the Creative Commons Attribution 4.0 License.
the Creative Commons Attribution 4.0 License.
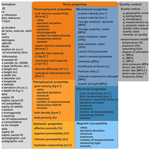
The PetroPhysical Property Database (P3) – a global compilation of lab-measured rock properties
Thomas Reinsch
Judith Bott
Petrophysical properties are key to populating local and/or regional numerical models and to interpreting results from geophysical investigation methods. Searching for rock property values measured on samples from a specific rock unit at a specific location might become a very time-consuming challenge given that such data are spread across diverse compilations and that the number of publications on new measurements is continuously growing and data are of heterogeneous quality. Profiting from existing laboratory data to populate numerical models or interpret geophysical surveys at specific locations or for individual reservoir units is often hampered if information on the sample location, petrography, stratigraphy, measuring method and conditions is sparse or not documented.
Within the framework of the EC-funded project IMAGE (Integrated Methods for Advanced Geothermal Exploration, EU grant agreement no. 608553), an open-access database of lab-measured petrophysical properties has been developed (Bär et al., 2017, 2019b: P3 – database, https://doi.org/10.5880/GFZ.4.8.2019.P3. The goal of this hierarchical database is to provide easily accessible information on physical rock properties relevant for geothermal exploration and reservoir characterisation in a single compilation. Collected data include classical petrophysical, thermophysical, and mechanical properties as well as electrical conductivity and magnetic susceptibility. Each measured value is complemented by relevant meta-information such as the corresponding sample location, petrographic description, chronostratigraphic age, if available, and original citation. The original stratigraphic and petrographic descriptions are transferred to standardised catalogues following a hierarchical structure ensuring inter-comparability for statistical analysis (Bär and Mielke, 2019: P3 – petrography, https://doi.org/10.5880/GFZ.4.8.2019.P3.p; Bär et al., 2018, 2019a: P3 – stratigraphy, https://doi.org/10.5880/GFZ.4.8.2019.P3.s). In addition, information on the experimental setup (methods) and the measurement conditions are listed for quality control. Thus, rock properties can directly be related to in situ conditions to derive specific parameters relevant for simulating subsurface processes or interpreting geophysical data.
We describe the structure, content and status quo of the database and discuss its limitations and advantages for the end user.
- Article
(12407 KB) - Full-text XML
- BibTeX
- EndNote
The characterisation and utilisation of subsurface reservoirs generally relies on applying geophysical investigation methods and/or numerical simulation codes – both requiring, in turn, the knowledge of physical rock properties at depth. The strategy of populating numerical models with petrophysical properties can differ. For local-scale models, laboratory data from individual samples collected from the geological unit of interest may exist. In this case, this direct information should be used together with sophisticated (physical and empirical) laws to populate the entire geological unit. For regional and continental-scale models, in contrast, parameters have to be generalised with respect to the spatial and physical variability of the investigated lithological units.
Individual rock types or petrographies typically exhibit a great variability in related properties due to heterogeneous mineral compositions, variable textures and differing porosity distribution (Schön, 2015). Existing rock property compilations are both an example for the high variability and for the different purposes of such databases (e.g. Cermak and Rybach, 1982; Clark, 1966; Clauser and Huenges, 1995a, b; Landolt-Börnstein et al., 2020; Mortimer, 2005; Hantschel and Kauerauf, 2009; Liolios and Exadaktylos, 2011; Descamps et al., 2013; Aretz et al., 2015; PetroMod, 2020). Since such compilations are mostly published with limited meta-information, it is difficult to extract data for formations of interest. This is even aggravated due to additional limitations like the focused coverage of certain rock types or geographic areas – e.g. Germany: FIS Geophysik hosted by the Leibniz Institute of Applied Geophysics (LIAG) (http://www.fis-geophysik.de, last access: 14 August 2020); Great Britain: BritGeothermal (http://www.britgeothermal.org, last access: 14 August 2020) hosted by the British Geological Survey (BGS); USA: National Geothermal Data System (NGDS) hosted by a federate infrastructure including national organisations and academia (e.g. the United States Geological Survey, Southern Methodist University, Association of American State Geologists, U.S. Department of Energy's Geothermal Data Repository, http://geothermaldata.org, last access: 14 August 2020); Ireland: IRETHERM project (http://www.iretherm.ie/, last access: 14 August 2020); Australia: Rock Properties Explorer (http://www.ga.gov.au/explorer-web/rock-properties.html, last access: 14 August 2020); New Zealand: PETLAB: National Rock and Geoanalytical Database (http://pet.gns.cri.nz/#/, last access: 14 August 2020); and many more.
In addition, different compilations do not provide a homogenised set of meta-information. Furthermore, exploration data availability often depends on national legislation. In some countries industrial resource exploration data, including petrophysical properties measured on cores of deep wells, may be public after a certain time period and then usually is incorporated in national information systems. In other cases exploration data remain confidential for longer time periods or even infinitely, resulting in scarce data availability for the respective countries.
Due to the current publication policy of international research institutions where a high number of peer-reviewed publications has become more and more important for the individual scientific career, the amount of petrophysical data recorded worldwide increased dramatically. These publications, however, are spread among many different geoscientific journals and dispersed in many hundreds of publications. Given the rate of newly published property data combined with the multitude of publishing journals, countries and authors, the research for and collection of data can be incredibly time-consuming. Recent studies show that domain experts spend nearly 80 % of their working hours collecting, cleansing and managing their domain-specific data (CrowdFlower, 2016). An effective, comprehensive collection, collation and dissemination of these data are deemed critical to promote rapid, creative and accurate research (Gard et al., 2019).
To facilitate (i) efficient search for and research on measured rock physical properties, (ii) further evaluation of the property data using complementing meta-information, and (iii) adequate property generalisation for specific units, a comprehensive database was developed within the framework of the EC-funded project IMAGE (Integrated Methods for Advanced Geothermal Exploration, grant agreement no. 608553). The aim of this database is to compile, store and publicly provide petrophysical property data from published laboratory test results on rock samples of any kind including as much meta-information as possible. So far, literature data relevant for the IMAGE project and laboratory data collected during the IMAGE project were fed into this novel PetroPhysical Property Database (P3). Here, we present the current state of P3 and release version 1.0 in excel format (Bär et al., 2019b: P3 – database, https://doi.org/10.5880/GFZ.4.8.2019.P3).
P3 is publicly accessible and contains physical rock properties measured in laboratory experiments. It is licensed under a creative commons (CC-BY 4.0) license, and its structure follows the FAIR guiding principles for scientific data management and stewardship (Wilkinson et al. (2016). All data are selected to represent the characteristic scale of rock samples of a few centimetres to decimetres, depending on the measurement methods (as described by numerous norming institutions or committees such as the International Society for Rock Mechanics and Rock Engineering (ISRM), European Committee for Standardization (C)EN, International Organization for Standardization (ISO), American Society for Testing and Materials (ASTM International) and many more) for the different properties. Within P3 we aimed at homogenising measurement method descriptions to increase the inter-comparability between individual reported values. Larger-scale data from geophysical well logging, hydraulic well testing, integrating geophysical methods or other field-scale measurements, which integrate over larger rock volumes or several rock types, have not yet been included in the database (Fig. 1). This shall reduce bias introduced by heterogeneities within larger geobodies including open or partly open discontinuities like fissures, fractures, bedding or schistosity. In addition, judged based on the lithological description, we did not include data from very small scale samples, where the volume of interest is likely smaller than the minimum representative elementary volume (REV) (e.g. Ringrose and Bentely, 2015) for the investigated rock type. The full range of the scale dependency of petrophysical properties as described in previous studies (e.g. Enge et al., 2007; Jahn et al., 2008; Howell et al., 2014; Rühaak et al., 2015) is thus not yet reflected by the database but is planned to be incorporated in future versions.
To ensure that source data are publicly available to researchers, only data from scientific publications (books or peer-reviewed journals) or proceedings (e.g. IGA Geothermal Papers/Conference Database) as well as published research reports (e.g. dissertations or publicly available student theses, project reports) were included in P3. The database only contains measurements with a minimum amount of meta-information to allow for reasonable interpretations, generalisations or simulations based on the collected data. The minimum associated meta-information is the reference to the data origin (citation) and information about the petrography to allow for a classification according to a certain lithotype. If available, additional meta-data were included, such as the sampling location (potentially including its type, e.g. outcrop, abandoned or active quarry, vertical or deviated well), the affiliation to a registered sample set (e.g. International Geo Sample Number (IGSN, cf. Devaraju et al., 2016; Lehnert et al., 2006)), stratigraphy, sample dimensions, measurement method, or device and measurement conditions (pressure, temperature, stress) including degree of saturation and type of saturating fluid. Conversion of published values to SI units as well as correction of some minor errors from published data or omissions from previous databases as they are identified is an ongoing process during the data curation.
The database was developed as a flat-file format using Microsoft Excel to keep it as simple and easy to handle even by the unexperienced user as possible. While other database structures are in comparison much more efficient, their database management schemes may render it too difficult for users not familiar with SQL to recover the desired data. However, the internal design of P3 with multiple sub-entities and tables is structured following a relational database management system (RDBMS, Codd, 1970) with an entity-relation (ER) model (see Appendix B) so that it could easily be transferred to for example the well-established structured query language (SQL, Chamberlin and Boyce, 1974). Following this ER model the database could easily be organised into multiple tables using the names of the tables as unique keys and as links to other sub-tables. The main advantages of a relational database over a flat-file format are that data are uniquely stored just once, eliminating data duplication, as well as performance increases due to greater memory efficiency and easy filtering and rapid queries (Gard et al., 2019). However, the current flat-file structure allows for easy modification and extensions as new requirements emerge; for example, by adding more sub-tables for newly developed property, measurements not fitting to any of the already included properties could be added at later stages. On the other hand, filtering and quality control to ensure that data are entered into the database only once and that no duplicates exist had to be done manually. In our case data duplicates where removed by checking the coordinates of each data point with a radius of uncertainty of 1 km and, if necessary, manually removing every double entry identified.
Following the minimum requirements, the database is structured into three main sections or super-entities (Fig. 2), which are sets of data tables (described in more detail in the following parts of the paper). The first, named “meta-information”, contains all meta-information on the sample including the sampling location, the sample type, and dimensions as well as information on its petrography and stratigraphy and thus acts as a primary table for unique sample identification. The second section or super-entity contains the measured property value(s) of the unique rock samples. This section is subgrouped into thermophysical properties, classical petrophysical properties, mechanical properties, and electrical and magnetic properties and fields for property-specific remarks. Finally, the third section or super-entity named “quality control” includes all information relevant for the quality assessment of each data record (property measurement of the unique samples). Here, especially information on the measurement conditions (methodology, pressure and temperature conditions, degree of saturation) is documented and used for the implemented semi-automatic quality control and assessment.
The first super-entity, meta-information, consist of five tables or entities: sample ID, reference, sampling location, sample information, petrography and stratigraphy. A description of each of these tables is included in the following sub-chapters. The tables for petrography and stratigraphy are available separately. The super-entity “rock properties” contains 28 separate sub-tables for all properties included so far into the database each following a similar internal structure (see Sect. 2.4). For many samples, measurements of multiple properties were available and included into the database, which results in multiple documentation of the meta-information of these samples in the current file structure. The super-entity quality control contains two tables or entities – the first one for documentation of the measurement conditions and the second one for the automated quality assessment of the entries (see Sect. 2.5).
2.1 Sample information
To distinguish measurements of different properties on a single sample or of the same properties performed at varying measurement conditions, every measurement is listed in a separate row. To group measurement data from individual samples, every sample receives a unique sample ID, which acts as the primary key of each record and links multiple measurements conducted on a single rock sample. The sample ID consists of the surname of the first author and the year of publication, together with a sequential number for the particular rock sample presented in the respective publication. In the case of several references per author and year, an additional letter (a, b, …) is introduced after the year.
For example, Fourier1822_1 stands for sample 1 within a publication of Fourier (1822). In the case of more than one publication per year, Fourier1822a_1 would represent sample 1 within a publication of Fourier (1822a). The sample ID is linked to an accompanying reference database, compatible with all major reference management tools (e.g. EndNote, Citavi, BibTeX, and JabRef), which contains the full information (co-authors, full title, journal, volume, pages, etc.) in the reference. The references are abbreviated in a Bibtexkey according to the terminology used for individual samples. At best, only primary references are given. In cases where the primary reference is unavailable, while the data point is published as part of a review (or the like), a secondary reference was introduced.
Additionally, the date of input and the name of the person who generated the entry into the database (the editor, listed as contributors in Appendix A, team list) are documented.
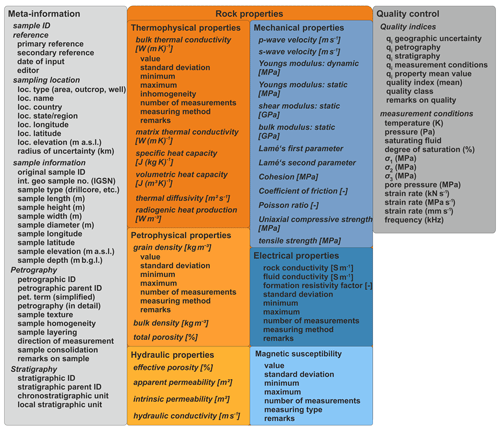
Figure 2Schematic structure of P3 illustrating the three sections or super-entities: meta-information, rock properties and quality control. Different input parameters (small font) are grouped according to the entities or property sub-tables (italics) they belong to.
2.1.1 Sampling location
The subsection “sampling location” in the P3 database contains all relevant information on the location where a sample was obtained. Generally, rock samples can be sampled in an outcrop, a quarry or a well. In cases where neither the sampling location is given as outcrop, quarry, or well nor any exact coordinates are given in the corresponding publication, the location type area is selected. Furthermore, for every location type, a name, a country and a state are given (e.g. location type: outcrop, location name: Fontainebleau, location country: France, location state/department: Seine-et-Marne).
2.1.2 Location coordinates
The location coordinates describe the latitude and longitude with the reference system WGS84 of the sampling point at the surface in decimal degrees. Another category of entry is the elevation given in metres above sea level (m a.s.l.). In the case of a core sample taken from a well, the latitude and longitude of the wellhead is given. In the case of an area with an undefined sampling point, e.g. sample from the Rhenish Massif, a midpoint from this geological province was assessed and a radius of uncertainty (in km) for the sampling location was estimated. For elongated areas (e.g. the Red Sea and the Upper Rhine Graben) the choice of a circular radius of uncertainty artificially increases the uncertainty. The introduction of polygons for the definition of an area is discussed to be included in future releases of the database. If no information is given for the location, the longitude and latitude are noted as 999 to avoid wrong map displays, and half the circumference of the earth is used as uncertainty.
For a conversion of the sample coordinates retrieved from the literature, we used either the map publisher Google Earth (Web Mercator projection) or the Geographic Information System (GIS) software ArcGIS to allocate a latitude–longitude value in decimal degrees and a rough estimation of the associated uncertainty to each data point. Exact geographic information is quite often not provided in the literature used for this compilation. Most common is the provision of location names or maps only. For all literature data points where both the exact coordinates and the reference system was given, or where the location was given on a georeferenced map with the required information on the coordinate system used, we used ArcGIS for transformation. Therein, we used the same geographic projection as given in the original literature and either included the points as tabular values or we georeferenced the given maps accordingly and picked the points on the maps. Afterwards, the resulting coordinates were transferred to decimal degrees in the WGS84 reference with the transformation method for the specific projected coordination system as suggested by ArcGIS.
2.1.3 Original sample ID
To allow for reviewing original publications, the primarily given sample identification numbers or names are documented in addition to the P3 sample ID. This makes it easier to search for a specific sample in a publication, which might have been used for further measurements or more detailed descriptions by other authors subsequently or individual users of the database.
2.1.4 International Geo Sample Number
The International Geo Sample Number (IGSN, cf. Devaraju et al., 2016; Lehnert et al., 2006) is a unique identifier for samples and specimens collected from the natural environment (http://www.igsn.org/, last access: 14 August 2020). In order to enable locating, identifying and citing physical samples, the IGSN number was listed if available. Furthermore, entries allow for cross-linking both the P3 and the IGSN database in order to ensure access to more meta-information like sampling methods and project-related information currently not implemented in P3. As described by Strong et al. (2016) the adoption of IGSNs will ensure compatibility and interoperability with other international databases, including the promotion of standard methods to locate, identify and cite physical samples.
2.1.5 Sample type
Samples can have different shapes that are particularly relevant for the measurement technique. Core samples do have different characteristics than rock blocks or drill cuttings so that P3 reserves a separate column for the sample type.
