘C), and subsequently their DM was recorded. All samples were finely ground using an agate mill or mortar (dependent upon sample size) and analysed by ICP-MS. A total of 15 samples that had a DM > 5 g also underwent gamma analysis.
2.2.12 Herbaceous plants and shrubs
Numerous opportunistic vegetation samples were collected from both the Kielder and Holystone main sampling areas, with most samples being collected on 20 August 2015 (Table 1); species selected for sampling were abundant at each site. Some species were also sampled from where C. capreolus were obtained (see Sect. 2.2.4); these samples were collected either on 22 January or 24 October 2016.
All samples were stored cool prior to transport to the UKCEH; upon receipt, species collected were positively identified and had their FM recorded. The samples were then air-dried (approximately 20 ∘C), and once dry the DM was recorded. Subsequently, they were all finely ground using an agate mill or mortar (dependent upon size) and analysed by ICP-MS; if, following ICP-MS analysis, there was remaining sample > 5 g (DM), gamma analysis was also conducted.
2.2.13 Fungal fruiting bodies
Opportunistic samples of fungal fruiting bodies were collected, placed in paper bags (to avoid sample degradation) and stored cool (4 ∘C) prior to transport to the UKCEH where species were positively identified. Generally single bulked samples of different species were collected from the sites.
-
Holystone wider sampling area (24 October 2016) – Galerina vittiformis, Clitocybe sp., Collybia sp., Hygrophoropsis aurantiaca, Hygrophorus sp., Laccaria laccata, Russula emetica, Suillus sp..
-
Kielder Site 3a (24 October 2016) – Galerina sp., Collybia sp., Cortinarius sp., H. aurantiaca, Mycena epipterygia, Psathyrella sp.
-
Kielder Site 3b (23 October 2016) – Calvatia excipuliformis, Galerina sp. (n=2), Gymnopilus sp., H. aurantiaca, Hygrophorus sp. (n=2), L. laccata.
After recording the FM of the individual samples they were oven-dried at approximately 60 ∘C and their DM recorded. Individual samples were then finely ground to approximately 2 mm using either an agate mortar or electric coffee grinder depending upon their size. With the exception of the single C. excipuliformis, all fungal fruiting bodies were bulked into six replicates by sampling location and, where possible, by feeding strategy. If after bulking the sample size was too small for gamma analysis, the sample volume was increased by mixing with cornflour (an inert material of a similar mass). Gamma analysis was then conducted on all samples.
2.3 Sample digestion
Acid digestions were undertaken at the University of Nottingham to determine elemental concentrations in soils, plant material and animal tissues. Alkaline extractions with tetramethylammonium hydroxide (TMAH) were also undertaken to determine concentrations of iodine in soils, plants and (where sample size was sufficient) animal tissues.
2.3.1 Soil
Acid digestion was undertaken by weighing approximately 0.2 g of dried ground soil into a Savillex™ vial, adding concentrated Primar grade HNO3 (4 mL) and heating at 80 ∘C overnight using a teflon-coated graphite hot block; this first step was undertaken due to the comparatively high organic matter contents in a number of soils. Then concentrated Primar grade HF (2.5 mL), HNO3 (2 mL) and HClO4 (1 mL) were added. A stepped heating programme up to 160 ∘C overnight was applied to fully digest silicate and oxide phases. The dry residue was reconstituted after warming with 2.5 mL ultrapure MilliQ water and 2.5 mL HNO3, and the final volume made up to 50 mL. The Standard Reference Material NIST SRM 2711a Montana soil in duplicate, and five blanks were all digested in a similar manner to check the accuracy and precision of the digestion and analysis methods. All the digests were diluted to 1-in-5 before analysis.
To determine iodine concentrations, an alkaline extraction was undertaken using TMAH. A portion of soil (approximately 1 g) was weighed into polypropylene tubes and 10 mL of 10 % TMAH were added. The soil suspensions were heated at 90 ∘C for 24 h and then centrifuged at 3500 rpm for 30 min. A 1 mL aliquot was pipetted off and diluted 10-fold to give a final TMAH concentration of 1 % for further analysis. Four blanks were prepared in a similar manner.
2.3.2 Plant material
Microwave-assisted acid digestion was conducted on a subsample of dried-ground plant material (approximately 0.2 g), which was weighed into digestion vessels and 6 mL concentrated Primar grade HNO3 added. The samples were digested using a Multiwave PRO Anton Paar microwave reaction system, with heating at 140 ∘C for 20 min and further cooling to 55 ∘C for 15 min. Once the digestion was complete, the samples were made to a final volume of 20 mL. Full dissolution was achieved in all cases. Digestion of five replicates of Standard Reference Material NIST 1573a Tomato Leaves and seven blanks were all undertaken in a similar manner to check the accuracy and precision of the digestion and analysis methods. The elemental recoveries for the certified reference material were typically > 90 %. Prior to analysis, the acid digests were diluted to 1-in-15 to give a final matrix of 2 % HNO3.
For microwave-assisted TMAH extraction, a portion of plant material (approximately 0.2 g) was weighed into digestion vessels and 5 mL of 5 % TMAH was added. The samples were microwave digested and heated at 110 ∘C for 30 min, followed by a cooling step at 40 ∘C for 12 min. The extracts were transferred to polypropylene centrifuge tubes and made up to a final volume of 25 mL to give a final TMAH concentration of 1 %. Before analysis, the samples were centrifuged (3500 rpm for 30 min) and a 5 mL aliquot of the supernatant transferred into an ICP auto-sampler tubes for analysis. Four replicates of CRM NIST 1573a Tomato Leaves and four blanks were all prepared in a similar manner to check the efficiency of the extraction and total iodine recovery.