2.1.6 Sample dimensions (m)
Together with the documentation of the sample type, if available, information about its length, height, and width as well as diameter for cores, all given in metres, are documented. If the rock property “density” is measured for any sample where the dimensions are given, sample volume and weight might be calculated as well. This additional information together with its petrography was essential to evaluate whether a sample reaches a representative elementary volume (REV) or not.
2.1.7 Sample coordinates
For several samples taken at a single sampling location (e.g. a large outcrop or quarry), eventually individual sample coordinates are given (longitude, latitude and elevation). For samples from a cored well, additionally, the depth of the sample is given in measured depth (MD) and, if available, in true vertical depth (TVD) referenced to the ground level (i.e. metres below ground level, m b.g.l.). If data on the geometry of deviated wells are available, it is optional to enter the sample location either relative to the wellhead or with its exact location and elevation (with respect to the sea level).
2.2 Petrography or rock type
The petrography or rock type classification scheme is defined in a complementary database (Bär et al., 2019a: P3 – petrography, https://doi.org/10.5880/GFZ.4.8.2019.P3.p) directly published together with P3. Its internal structure is based on a hierarchical subdivision of rock types, where the rock description generally becomes more detailed with increasing rank of petrographic classification (based on the well database of the Geological Survey of Hesse, Germany: Hessisches Landesamt für Umwelt, Naturschutz, Umwelt und Geologie, HLNUG). This hierarchical subdivision is based on international conventions (e.g. Bates and Jackson, 1987; Gillespie and Styles, 1999; Robertson, 1999; Hallsworth and Knox, 1999; Le Bas and Streckeisen, 1991; Schmid, 1981; Fisher and Smith, 1991). Furthermore, the classification corresponds to the subdivision provided by existing property data compilations such as Hantschel and Kauerauf (2009), Schön (2011), and Clauser and Huenges (1995a, b).
Petrographic classifications from rank 1 to rank 4 can usually be identified from macroscopic descriptions of well logs, cores and geological mapping (Fig. 3). The petrographic classifications from rank 5 to rank 9 require additional information on the texture or grain size, the modal composition, or the geochemistry, which can usually only be acquired by microscopic or comparable special investigations. Overall, there are nine ranks covering a total of 1494 petrographies. The petrographic classification of a sample in P3 is based on the sample description within the original literature reference. A petrographic ID and a corresponding petrographic parental ID directly correlate the different classifications and their ranks (Table 1). This allows, for example, integrating all petrographies with higher ranks to a corresponding general term of lower rank and statistically analysing the associated physical rock property values across petrographic definition boundaries (Fig. 3).
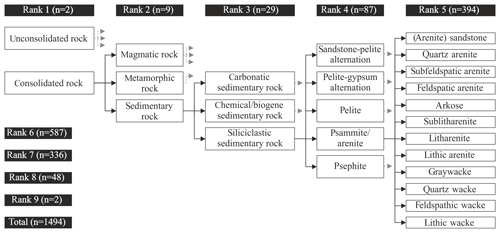
Figure 3Hierarchical system of standardised petrographic terms used for the database. White boxes are sample chosen extracts to illustrate the structure of the petrography classification. Black boxes document the number of rock type categories per rank for the entire classification scheme. These interconnected standardised terms allow for the connection of certain lithologies/petrographies to specific petrophysical properties and are thus the basis for statistical analysis. Black arrows show direct connections, while grey arrows indicate that there are additional terms not displayed here.
In P3, the petrographic ID, the petrographic parent ID and the simplified petrographic term are documented. Additionally, for each sample, original petrographic descriptions of the primary references can be presented if available. Details on the texture, homogeneity, layering, consolidation state of the sample and the direction of measurement with regard to internal structural features (such as bedding) as well as the degree of alteration or weathering can be documented together with specific remarks.
Table 1Excerpt from the rock classification table used for P3. Different ranks and their interconnection by petrographic ID and petrographic parent ID as well as their connection to international definitions as indicated. QAPF: quartz–alkali feldspar–plagioclase–foids; PL: plagioclase (Le Maitre and Streckeisen, 2003).
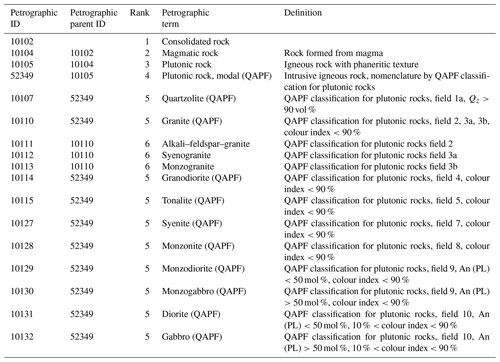
2.3 Stratigraphy
The stratigraphy of each sample was inserted into the database in two complementary ways. The first way is to use the definitions of the international chronostratigraphic chart of the IUGS v2016/04 (Cohen et al., 2013, updated) according to international standardisation. These chronostratigraphic units are also compiled in a complementary database (Bär et al., 2019a: P3 – stratigraphy, https://doi.org/10.5880/GFZ.4.8.2019.P3.s) to ensure that formations of a certain age are connected to the corresponding stratigraphic epoch, period or erathem. Thus, the chronostratigraphic units are directly correlated to each other by their stratigraphic ID and stratigraphic parent ID, allowing for statistical analysis of the properties of certain stratigraphic units (Table 2). In contrast, a more detailed description of the local stratigraphic unit can also be documented if provided in the primary reference.
2.4 Petrophysical properties
The properties included in P3 can be grouped into classical petrophysical properties, thermophysical properties, mechanical properties, and electrical and magnetic properties (Fig. 2). Overall, 28 different rock properties have been included so far and documented in separate sub-tables of the database following a similar internal structure. Based on the original reference, the measurement is given as a value, which if available is complemented by a standard deviation, a minimum and maximum value, and the number of measurements. Thus, it is possible to include either single measurements or mean values while still offering the opportunity of statistical evaluation by incorporating the number of measurements corresponding to a mean value. Furthermore, the measurement method for each property value is presented by means of a common nomenclature documented in the supplementary report (Bär et al., 2019b: P3 – data description, https://doi.org/10.5880/GFZ.4.8.2019.P3). This is important for statistical analysis and comparability of the results of different methods. Particularly, the type of method might have a large impact on the quality and device-specific error of any measurement. Finally, specific remarks can be made for each value separately.
2.5 Quality control
In addition to the primary option of manual database quality control, which is by providing the information of the original data source, an automatic process of quality control was implemented in P3. Therefore minimum requirements for a value to be included in the database were defined as already described in Sect. 2.
To provide a quality estimate for each data entry in terms of provided meta-information, a set of key criteria is automatically analysed: (i) uncertainty of the geographic location, (ii) the rank of petrographic classification, (iii) the rank of stratigraphic classification, (iv) the completeness of information on measurement conditions, and (v) the statistical type of a value (e.g. single value and mean value). For each key criterion, four different quality classes (excellent =1, average =2, poor =3; and minimum) are defined and computed to numerical quality indices (qi, Table 3). A bulk quality index is calculated according to the arithmetic mean of the quality indices of the different criteria, where values <1.5 are considered excellent, values are considered average, values ≥2.5 are considered poor and values >3.5 only meet the minimum requirements.
2.5.1 Geographic uncertainty
Concerning the location of the sample, a geodetic accuracy of less than 100 m is considered to be excellent quality, which should always be the case for outcrop samples or drill cores. If the information on the location only contains a description of a geological unit in a certain region or area, the related size of this area is considered for the definition of the quality indices. If the location can be constrained to a region with a radius of less than 1 km, the quality is considered average, whereas if the radius of uncertainty is between 1 and 100 km, it is considered poor. A larger radius of uncertainty is considered as quality class 4.
2.5.2 Petrography or rock type
If the original petrographic or lithological description allows for the allocation of a petrographic term with a rank of 6 or higher, the quality is considered excellent; for a rank of 5 it is considered average because these petrographic terms usually allow for a distinction of petrographies as used for reservoir- or site-scale geological models. For a rank of ≤4 the quality is considered poor (compare Fig. 3 and Table 1). To enter the database at all, the petrographic description of a sample has to allow for an allocation of a petrographic term of rank ≥2. This classification at least allows for a distinction of petrographies on a level used for continental-scale geological models.
2.5.3 Stratigraphy
Concerning the stratigraphy of the sample, (i) information on the chronostratigraphic stage or age is considered to be excellent; (ii) information on the stratigraphic series or epoch is defined as average; and (iii) if only the chronostratigraphic system or period is given, it is considered poor. To enter the database, there is no minimum requirement for the information on the stratigraphic age, since (i) stratigraphy does not directly control physical properties and (ii) scientific users might retrospectively derive stratigraphic information from the sampling location in combination with the petrography of the sample and additional information such as geological maps.
2.5.4 Measurement conditions
For every data point, the measurement conditions can be entered. These are the temperature (K), pressure (Pa), saturating fluid, and the degree of saturation (%), as well as for the mechanical properties additional information about the ambient stress field, σ1, σ2, σ3 (MPa) and the pore pressure of the sample (MPa). For the sonic velocities (vp and vs) the frequency of the sonic pulse and, for the uniaxial compressive strength and related mechanical properties, the strain rate can be given as additional measurement conditions.
The quality assessment of the measurement conditions is based on both the measurement conditions and the measurement device, which is needed to be able to quantify the specific measurement error typical for a certain method. Excellent quality is only provided if information is available on all these points. If only the measurement device and the temperature and pressure conditions or the degree of saturation are available, the data quality is defined as average. If only the device, the temperature and pressure conditions, or the degree of saturation is described in the original reference, the quality is considered to be poor.
2.5.5 Measurement parameter
The last criterion for the quality control is the type of value representing the property. In general, single measurement values for a sample are ranked higher in quality than mean values of various measurements applied to a sample. Accordingly, single measurements are considered to be excellent and mean values are considered to be average or poor. If the mean value is not only accompanied by the number of measurements to calculate the mean value, but also by the minimum and maximum as well as the standard deviation from this set of measurements, the quality is defined as average. In contrast, a mean value accompanied only by a number of measurements is defined as poor. Values resulting from an unspecified number of measurements are not considered for quality control but still included into the database with NA (not available) in the respective column for a number of measurements to enable the user to exclude these values in statistical analyses.
Up to now, data that entered the database are either from published data collections, scientific papers, student's theses and scientific projects, or technical reports (316 references altogether; see Appendix A). So far, 75 573 data points from all over the world (Figs. 4 and 5) have been collected. The data are not reasonably good around the globe but rather show a strong dominance of samples sourced from central Europe and the United States. This reflects the original purpose of the IMAGE project as well as public availability of existing databases. Data are only scarcely available for Africa, South America, Australia, Russia and China.
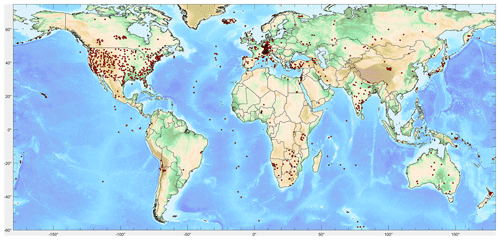
Figure 4Locations of all data points currently included in P3 (for references see Appendix A). Topographic map is the ETOPO1 map (Amante and Eakins, 2009).
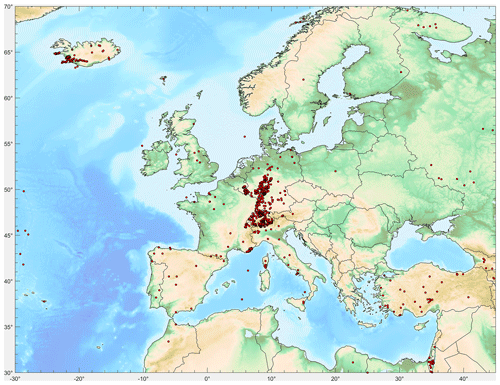
Figure 5Locations of data points currently included in P3 for Europe (for references see Appendix A). Topographic map is the ETOPO1 map (Amante and Eakins, 2009).
The number of data entries for different petrographies shows that all main consolidated rock types are well represented. With 38 219 property measurements from sedimentary rocks, 25 261 from magmatic rocks, 9235 from metamorphic rocks and 1308 from unconsolidated rocks, petrographies usually considered as reservoir rocks are dominant, making up more than 75 % of the data.
Since P3 was collected to serve the goals of the IMAGE project and will always represent work in progress, its data entries are unevenly distributed among the different properties (Table 4) as well as regions. In its current version, the entries for some properties derive from only a few sources. For example, radiogenic heat production values contained in the database have mainly been derived from the compilation of Vilà et al. (2010). This compilation, which is based on many secondary references, includes more than 2100 representative U, Th and K concentrations from all over the world (originally published in 102 studies). Based on this chemical composition database, Vilà et al. (2010) calculated values of radiogenic heat production for a large variety of rock types. Of the original compilation (of Vilà et al., 2010), we have incorporated into the database only those values that were associated with sufficient metadata and based on actual lab investigations and not on spectral gamma ray and density data of borehole geophysical logs. Newer compilations on radiogenic heat production (e.g. Hasterok and Webb, 2017; Hasterok et al., 2018) have not yet been included.
Concerning the data quality, the quality indices both for the bulk index and for the five indices defined in Table 3 show a wide dispersion over all quality classes. The quality indices for the petrography, the geographic uncertainty and the measurement parameter show mainly quality values of 1 to 3 representing a good quality of input data documentation on average. Only the quality indices for measurement conditions and for the stratigraphy where quality index values of 3 and 4 are dominant show that the documentation of this metadata is not satisfactory for a large share of the compiled data.
The current status of the database already shows a lot of benefits that such a compilation has automatically brought along but also some limitations, which have to be addressed in future amendments. The defined minimum requirements for a datum to be integrated into P3 guarantee its usability in terms of statistical, spatial, petrographic and stratigraphic analyses. Since it also contains multiple properties measured on a single sample, direct correlations with other data and properties are facilitated. This may help in identifying new relationships (formal, causal or statistical correlations) and contribute to a better understanding of the limitations of generalisation or possibilities for upscaling approaches. The automatic quality assessment allows for a quick evaluation of a single datum within a group of selected entries. The possibility of correlating data also simplifies and accelerates the identification of key references for rock parameters in specific regions, for specific rock types, or stratigraphic units. Furthermore, the database allows us to systematically analyse the dependency of property values on the corresponding measurement conditions. Thus, the most important added value of P3 compared to existent databases is its dimension (large number of entries corresponding to a large number of petrophysical properties) as well as the documented meta-information.
Despite all benefits, such a database can never be complete and is always prone to uncertainties. To identify errors in original publications (in terms of property values and meta-information, e.g. sample preparation, accuracy of measurements, sampling bias, lab worker bias, measurement methods, reference standards and many more) is beyond the scope of this compilation. In addition, data-input errors, errors concerning the interpretation, or the petrographic and stratigraphic classification cannot be excluded. We assume that the quality check of the original publications and the data therein have already been done by skilled reviewers or editors of the corresponding scientific journals and theses. In addition to that, the quality indices developed as part of P3 allow the user to quickly evaluate the quality of each data point and thus help with the decision of whether the original reference should be reassessed or not.
Additionally, P3 includes values generated with different established or newly developed measurement methods, delivering data of different quality and uncertainty. Hence, data comparability is not necessarily granted, and a statistic assessment can only be representative if these effects are considered. Due to the documentation of the original source, however, the related detailed information of a chosen sample set can be verified if necessary. For subsequent applications, such as modelling, the spatial distribution of the data has to be considered as well as the origin of the samples. Due to diverse effects (such as temperature, pressure, weathering and diagenetic history), properties measured from outcrop analogue samples might differ considerably in quality from those of the same formation at in situ conditions within a deep reservoir formation. It is up to the experienced user to evaluate if the tabulated datum is applicable and if sufficient meta-information is given. In case of doubt, the users are referred to the original publications.
The excel version of the P3 database (Bär et al., 2019b: P3 – PetroPhysical Property Database, V. 1.0, GFZ Data Services, Potsdam, https://doi.org/10.5880/GFZ.4.8.2019.P3) is available under the given DOI.
The petrographic classification table (Petrography), which is included in P3 (Bär and Mielke, 2019: Petrographic classification table (Petrography): P3 – Petrography, V. 1.0, GFZ Data Services, Potsdam, https://doi.org/10.5880/GFZ.4.8.2019.P3.p), is available under the given DOI.
The stratigraphic classification table (Stratigraphy), which is also included in P3 (Bär et al., 2019a: Stratigraphic classification table (Stratigraphy): P3 – Stratigraphy, V. 1.0, GFZ Data Services, Potsdam, https://doi.org/10.5880/GFZ.4.8.2019.P3.s), is available under the given DOI.