TMAH extraction is not able to fully digest plant material and variable degrees of dissolution were observed. However, the analysis of CRM NIST 1573a showed a recovery of 78 %–85 % iodine, calculated on the basis of a non-certified iodine concentration of 0.85 mg kg−1. This suggests that even though plant tissues were not fully dissolved, the TMAH extraction solubilizes a sufficient proportion of iodine provided that the material is finely ground; samples with coarse particle size, i.e. pine tree cores, showed poor dissolution rates and therefore the reported iodine concentrations may be underestimates.
2.3.3 Animal tissues
For microwave-assisted acid digestion, where available a portion of dried-ground animal tissue (approximately 0.2 g) was weighed into digestion vessels and a mixture of 3 mL Primar grade HNO3 + 3 mL MilliQ ultrapure water + 2 mL 30 % v/v H2O2 was added. The samples were allowed to froth for 30 min in uncovered vessels before microwave digestion at 140 ∘C for 20 min. Once the digestion was complete, the extracts were made to a final volume of 20 mL. Seven replicates of CRM NIST 1577c bovine liver and 14 blanks were all prepared in a similar manner. Prior to analysis, the acid digests were diluted 8-fold to give a final HNO3 concentration of approx. 2 %. Recoveries of typically 90 %–110 % were obtained for the certified elements for NIST 1577c. Full dissolution was achieved for all untreated samples, but variable dissolution rates were observed for ashed samples (e.g. vole and toad carcasses) where the presence of undissolved black particles in the solutions suggested that the digestion was incomplete.
For TMAH extraction of animal tissues, a portion of dried-ground tissue (approximately 0.2 g) was weighed into polypropylene tubes and 5 mL of 5 % TMAH was added. The tubes were then placed in an oven (90 ∘C ± 3 ∘C for 5 h), occasionally tapping and swirling to help dissolution. The extracts were allowed to cool, diluted with ultrapure water to a final volume of 25 mL and then centrifuged at 3500 rpm for 25 min. An aliquot of 5 mL of supernatant was then transferred to ICP tubes ready for analysis. Exceptions were the thyroid gland from the deer, for which a further 200-fold dilution was required to bring concentrations within the calibration range (0.5–100 µg L−1 iodine). The majority of samples were fully or largely digested; however, a number of them showed undissolved white particles, most likely undigested bone. In addition, as reported above for acid digests, ashed samples showed poor dissolution rates. For the three frogs for which a tissue containing thyroid was available, there was insufficient sample for other tissues types to conduct analyses.
2.4 Stable-element analysis
With the exception of the water samples, which were analysed at UKCEH, multi-element analysis of soils, plants and animal tissues was conducted at the University of Nottingham using an iCAP-Q ICP-MS (Thermo Fisher Scientific, Bremen, Germany).
For acid digests, the instrument was run employing three operational modes: (i) a collision cell (Q cell) using He with kinetic energy discrimination (He-cell) to remove polyatomic interferences, (ii) a standard mode (STD) in which the collision cell is evacuated and (iii) a hydrogen mode (H2-cell) in which H2 gas is used as the cell gas. Samples were introduced from an autosampler (Cetac ASX-520) incorporating an ASXpress™ rapid-uptake module through a PEEK nebulizer (Burgener Mira Mist). Internal standards were introduced to the sample stream on a separate line via the ASXpress unit and included Ge (10 µg L−1), Rh (10 µg L−1) and Ir (5 µg L−1) in 2% trace analysis grade (Fisher Scientific, UK) HNO3. External multi-element calibration standards (Claritas-PPT grade CLMS-2 from SPEX Certiprep Inc., Metuchen, NJ, USA) included Ag, Al, As, Ba, Be, Ca, Cd, Co, Cr, Cs, Cu, Fe, K, Li, Mg, Mn, Mo, Na, Ni, P, Pb, Rb, S, Se, Sr, Tl, U, V and Zn in the range 0–100 µg L−1 (0, 20, 40, 100 µg L−1). A bespoke external multi-element calibration solution (PlasmaCAL, SCP Science, France) was used to create Ca, Mg, Na and K standards in the range 0–30 mg L−1. Phosphorus, B and S calibration utilized in-house standard solutions (KH2PO4, K2SO4 and H3BO3). In-sample switching was used to measure B and P in STD mode, Se was used in H2-cell mode and all other elements were used in He-cell mode. Peak dwell times were 10 ms for most elements, with 150 scans per sample. Sample processing was undertaken using Qtegra™ software (Thermo Fisher Scientific) utilizing external cross-calibration between pulse-counting and analogue detector modes when required.
Iodine analysis in the alkaline extracts was undertaken separately using a 1 % TMAH matrix for both standards and samples. The concentrations were determined in standard (STD) mode (evacuated collision cell) using Re (5 µg L−1) in 2 % trace analysis grade as internal standard to correct for suppression or enhancement effects. The instrument was calibrated (0–100 µg L−1 127I) using synthetic chemical solutions diluted from NaIO3 stock solution.
Where the amount of sample provided was > 0.3 g, both extractions were conducted; in those cases where the amount of available sample was limited, acidic digestion was prioritized unless the samples were tissue containing thyroid (Guillén et al., 2018). For each element, extraction form and sample type (i.e. soil, plant or animal tissue), limits of detection (LODs) were calculated as 3 times the standard deviation of the reagent blanks. The suite of elements reported for soil, vegetation and animal samples is Ag, Al, As, Ba, Be, Ca, Cd, Co, Cr, Cs, Cu, Fe, I, K, Li, Mg, Mn, Mo, Na, Ni, P, Pb, Rb, Se, Sr, Ti, Tl, U, V and Zn.
Water analysis was conducted at UKCEH for the full suite of trace metals (As, Be, Ce, Cr, Cu, Li, Ni, Pr, Sb, Sn, Ti, V, Zn, Ba, Cd, Co, Cs, La, Mo, Pb, Rb, Se, Sr, U and W) on a Perkin Elmer ICP-MS. Analysis of a core suite of elements (Al, Ca, K, Mn, Si, Fe, Mg, Na and B) was also conducted by ICP-OES (Perkin Elmer DV 7300). Results for quality control and duplicate samples analysed alongside test samples were within expected ranges.
2.5 Gamma analysis
To determine the activity concentration of 40K and 137Cs, soil, water, fungal fruiting bodies and selected plant (see Sect. 2.2.10, 2.2.11, 2.2.12) and animal (see Sect. 2.2.4, 2.2.6) samples were analysed in suitably sized containers (ca. 130 or 700 mL (Dormex Ltd.) or 15 mL petri dishes) on hyper-pure germanium detectors using an efficiency calibration suited to sample density. Dried-ground soil samples were analysed for 2 d, dried-ground plant samples and fungal fruit body samples were analysed for 4 d, and animal samples were analysed as fresh material (with the exception of frogspawn, which was analysed dry) for up to 4 d. The size of the available sample determined whether gamma analyses were conducted; the minimum sample size was 15 mL.
The detectors were calibrated for efficiency against standards of various density and volume using a certified reference solution (National Physics Laboratory, R08-04 “Mixed nuclide standard solution”; http://www.npl.co.uk/upload/pdf/mixed_nuclide_standard_solutions.pdf, last access: 20 September 2020), which covers an energy range of approximately 59–1850 keV. The resultant spectra were analysed using the Canberra Apex software, and the estimated activity concentrations (and a two sigma counting error) were decay-corrected to the day of sampling. Replicate analyses were conducted on random samples and process blanks for quality assurance purposes and the laboratory regularly participates in IAEA (https://nucleus.iaea.org/sites/ReferenceMaterials/Pages/Interlaboratory-Studies.aspx, last access: 20 September 2020), IARMA (http://www.iarma.co.uk/services/analytical-proficiency-tests-2/, last access: 20 September 2020) and US Department of Energy MAPEP (https://mapep.inl.gov/, last access: 20 September 2020) proficiency testing schemes.
2.6 Calculation of whole-organism concentrations for mammals and amphibians
To calculate whole-organism concentrations for roe deer, small mammals and amphibians we used the total masses and elemental concentrations determined in the analysed tissues. The approach and assumptions used are similar to those used in previous papers (Barnett et al., 2014; Guillén et al., 2018).
Roe deer whole-organism stable-element concentrations were calculated assuming bone, muscle, kidney, liver and thyroid comprised the whole organism. To calculate total concentrations in a given tissue the measured total organ mass, or, in the case of bone and muscle, estimated total tissue masses were used. Total bone and muscle masses were estimated using the average percentage contributions of these tissues to the whole-body mass as determined from the animal on which the half carcass dissection was performed in this study and similar results from three roe deer from an earlier study (see Barnett et al., 2014). The exception was for the deer subjected to the half carcass dissection in this study, which used estimates of total muscle and bone mass derived for this animal. For 137Cs it has been assumed that activity concentrations in muscle are representative of those in the whole organism (Beresford et al., 2008c; Yankovich et al., 2010).
Wood mice whole-organism stable-element concentrations have been calculated using an estimate of concentrations in total bone and of total muscle and concentrations in the liver (and for iodine concentrations in tissue containing thyroid). For European common frogs, whole-organism stable-element concentrations were calculated in the same manner as for wood mice with the exception that gonad masses and concentrations were also used. In Barnett et al. (2014), we demonstrated that the contribution of gonads to the whole-organism concentrations of elements in wood mice was minimal, and hence we did not analyse mice gonads in this study. It was not possible to calculate whole-organism concentrations of iodine for the frogs due to the lack of results for tissues other than the sample containing the thyroid.
For some animals an element may not have been detectable in all analysed tissues. If this was the case the LOD value was used for that element tissue. If the contribution of an element to the total body content was estimated to be ≥ 10 % from tissues with concentrations below the LOD, then the whole-organism concentration is reported as a “less than” value in Barnett et al. (2020). If the contribution from tissues with concentrations below the LOD was < 10 % of the total body content, then the estimated whole-organism concentration was assumed to be a reasonable approximation.
For bank voles and common toads no data manipulation was necessary as whole (ashed) carcasses were analysed.
2.7 Calculation of whole-organism concentration ratios
Whole-organism concentration ratios were calculated using Eq. (1), with soil concentrations being the arithmetic mean value for the appropriate sampling site (e.g. for all samples types obtained from the main sampling areas the mean soil concentration over the sampling area was used); soils used to calculate CRwo-soil values are identified within the accompanying dataset (Barnett et al., 2020). For mammals and amphibians, whole-organism concentrations (as estimated in the manner described above) were used to calculate whole-organism concentration ratios. For earthworms and bees, whole organisms were analysed to determine their elemental concentrations. For plants and fungi, CRwo-soil values were estimated for the components analysed; for the RAP pine tree the ICRP geometry is the trunk (i.e. heartwood, ICRP, 2008), although CRwo-soil values are also presented here for needles. For frogspawn, CRwo values were estimated as CRwo-water values. All CRwo values are expressed on a FM basis.
All data associated with this study are available (as .csv files) from Barnett et al. (2020); the supporting documentation associated with the dataset contains descriptions of the contents of each .csv file that forms the dataset. In summary, the data available from Barnett et al. (2020) are as follows.
3.1 Bombus spp. (bee spp.)
-
stable-element concentrations (mg kg−1,FM and DM) for composite (single species) samples and for a sample containing a single B. hortorum queen,
-
whole-organism concentration ratios (FM),
-
FM and DM of bees sampled but not analysed.