We developed the P3 database of petrophysical rock properties measured on rock samples in various laboratories. P3 is designed to be as transparent and useful for various purposes as possible through the integration of multiple sources of meta-information (including the original reference) for each data point. The database already comprises a great variety of properties, petrographies, stratigraphies, etc. from samples investigated all over the world. In this first release, 75 573 data points from 316 publications were included. The current compilation of samples mainly reflects the project goals of the geothermal project IMAGE (van Wees et al., 2015), while the applicability of P3 certainly can be seen in various geoscientific fields focusing on subsurface utilisation (e.g. oil and gas, carbon capture and storage (CCS), hydrogeology, and subsurface storage of radioactive waste). The collected data will help researchers and users particularly in the early stages of new geothermal or any other projects to make a first assessment of the subsurface geothermal rock properties. This will help planning future exploration needs and, in areas where the existing data density is sufficient, even support direct modelling or exploitation projects. Additionally, the database will help improve local and regional geoscientific studies with a different focus on the utilisation of the subsurface. A first release of this database (Bär et al., 2019b: P3 – database, https://doi.org/10.5880/GFZ.4.8.2019.P3) including a report and a reference list of all included publications is available as supplementary data to this publication.
Compiling the data from various sources, however, has shown that the general documentation of measured petrophysical properties is very heterogeneous, and often the minimum requirements defined for our P3 were not fulfilled. We therefore appeal to the reviewers and editors of scientific journals to ensure that any publication containing original measurements of petrophysical properties should come along with all the helpful and necessary meta-information as described here. Only if these requirements are fulfilled, a published dataset is of added value for the scientific community and can be used for subsequent investigations or applications.
Since a database like P3 can never be complete, a further extension based on not-yet-considered publications, newly published data or own measurements is foreseen. Furthermore, we both hope to collaborate with existing compilation authors in the future to combine the collations into one more useful systems but also support the use of this version of the P3 database for other database initiatives as a supplement of their own records. We plan to develop a publicly accessible web-based interface to facilitate the ability of external users to perform specific queries on petrophysical properties. In addition, such queries shall be feasible based on a web-based geographic information system, which may be connected to additional information such as worldwide geological maps (e.g. OneGeology, http://www.onegeology.org, last access: 14 August 2020). With this system, external users shall be given the opportunity to contribute to the database and thereby simplifying the access to measurements, which may improve their visibility considerably. Thus, the database will be continuously updated and at certain stages newly released by the editors. For this purposes the database will be implemented using a relational database management system (RDBMS) following the third normal form (3NF) according to Codd (1970) and Maier (1983) to reduce the volume of stored data by elimination of multiple storage of the same information for a sampling location, which will strongly increase its flexibility, durability and applicability especially for the SQL-experienced user. This will facilitate the linking of P3 to similar databases in the future.
To broaden the applicability of P3 for reservoir characterisation in the future, the integration of lab measurement or geophysical exploration methods aiming at the determination of petrophysical reservoir properties on a smaller or larger scale is currently in discussion and will allow us to include information on the scale dependence of petrophysical properties (e.g. Enge et al., 2007) which are of paramount importance for understanding of reservoir behaviour. This could include properties derived from geophysical well logging (Hartmann et al., 2005; Fuchs et al., 2015), hydraulic testing in wells (Achtziger-Zupančič et al., 2017, and references therein) or other integrating geophysical exploration methods, e.g. seismics (Gu et al., 2017), gravimetry or electromagnetic methods (e.g. Munoz et al., 2010; Meqbel and Ritter, 2015). Furthermore, additional information on the sample like their geochemical or modal composition from X-ray fluorescence (XRF), inductively coupled plasma mass spectrometry (ICP-MS) or inductively coupled plasma optical emission spectrometry (ICP-OES) analyses, point counting of thin sections or electron microscopic investigation of e.g. cementation, pore geometry or microfractures, which can all act as controlling factors of petrophysical properties, would be a helpful extension of the database. Therefore existing databases could be easily implemented (e.g. Lehnert et al., 2000; Mortimer et al., 2005; Strong et al., 2016; Gard et al., 2019, and references therein). This will extend the opportunity to also use the database to derive phenomenological constitutive models for petrophysical rock properties from their chemical or mineralogical composition (Chopra et al., 2018; Gard et al., 2019) or from their microstructure (Pimienta et al., 2014) or to develop empirical correlations between distinct properties of certain rock types (Gegenhuber and Schön, 2012; Esteban et al., 2015; Mielke et al., 2017).
Adelinet et al. (2013), Alam et al. (2011), Altherr et al. (2000), Aretz (2015), Ashwal et al. (1987), Aswathanarayana (1986), Atal et al. (1978), Attoh (2000), Babaie et al. (2001), Bär (2008, 2012), Baker et al. (1997), Balakrishna and Ramana (1968), Ballard et al. (1987), Barrett and Aumento (1970), Bauluz et al. (2000), Bea and Montero (1999), Bemmlott (2014), Best and Christiansen (2002), Betten (2015), Bhatia and Crook (1986), Biewer (2014, 2017), Birch and Clark (1940), Birch (1942), Blackwell and Richards (2004), Brady et al. (2006), Brandt et al. (2004), Brehme et al. (2016a, b), Brettreich (2016), Bridgman (1924), Brigaud et al. (1992), Brown et al. (1981), Bullard (1939), Bultitude et al. (1978), Buntebarth (1980), Carroll (1969), Cebriá et al. (2000), Ceryan (2008), Ceryan et al. (2008), Chapman and Pollack (1977), Chappell (1999), Chung (1999), Chung et al. (1995, 2001), Clark (1957, 1961, 1966), Clauser (2001), Cocherie (1984), Cocherie et al. (1994), Condie (1993), Coster (1948), Côté and Konrad (2005), Crecraft et al. (1981), Creutzburg (1965), Dahmani and Sawyer (2001), Damm et al. (1990), Dickson and Scott (1997), Diment (1964), Dodge et al. (1986), Dongmo (2016), Ducea and Saleeby (1998), Dupré and Echeverría (1984), Eberhard (2005), El Dakak (2015), Ensor (1931), Esteban et al. (2015), Ewart et al. (1973, 1977), Faridfar (2010), Farmer (2003), Farmer et al. (2002), Farquharson et al. (2016, 2017), Förster and Förster (2000), Fountain et al. (1987), Fourcade and Allegre (1981), Fowler et al. (2005), Francois and Lemmet (1999), Franzson et al. (2010, 2011), Fridleifson and Vilmundardottir (1998), Fuji-ta et al. (2004, 2007, 2011), Gärtner (2017), Galán et al. (1996), Gangadharam and Aswathanarayana (1969), Gao et al. (1998), Garcia-Gutierrez and Contreras (2007), Gaunt et al. (2014), Gehlin (2002), Gehlin and Hellstrom (2003), Geist (2011), GeORG-Projektteam (2013), Ghazi and Hassanipak (1999), Gill (1981), Gill and Whelan (1989), Glover (1989), Glover and Vine (1992, 1994, 1995), Grecksch et al. (2003), Grunert (2007), Gu (2010), Gudmundsson et al. (1995), Gühne (2016), Guillot and Fort (1995), Guillou-Frottier et al. (1995), Gupta et al. (1991), Haack (1983), Haffen et al. (2012, 2013), Hartmann et al. (2005), Hauff et al. (2000), Heap et al. (2009, 2010, 2011, 2012, 2014a, b, 2015a, b, c, 2016), Herrin and Clark (1956), Hesse (2011), Hill et al. (1981), Hoffmann (2011, 2015), Hofmann (1988), Homuth (2014), Hooper and Hawkesworth (1993), Huber et al. (2001), Hückel and Kappelmeyer (1966), Hurtig and Brugger (1970), Hutt and Berg (1968), Hyndman and Jessop (1971), Inger and Harris (1993), Innocent et al. (1994), Islam (2010), Iyer et al. (1984), Jaupart et al. (1982), Jaya et al. (2010), Jensen (2014), Jeong et al. (2007), Jochum et al. (1983), Jodocy and Stober (2011), Johannes et al. (2003), John and Wooden (1990), Jones (1987, 1988), Kahraman (2007), Kalsbeek et al. (2001), Kappelmeyer and Haenel (1974), Kassab and Weller (2011, 2015), Kawada (1964, 1966), Kay and Kay (1994), Kay et al. (1990), Keen and Lewis (1982), Kelemen et al. (2007), Kemp and Hawkesworth (2003), Kendrick et al. (2013a, b), Kennedy and Russell (2012), Kennedy et al. (2009), Kerr (2003), Khitarov et al. (1959), Kidder et al. (2003), Kläske (2010), Klein (2003), Klug and Cashman (1996), Klumbach (2008, 2010), Knopoff (1968), Königsberger and Weiss (1911), Kolzenburg et al. (2012), Konzack (2015), Kramers et al. (2001), Kraus (2009), Kristinsdóttir et al. (2010), Kukkonen et al. (1997), Kumar and Reddy (2004), Kumari et al. (2017), Lambert (2016), Laštovičková et al. (1993), Ledesert (1993), Leonidov (1967), Leonidov et al. (1966), Lesquer et al. (1983), Leu et al. (2006), Linsel (2014), Loaiza et al. (2012), Luais and Hawkesworth (1994), Lucazeau and Mailhe (1986), Mack (2007), Mahood (1981), Maire (2014), Manghnani and Woollard (2013), Mareschal et al. (2000), Mariucci et al. (2008), Marzán Blas (2000), McDermott et al. (1999), McDonough (1990), McKenna and Sharp (1998), McLaren et al. (1999), McLennan et al. (1990), Mengel et al. (2001), Mielke (2009), Mielke et al. (2015), Milicevic (2015), Milord et al. (2001), Misener et al. (1951), Mitchell (1995), Moiseenko (1968), Moiseenko and Sokolova (1965, 1968), Moiseenko et al. (1965, 1967), Montanini and Tribuzio (2001), Mossop and Gafner (1951), Mottaghy et al. (2005), Müller (2014), Munck et al. (1979), Murti (1980), Nabawy et al. (2009), Nabawy et al. (2010), Narayanaswamy and Venkatasubramanian (1969), Nehler (2011), Nicolaysen et al. (1981), Noritomi and Asada (1955), Nyblade et al. (1990), Oelsner (1981), Orendt (2014), Pannike et al. (2006), Pannike (2005), Parkhomenko and Bondarenko (1972), Paslick et al. (1995), Peate (1997), Peccerillo and Taylor (1976), Pei (2009), Pereira et al. (1986), Pickett and Saleeby (1993), Plank and Langmuir (1998), Pola et al. (2012, 2014), Popov et al. (1999), Pribnow et al. (2000), Price et al. (1997), Priebs (2011), Rao and Rao (1979, 1983), Rao et al. (1972), Ray et al. (2015), Rebay and Spalla (2001), Reyer and Philipp (2014), Reynaud et al. (1999), Roberts et al. (2000), Rogers et al. (1998), Rolandone et al. (2002), Rosener (2007), Rudnick and Fountain (1995), Rudnick et al. (1998), Rudnick (1992), Rüther (2011), Rummel (1991, 1992), Rummel and Schreiber (1993), Russell et al. (2001), Rybach et al. (2003), Sakvarelidze (1973), Salters et al. (1992), Salton (1999), Sandiford et al. (2002), Sandkühler (2015), Sanner and Anderson (2001), Sanner et al. (2000), Sass et al. (1971), Sawka and Chappell (1988), Schäffer (2012), Schärli and Kohl (2002), Schintgen (2015), Schön (1983, 1996, 1998, 2004), Schöpflin (2013), Schubert (2011), Schumann (2008), Schütz (2013), Schwalb (2012, 2014), Shimojuku et al. (2012), Sighinolfi et al. (1981), Sikora (2015), Sims et al. (1984), Siratovich (2010), Siratovich et al. (2014, 2016), Sizun (1995), Smith and Johnson (1981), Smith et al. (2011), Southern Methodist University (2000), Stober and Bucher (2007), Stober and Jodocy (2009), Sun and McDonough (1989), Sundberg (1988), Surma (2003), Surma and Geraud (2003), Swanberg (1972), Taylor et al. (1983, 1994), Tchameni et al. (2000), Tejada et al. (1996, 2002), Thompson et al. (2001), Trautwein (2005), Ucok (1979), Ufondu (2012), VDI 4640 Blatt 1 (2010), Verdoya et al. (1998), Vernoux and Lambert (1993), Vernoux et al. (1995), Vilà et al. (2010), Villaseca et al. (1999), Violay et al. (2010), Violay et al. (2012a, b), Vogel (2016), Volarovich (1968), Walters and Combs (1991), Wang et al. (2009), Weaver et al. (1987), Weber (2014, 2016), Weinert (2014), Welsch (2011, 2012), Welsch et al. (2015), Wenk and Wenk (1969), Weydt (2016), Whitford (1975), Wicke (2009), Wickham (1987), Wiesner (2014), Winkler (1952), Wittig (2017), Zhao et al. (2016), Zhu et al. (2011, 2016), Zilch (2011) and Zoth (1986).
KB, TR and JB defined the scope of this work; designed and structured the database; and defined the criteria for the quality control. Furthermore, they collected and reviewed most of the referenced literature and supervised the team, who reviewed and collected the literature, transferred the data from literature or own sources into the database, and restructured the database where needed. KB performed the majority of the quality control. KB prepared the manuscript and managed the manuscript during the review process with significant contributions from TR and JB.
The authors declare that they have no conflict of interest.
We thank all contributors and also our external cooperation partners for their support and work to fill the database with valuable data or by providing valuable reports or publications to be included in the compilation. We especially thank Alexander Strom and Philipp Mielke for reviewing and collecting the literature, transferring the data from the literature or own sources into the database, and restructuring the database where needed. Alexander Strom and Philipp Mielke, Jessica Freymark, Peter Wiesner, Rebecca Schmidt, Stina Krombach, Christian Meeßen, Christiane Sikora, Eike Reinosch, Lisa Dieck, Paul Knöll, and Benoît Gibert also collected and reviewed publications for the database; we appreciate their contribution. Ingo Sass contributed to the development of the general concept of such a database in early discussions. We also thank two anonymous reviewers for their helpful comments and suggestions to improve the quality of this paper.
This research has been supported by the European Commission, FP7 Energy (IMAGE (grant no. 608553)).
This paper was edited by Birgit Heim and reviewed by two anonymous referees.
Achtziger-Zupančič, P., Loew, S., and Mariethoz, G.: A new global data base to improve predictions of permeability distribution in crystalline rocks at site scale, J. Geophys. Res.-Sol. Ea., 122, 3513–3539, https://doi.org/10.1002/2017JB014106, 2017.
Adelinet, M., Fortin, J., Schubnel, A., and Guéguen, Y.: Deformation modes in an Icelandic basalt: From brittle failure to localized deformation bands, J. Volcanol. Geoth. Res., 255, 15–25, https://doi.org/10.1016/j.jvolgeores.2013.01.011, 2013.
Alam, M. M., Fabricius, I. L., and Prasad, M.: Permeability prediction in chalks, AAPG Bull., 95, 1991–2014, https://doi.org/10.1306/03011110172, 2011.
Altherr, R., Holl, A., Hegner, E., Langer, C., and Kreuzer, H.: High-potassium, calc-alkaline I-type plutonism in the European Variscides: northern Vosges (France) and northern Schwarzwald (Germany), Lithos, 50, 51–73, https://doi.org/10.1016/S0024-4937(99)00052-3, 2000.
Amante, C. and Eakins, B. W.: ETOPO1 1 Arc-Minute Global Relief Model: Procedures, Data Sources and Analysis, NOAA Technical Memorandum NESDIS NGDC-24, National Geophysical Data Center, NOAA, https://doi.org/10.7289/V5C8276M, 2009.
Aretz, A.: Aufschlussanalogstudie zur geothermischen Reservoircharakterisierung des Permokarbons im nördlichen Oberrheingraben, PhD Thesis, TU Darmstadt, available at: http://nbn-resolving.de/urn:nbn:de:tuda-tuprints-52485 (last access: 14 August 2020), 2015.
Aretz, A., Bär, K., Götz, A. E., and Sass, I.: Outcrop analogue study of Permocarboniferous geothermal sandstone reservoir formations (northern Upper Rhine Graben, Germany): Impact of mineral content, depositional environment and diagenesis on petrophysical properties, Int. J. Earth Sci. (Geol. Rundsch.), 135, 1431–1452, https://doi.org/10.1007/s00531-015-1263-2, 2015.
Ashwal, L. D., Morgan, P., Kelley, S. A., and Percival, J. A.: Heat production in an Archean crustal profile and implications for heat flow and mobilization of heat-producing elements, Earth Planet. Sc. Lett., 85, 439–450, https://doi.org/10.1016/0012-821X(87)90139-7, 1987.
Aswathanarayana, U.: Principles of Nuclear Geology, Balkema Rotterdam, ISBN 90-619-1572-4, available at: https://inis.iaea.org/search/search.aspx?orig_q=RN:19076043 (last access: 14 August 2020), 1986.
Atal, B. S., Bhalla, N. S., Lall, Y., Mahadevan, T. M., and Udas, G. R.: Padioactive Elemental Distribution in the Granjlite Terrains and Dearwar Schist Belis of Peninsular India, in: Archaean Geochemistry, 1, 205–220, edited by: Windley, B. F. and Naqvi, S. M., Elsevier, https://doi.org/10.1016/S0166-2635(08)70099-9, 1978.
Attoh, K.: Contrasting Metamorphic Record of Heat Production Anomalies in the Penokean Orogen of Northern Michigan, J. Geol., 108, 353–361, https://doi.org/10.1086/314406, 2000.
Australian Government – Geoscience Australia: Rock Properties Explorer Version 2.0.5, available at: http://www.ga.gov.au/explorer-web/rock-properties.html, last access: 26 February 2020.
Babaie, H. A., Ghazi, A. M., Babaei, A., Tour, T. E., and Hassanipak, A. A.: Geochemistry of arc volcanic rocks of the Zagros Crush Zone, Neyriz, Iran, J. Asian Earth Sci., 19, 61–76, https://doi.org/10.1016/S1367-9120(00)00012-2, 2001.
Baker, J. A., Menzies, M. A., Thirlwall, M. F., and MacPherson, C. G.: Petrogenesis of Quaternary Intraplate Volcanism, Sana'a, Yemen: Implications for Plume-Lithosphere Interaction and Polybaric Melt Hybridization, J. Petrol., 38, 1359–1390, https://doi.org/10.1093/petroj/38.10.1359, 1997.
Balakrishna, S. and Ramana, Y. V.: Integrated Studies of the Elastic Properties of Some Indian Rocks, in: Geophysical Monograph 12: The Crust and Upper Mantle of the Pacific Area, edited by: Knopoff, L., Drake, C. L., and Heart, P. J., American Geophysical Union, 12, 489–500, https://doi.org/10.1029/GM012p0489, 1968.
Ballard, S., Pollack, H. N., and Skinner, N. J.: Terrestrial heat flow in Botswana and Namibia, J. Geophys. Res.-Sol. Ea., 92, 6291–6300, https://doi.org/10.1029/JB092iB07p06291, 1987.
Bär, K. M.: 3D-Modellierung des tiefengeothermischen Potenzials des nördlichen Oberrheingrabens und Untersuchung der geothermischen Eigenschaften des Rotliegend, Diplomathesis, TU Darmstadt, 2008.
Bär, K. M.: Untersuchung der tiefengeothermischen Potenziale von Hessen, PhD Thesis, TU Darmstadt, available at: http://nbn-resolving.de/urn:nbn:de:tuda-tuprints-30671 (last access: 14 August 2020), 2012.
Bär, K. and Mielke, P.: Stratigraphic classification table (Stratigraphy): P3 - Stratigraphy. V. 1.0. GFZ Data Services. Potsdam. https://doi.org/10.5880/GFZ.4.8.2019.P3.s, 2019.
Bär, K., Reinsch, T., Sippel, S., Strom, A., Mielke, P., and Sass, I.: P3 – International PetroPhysical Property Database, Proceedings, 42nd Workshop on Geothermal Reservoir Engineering, Stanford University, Stanford, California, 13–15 February 2017.
Bär, K., Mielke, P., and Knorz, K.: Petrographic classification table (Petrography): P3 – Petrography, V. 1.0, GFZ Data Services, Potsdam, https://doi.org/10.5880/GFZ.4.8.2019.P3.p, 2019a.
Bär, K., Reinsch, T., and Bott, J.: P3 – PetroPhysical Property Database, V. 1.0, GFZ Data Services, Potsdam, https://doi.org/10.5880/GFZ.4.8.2019.P3, 2019b.
Barrett, D. L. and Aumento, F.: The Mid-Atlantic Ridge near 45 N. XI. Seismic velocity, density, and layering of the crust, Can. J. Earth Sci., 7, 1117–1124, https://doi.org/10.1139/e70-105, 1970.
Bates, R. L. and Jackson, J. A.: Glossary of geology, Third Edition, American Geological Institute, Alexandria, Virginia, USA, 1987.
Bauluz, B., Mayayo, M. J., Fernandez-Nieto, C., and Lopez, J. M.: Geochemistry of Precambrian and Paleozoic siliciclastic rocks from the Iberian Range (NE Spain): implications for source-area weathering, sorting, provenance, and tectonic setting, Chem. Geol., 168, 135–150, https://doi.org/10.1016/S0009-2541(00)00192-3, 2000.
Bea, F. and Montero, P.: Behavior of accessory phases and redistribution of Zr, REE, Y, Th, and U during metamorphism and partial melting of metapelites in the lower crust: an example from the Kinzigite Formation of Ivrea-Verbano, NW Italy, Geochim. Cosmochim. Ac., 63, 1133–1153, https://doi.org/10.1016/S0016-7037(98)00292-0, 1999.
Bemmlott, J.: Untersuchung der geothermischen Gesteinskennwerte von Kulmgrauwacke (Oberharz) und Tanner Grauwacke (Nordhessen), Masterthesis, TU Darmstadt, 2014.
Best, M. G. and Christiansen, E. H.: Igneous Petrology, Blackwell Science, https://doi.org/10.1017/S0016756802216507, 2002.
Betten, I.: 3D-Laserscanning, DFN-Modellierung und Aufschlussaufnahme im Rotliegend des östlichen Saar-Nahe-Beckens, Masterthesis, TU Darmstadt, 2015.
Bhatia, M. R. and Crook, K. A.: Trace element characteristics of graywackes and tectonic setting discrimination of sedimentary basins, Contrib. Mineral. Petr., 92, 181–193, https://doi.org/10.1007/BF00375292, 1986.
Biewer, H.: Bestimmung der Temperaturabhängigkeit der Wärmekapazität und Wärmeleitfähigkeit von Karbonatgesteinen der Schwäbischen und Fränkischen Alb, Bachelorthesis, TU Darmstadt, 2014.
Biewer, H.: Vergleich von Eigenschaften petrothermaler Reservoirgesteine des Gonghe-Beckens und Tromm-Granits/Weschnitz-Plutons, Masterthesis, TU Darmstadt, 2017.
Birch, A. F. and Clark, H.: The thermal conductivity of rocks and its dependence upon temperature and composition, Am. J. Sci., 238, 529–558. https://doi.org/10.2475/ajs.238.8.529, 1940.
Birch, F.: Handbook of physical constants, in: Birch, F., Schairer, J. F., and Spicer, H. C., Geological Society of America, https://doi.org/10.1130/SPE36, 1942.
Blackwell, D. D. and Richards, M. C.: Geothermal Map of North America: American Association of Petroleum Geologists. Tulsa, Oklahoma, scale, 1, available at: https://www.smu.edu/-/media/Site/Dedman/Academics/Programs/Geothermal-Lab/Graphics/Geothermal_MapNA_7x10in.gif?la=en (last access: 14 August 2020), 2004.
Brady, R. J., Ducea, M. N., Kidder, S. B., and Saleeby, J. B.: The distribution of radiogenic heat production as a function of depth in the Sierra Nevada Batholith, California, Lithos, 86, 229–244, https://doi.org/10.1016/j.lithos.2005.06.003, 2006.
Brandt, W., Hanschke, T., and Kaiser, H.: Zum Stand der Nutzung der oberflächennahen Geothermie in Norddeutschland, Proceedings, 8. Geothermische Fachtagung, Landau/Pfalz, Germany, 10–12 November 2004.
Brehme, M., Blöcher, G., Cacace, M., Kamah, Y., Sauter, M., and Zimmermann, G.: Permeability distribution in the Lahendong geothermal field: A blind fault captured by thermal–hydraulic simulation, Environ. Earth Sci., 75, 1088, https://doi.org/10.1007/s12665-016-5878-9, 2016a.
Brehme, M., Deon, F., Haase, C., Wiegand, B., Kamah, Y., Sauter, M., and Regenspurg, S.: Fault controlled geochemical properties in Lahendong geothermal reservoir Indonesia, Grundwasser, 21, 29–41, https://doi.org/10.1007/s00767-015-0313-9, 2016b.
Brettreich, F.: Thermophysical and hydraulic rock properties of greywackes and andesites of the Taupo Volcanic Zone in New Zealand, Masterthesis, TU Darmstadt, 2016.
Bridgman, P. W.: The thermal conductivity and compressibility of several rocks under high pressures, Am. J. Sci., 5–7, 81–102, https://doi.org/10.2475/ajs.s5-7.38.81, 1924.
Brigaud, F., Vasseur, G., and Caillet, G.: Thermal state in the north Viking Graben (North Sea) determined from oil exploration well data, Geophysics, 57, 69–88, https://doi.org/10.1190/1.1443190, 1992.
BritGeothermal: BritGeothermal, available at: http://www.britgeothermal.org/, last access: 26 February 2020.
Brown, G. C., Cassidy, J., Locke, C. A., Plant, J. A., and Simpson, P. R.: Caledonian plutonism in Britain: A summary, J. Geophys. Res.-Sol. Ea., 86, 10502–10514, https://doi.org/10.1029/JB086iB11p10502, 1981.
Bullard, E. C.: Heat Flow in South Africa, P. Roy. Soc. A-Math. Phy., 173, 474–502, https://doi.org/10.1098/rspa.1939.0159, 1939.
Bultitude, R. J., Johnson, R. W., and Chappell, B. W.: Andesites of Bagana volcano, Papua New Guinea: chemical stratigraphy, and a reference andesite composition, BMR J. Aust. Geol. Geophys., 3, 281–295, 1978.
Buntebarth, G.: Geothermie, Springer, Berlin Heidelberg, https://doi.org/10.1007/978-3-662-00910-9, 1980.
Carroll, R. D.: The determination of the acoustic parameters of volcanic rocks from compressional wave velocity measurements, Int. J. Rock Mech. Min., 6, 557–579, https://doi.org/10.1016/0148-9062(69)90022-9, 1969.
Cebriá, J. M., López-Ruiz, J., Doblas, M., Oyarzun, R., Hertogen, J., and Benito, R.: Geochemistry of the Quaternary alkali basalts of Garrotxa (NE Volcanic Province, Spain): a case of double enrichment of the mantle lithosphere, J. Volcanol. Geoth. Res., 102, 217–235, https://doi.org/10.1016/S0377-0273(00)00189-X, 2000.
Cermak, V. and Rybach, L.: Thermal properties, in: Zahlenwerte und Funktionen aus Naturwissenschaft und Technik: Neue Serie [Numerical Data and Functional Relationships in Science and Technology: New Series], c. Landolt-Börnstein – Group V Geophysics, edited by: Angenheister, G., Cermák, V., Hellwege, K.-H., and Landolt, H., 310–314, Springer, Berlin, 1982.
Ceryan, S.: New Chemical Weathering Indices for Estimating the Mechanical Properties of Rocks: A Case Study from the Kürtün Granodiorite, NE Turkey, Turk. J. Earth Sci., 17, 187–207, 2008.
Ceryan, S., Tudes, S., and Ceryan, N.: Influence of weathering on the engineering properties of Harsit granitic rocks (NE Turkey), B. Eng. Geol. Environ., 67, 97–104, https://doi.org/10.1007/s10064-007-0115-0, 2008.
Chamberlin, D. and Boyce, R.: Sequel: a structured English query language, Proceedings of the1974 ACM SIGFIDET(now SIGMOD) Workshop on Data Description, Access and Control, New York, 149–264, 1974.
Chapman, D. S. and Pollack, H. N.: Heat flow and heat production in Zambia: Evidence for lithospheric thinning in central Africa, Tectonophysics, 41, 79–100, https://doi.org/10.1016/0040-1951(77)90181-0, 1977.
Chappell, B. W.: Aluminium saturation in I- and S-type granites and the characterization of fractionated haplogranites, Lithos, 46, 535–551, https://doi.org/10.1016/S0024-4937(98)00086-3, 1999.
Chopra, N., Ray, L., Satyanarayanan, M., and Elangovan, R.: Evaluate best-mixing model for estimating thermal conductivity from mineralogy: A case study for the granitoids of the Bundelkhand craton, central India, Geothermics, 75, 1–14, https://doi.org/10.1016/j.geothermics.2018.03.011, 2018.
Chung, S.-L.: Trace Element and Isotope Characteristics of Cenozoic Basalts around the Tanlu Fault with Implications for the Eastern Plate Boundary between North and South China, J. Geol., 107, 301–312, https://doi.org/10.1086/314348, 1999.
Chung, S.-L., Jahn, B.-M., Chen, S.-J., Lee, T., and Chen, C.-H.: Miocene basalts in northwestern Taiwan: Evidence for EM-type mantle sources in the continental lithosphere, Geochim. Cosmochim. Ac., 59, 549–555, https://doi.org/10.1016/0016-7037(94)00360-X, 1995.
Chung, S.-L., Wang, K.-L., Crawford, A. J., Kamenetsky, V. S., Chen, C.-H., Lan, C.-Y., and Chen, C.-H.: High-Mg potassic rocks from Taiwan: implications for the genesis of orogenic potassic lavas, Lithos, 59, 153–170, https://doi.org/10.1016/S0024-4937(01)00067-6, 2001.
Clark, S. P.: Heat flow at Grass Valley, California, Transactions, American Geophysical Union, 38, 239, https://doi.org/10.1029/tr038i002p00239, 1957.
Clark, S. P.: Heat Flow in the Austrian Alps, Geophys. J. Int., 6, 54–63, https://doi.org/10.1111/j.1365-246X.1961.tb02961.x, 1961.
Clark, S. P.: Handbook of physical constants (Bd. 97), Geol. Soc. Am., https://doi.org/10.1130/MEM97, 1966.
Clauser, C.: Strukturelle, Advektive und Paläoklimatische Einflüsse auf den Wärmetransport in der Kristallinen Oberkruste am Beispiel der Kola-Halbinsel. DFG Projekt-Nr.: Cl 121/4–2; Zwischenbericht für den Zeitraum 1. 1. 1998–31. 12. 2001, available at: https://www.eonerc.rwth-aachen.de/go/id/dmwf/file/48298 (last access: 14 August 2020), 2001.
Clauser, C. and Huenges, E.: Thermal Conductivity of Rocks and Minerals, American Geophysical Union, 105 pp., https://doi.org/10.1029/RF003p0105, 1995a.
Clauser, C. and Huenges, E.: Thermal Conductivity of Rocks and Minerals – Rock Physics and Phase Relations, A Handbook of Physical Constants, AGU Reference Shelf, 3, 105–126, American Geophysical Union, Washington, 1995b.
Cocherie, A.: Interaction manteau-croûte: son rôle dans la genèse d'associations plutoniques calo-alcalines, contraintes géochimiques (éléments en traces et isotopes du strontium et de l'oxygène), PhD dissertation, CNRS-BRGM, 1984.
Cocherie, A., Rossi, P., Fouillac, A. M., and Vidal, P.: Crust and mantle contributions to granite genesis - An example from the Variscan batholith of Corsica, France, studied by trace-element and Nd-isotope systematics, Chemical Geology, 115, 173–211, https://doi.org/10.1016/0009-2541(94)90186-4, 1994.
Codd, E. F.: A Relational Model of Data for Large Shared Data Banks, Communications of the ACM, 13, 377–387, https://doi.org/10.1145/362384.362685, 1970.
Cohen, K. M., Finney, S. C., Gibbard, P. L., and Fan, J.-X.: The ICS International Chronostratigraphic Chart, Episodes, 36, 199–204, 2013.
Condie, K. C.: Chemical composition and evolution of the upper continental crust: Contrasting results from surface samples and shales, Chem. Geol., 104, 1–37, https://doi.org/10.1016/0009-2541(93)90140-E, 1993.
Coster, H. P.: The Electrical Conductivity of Rocks at High Temperatures, Geophysical Supplements to the Monthly Notices of the Royal Astronomical Society, 5, 193–199, https://doi.org/10.1111/j.1365-246X.1948.tb02935.x, 1948.
Côté, J. and Konrad, J.-M.: Thermal conductivity of base-course materials, Can. Geotech. J., 42, 61–78, https://doi.org/10.1139/t04-081, 2005.
Crecraft, H. R., Nash, W. P., and Evans, S. H.: Late Cenozoic volcanism at Twin Peaks, Utah: Geology and petrology, J. Geophys. Res.-Sol. Ea., 86, 10303–10320. https://doi.org/10.1029/JB086iB11p10303, 1981.