C. capreolus (roe deer)
-
stable-element concentrations (mg kg−1, FM and DM) for hind-leg bone, muscle, liver, kidney, thyroid gland, and rumen contents;
-
stable-element concentrations presented as “mg per tissue” (i.e. the total amount, in mg, of an element in a given tissue) for all tissue types other than rumen contents;
-
whole-organism stable-element concentrations (FM);
-
whole-organism concentration ratios (FM);
-
radionuclide activity concentrations (Bq kg−1, FM and DM) for 137Cs for muscle;
-
caesium-137 concentration ratios (calculated using the 137Cs activity concentrations, FM, measured in muscle);
-
FM and DM of various organs and tissues sampled but not analysed.
Lumbricidae (earthworms)
-
stable-element concentrations (mg kg−1, FM and DM) for composite (single-species) samples,
-
whole-organism concentration ratios (FM),
-
FM, DM and dimensions of earthworms sampled but not analysed.
R. temporaria (European common frog) and Rana sp. spawn
-
stable-element concentrations (mg kg−1, FM and DM) for hind-leg bone, muscle, liver, gonad, and tissue containing thyroid (see Guillén et al., 2018);
-
stable-element concentrations presented (as mg per tissue) for all the tissue types listed above other than tissue containing thyroid;
-
whole-organism stable-element concentrations (FM);
-
whole-organism concentration ratios (FM);
-
FM and DM of some organs and tissues (e.g. lung, spleen, renal organ and fat bodies, etc.) sampled but not analysed;
-
stable-element concentrations (mg kg−1, FM and DM) for Rana sp. spawn;
-
stable-element concentration ratios (FM) for Rana sp. spawn (calculated using the stable-element concentrations in water);
-
radionuclide activity concentrations (Bq kg−1, FM and DM) for 40K and 137Cs in Rana sp. spawn.
B. bufo (European toads)
-
whole-organism stable-element concentrations (mg kg−1 (FM and AM)),
-
whole-organism stable-element concentration ratios (FM),
-
FM and DM of some organs and tissues (e.g. lung, spleen, renal organ, fat bodies, and gastrointestinal tract and contents, etc.) sampled but not analysed.
A. sylvaticus (wood mice)
-
stable-element concentrations (mg kg−1, FM and DM) for hind-leg bone, muscle, liver, and tissue containing thyroid (see Guillén et al., 2018), and no data for Be is presented as too few tissues had concentrations in excess of the LOD ( mg kg−1 FM);
-
stable-element concentrations presented as “mg per tissue” for all tissue types listed above;
-
whole-organism stable-element concentrations (FM);
-
whole-organism concentration ratios (FM);
-
the FM and DM of some additional organs and tissues (e.g. kidney, spleen, lung, gonad, pelt) that were sampled but not analysed.
M. glareolus (bank voles)
-
whole-organism stable-element concentrations (mg kg−1, FM and AM),
-
whole-organism concentration ratios (FM),
-
FM of voles sampled but not analysed.
A. alba, P. sylvestris and P. sitchensis (silver fir, Scots pine, sitka spruce)
-
stable-element concentrations (mg kg−1, FM and DM) for needles and heartwood (core),
-
radionuclide activity concentrations (Bq kg−1, FM and DM) for 137Cs and 40K for the needles and branches,
-
stable-element concentration ratios (FM),
-
caesium-137 concentration ratios (FM and DM),
-
FM and DM for samples not analysed.
M. caerulea, A. giganteana and D. flexuosa
-
stable-element concentrations (mg kg−1, FM and DM),
-
radionuclide activity concentrations (Bq kg−1, FM and DM) for 137Cs and 40K for samples large enough for analysis,
-
stable-element concentration ratios (FM),
-
radionuclide concentration ratios (FM and DM) for 137Cs.
Herbaceous species and shrubs (see Table 1)
-
stable-element concentrations (mg kg−1, FM and DM),
-
radionuclide activity concentrations (Bq kg−1, FM and DM) for 137Cs and 40K for samples large enough to analyse,
-
stable-element concentration ratios (FM),
-
radionuclide concentration ratios (FM and DM) for 137Cs.
Fungal fruiting bodies (see Sect. 2.2.13 for a list of species sampled)
-
radionuclide activity concentrations (Bq kg−1 FM and DM) for 137Cs and 40K for composite samples and also for a single sample of C. excipuliformis,
-
radionuclide concentration ratios for 137Cs (FM and DM).
Soil
-
stable-element concentrations (mg kg−1, DM),
-
Radionuclide activity concentrations (Bq kg−1, DM) for 40K and 137Cs,
-
pH and LOI measurements for some samples.
Water
-
stable-element concentrations (mg kg−1, filtered),
-
radionuclide activity concentrations (Bq kg−1, filtered) for 40K and 137Cs.
This study has significantly increased the data available for the ICRP RAPs. For instance, ICRP (2009), the compilation of CRwo-media values for the ICRP RAPs, has no data for bees or frogspawn, data for only four elements for deer, and data for only five elements for frogs. The most numerous data in ICRP (2009) were for earthworms and, even for this RAP, data were only available for 17 elements compared to the approximately 40 elements considered in some radiological environmental assessment tools (e.g. Brown et al., 2016).
Detailed analyses and discussion of the data are outside the scope of this paper and consequently we provide an overview only. As noted, the published ICRP compilation of CRwo-media values (ICRP, 2009) had rather limited data, making meaningful comparisons difficult. Where comparisons are possible then the data reported here and presented in ICRP (2009) are generally within the same range. There are some instances where the CRwo-media values reported here are at the lower end of the ICRP range1, these include Co and Sr for Reference Rat; Cs, Pb, and Sr for Reference Wild grass; and Pb for Reference Pine tree. Since the publication of ICRP (2009), a number of studies have been conducted using a protocol similar to that described here in an attempt to provide data for the developing ICRP environmental protection framework. These studies were conducted in north-western England (Barnett et al., 2014), Mediterranean Spain (Guillén et al., 2018), the Ukrainian Chernobyl Exclusion Zone (Beresford et al., 2020) and Norway (Thørring et al., 2016). As in some instances these new data represent a larger dataset than considered in ICRP (2009), we have compared data from them to the data reported here for selected elements of radiological interest (Ag, Co, Cs, Fe, Pb, Se, Sr and U). In most instances, the data reported here were within the range of those from the other studies. The exception was for Cs and roe deer, where the CRwo-soil values for both stable Cs and 137Cs were about an order of magnitude higher for the sites reported here than those for the other four sites.