Creutzburg, H.: Bestimmung thermischer Stoffwerte von Salzgesteinen und Nebengesteinen, Kali und Steinsalz, 5, 170–172, 1965.
CrowdFlower: Data Scientist Report, available at: https://visit.figure-eight.com/rs/416-ZBE-142/images/CrowdFlower_DataScienceReport_2016.pdf (last access: 26 February 2020), 2016.
Dahmani, A. and Sawyer, E. W.: Variabilité géochimique des métapélites dans l'auréole métamorphique d'Oulmès (Maroc central), Bulletin de l'Institut scientifique, section Sciences de la Terre, 2001, 7–20, 2001.
Damm, K.-W., Pichowiak, S., Harmon, R. S., Todt, W., Kelley, S., Omarini, R., and Niemeyer, H.: Pre-Mesozoic evolution of the central Andes The basement revisited, Geol. S. Am. S., 241, 101–126, https://doi.org/10.1130/SPE241-p101, 1990.
Descamps, F., Tshibangu, J.-P., da Silva, N., and Regnard, S.: A Database to Manage Rock Mechanical Properties and Assess Formation Abrasiveness for Drilling, Conference paper: International Symposium of the International Society for Rock Mechanics, Eurock 2013, Wroclaw, Poland, https://doi.org/10.1201/b15683-39, 2013.
Devaraju, A., Klump, J., Cox, S. J. D., and Golodoniuc, P.: Representing and publishing physical sample descriptions, Comput. Geosci., 96, 1–10, https://doi.org/10.1016/j.cageo.2016.07.018, 2016.
Dickson, B. L. and Scott, K. M.: Interpretation of aerial gamma-ray surveys-adding the geochemical factors, Geoscience Australia, 17, 187–200, 1997.
Diment, W. H.: Thermal conductivity of serpentinite from Mayaguez, Puerto Rico, and other localities. National Academy of Sciences, National Research Council Publication, 1188, 92–106, 1964.
Dodge, F. C., Calk, L. C., and Kistler, R. W.: Lower Crustal Xenoliths, Chinese Peak Lava Flow, Central Sierra Nevada, J. Petrol., 27, 1277–1304, https://doi.org/10.1093/petrology/27.6.1277, 1986.
Dongmo, C. L.: Outcrop Analogue Study of Geothermal Properties of the Southesk-Cairn Carbonate Complex in the Alberta Basin, Canada, Masterthesis, TU Darmstadt, 2016.
Ducea, M. N. and Saleeby, J. B.: The age and origin of a thick mafic – ultramafic keel from beneath the Sierra Nevada batholith, Contrib. Mineral. Petr., 133, 169–185, https://doi.org/10.1007/s004100050445, 1998.
Dupré, B. and Echeverría, L. M.: Pb isotopes of Gorgona Island (Colombia): isotopic variations correlated with magma type, Earth Planet. Sc. Lett., 67, 186–190, https://doi.org/10.1016/0012-821X(84)90113-4, 1984.
Eberhard, M.: Erdwärmesondenfeld Aarau. Heizen und Kühlen eines grossen Bürogebäudes mit teilweise wärmeisolierten Erdwärmesonden, Bundesamt für Energie, Schlussbericht Oktober, 1–18, 2005.
El Dakak, W.: Influence of Temporal Variation of Water Saturation on Shallow Geothermal Systems Using Numerical Modeling, Masterthesis, TU Darmstadt, 2015.
Enge, H. D., Buckley, S. J., Rotevatn, A., and Howell, J. A.: From outcrop to reservoir simulation model: workflow and procedures, Geosphere, 3, 469–490, https://doi.org/10.1130/GES00099.1, 2007.
Ensor, C. R.: The determination of thermal conductivity and its temperature-variation for medium conductors, P. Phys. Soc., 43, 581–591, https://doi.org/10.1088/0959-5309/43/5/314, 1931.
Esteban, L., Pimienta, L., Sarout, J., Piane, C. D., Haffen, S., Geraud, Y., and Timms, N. E.: Study cases of thermal conductivity prediction from P-wave velocity and porosity, Geothermics, 53, 255–269, https://doi.org/10.1016/j.geothermics.2014.06.003, 2015.
Ewart, A., Bryan, W. B., and Gill, J. B.: Mineralogy and Geochemistry of the Younger Volcanic Islands of Tonga, S.W. Pacific, J. Petrol., 14, 429–465, https://doi.org/10.1093/petrology/14.3.429, 1973.
Ewart, A., Brothers, R. N., and Mateen, A.: An outline of the geology and geochemistry, and the possible petrogenetic evolution of the volcanic rocks of the Tonga-Kermadec-New Zealand island arc, J. Volcanol. Geoth. Res., 2, 205–250, https://doi.org/10.1016/0377-0273(77)90001-4, 1977.
Fachinformationssystem (FIS) Geophysik: Geophysics Information System, available at: http://www.fis-geophysik.de, last access: 19 October 2019.
Faridfar, N.: Untersuchung und Bewertung der geothermischen Eigenschaften der Gesteine der Noerdlichen Pyllitzone, Vordertaunus, Bachelorthesis, TU Darmstadt, Goethe Universität Frankfurt am Main, 2010.
Farmer, G. L.: Continental Basaltic Rocks, in: Treatise on Geochemistry, edited by: Holland, H. D. and Turekian, K. K., Elsevier-Pergamon, 85–121 https://doi.org/10.1016/B0-08-043751-6/03019-x, 2003.
Farmer, G. L., Glazner, A. F., and Manley, C. R.: Did lithospheric delamination trigger late Cenozoic potassic volcanism in the southern Sierra Nevada, California?, Geol. Soc. Am. B., 114, 754–768, https://doi.org/10.1130/0016-7606(2002)114<0754:DLDTLC>2.0.CO;2, 2002.
Farquharson, J. I., Heap, M. J., and Baud, P.: Strain-induced permeability increase in volcanic rock, Geophys. Res. Lett., 43, 11603–11610, https://doi.org/10.1002/2016GL071540, 2016.
Farquharson, J. I., Baud, P., and Heap, M. J.: Inelastic compaction and permeability evolution in volcanic rock, Solid Earth, 8, 561–581, https://doi.org/10.5194/se-8-561-2017, 2017.
Fisher, R. V. and Smith, G. A.: Volcanism, tectonics and sedimentation, 1-5. Sedimentation in volcanic settings, Society for Sedimentary Geology, Special Publication No. 45, https://doi.org/10.2110/pec.91.45, 1991.
Förster, A. and Förster, H.-J.: Crustal composition and mantle heat flow: Implications from surface heat flow and radiogenic heat production in the Variscan Erzgebirge (Germany), J. Geophys. Res.-Sol. Ea., 105, 27917–27938. https://doi.org/10.1029/2000JB900279, 2000.
Fountain, D. M., Salisbury, M. H., and Furlong, K. P.: Heat production and thermal conductivity of rocks from the Pikwitonei–Sachigo continental cross section, central Manitoba: implications for the thermal structure of Archean crust, Can. J. Earth Sci., 24, 1583–1594, https://doi.org/10.1139/e87-154, 1987.
Fourcade, S. and Allegre, C. J.: Trace element behaviour in granite genesis. The calc-alkaline plutonic association from the Querigut Complex (Pyrenees, France), Contrib. Mineral. Petr., 76, 177–195, https://doi.org/10.1007/BF00371958, 1981.
Fowler, C. M., Stead, D., Pandit, B. I., Janser, B. W., Nisbet, E. G., and Nover, G.: A database of physical properties of rocks from the Trans-Hudson Orogen, Canada, Can. J. Earth Sci., 42, 555–572, https://doi.org/10.1139/e05-006, 2005.
Francois, D. and Lemmet, M.: Evolution of Mg/Fe Ratios in Late Variscan Plutonic Rocks from the External Crystalline Massifs of the Alps (France, Italy, Switzerland), J. Petrol., 40, 1151–1185, https://doi.org/10.1093/petroj/40.7.1151, 1999.
Franzson, H., Guðfinnsson, G. H., Helgadóttir, H. M., and Frolova, J.: Porosity, density and chemical composition relationships in altered Icelandic hyaloclastites, in: Water-Rock Interaction, edited by: Birkle and Torres-Alvarado, 199–202, CRC Press, London, ISBN 978-0-415-60426-0, 2010.
Franzson, H., Gudfinnsson, G. H., Frolova, J., Helgadóttir, H. M., Pauly, B., Mortensen, A. K., and Jakobsson, S. P.: Icelandic Hyaloclastite Tuffs – Petrophysical properties, alteration and geochemical mobility, Tech. rep., ISOR – Icelandic Geosurvey, Reykjavik, Iceland, 2011.
Fridleifson, G. O. and Vilmundardottir, E. G.: Reservoir Parameters TCP-Project A Thin-section Study of the Öskjuhlid Samples. Progress Report, ORKUSTOFNUN National Energy Authority Project No. 720 105, 1998.
Fuchs, S., Balling, N., and Förster, A.: Calculation of thermal conductivity, thermal diffusivity and specific heat capacity of sedimentary rocks using petrophyscial well logs, Geophys. J. Int., 203, 1977–2000, 2015.
Fuji-ta, K., Katsura, T., and Tainosho, Y.: Electrical conductivity measurement of granulite under mid- to lower crustal pressure-temperature conditions, Geophys. J. Int., 157, 79–86, https://doi.org/10.1111/j.1365-246X.2004.02165.x, 2004.
Fuji-ta, K., Katsura, T., Matsuzaki, T., Ichiki, M., and Kobayashi, T.: Electrical conductivity measurement of gneiss under mid- to lower crustal P–T conditions, Tectonophysics, 434, 93–101, https://doi.org/10.1016/j.tecto.2007.02.004, 2007.
Fuji-ta, K., Katsura, T., Ichiki, M., Matsuzaki, T., and Kobayashi, T.: Variations in electrical conductivity of rocks above metamorphic conditions, Tectonophysics, 504, 116–121, https://doi.org/10.1016/j.tecto.2011.03.008, 2011.
Galán, G., Pin, C., and Duthou, J.-L.: Sr-Nd isotopic record of multi-stage interactions between mantle-derived magmas and crustal components in a collision context – The ultramafic-granitoid association from Vivero (Hercynian belt, NW Spain), Chem. Geol., 131, 67–91, https://doi.org/10.1016/0009-2541(96)00027-7, 1996.
Gangadharam, E. V. and Aswathanarayana, U.: Trace elements in the kimberlites of Andhra Pradesh, India, Trans. Amer. Geophy., 50, 341, 1969.
Gao, S., Luo, T.-C., Zhang, B.-R., Zhang, H.-F., Han, Y.-w., Zhao, Z.-D., and Hu, Y.-K.: Chemical composition of the continental crust as revealed by studies in East China, Geochim. Cosmochim. Ac., 62, 1959–1975, https://doi.org/10.1016/S0016-7037(98)00121-5, 1998.
Garcia-Gutierrez, A. and Contreras, E.: Measurement of Thermal Conductivity and Diffusivity of Drill Core Samples from the Los Humeros Geothermal Field, Mexico, by a Line-Source Technique, GRC Transactions, Davis, California, 31, available at: https://www.geothermal-library.org/index.php?mode=pubs&action=view&record=1025278 (last access: 14 August 2020), 2007.
Gard, M., Hasterok, D., and Halpin, J. A.: Global whole-rock geochemical database compilation, Earth Syst. Sci. Data, 11, 1553–1566, https://doi.org/10.5194/essd-11-1553-2019, 2019.
Gärtner, D.: Comparison of geothermal properties of the carbonatic aquifer systems in the Western Canada Sedimentary Basin and the South German Molasse Basin, Masterthesis, TU Darmstadt, 2017.
Gaunt, H. E., Sammonds, P. R., and Meredith, R. P.: Pathways for degassing during the lava dome eruption of Mount St. Helens 2004–2008, Geology, 42, 947–950, https://doi.org/10.1130/G35940.1, 2014.
Gegenhuber, N. and Schoen, J.: New approaches for the relationship between compressional wave velocity and thermal conductivity, J. Appl. Geophys., 76, 50–55, 2012.
Gehlin, S.: Thermal response test method development and evaluation, Luleå University of Technology, Luleå tekniska universitet, available at: http://urn.kb.se/resolve?urn=urn:nbn:se:ltu:diva-18433 (last access: 14 August 2020), 2002.
Gehlin, S. E. and Hellstrom, G.: Comparison of Four Models for Thermal Response Test Evaluation, ASHRAE Transactions-American Society of Heating Refrigerating Airconditioning Engin, 109, 131–142, 2003.
Geist, O.: Geothermische und gesteinsmechanische Untersuchung an ausgewaehlten Standorten der Fraenkischen Alb. Bachelorthesis, TU Darmstadt, 2011.
GeORG-Projektteam: Geopotenziale des tieferen Untergrundes im Oberrheingraben, Fachlich-Technischer Abschlussbericht des INTERREG-Projekts GeORG, Teil 1, Landesamt für Geologie, Rohstoffe und Bergbau, available at: http://www.geopotenziale.org/products/fta?lang=1 (last access: 14 August 2020), 2013.
Ghazi, A. M. and Hassanipak, A. A.: Geochemistry of subalkaline and alkaline extrusives from the Kermanshah ophiolite, Zagros Suture Zone, Western Iran: implications for Tethyan plate tectonics, J. Asian Earth Sci., 17, 319–332, https://doi.org/10.1016/S0743-9547(98)00070-1, 1999.
Gill, J.: Orogenic Andesites and Plate Tectonics (Bd. 16), Springer, Berlin Heidelberg, https://doi.org/10.1007/978-3-642-68012-0, 1981.
Gill, J. and Whelan, P.: Early rifting of an oceanic island arc (Fiji) produced shoshonitic to tholeiitic basalts, J. Geophys. Res.-Sol. Ea., 94, 4561–4578, https://doi.org/10.1029/JB094iB04p04561, 1989.
Gillespie, M. R. and Styles, M. T.: Classification of igneous rocks, BGS Rock Classification Scheme, Volume 1, British Geological Survey, Research Report Number RR 99-06, Nottingham, UK, 1999.
Glover, P. W.: Electrical conductivity of rock samples subjected to high temperatures and pressures. Ph.D. dissertation, University of East Anglia, available at: http://ethos.bl.uk/OrderDetails.do?uin=uk.bl.ethos.236438 (last access: 14 August 2020), 1989.
Glover, P. W. and Vine, F. J.: Electrical conductivity of carbonbearing granulite at raised temperatures and pressures, Nature, 360, 723–726, https://doi.org/10.1038/360723a0, 1992.
Glover, P. W. and Vine, F. J.: Electrical conductivity of the continental crust, Geophys. Res. Lett., 21, 2357–2360, https://doi.org/10.1029/94GL01015, 1994.
Glover, P. W. and Vine, F. J.: Beyond KTB – electrical conductivity of the deep continental crust, Surv. Geophys., 16, 5–36, https://doi.org/10.1007/BF00682710, 1995.
Grecksch, G., Ortiz, A., and Schellschmidt, R.: HDR project Soultz – thermophysical study of GPK2 and GPK3 granite samples, ZIP Vorhaben “Hot-Dry-Rock-Project Soultz – Hydrogeothermische Modellierung des HDR-Wärmetauschers” (Förderkennzeichen: 0327109B), “Hot Dry Rock Energy” (EC contract ENK5-CT-2000-00301), 2003.
Grunert, S.: Der Elbsandstein: Vorkommen, Verwendung, Eigenschaften Elbe Sandstone: deposits, use, properties, Geologica Saxonia, 52/53, 3–22, 2007.
Gu, Y.: Calculations of the optimized design for an existing multifunctional building with borehole heat exchangers, Masterthesis, TU Darmstadt, 2010.
Gu, Y., Rühaak, W., Bär, K., and Sass, I.: Using seismic data to estimate the spatial distribution of rock thermal conductivity at reservoir scale, Geothermics, 66, 61–72, https://doi.org/10.1016/j.geothermics.2016.11.007, 2017.
Gudmundsson, A., Franzson, H., and Fridleifson, G. O.: ForOafrceOistuOlar Sofnun syna AfangaskYrsla urn sarnvinnuverk Hitaveitu Reykjavfkur, Hitaveitu SuOurnesja og Orkustofnunar, Tech. rep., ORKUSTOFNUN, Reykjavik, Iceland, 1995.
Gühne, S.: Petrographie und Petrophysik des Rotliegend der Forschungsbohrung Messel GA2, Bachelorthesis, TU Darmstadt, 2016.
Guillot, S. and Fort, P. L.: Geochemical constraints on the bimodal origin of High Himalayan leucogranites, Lithos, 35, 221–234, https://doi.org/10.1016/0024-4937(94)00052-4, 1995.
Guillou-Frottier, L., Mareschal, J.-C., Jaupart, C., Gariépy, C., Lapointe, R., and Bienfait, G.: Heat flow variations in the Grenville Province, Canada, Earth Planet. Sc. Lett., 136, 447–460, https://doi.org/10.1016/0012-821X(95)00187-H, 1995.
Gupta, M. L., Sundar, A., and Sharma, S. R.: Heat flow and heat generation in the Archaean Dharwar cratons and implications for the Southern Indian Shield geotherm and lithospheric thickness, Tectonophysics, 194, 107–122, https://doi.org/10.1016/0040-1951(91)90275-W, 1991.
Haack, U.: On the content and vertical distribution of K, Th and U in the continental crust, Earth Planet. Sc. Lett., 62, 360–366, https://doi.org/10.1016/0012-821X(83)90006-7, 1983.
Haffen, S., Géraud, Y., Diraison, M., and Dezayes, C.: Determining majors fluid-flow zones in a geothermal sandstone reservoir from thermal conductivity and temperature logs, EGU General Assembly Conference Abstracts, 14, 9043, 2012.
Haffen, S., Geraud, Y., Diraison, M., and Dezayes, C.: Determination of fluid-flow zones in a geothermal sandstone reservoir using thermal conductivity and temperature logs, Geothermics, 46, 32–41, https://doi.org/10.1016/j.geothermics.2012.11.001, 2013.
Hallsworth, C. R. and Knox, R. W. O'B.: Classification of sediments and sedimentary rocks, BGS Rock Classification Scheme, Volume 3, British Geological Survey, Research Report Number RR 99-03, Nottingham, UK, 1999.
Hantschel, A. I. and Kauerauf, T.: Fundamentals of Basin and Petroleum Systems Modeling, 476 pp., Springer Verlag, Berlin Heidelberg, ISBN 978-3-5-540-72318-9_1, 2009.
Hartmann, A., Rath, V., and Clauser, C.: Thermal conductivity from core and well log data, Int. J. Rock Mech. Min., 42, 1042–1055, https://doi.org/10.1016/j.ijrmms.2005.05.015, 2005.
Hasterok, D. and Webb, J.: On the radiogenic heat production of igneous rocks, Geosci. Front., 8, 919–940, https://doi.org/10.1016/j.gsf.2017.03.006, 2017.
Hasterok, D., Gard, M., and Webb, J.: On the radiogenic heat production of metamorphic, igneous, and sedimentary rocks, Geosci. Front., 9, 1777–1794, https://doi.org/10.1016/j.gsf.2017.10.012, 2018.
Hauff, F., Hoernle, K., van den Bogaard, P., Alvarado, G., and Garbe-Schönberg, D.: Age and geochemistry of basaltic complexes in western Costa Rica: Contributions to the geotectonic evolution of Central America, Geochem. Geophys. Geosyst., 1, 1009, https://doi.org/10.1029/1999GC000020, 2000.
Heap, M. J., Vinciguerra, S., and Meredith, P. G.: The evolution of elastic moduli with increasing crack damage during cyclic stressing of a basalt from Mt. Etna volcano, Tectonophysics, 471, 153–160, https://doi.org/10.1016/j.tecto.2008.10.004, 2009.
Heap, M. J., Faulkner, D. R., Meredith, P. G., and Vinciguerra, S.: Elastic moduli evolution and accompanying stress changes with increasing crack damage: implications for stress changes around fault zones and volcanoes during deformation, Geophys. J. Int., 183, 225–236, https://doi.org/10.1111/j.1365-246X.2010.04726.x, 2010.
Heap, M. J., Baud, P., Meredith, P. G., Vinciguerra, S., Bell, A. F., and Main, I. G.: Brittle creep in basalt and its application to time-dependent volcano deformation, Earth Planet. Sc. Lett., 307, 71–82, https://doi.org/10.1016/j.epsl.2011.04.035, 2011.
Heap, M. J., Lavallee, Y., Laumann, A., Hess, K.-U., Meredith, P. G., and Dingwell, D. B.: How tough is tuff in the event of fire?, Geology, 40, 311–314, https://doi.org/10.1130/G32940.1, 2012.
Heap, M. J., Baud, P., Meredith, P. G., Vinciguerra, S., and Reuschlé, T.: The permeability and elastic moduli of tuff from Campi Flegrei, Italy: implications for ground deformation modelling, Solid Earth, 5, 25–44, https://doi.org/10.5194/se-5-25-2014, 2014a.
Heap, M. J., Lavallée, Y., Petrakova, L., Baud, P., Reuschlé, T., Varley, N. R., and Dingwell, D. B.: Microstructural controls on the physical and mechanical properties of edifice-forming andesites at Volcán de Colima, Mexico, J. Geophys. Res.-Sol. Ea., 119, 2925–2963, https://doi.org/10.1002/2013JB010521, 2014b.
Heap, M. J., Farquharson, B. P., Lavallee, Y., and Reuschle, T.: Fracture and compaction of andesite in a volcanic edifice, Bull. Volcanol., 77, 55, https://doi.org/10.1007/s00445-015-0938-7, 2015a.
Heap, M. J., Farquharson, J. I., Wadsworth, F. B., Kolzenburg, S. and Russell, J. K.: Timescales for permeability reduction and strength recovery in densifying magma, Earth Planet. Sc. Lett., 429, 223–233, https://doi.org/10.1016/j.epsl.2015.07.053, 2015b.
Heap, M. J., Kennedy, B. M., Pernin, N., Jacquemard, L., Baud, P., Farquharson, J. I., and Dingwell, D. B.: Mechanical behaviour and failure modes in the Whakaari (White Island volcano) hydrothermal system, New Zealand, J. Volcanol. Geoth. Res., 295, 26–42, https://doi.org/10.1016/j.jvolgeores.2015.02.012, 2015c.
Heap, M. J., Russell, J. K., and Kennedy, L. A.: Mechanical behaviour of dacite from Mount St. Helens (USA): A link between porosity and lava dome extrusion mechanism (dome or spine)?, J. Volcanol. Geoth. Res., 328, 159–177, https://doi.org/10.1016/j.jvolgeores.2016.10.015, 2016.
Herrin, E. and Clark, S. P.: Heat flow in west Texas and eastern New Mexico, Geophysics, 21, 1087–1099, https://doi.org/10.1190/1.1438306, 1956.
Hesse, J.: Untersuchung des geothermischen Potenzials der effusiven Vulkanite der permischen Donnersberg-Formation, Bachelorthesis, TU Darmstadt, 2011.
Hill, M., Morris, J., and Whelan, J.: Hybrid granodiorites intruding the accretionary prism, Kodiak, Shumagin, and Sanak Islands, southwest Alaska, J. Geophys. Res.-Sol. Ea., 86, 10569–10590, https://doi.org/10.1029/JB086iB11p10569, 1981.
Hoffmann, H.: Bestimmung von geothermischen Kennwerten an Gesteinen des Kellerwaldes, Bachelorthesis, TU Darmstadt, Goethe Universität Frankfurt am Main, 2011.
Hoffmann, H.: Petrophysikalische Eigenschaften der Mitteldeutschen Kristallinschwelle im Bereich des Oberrheingrabens, Masterthesis, TU Darmstadt, 2015.
Hofmann, A. W.: Chemical differentiation of the Earth: the relationship between mantle, continental crust, and oceanic crust, Earth Planet. Sc. Lett., 90, 297–314, https://doi.org/10.1016/0012-821X(88)90132-X, 1988.
Homuth, S.: Aufschlussanalogstudie zur Charakterisierung oberjurassischer geothermischer Karbonatreservoire im Molassebecken, PhD dissertation, TU Darmstadt, available at: http://nbn-resolving.de/urn:nbn:de:tuda-tuprints-42094 (last access: 14 August 2020), 2014.
Hooper, P. R. and Hawkesworth, C. J.: Isotopic and Geochemical Constraints on the Origin and Evolution of the Columbia River Basalt, J. Petrol., 34, 1203–1246, https://doi.org/10.1093/petrology/34.6.1203, 1993.
Howell, J. A., Allard, W. M., and Good, T. R.: The application of outcrop analogues in geological modeling: a review, present status and future outlook, Geol. Soc. Lond. Spec. Publ., 387, 1–25, 2014.
Huber, A., Good, J., Widmer, P., Nussbaumer, T., Trüssel, D., and Schmid, C.: Gekoppelte Kälte-und Wärmeerzeugung mit Erdwärmesonden-Handbuch zum Planungsvorgehen, Final report Forschungsprogramm Umgebungs- und Abwärme, Wärme-Kraft-Kopplung (UAW), Bundesamt für Energie, Zürich, Schweiz, 64 p., available at: http://www.hetag.ch/download/gekoppelte_ews.pdf (last access: 14 August 2020), 2001.
Hückel, B. and Kappelmeyer, O.: Geothermische Untersuchungen im Saarkarbon, Zeitschrift der Deutschen Geologischen Gesellschaft, 117, 280–311, 1966.
Hurtig, E. and Brugger, H.: Wärmeleitfähigkeitsmessungen unter einaxialem Druck, Tectonophysics, 10, 67–77, https://doi.org/10.1016/0040-1951(70)90098-3, 1970.
Hutt, J. R. and Berg, J. W.: Thermal and electrical conductivities of sandstone rocks and ocean sediments, Geophysics, 33, 489–500, https://doi.org/10.1190/1.1439946, 1968.
Hyndman, R. D. and Jessop, A. M.: The Mid-Atlantic Ridge Near 45 N. XV. Thermal Conductivity of Dredge and Drill Core Samples, Can. J. Earth Sci., 8, 391–393, https://doi.org/10.1139/e71-040, 1971.
Inger, S. I. and Harris, N. I.: Geochemical Constraints on Leucogranite Magmatism in the Langtang Valley, Nepal Himalaya, J. Petrol., 34, 345–368, https://doi.org/10.1093/petrology/34.2.345, 1993.
Innocent, C., Briqueu, L., and Cabanis, B.: Sr-Nd isotope and trace-element geochemistry of late Variscan volcanism in the Pyrenees: Magmatism in post-orogenic extension?, Tectonophysics, 238, 161–181, https://doi.org/10.1016/0040-1951(94)90054-X, 1994.
IRETHERM Project: IREland's geoTHERMal potential, available at: http://www.iretherm.ie/, last access: 26 February 2020.
Islam, M. A.: Petrophysical evaluation of subsurface reservoir sandstones of Bengal Basin, Bangladesh, J. Geol. Soc. India, 76, 621–631, https://doi.org/10.1007/s12594-010-0122-9, 2010.
Iyer, S. S., Choudhuri, A., Vasconcellos, M. B., and Cordani, U. G.: Radioactive element distribution in the Archean granulite terrane of Jequié – Bahia, Brazil. Contrib. Mineral. Petr., 85, 95–101, https://doi.org/10.1007/BF00380226, 1984.
Jahn, F., Graham, M., and Cook, M.: Hydrocarbon Exploration & Production, vol. 55, Second Edition (Developments in Petroleum Science), Elsevier Science, 470 pp., 2008.
Jaupart, C., Mann, J. R., and Simmons, G.: A detailed study of the distribution of heat flow and radioactivity in New Hampshire (U.S.A.), Earth Planet. Sc. Lett., 59, 267–287, https://doi.org/10.1016/0012-821X(82)90131-5, 1982.
Jaya, M. S., Shapiro, S. A., Kristinsdóttir, L. H., Bruhn, D., Milsch, H., and Spangenberg, E.: Temperature dependence of seismic properties in geothermal rocks at reservoir conditions, Geothermics, 39, 115–123, https://doi.org/10.1016/j.geothermics.2009.12.002, 2010.
Jensen, B.: Geothermische Untersuchung des Hauptrogenstein im suedlichen Oberrheingraben. Bachelorsthesis, TU Darmstadt, 2014.
Jeong, H.-S., Kang, S.-S., and Obara, Y.: Influence of surrounding environments and strain rates on the strength of rocks subjected to uniaxial compression, Int. J. Rock Mech. Min., 44, 321–331, https://doi.org/10.1016/j.ijrmms.2006.07.009, 2007.
Jochum, K. P., Hofmann, A. W., Ito, E., Seufert, H. M., and White, W. M.: K, U and Th in mid-ocean ridge basalt glasses and heat production, K/U and K/Rb in the mantle, Nature, 306, 431–436, https://doi.org/10.1038/306431a0, 1983.
Jodocy, M. and Stober, I.: Porositäten und Permeabilitäten im Oberrheingraben und Südwestdeutschen Molassebecken, Erdöl, Erdgas, Kohle, 127, 20–27, 2011.
Johannes, W., Ehlers, C., Kriegsman, L. M., and Mengel, K.: The link between migmatites and S-type granites in the Turku area, southern Finland, Lithos, 68, 69–90, https://doi.org/10.1016/S0024-4937(03)00032-X, 2003.
John, B. E. and Wooden, J.: Chapter 5: Petrology and geochemistry of the metaluminous to peraluminous Chemehuevi Mountains Plutonic Suite, southeastern California, Geol. Soc. Am. Mem., 174, 71–98, https://doi.org/10.1130/MEM174-p71, 1990.
Jones, M. Q.: Heat flow and heat production in the Namaqua Mobile Belt, South Africa, J. Geophys. Res.-Sol. Ea., 92, 6273, https://doi.org/10.1029/JB092iB07p06273, 1987.
Jones, M. Q.: Heat flow in the Witwatersrand Basin and environs and its significance for the South African Shield Geotherm and lithosphere thickness, J. Geophys. Res.-Sol. Ea., 93, 3243, https://doi.org/10.1029/JB093iB04p03243, 1988.
Kahraman, S.: The correlations between the saturated and dry P-wave velocity of rocks, Ultrasonics, 46, 341–348, https://doi.org/10.1016/j.ultras.2007.05.003, 2007.
Kalsbeek, F., Jepsen, H. F., and Jones, K. A.: Geochemistry and petrogenesis of S-type granites in the East Greenland Caledonides, Lithos, 57, 91–109, https://doi.org/10.1016/S0024-4937(01)00038-X, 2001.
Kappelmeyer, O. and Haenel, R.: Geothermics with special reference to application, edited by: Rosenbach, O. and Morelli, C., Gebrüder Bornträger, Stuttgart, 1974.
Kassab, M. A. and Weller, A.: Porosity estimation from compressional wave velocity: A study based on Egyptian sandstone formations, J. Petr. Sci. Eng., 78, 310–315, https://doi.org/10.1016/j.petrol.2011.06.011, 2011.
Kassab, A. and Weller, A.: Study on P-wave and S-wave velocity in dry and wet sandstones of Tushka region, Egypt, Egyptian Journal of Petroleum, 24, 1–11, https://doi.org/10.1016/j.ejpe.2015.02.001, 2015.
Kawada, K.: Studies of the Thermal State of the Earth. The 15th Paper: Variation of Thermal Conductivity of Rocks. Part 1, Bulletin of the Earthquake Research Institute, 42, 631–647, 1964.
Kawada, K.: Studies of the Thermal State of the Earth. The 17th Paper: Variation of Thermal Conductivity of Rocks. Part 2, Bulletin of the Earthquake Research Institute, 44, 1071–1091, 1966.
Kay, S. M. and Kay, R. W.: Aleutian magmas in space and time, in: The Geology of Alaska, edited by: Plafker, G. and Berg, H. C., Geol. Soc. Am., 687–717, https://doi.org/10.1130/dnag-gna-g1.687, 1994.
Kay, S. M., Kay, R. W., Citron, G. P., and Perfit, M. R.: Calc-alkaline plutonism in the intra-oceanic Aleutian arc, Alaska, Geol. S. Am. S., 241, 233–256, https://doi.org/10.1130/SPE241-p233, 1990.
Keen, C. E. and Lewis, T.: Measured Radiogenic Heat Production in Sediments from Continental Margin of Eastern North America: Implications for Petroleum Generation: Geologic Note, AAPG Bull., 66, 1402–1407, 1982.
Kelemen, P. B., Hanghøj, K., and Greene, A. R.: One View of the Geochemistry of Subduction-Related Magmatic Arcs, with an Emphasis on Primitive Andesite and Lower Crust, Treatise on Geochemistry, 3, 1–70, https://doi.org/10.1016/b0-08-043751-6/03035-8, 2007.
Kemp, A. I. and Hawkesworth, C. J.: Granitic perspectives on the generation and secular evolution of the continental crust, Treatise on Geochemistry, Elsevier-Pergamon, 3, 349-410. https://doi.org/10.1016/B0-08-043751-6/03027-9, 2003.