This study and those reported using a similar protocol (Barnett et al., 2014; Thørring et al., 2016; Guillén et al., 2018; Beresford et al., 2020) have significantly increased the available data for the ICRP RAPs for a large number of elements. However, as the studies have tended to focus on stable elements and gamma-emitting radionuclides, some radionuclides which may need to be included in radiological environmental assessments have been neglected (e.g. only Thørring et al., 2016, considers 226Ra, whilst Beresford et al., 2020, was the only study to include Am and Pu radioisotopes). In future, studies using protocols similar to that described here should, resources allowing, consider a broader range of analyses to encompass a wider range of elements or radiological concern.
Transfer parameters for radioecological models for both wildlife and human foodstuffs are increasingly being derived from measurements of stable elements using ICP-MS analyses (e.g. Copplestone et al., 2013; IAEA, 2010, 2014b) as we have done in the study reported here. This has the advantage that multiple elements can be determined in a single analysis and for many elements it is the only feasible approach to obtaining data. These data are used in radioecological models, assuming that stable-element transfer parameters will be reflective of those for radioisotopes at equilibrium. However, we note that where CRwo-soil values from this study could be compared for stable Cs and 137Cs, the values for 137Cs were consistently higher than those for stable Cs. Comparison was possible for 30 samples (roe deer, grassy and herbaceous vegetation species, moss, heather species, and pine trees), the ratio of 137Cs (ca. 30-year physical half-life) to stable Cs CRwo-soil values ranged from 1.3 to 10.5 across these samples (median = 4.7). Similar observations for Cs, and to a lesser extent Sr (which also has a ca. 30-year physical half-life), have recently been made at other sites (Barnett et al., 2014; Beresford et al., 2019, 2020; Thørring et al., 2016). The increasing number of similar observations questions the use of stable-element CRwo-soil values within radiological assessments; radiological assessments typically aim to be conservative. However, as already noted, for many elements there is no other realistic approach to obtaining data on environmental transfer other than to use stable-element data.
4.1 Use of data
The data described here will be added to the “Wildlife Transfer Database” (Copplestone et al., 2013; http://www.wildlifetransferdatabase.org/, last access: 1 June 2020), which supports ICRP and IAEA activities and provides a resource for the updating of models (e.g. Brown et al., 2016; Beresford et al., 2018b).
In this paper we report on the derivation of 3889 CRwo-media values for 30 elements (and 90 CRwo-media values for Cs-137) in total across a range of plant and animal species collected in temperate forest ecosystems. The study focused on obtaining data for species falling within the definition of the ICRP's RAPs (ICRP, 2008) given the lack of data for these (ICRP, 2009). As noted above, this study makes a considerable contribution to addressing this lack of data and as such the results are, and will continue to be, used by the ICRP in the ongoing development of their environmental assessment framework (e.g. see https://www.icrp.org/icrp_group.asp?id=92, last access: 1 June 2020). Data are described here for elements for which CRwo-media values were previously lacking, for instance ICRP (2009) contains no data for iodine (nor does the more comprehensive compilation of CRwo-media values presented in IAEA, 2014b).
In wildlife assessment models, the concentrations of the vast majority of elements for terrestrial species are estimated via CRwo-soil values (e.g. Beresford et al., 2010). However, for logical reasons (Galeriu et al., 2003) activity concentrations of 3H and 14C are related to air not soil. Because of a lack of any data for P-isotopes when models were originally developed, an assumption was made that 14C CRwo-air concentrations could be used as proxies (Copplestone et al., 2001; Beresford et al., 2008c; Brown et al., 2016). This assumption has never been tested and is likely not very robust (e.g. if terrestrial ecosystems are contaminated via discharges to sewers or rivers). The data reported here present some of the first CRwo-soil values for P, which will allow us to start to establish a more robust modelling approach for radioisotopes of this element. To our knowledge, the CRwo-water values we report for frogspawn are amongst the first available, along with data we recently published for samples obtained from within the Chernobyl Exclusion Zone (Beresford et al., 2020).
Often data are available for radionuclides in wild animal tissues that are used in the human food chain (e.g. RIFE, 2019). To be able to use such data in the derivation of transfer parameters for application in wildlife assessment models, a methodology to convert tissue-specific concentrations to whole-organism concentrations is required. Yankovich et al. (2010) published tissue to whole-organism conversion factors and these were used to help populate the Wildlife Transfer Database (Copplestone et al., 2013). However, these factors are often based on few data. For instance, for terrestrial mammals, the recommended Ag, Cs, Se and U conversion factors are each based on one value only, and no values are presented for Co and Sr; no recommended conversion factors for amphibians are based on more than seven observations. The data discussed in this paper will contribute to improving the available conversion factors for both mammals and amphibians, including for elements for which Yankovich et al. present no data. Our data also enable a comparison of the transfer of radionuclides to the whole organisms and the gonads of amphibians. Assessment of dose to gonads is of interest as reproductive effects are a key endpoint when considering population level effects of exposure to ionizing radiation (ICRP, 2009); data for mammals were previously available (ICRP, 2008; Barnett et al., 2014). Our data suggest little difference in the likely transfer of radionuclides to the gonad of amphibians compared to that of the whole organism. Where there were differences (Ca, Mn, Pb, Sr and Ti) then gonad concentrations were about 2 orders of magnitude lower than whole-organism concentrations. Consequently, based on our data, assuming whole-organism concentrations is likely to give a reasonable, or conservative, estimate of amphibian gonad concentrations (as the data of Barnett et al. , 2014, demonstrates for mammals).