Kendrick, J. E., Lavallée, Y., Hess, K.-U., Heap, M. J., Gaunt, H. E., Meredith, P. G., and Dingwell, D. B.: Tracking the permeable porous network during strain-dependent magmatic flow, J. Volcanol. Geoth. Res., 260, 117–126, https://doi.org/10.1016/j.jvolgeores.2013.05.012, 2013a.
Kendrick, J. E., Smith, R., Sammonds, M. P., Dainty, M., and Pallister, J. S.: The influence of thermal and cyclic stressing on the strength of rocks from Mount St. Helens, Washington, B. Volcanol., 75, 728, https://doi.org/10.1007/s00445-013-0728-z, 2013b.
Kennedy, L. A. and Russell, J. K.: Cataclastic production of volcanic ash at Mount Saint Helens, Phys. Chem. Earth A/B/C, 45–46, 40–49, https://doi.org/10.1016/j.pce.2011.07.052, 2012.
Kennedy, L. A., Russell, J. K., and Nelles, E.: Origins of Mount St. Helens cataclasites: Experimental insights, Am. Mineral., 94, 995–1004, https://doi.org/10.2138/am.2009.3129, 2009.
Kerr, A. C.: Oceanic Plateaus. In Treatise on Geochemistry, Elsevier, 3, 659, https://doi.org/10.1016/B0-08-043751-6/03033-4, 2003.
Khitarov, N. I., Lebedev, Y. B., Rengarten, Y. V., and Arsenyev, R. V.: Solubility of water in basaltic and granitic melts, Geokhimya, 10, 41–49, 1959.
Kidder, S., Ducea, M., Gehrels, G., Patchett, P. J., and Vervoort, J.: Tectonic and magmatic development of the Salinian Coast Ridge Belt, California, Tectonics, 22, 1058, https://doi.org/10.1029/2002TC001409, 2003.
Kläske, U.: Bestimmung des geothermischen Potenzials des Kristallinen Odenwaldes, Bachelorthesis, TU Darmstadt, 2010.
Klein, E. M.: Geochemistry of the Igneous Oceanic Crust, in: Treatise on Geochemistry, edited by: Holland, H. D. and Turekian, K. K., Elsevier, 3, 433–463, https://doi.org/10.1016/B0-08-043751-6/03030-9, 2003.
Klug, C. and Cashman, K. V.: Permeability development in vesiculating magmas: implications for fragmentation, B. Volcanol., 58, 87–100, https://doi.org/10.1007/s004450050128, 1996.
Klumbach, S.: Photogrammetrische und felsmechanische Untersuchungen am Piesberger Karbon-Quarzit, Bachelorthesis, Albert-Ludwigs-Universität Freiburg, 2008.
Klumbach, S.: Petrophysical properties of geothermal reservoir rocks in the Upper Rhine Graben from surface outcrops, Masterthesis, Albert-Ludwigs-Universität Freiburg, 2010.
Knopoff, L.: Geophysical Monograph 12: The Crust and Upper Mantle of the Pacific Area, edited by: Knopoff, L., Drake, C. L., and Hart, P. J., American Geophysical Union, https://doi.org/10.1029/gm012, 1968.
Königsberger, J. and Weiss, J.: Über die thermoelektrischen Effekte (Thermokräfte, Thomsonwärme) und die Wärmeleitung in einigen Elementen und Verbindungen und über die experimentelle Prüfung der Elektronentheorien, Annalen der Physik, 340, 1–46, https://doi.org/10.1002/andp.19113400602, 1911.
Kolzenburg, S., Heap, M. J., Lavallée, Y., Russell, J. K., Meredith, P. G., and Dingwell, D. B.: Strength and permeability recovery of tuffisite-bearing andesite, Solid Earth, 3, 191–198, https://doi.org/10.5194/se-3-191-2012, 2012.
Konzack, D.: Untersuchung des Einflusses der Wassersättigung auf die thermophysikalischen Eigenschaften von Buntsandstein, Bachelorthesis, TU Darmstadt, 2015.
Kramers, J. D., Kreissig, K., and Jones, M. Q.: Crustal heat production and style of metamorphism: a comparison between two Archean high grade provinces in the Limpopo Belt, southern Africa, Precambrian Res., 112, 149–163, https://doi.org/10.1016/S0301-9268(01)00173-5, 2001.
Kraus, E.: 3D Modellierung des tiefengeothermischen Potentials und der Petrologie des west-lichen Taunus/Rheinisches Schiefergebirge, Diplomathesis, TU Darmstadt, 2009.
Kristinsdóttir, L. H., Flóvenz, Ó. G., Árnason, K., Bruhn, D., Milsch, H., Spangenberg, E., and Kulenkampff, J.: Electrical conductivity and P-wave velocity in rock samples from high-temperature Icelandic geothermal fields, Geothermics, 39, 94–105, https://doi.org/10.1016/j.geothermics.2009.12.001, 2010.
Kukkonen, I. T., Golovanova, I. V., Khachay, Y. V., Druzhinin, V. S., Kosarev, A. M., and Schapov, V. A.: Low geothermal heat flow of the Urals fold belt – implication of low heat production, fluid circulation or palaeoclimate?, Tectonophysics, 276, 63–85, https://doi.org/10.1016/S0040-1951(97)00048-6, 1997.
Kumar, P. S. and Reddy, G. K.: Radioelements and heat production of an exposed Archaean crustal cross-section, Dharwar craton, south India, Earth Planet. Sc. Lett., 224, 309–324, https://doi.org/10.1016/j.epsl.2004.05.032, 2004.
Kumari, W. G., Ranjith, P. G., Perera, M. S., Shao, S., Chen, B. K., Lashin, A., and Rathnaweera, T. D.: Mechanical behaviour of Australian Strathbogie granite under in-situ stress and temperature conditions: An application to geothermal energy extraction, Geothermics, 65, 44–59, https://doi.org/10.1016/j.geothermics.2016.07.002, 2017.
Lambert, A.-D.: Bestimmung der petrophysikalischen und felsmechanischen Kennwerte des Tromm- und Weschnitz-Plutons, Odenwald, Deutschland, Bachelorthesis, TU Darmstadt, 2016.
Landolt-Börnstein – Springer Materials: the Landolt-Börnstein database, available at: http://www.springermaterials.com, last access: 14 August 2020.
Laštovičková, M., Losito, G., and Trova, A.: Anisotropy of electrical conductivity of dry and saturated KTB samples, Phys. Earth Planet. In., 81, 315–324, https://doi.org/10.1016/0031-9201(93)90138-Y, 1993.
Le Bas, M. J. and Streckeisen, A. L.: The IUGS systematics of igneous rocks, J. Geol. Soc. London, 148, 825–833, 1991.
Ledesert, B.: Fracturation et paléocirculations hydrothermales. Application au granite de Soultz-sous-Forêts, PhD dissertation, Université de Poitiers, France, 170 p., 1993POIT2308, 1993.
Lehnert, K., Su, Y., Langmuir, C., Sarbas, B., and Nohl, U.: A global geochemical database structure for rocks, Gechem. Geophys. Geosy., 1, 1012, https://doi.org/10.1029/1999GC000026, 2000.
Lehnert, K., Vinayagamoorthy, S., Djapic, B., and Klump, J.: The Digital Sample: Metadata, Unique Identification, and Links to Data and Publications, EOS, Transactions, American Geophysical Union, 87, Abstract IN53C–07, 2006.
Le Maitre, R. W. and Streckeisen, A.: Igneous rocks: a classification and glossary of terms – recommendations of the International Union of Geological Sciences Subcommission on the Systematics of Igneous Rocks, 2nd edn., Cambridge University Press, Cambridge, 2003.
Leonidov, Y.: Heat capacities of rocks at high temperatures, Geochem. Int., 4, 400, 1967.
Leonidov, Y., Barskiy, Y., and Khitarov N., I.: Measurement of heat capacities of quartz, kyanite and granite at high temperatures, Geochem. Int., 2, 1966.
Lesquer, A., Pagel, M., Orsini, J. B., and Bonin, B.: Premières détermination du flux de chaleur et de la production de chaleur en Corse, C.R. Acad. Sci., 491–494, 1983.
Leu, W., Keller, G., Matter, A., Schärli, U., and Rybach, L.: Geothermische Eigenschaften Schweizer Molassebecken (Tiefenbereich 0–500 m), Bundesamt für Energie, Bern, available at: https://inis.iaea.org/collection/NCLCollectionStore/_Public/39/015/39015171.pdf (last access: 28 September 2020), 2006
Linsel, A.: Lithofaziesanalyse der Forschungsbohrung Messel GA 1, Bachelorthesis, TU Darmstadt, 2014.
Liolios, P. and Exadaktylos, G.: A relational rock mechanics database scheme with a hierarchical structure, Comput. Geosci., 37, 1192–1204, https://doi.org/10.1016/j.cageo.2011.02.014, 2011.
Loaiza, S., Fortin, J., Schubnel, A., Gueguen, Y., Vinciguerra, S., and Moreira, M.: Mechanical behavior and localized failure modes in a porous basalt from the Azores, Geophys. Res. Lett., 39, L19304, https://doi.org/10.1029/2012GL053218, 2012
Luais, B. É. and Hawkesworth, C. H.: The Generation of Continental Crust: An Integrated Study of Crust-Forming Processes in the Archaean of Zimbabwe, J. Petrol., 35, 43–94, https://doi.org/10.1093/petrology/35.1.43, 1994.
Lucazeau, F. and Mailhe, D.: Heat flow, heat production and fission track data from the Hercynian basement around the Provençal Basin (Western Mediterranean), Tectonophysics, 128, 335–356, https://doi.org/10.1016/0040-1951(86)90300-8, 1986.
Mack, C.: Geothermische Untersuchungen in Calw, Diplomathesis, TU Darmstadt, 2007.
Mahood, G. A.: A summary of the geology and petrology of the Sierra La Primavera, Jalisco, Mexico, J. Geophys. Res.-Sol. Ea., 86, 10137–10152. https://doi.org/10.1029/JB086iB11p10137, 1981.
Maier, D.: Theory of Relational Databases, Computer Science Pr, Rockville, Maryland, USA, 640 pp., 1983.
Maire, R.: Investigation of thermo-physical and mechanical parameters of crystalline geothermal reservoir rocks of the Upper Rhine Graben (Germany), Masterthesis, LaSalle Beauvais, TU Darmstadt, 2014.
Manghnani, M. H. and Woollard, G. P.: Elastic Wave Velocities in Hawaiian Rocks at Pressures to Ten Kilobars, in: Geophysical Monograph 12:The Crust and Upper Mantle of the Pacific Area, edited by: Knopoff, L., Drake, C. L., and Heart, P. J., American Geophysical Union, 12, 501–516, https://doi.org/10.1029/GM012p0501, 2013.
Mareschal, J. C., Poirier, A., Rolandone, F., Bienfait, G., Gariépy, C., Lapointe, R., and Jaupart, C.: Low mantle heat flow at the edge of the North American Continent, Voisey Bay, Labrador, Geophys. Res. Lett., 27, 823–826, https://doi.org/10.1029/1999GL011069, 2000.
Mariucci, M. T., Pierdominici, S., Pizzino, L., Marra, F., and Montone, P. Looking into a volcanic area: An overview on the 350 m scientific drilling at Colli Albani (Rome, Italy), J. Volcanol. Geoth. Res., 176, 225–240, https://doi.org/10.1016/j.jvolgeores.2008.04.007, 2008.
Marzán Blas, I.: Régimen tér mico en la Península Ibérica. Estructura litosférica a través del Macizo Ibérico y el Margen Surportugués, PhD dissertation, PhD Universitat de Barcelona, 2000.
McDermott, F., Harris, N. B., and Hawkesworth, C. J.: Geochemical constraints on crustal anatexis: a case study from the Pan-African Damara granitoids of Namibia, Contrib. Mineral. Petr., 123, 406–423, https://doi.org/10.1007/s004100050165, 1999.
McDonough, W. F.: Constraints on the composition of the continental lithospheric mantle, Earth Planet. Sc. Lett., 101, 1–18, https://doi.org/10.1016/0012-821X(90)90119-I, 1990.
McKenna, T. E. and Sharp Jr., J. M.: Radiogenic heat production in sedimentary rocks of the Gulf of Mexico Basin, south Texas, AAPG Bull., 82, 484–496, 1998.
McLaren, S., Sandiford, M., and Hand, M.: High radiogenic heat–producing granites and metamorphism – An example from the western Mount Isa inlier, Australia, Geology, 27, 679, https://doi.org/10.1130/0091-7613(1999)027<0679:hrhpga>2.3.co;2, 1999.
McLennan, S. M., Taylor, S. R., McCulloch, M. T., and Maynard, J. B.: Geochemical and Nd-Sr isotopic composition of deep-sea turbidites: Crustal evolution and plate tectonic associations, Geochim. Cosmochim. Ac., 54, 2015–2050, https://doi.org/10.1016/0016-7037(90)90269-Q, 1990.
Mengel, K., Richter, M., and Johannes, W.: Leucosome-forming small-scale geochemical processes in the metapelitic migmatites of the Turku area, Finland, Lithos, 56, 47–73, https://doi.org/10.1016/S0024-4937(00)00059-1, 2001.
Meqbel, N. and Ritter, O.: Joint 3D inversion of multiple electromagnetic datasets, Geophys. Prospect., 63, 1450–1467, https://doi.org/10.1111/1365-2478.12334, 2015.
Mielke, P.: Properties of the Reservoir Rocks in the Geothermal Field of Wairakai/New Zealand, Diplomathesis, TU Darmstadt, 2009.
Mielke, P., Nehler, M., Bignall, G., and Sass, I.: Thermo-physical rock properties and the impact of advancing hydrothermal alteration – A case study from the Tauhara geothermal field, New Zealand, J. Volcanol. Geoth. Res., 301, 14–28, https://doi.org/10.1016/j.jvolgeores.2015.04.007, 2015.
Mielke, P., Bär, K., and Sass, I.: Determining the relationship of thermal conductivity and compressional wave velocity of common rock types as a basis for reservoir characterization, J. Appl. Geophys., 140, 135–144, https://doi.org/10.1016/j.jappgeo.2017.04.002, 2017.
Milicevic, M.: Untersuchung thermophysikalischer Eigenschaften an hessischen Zechstein- und Buntsandsteinproben. Bachelorthesis, TU Darmstadt, 2015.
Milord, I., Sawyer, E. W., and Brown, M.: Formation of Diatexite Migmatite and Granite Magma during Anatexis of Semi-pelitic Metasedimentary Rocks: an Example from St. Malo, France, J. Petrol., 42, 487–505, https://doi.org/10.1093/petrology/42.3.487, 2001.
Misener, A. D., Thompson, L. G., and Uffen, R. J.: Terrestrial heat flow in Ontario and Quebec. Transactions, American Geophysical Union, 32, 729, https://doi.org/10.1029/tr032i005p00729, 1951.
Mitchell, R. H.: Kimberlites, Orangeites, and Related Rocks, Springer US, https://doi.org/10.1007/978-1-4615-1993-5, 1995.
Moiseenko, U. I.: Wärmeleitfähigkeit der Gesteine bei hohen Temperaturen, Freiberger Forschungshefte, 100, 89–94, 1968.
Moiseenko, U. I. and Sokolova, L. S.: Thermal conductivity of some rocks of the East Sayan and Eastern Kazhakstan, Reports of the Academy of Sciences of the USSR, 165, 1965.
Moiseenko, U. I. and Sokolova, L. S: Ergebnisse geothermischer Untersuchungen in Sibirien, Freiberger Forschungshefte, C238, 79, 1968.
Moiseenko, U. I., Soloviev, S. A., and Kutolin, V. A.: Thermal conductivity of granite at high temperatures, Reports of the Academy of Sciences of the USSR, 165, 1965.
Moiseenko, U. I., Solovyeva, Z. A., and Kutolin, V. A.: Heat capacities of eclogite and dolerite at high temperatures, Doklady Akademii Nauk SSSR, 173, 669–671, 1967.
Montanini, A. and Tribuzio, R.: Gabbro-derived Granulites from the Northern Apennines (Italy): Evidence for Lower-crustal Emplacement of Tholeiitic Liquids in Post-Variscan Times, J. Petrol., 42, 2259–2277, https://doi.org/10.1093/petrology/42.12.2259, 2001.
Mortimer, N.: PETLAB: New Zealand's rock and geoanalytical database, Geological Society of New Zealand Newsletter, 136, 27–31, 2005.
Mossop, S. C. and Gafner, G.: The thermal constants of some rocks from the Orange Free State, J. Chem. Met. Min. Soc. S. Afr., 52, 61–76, 1951.