Although not acknowledged in the accompanying documentation (i.e. Copplestone et al., 2013), the Wildlife Transfer Database does not differentiate between tree components (i.e. data for wood, needles, etc. are pooled together to derive summarized values) and some studies only sample needles (e.g. Thørring et al., 2016) even though the ICRP geometry for their Reference Pine Tree is arguably the trunk wood (ICRP, 2008). In Barnett et al. (2014), we suggested that the sampling and analysis of needles would generally result in a conservative CRwo-soil value. The results from the sites discussed in this paper are supportive of this suggestion: for most elements concentrations in needles and wood (heartwood core) were within an order of magnitude of each other; for I, Na, P and K concentrations in needles were more than an order of magnitude higher than those in the wood. Only Fe and Ag had concentrations in wood of more than an order of magnitude higher than those in needles; in the case of Fe we have to acknowledge the potential for contamination (from sample preparation equipment) during the sampling and preparation of the heartwood core samples for analyses.
A potential issue of studies such as that described here is that they generate site-specific data, which may not be applicable to other ecosystems (e.g. Hirth et al., 2017, suggests that CRwo-soil values for some Australian species differ to those from temperate ecosystems). Novel approaches to estimating the transfer of radionuclides to wildlife using phylogenetic or taxonomic models are being investigated (e.g. Beresford et al., 2013, 2016; Brown et al., 2016; Beresford and Willey, 2019). These models would have a number of advantages over the CRwo-media approach, the most significant being that they account for the influence of site on radionuclide transfer (see Beresford and Willey, 2019, for a discussion). The parameterization of these models requires data for multiple species from a single site, and as such the data from the study described in this paper are ideal (data presented here for Pb have already been used to establish such a model for Pb transfer to terrestrial wildlife; Beresford and Willey, 2019). Data presented here will be directly useful in conducting assessments of the exposure of poorly studied organisms. For example, Tagami et al. (2018) assessed the radiation exposure of frogs in Fukushima Prefecture. Whilst the authors had data for adult and tadpole life-stages, no data were available for frogspawn, and consequently the provisional CRwo-water value from our study was used to estimate activity concentrations in frogspawn based on available measurements of activity concentrations in water.
The data described here (Barnett et al., 2020) have a Digital Object Identifier (https://doi.org/10.5285/8f85c188-a915-46ac-966a-95fcb1491be6) and are freely available from the NERC-Environmental Information Data Centre (https://eidc.ac.uk/, last access: 13 November 2020). Data are made available under the terms of the Open Government Licence.
CLB arranged sampling sites, led field studies and data compilation, conducted some sample preparation and analysis, and made the initial draft of the manuscript. NAB helped define the study protocols, participated in the fieldwork and sample preparation, and wrote the final draft of the paper with CLB. MDW helped define the study protocols, participated in the fieldwork and sample preparation, and commented on the manuscript. MI conducted the sample analysis and commented on the manuscript. LAW participated in the field sampling and assisted with the initial processing of samples. RF participated in the field sampling, assisted with the initial processing of samples and commented on the manuscript.
The authors declare that they have no conflict of interest.
The authors are grateful to the Forestry Commission for providing permission to use the sampling sites and for their assistance in obtaining samples. We would also like to thank various staff members of UKCEH: Claire Wells for assistance during sample preparation and analysis, Claire Carvell for identifying the bee species, Alan Lawlor and Sarah Thacker for ICP-MS analysis of the water samples, and Jacky Chaplow for QC of the accompanying dataset. We also thank Scott Young of University of Nottingham for advice and assistance with sample analysis.
This study was funded by the NERC Environment Agency and Radioactive Waste Management Ltd. under the RATE programme as part of the TREE project (https://tree.ceh.ac.uk/, last access: 13 November 2020; grant no. NE/L000318/1).
This paper was edited by David Carlson and reviewed by Che Doering and two anonymous referees.
Allen, S. E.: Chemical analysis of ecological materials, 2nd edn., Blackwell Scientific Publications, Oxford, 1989.
Barnett, C. L., Wells, C., Izquierdo, M., Beresford, N. A., and Walker, L.: Element and radionuclide concentrations in wildlife (including representative species of the ICRP's Reference Animals and Plants) and associated soils from forests in north-east England, 2015–2016, NERC-Environmental Information Data Centre, https://doi.org/10.5285/8f85c188-a915-46ac-966a-95fcb1491be6, 2020.
Barnett, C. L., Beresford, N. A., Walker, L. A., Baxter, M., Wells, C., and Copplestone, D.: Transfer parameters for ICRP reference animals and plants collected from a forest ecosystem, Radiat. Environ. Biophys., 53, 125–149, https://doi.org/10.1007/s00411-013-0493-6, 2014.
Barnett, C. L., Beresford, N. A., Walker, L. A., Baxter, M., Wells, C., and Copplestone, D.: Element and radionuclide concentrations in representative species of the ICRP's reference animals and plants and associated soils from a forest in north-west England, NERC-Environmental Information Data Centre, https://doi.org/10.5285/e40b53d4-6699-4557-bd55-10d196ece9ea, 2013.
Beresford, N. A.: The transfer of radionuclides to wildlife (Editorial), Radiat. Environ. Biophys., 49, 505–508, https://doi.org/10.1007/s00411-010-0325-x, 2010.
Beresford, N. A. and Willey, N.: Moving radiation protection on from the limitations of empirical concentration ratios, J. Environ. Radioact., 208–209, 106020, https://doi.org/10.1016/j.jenvrad.2019.106020, 2019.
Beresford, N. A., Balonov, M., Beaugelin-Seiller, K., Brown, J., Copplestone, D., Hingston, J. L., Horyna, J., Hosseini, A., Howard, B. J., Kamboj, S., Nedveckaite, T., Olyslaegers, G., Sazykina, T., Vives i Batlle, J., Yankovich, T. L., and Yu, C.: An international comparison of models and approaches for the estimation of the radiological exposure of non-human biota, Appl. Radiat. Isot., 66, 1745–1749, https://doi.org/10.1016/j.apradiso.2008.04.009, 2008a.
Beresford, N. A., Barnett, C. L., Brown, J., Cheng, J.-J. Copplestone, D., Filistovic, V., Hosseini, A., Howard, B. J., Jones, S. R., Kamboj, S., Kryshev, A., Nedveckaite, T., Olyslaegers, G., Saxén, R., Sazykina, T., Vives i Batlle, J., Vives-Lynch, S., Yankovich, T., and Yu, C.: Inter-comparison of models to estimate radionuclide activity concentrations in non-human biota, Radiat. Environ. Biophys., 47, 491–514, https://doi.org/10.1007/s00411-008-0186-8, 2008b.
Beresford, N. A., Barnett, C. L., Howard, B. J., Scott, W. A., Brown, J. E., and Copplestone, D.: Derivation of transfer parameters for use within the ERICA Tool and the default concentration ratios for terrestrial biota, J. Environ. Radioact., 99, 1393–1407, https://doi.org/10.1016/j.jenvrad.2008.01.020, 2008c.
Beresford, N. A., Barnett, C. L., Brown, J. E., Cheng, J.-J., Copplestone, D., Gaschak, S., Hosseini, A., Howard, B. J., Kamboj, S., Nedveckaite, T., Olyslaegers, G., Smith, J. T., Vives I Batlle, J., Vives-Lynch, S., and Yu, C.: Predicting the radiation exposure of terrestrial wildlife in the Chernobyl exclusion zone: an international comparison of approaches, J. Radiol. Prot., 30, 341–373, https://doi.org/10.1088/0952-4746/30/2/S07, 2010.
Beresford, N. A., Yankovich, T. L., Wood, M. D., Fesenko, S., Andersson, P., Muikku, M., and Willey, N. J.: A new approach to predicting environmental transfer of radionuclides to wildlife taking account of inter-site variation using Residual Maximum Likelihood mixed-model regression: a demonstration for freshwater fish and caesium, Sci. Total Environ., 463–464, 284–292, https://doi.org//10.1016/j.scitotenv.2013.06.013, 2013.
Beresford, N. A., Wood, M. D., Vives I Batlle, J., Yankovich, T. L., Bradshaw, C., and Willey, N.: Making the most of what we have: application of extrapolation approaches in radioecological wildlife transfer models, J. Environ. Radioact., 151, 373–386. https://doi.org/10.1016/j.jenvrad.2015.03.022, 2016.
Beresford, N. A., Gaschak, S., Barnett, C. L., Maksimenko, A., Guliaichenko, E., Wells, C., and Chaplow, J. S.: A 'Reference Site' in the Chernobyl Exclusion Zone: radionuclide and stable element data, and estimated dose rates, NERC Environmental Information Data Centre, https://doi.org/10.5285/ae02f4e8-9486-4b47-93ef-e49dd9ddecd4, 2018a.
Beresford, N., Brown, J., Bonchuk, Y., Phillips, H., Thiessen, K., Ulanowski, A., and Yankovich, T.: Quantifying exposure of plants and animals to radiation: a new methodology, 3rd European Radiological Protection Research Week, Rovinj-Rovingo, Croatia, 1–5 October 2018, available at: http://nora.nerc.ac.uk/id/eprint/521145/ (last access: 1 June 2020), 2018b.
Beresford, N. A., Barnett, C. L., Brown, J. E., and Hosseini, A. (Eds.): CONFIDENCE Overview of model improvements and future needs, EJP-CONCERT, CONFIDENCE project Deliverable 9.17, available at: https://www.concert-h2020.eu/en/Publications (last access: 1 June 2020), 2019.
Beresford, N. A., Barnett, C. L., Gaschak, S., Maksimenko, A., Guliaichenko, E., Wood, M. D., and Izquierdo, M.: Radionuclide transfer to wildlife at a `Reference Site' in the Chernobyl Exclusion Zone and resultant radiation exposures, J. Environ. Radioact., 211, 105661, https://doi.org/10.1016/j.jenvrad.2018.02.007, 2020.
Brown, J. E., Alfonso, B., Avila, R., Beresford, N. A., Copplestone, D., and Hosseini, A.: A new version of the ERICA tool to facilitate impact assessments of radioactivity on wild plants and animals, J. Environ. Radioact., 153, 141–148, https://doi.org/10.1016/j.jenvrad.2015.12.011, 2016.
Copplestone, D., Bielby, S., Jones, S. R., Patton, D., Daniel, P., and Gize, I.: Impact assessment of ionising radiation on wildlife, R&D Publication 128, Environment Agency, Bristol, available at: https://www.gov.uk/government/publications/impact-assessment-of-ionising-radiation-on-wildlife (last access: 13 November 2020), 2001.
Copplestone, D. C., Beresford, N. A., Brown, J., and Yankovich, T.: An International database of radionuclide concentration ratios for wildlife: development and uses, J. Environ. Radioact., 126, 288–298, https://doi.org/10.1016/j.jenvrad.2013.05.007, 2013.
Forestry Commission: History of Redesdale Forest, available at: https://www.forestresearch.gov.uk/documents/6682/FCFH050.pdf (last access: 25 March 2020), 1951.
Forestry Commission England: Redesdale Forest design plan 2011, available at https://www.forestryengland.uk/sites/default/files/documents/Kielder Forest Plan Redesdale Forest Design Plan 2011.pdf2011 (last access: 29 April 2020), 2011.
Forestry Commission England: Harbottle and Holystone Forest plan 2016, available at https://www.forestryengland.uk/sites/default/files/documents/Harbottle and Holystone Forest Plan 2016.pdf (last access: 25 March 2020), 2016.
Galeriu, D., Beresford, N. A., Melintescu, A., Avila, R., and Crout, N. M. J.: Predicting tritium and radiocarbon in wild animals, International Conference on the Protection of the Environment from the effects of Ionizing Radiation, IAEA-CN-109, Stockholm, 186–189, https://inis.iaea.org/collection/NCLCollectionStore/_Public/35/005/35005520.pdf?r=1&r=1 (last access: 13 November 2020), 2003.
Guillén, J., Izquierdo, M., Young, S., Barnett, C. L., Wells, C., Beresford, N. A., Baeza, A., Salas, A., Muñoz-Serrano, A., Corrales-Vázquez, J. M., Muñoz-Muñoz, J. G., Tovar, E., and Chaplow, J. S.: Elemental concentrations in representative species of the ICRP's Reference Animals and Plants and associated soils in terrestrial Mediterranean ecosystems in Spain, NERC Environmental Information Data Centre, https://doi.org/10.5285/a1ab8c79-3426-43a4-ab42-6d1b218d1cc6,2017.
Guillén, J., Baeza, A., Izquierdo, M., Beresford, N. A., Wood, M. D., Salas, A., Muñoz-Serrano, A., Corrales-Vázquez, J. M., and Muñoz-Muñoz, J. G.: Transfer parameters for ICRP's Reference Animals and Plants in a terrestrial Mediterranean ecosystem, J. Environ. Radioact., 186, 9–22, https://doi.org/10.1016/j.jenvrad.2017.06.024, 2018.
Hirth, G. A., Johansen, M. P., Carpenter, J. G., Bollhofer, A., and Beresford, N. A.: Whole-organism concentration ratios in wildlife inhabiting Australian uranium mining environments, J. Environ. Radioact., 178–179, 385–393, https://doi.org/10.1016/j.jenvrad.2017.04.007, 2017.
International Atomic Energy Agency (IAEA): Radiation protection and safety of radiation sources: international basic safety standards, General Safety Requirements No. GSR Part 3, IAEA, Vienna, 2014a.
International Atomic Energy Agency (IAEA): Handbook of parameter values for the prediction of radionuclide transfer to wildlife, Technical Reports Series No. 479, International Atomic Energy Agency, Vienna, 2014b.
International Commission on Radiological Protection (ICRP): The 2007 Recommendations of the International Commission on Radiological Protection, ICRP Publication 103, Ann. ICRP 37, 2–4, 2007.
International Commission on Radiological Protection (ICRP): Environmental protection: the concept and use of reference animals and plants, ICRP publication 108, Ann. ICRP 38, 4–6, 2008.
International Commission on Radiological Protection (ICRP): Environmental Protection: Transfer Parameters for Reference Animals and Plants, ICRP Publication 114, Ann. ICRP 39, 2009.
Natural England: To take shrew (Soricidae) for scientific or educational purposes, or for the purpose of ringing or marking, or examining any ring or mark, WML-GL01, available at: https://assets.publishing.service.gov.uk/government/uploads/system/uploads/attachment_data/file/763850/gl01-shrews-licence.PDF (last access: 5 February 2020), 2017.
RIFE 2018: Radioactivity in Food and the Environment 2018, RIFE-24, available at: https://www.foodstandards.gov.scot/publications-and-research/publications/the-radioactivity-in-food-and-the-environment-rife-report-2018 (last access: 13 November 2020), 2019.
Spellerberg, I. F. and Gaywood, M. J.: Linear features: linear habitats & wildlife corridors, English Nature Research Reports No. 60, University of Southampton, Southampton, available at: http://publications.naturalengland.org.uk/file/154105 (last access 13 February 2020), 1993.
Tagami, K., Uchida, S., Wood, M. D., and Beresford, N. A.: Radiocaesium transfer and radiation exposure of frogs in Fukushima Prefecture, Sci. Rep., 8, 10662, https://doi.org/10.1038/s41598-018-28866-0, 2018.
Thørring, H., Brown, J., Aanensen, L., and Hosseini, A.: Tjøtta – ICRP Reference Site in Norway, Stralavn Rapport 2016:9, ISSN 1891-5191, Norwegian Radiation Protection Authority, Østerås, available at: https://dsa.no/sok/_/attachment/download/687e0a23-5ed3-4daf-9c1e-2e5cb218494b:ebc8cd54c993be743f8ae6dac4be78cf349af396/StrålevernRapport_09-2016.pdf (last access: 20 September 2020), 2016.
UK Parliament: Animals (Scientific Procedures) Act 1986: HMSO, London, 1994.
Westphal, C., Bommarco, R., Carre, G., Lamborn, E., Morison, N., Petanidou, T., Potts, S. G., Roberts, S. P. M., Szentgyorgyi, H., Tscheulin, T., Vaissiere, B. E., Woyciechowski, M., Biesmeijer, J. C., Kunin, W. E., Settele, J., and Steffan-Dewenter, I.: Measuring bee diversity in different European habitats and biogeographical regions, Ecol. Monogr., 78, 653–671, https://doi.org/10.1890/07-1292.1, 2008.
Yankovich, T. L., Vives, i Batlle, J., Vives-Lynch, S., Beresford, N. A., Barnett, C. L., Beaugelin-Seiller, K., Brown, J. E., Cheng, J. J., Copplestone, D., Heling, R., Hosseini, A., Howard, B. J., Kamboj, S., Kryshev, A. I., Nedveckaite, T., Smith, J. T., and Wood, M. D.: An international model validation exercise on radionuclide transfer and doses to freshwater biota, J. Radiol. Prot., 30, 299–340, https://doi.org/10.1088/0952-4746/30/2/s06, 2010.
Note that ICRP (2009) does not present ranges; however, those for the data reviewed by ICRP can be found here: http://www.wildlifetransferdatabase.org/downloadsummary.asp (last access: 1 June 2020), i.e. ICRP RAP tables (Feb 2011).