Mottaghy, D., Schellschmidt, R., Popov, Y. A., Clauser, C., Kukkonen, I. T., Nover, G., and Romushkevich, R. A.: New heat flow data from the immediate vicinity of the Kola super-deep borehole: Vertical variation in heat flow confirmed and attributed to advection, Tectonophysics, 401, 119–142, https://doi.org/10.1016/j.tecto.2005.03.005, 2005.
Müller, D.: Investigation on the spatial variability of petrophysical parameters of sandstone (Buntsandstein) in the Spessart, Germany, Bachelorthesis, TU Darmstadt, 2014.
Munck, F., Walgenwitz, F., Maget, P., Sauer, K., and Tietze, R.: Synthese géothermique du Fossé rhénan Supérieur, Commission of the European Communities, Strasbourg, France, Freiburg i.Br., Germany, 1979.
Munoz, G., Bauer, K., Moeck, I., Schultze, A., and Ritter, O.: Exploring the Gross Schönebeck (Germany) geothermal site using a statistical joint interpretation of magnetotelluric and seismic tomography models, Geothermics, 39, 35–45, https://doi.org/10.1016/j.geothermics.2009.12.004, 2010.
Murti, M.: Patterns of sedimentation in the southeastern part of the Chhattisgarh Basin, PhD dissertation, University of Saugar, India, 1980.
Nabawy, B. S., Rochette, P., and Geraud, Y.: Petrophysical and magnetic pore network anisotropy of some cretaceous sandstone from Tushka Basin, Egypt, Geophys. J. Int., 177, 43–61, https://doi.org/10.1111/j.1365-246X.2008.04061.x, 2009.
Nabawy, B. S., Rochette, P., and Géraud, Y.: Electric pore fabric of the Nubia sandstones in south Egypt: characterization and modelling, Geophys. J. Int., 183, 681–694, https://doi.org/10.1111/j.1365-246X.2010.04789.x, 2010.
Narayanaswamy, R. and Venkatasubramanian, V. S.: Uranium and thorium contents of coexisting gneisses, granites and pegmatites, Geochim. Cosmochim. Ac., 33, 1007–1009, https://doi.org/10.1016/0016-7037(69)90111-2, 1969.
National Geothermal Data System (NGDS): National Geothermal Data System, available at: http://geothermaldata.org/, last access: 26 February 2020.
Nehler, M.: Geothermische Untersuchungen des Zechsteins im Raum Sontra, Bachelorthesis, TU Darmstadt, 2011.
Nicolaysen, L. O., Hart, R. J., and Gale, N. H.: The Vredefort radioelement profile extended to supracrustal strata at Carletonville, with implications for continental heat flow, J. Geophys. Res.-Sol. Ea., 86, 10653–10661, https://doi.org/10.1029/JB086iB11p10653, 1981.
Noritomi, K. and Asada, A.: Studies on the Electrical Conductivity of a few Samples of Granite and Andesite, Sci. Rep. Tohoku Univ. Ser., 5, 201–207, 1955.
Nyblade, A. A., Pollack, H. N., Jones, D. L., Podmore, F., and Mushayandebvu, M.: Terrestrial heat flow in east and southern Africa, J. Geophys. Res.-Sol. Ea., 95, 17371, https://doi.org/10.1029/JB095iB11p17371, 1990.
Oelsner, C.: Grundlagen der Geothermik, Sektion Geowissenschaften, Bergakademie, Freiberg, Germany, 1981.
Orendt, R.: Geothermisches Potenzial im Erlaubnisfeld Südtaunus, Diplomathesis, TU Darmstadt, 2014.
Pannike, D. G., Kölling, M., Schulz, H. D., Panteleit, B., Reichling, J., and Scheps, V.: Auswirkung hydrogeologischer Kenngrößen auf die Kältefahnen von Erdwärmesondenanlagen in Lockersedimenten, Grundwasser, 11, 6–18, https://doi.org/10.1007/s00767-006-0114-2, 2006.
Pannike, S.: Ausbreitung von Kältefahnen oberflächennaher Erdwärmesonden in Lockergestein, 48 pp., PhD dissertation, Universität Bremen, 2005.
Parkhomenko, E. I. and Bondarenko, A. T.: Electrical conductivity of rocks at high pressures and temperatures, Nauka Press, Moscow, 1972.
Paslick, C., Halliday, A., James, D., and Dawson, J. B.: Enrichment of the continental lithosphere by OIB melts: Isotopic evidence from the volcanic province of northern Tanzania, Earth Planet. Sc. Lett., 130, 109–126, https://doi.org/10.1016/0012-821X(95)00002-T, 1995.
Peate, D. W.: The Paraná-Etendeka Province, in: Large Igneous Provinces: Continental, Oceanic, and Planetary Flood Volcanism, American Geophysical Union, 217–245, https://doi.org/10.1029/gm100p0217, 1997.
Peccerillo, A. and Taylor, S. R.: Geochemistry of eocene calc-alkaline volcanic rocks from the Kastamonu area, Northern Turkey, Contrib. Mineral. Petr., 58, 63–81, https://doi.org/10.1007/BF00384745, 1976.
Pei, L.: Analysis of initiation and propagation of hydraulically induced fracture, Masterthesis, TU Darmstadt, 2009.
Pereira, E. B., Hamza, V. M., Furtado, V. V., and Adams, J. A.: U, Th and K content, heat production and thermal conductivity of São Paulo, Brazil, continental shelf sediments: A reconnaissance work, Chem. Geol., 58, 217–226, https://doi.org/10.1016/0168-9622(86)90011-4, 1986.
PetroMod – Petroleum systems Modeling Software: PetroMod – Petroleum systems Modeling Software, available at: http://www.slb.com/petromod, last access: 26 February 2020.
PETLAB: National Rock and Geoanalytical Database: Petlab, available at: http://pet.gns.cri.nz/#/ or https://www.gns.cri.nz/Home/Products/Databases/PETLAB-National-Rock-and-Geoanalytical-Database, last access: 26 February 2020.
Pickett, D. A. and Saleeby, J. B.: Thermobarometric constraints on the depth of exposure and conditions of plutonism and metamorphism at deep levels of the Sierra Nevada Batholith, Tehachapi Mountains, California, J. Geophys. Res.-Sol. Ea., 98, 609–629, https://doi.org/10.1029/92JB01889, 1993.
Pimienta, L., Sarout, J., Esteban, L., and Piane, C. D.: Prediction of rocks thermal conductivity from elastic wave velocities, mineralogy and microstructure, Geophys. J. Int., 197, 860–874, https://doi.org/10.1093/gji/ggu034, 2014.
Plank, T. and Langmuir, C. H.: The chemical composition of subducting sediment and its consequences for the crust and mantle, Chem. Geol., 145, 325–394, https://doi.org/10.1016/S0009-2541(97)00150-2, 1998.
Pola, A., Crosta, Fusi, N., Barberini, V., and Norini, G.: Influence of alteration on physical properties of volcanic rocks, Tectonophysics, 566–567, 67–86, https://doi.org/10.1016/j.tecto.2012.07.017, 2012.
Pola, A., Crosta, G. B., Fusi, N., and Castellanza, R.: General characterization of the mechanical behaviour of different volcanic rocks with respect to alteration, Eng. Geol., 169, 1–13, https://doi.org/10.1016/j.enggeo.2013.11.011, 2014.
Popov, Y. A., Pribnow, D. F., Sass, J. H., Williams, C. F., and Burkhardt, H.: Characterization of rock thermal conductivity by high-resolution optical scanning, Geothermics, 28, 253–276, https://doi.org/10.1016/S0375-6505(99)00007-3, 1999.
Pribnow, D. F., Kinoshita, M., and Stein, C. A.: Thermal Data Collection and Heat Flow Recalculations for ODP Legs 101–180, Inst. Joint Geosci. Res., Inst. Geowiss. Gemeinschaftsauf. (GGA), Hanover, Germany, 2000.
Price, R. C., Gray, C. M., and Frey, F. A.: Strontium isotopic and trace element heterogeneity in the plains basalts of the Newer Volcanic Province, Victoria, Australia, Geochim. Cosmochim. Ac., 61, 171–192, https://doi.org/10.1016/s0016-7037(96)00318-3, 1997.
Priebs, R.: Properties of geothermal reservoir rocks. Comparison between Ohaaki Field (NZ) and Saar-Nahe Basin (Germany), Diplomathesis, TU Darmstadt, 2011.
Rao, G. V. and Rao, R. U.: Heat flow in Indian Gondwana basins and heat production of their basement rocks, Tectonophysics, 91, 105–117, https://doi.org/10.1016/0040-1951(83)90060-4, 1983.
Rao, R. U. and Rao, G. V.: Radiogeologic studies at NGRI – a review, Geophys. Research Bull., 17, 163–173, 1979.
Rao, V. D., Aswathanarayana, U., and Qureshy, M. N.: Trace element geochemistry of parts of the Closepet granite, Mysore State, India, Mineral. Mag., 38, 678–686, https://doi.org/10.1180/minmag.1972.038.298.04, 1972.
Ray, L., Förster, H.-J., Förster, A., Fuchs, S., Naumann, R., and Appelt, O.: Tracking the thermal properties of the lower continental crust: Measured versus calculated thermal conductivity of high-grade metamorphic rocks (Southern Granulite Province, India), Geothermics, 55, 138–149, https://doi.org/10.1016/j.geothermics.2015.01.007, 2015.
Rebay, G. and Spalla, M. I.: Emplacement at granulite facies conditions of the Sesia–Lanzo metagabbros: an early record of Permian rifting?, Lithos, 58, 85–104, https://doi.org/10.1016/s0024-4937(01)00046-9, 2001.
Reyer, D. and Philipp, S. L.: Empirical relations of rock properties of outcrop and core samples from the Northwest German Basin for geothermal drilling, Geothermal Energy Science, 2, 21–37, https://doi.org/10.5194/gtes-2-21-2014, 2014.
Reynaud, C., Jaillard, É., Lapierre, H., Mamberti, M., and Mascle, G. H.: Oceanic plateau and island arcs of southwestern Ecuador: their place in the geodynamic evolution of northwestern South America, Tectonophysics, 307, 235–254, https://doi.org/10.1016/s0040-1951(99)00099-2, 1999.
Ringrose, P. and Bentley, M.: Reservoir Model Design, Springer, the Netherlands, 249, https://doi.org/10.1007/978-94-007-5497-3, 2015.
Roberts, J. J., Bonner, B. P., and Duba, A. G.: Electrical resistivity measurements of andesite and hydrothermal breccia from the Awibengkok geothermal field, Indonesia. TwentyFifth Annual Stanford Geothermal Reservoir Engineering Workshop, 339–344, available at: http://www.geothermal-energy.org/pdf/IGAstandard/SGW/2000/Roberts.pdf (last access: 14 August 2020), 2000.
Robertson S.: Classification of metamorphic rocks, BGS Rock Classification Scheme, Volume 2, British Geological Survey, Research Report Number RR 99-02, Nottingham, UK, 1999.
Rogers, N. W., James, D., Kelley, S. P., and Mulder, M. D.: The Generation of Potassic Lavas from the Eastern Virunga Province, Rwanda, J. Petrol., 39, 1223–1247, https://doi.org/10.1093/petroj/39.6.1223, 1998.
Rolandone, F., Jaupart, C., Mareschal, J. C., Gariépy, C., Bienfait, G., Carbonne, C., and Lapointe, R.: Surface heat flow, crustal temperatures and mantle heat flow in the Proterozoic Trans-Hudson Orogen, Canadian Shield, J. Geophys. Res.-Sol. Ea., 107, ETG 7-1–ETG 7-19, https://doi.org/10.1029/2001jb000698, 2002.
Rosener, M.: Etude pétrophysique et modélisation des effets des transferts thermiques entre roche et fluide dans le contexte géothermique de Soultz-sous-Forêts, PhD dissertation, Université Louis Pasteur-Strasbourg I, available at: https://tel.archives-ouvertes.fr/tel-00202959/ (last access: 14 August 2020), 2007.
Rudnick, R. L. and Fountain, D. M.: Nature and composition of the continental crust: A lower crustal perspective, Rev. Geophys., 33, 267, https://doi.org/10.1029/95rg01302, 1995.
Rudnick, R. L., McDonough, W. F., and O'Connell, R. J.: Thermal structure, thickness and composition of continental lithosphere, Chem. Geol., 145, 395–411, https://doi.org/10.1016/s0009-2541(97)00151-4, 1998.
Rudnick, R. O.: Xenolith-samples of the lower continental crust, Continental lower crust, Elsevier, Amsterdam, 23, 269–316, 1992.
Rühaak, W. Guadagnini, A., Geiger, S., Bär, K., Gu, Y., Aretz, A., Homuth, S., and Sass, I.: Upscaling thermal conductivities of sedimentary formations for geothermal exploration, Geothermics, 58, 49–61, https://doi.org/10.1016/j.geothermics.2015.08.004, 2015.
Rüther, J.: The Validation of the LMC device – Analysis of Icelandic basaltic rocks, Masterthesis, TU Darmstadt, RES – The School of Renewable Energy Science, Iceland, Akureyri, 2011.
Rummel, F.: Physical properties of the rock in the granitic section of borehole GPK1, Soultzsous- Forêts, Geotherm. Sci. & Tech., 3, 199–216, 1991.
Rummel, F.: Physical properties of the rock in the granitic section of borehole GPK1, Soultz-sous-Forêts, in: Geothermal Energy in Europe: the soultz hot dry rock project, edited by: Bresee, J. C., Gordon and Breach Science Publishers, Montreaux, Switzerland, 199–216, 1992.
Rummel, F. and Schreiber, D.: Physical properties of the core K21, borehole GPK1, Soultz-sous-Forêts, depth interval 3522.58–3525.88 m, Yellow Report 12, Ruhr-Universität Bochum, 1993.
Russell, J. K., Dipple, G. M., and Kopylova, M. G.: Heat production and heat flow in the mantle lithosphere, Slave craton, Canada, Phys. Earth Planet. In., 123, 27–44, https://doi.org/10.1016/S0031-9201(00)00201-6, 2001.
Rybach, L., Bassetti, S., Baumgärtner, R., Rohner, E., and Schärli, U.: Projekt Drahtloser Minidatenlogger für Temperaturmessungen in Erdwärmesonden, Schlussbericht, 2003.
Sakvarelidze, E. A.: Verkhnaya Mantiya, Izd. Nauka, 125, 1973.
Salters, V. J., Storey, M., Sevigny, J. H., and Whitechurch, H.: Trace element and isotopic characteristics of Kerguelen-Heard Plateau basalts, Proc. Ocean Drill. Program Sci. Results, 120, 55–62, 1992.
Salton, M.: Untersuchungen zum Verhalten von Erdwärmesonden, Masterthesis, Diplomarbeit an der Abt. für Erdwissenschaften der ETH Zürich, 1999.
Sandiford, M., Mclaren, S., and Neumann, N.: Long-term thermal consequences of the redistribution of heat-producing elements associated with large-scale granitic complexes, J. Metamorph. Geol., 20, 87–98, https://doi.org/10.1046/j.0263-4929.2001.00359.x, 2002.
Sandkühler, L.: Untersuchung des Einflusses der Wassersättigung auf die thermophysikalischen Eigenschaften von Kernproben der Bohrungen Aura und Rosenthal, Bachelorthesis, TU Darmstadt, 2015.
Sanner, B. and Anderson, O.: Drilling methods for shallow geothermal installations, Tech. rep., International Geothermal Days, Germany, 2001.
Sanner, B., Reuß, M., Mands, E., and Müller, J.: Erfahrungen mit dem thermal response test in Deutschland, Tagungsband 6, Geothermische Fachtagung Herne, GtV, Geeste, 2000.
Sass, J. H., Lachenbruch, A. H., and Munroe, R. J.: Thermal conductivity of rocks from measurements on fragments and its application to heat-flow determinations, J. Geophys. Res., 76, 3391–3401, https://doi.org/10.1029/jb076i014p03391, 1971.
Sawka, W. N. and Chappell, B. W.: Fractionation of uranium, thorium and rare earth elements in a vertically zoned granodiorite: Implications for heat production distributions in the Sierra Nevada batholith, California, U.S.A., Geochim. Cosmochim. Ac., 52, 1131–1143, https://doi.org/10.1016/0016-7037(88)90267-0, 1988.
Schäffer, R.: Hydrogeologische und geothermische Untersuchungen der Heilquellen und Heil-brunnen Bad Soden-Salmuensters, Diplomathesis, TU Darmstadt, 2012.
Schärli, U. and Kohl, T.: Archivierung und Kompilation geothermischer Daten der Schweiz und angrenzender Gebiete, Schweizerische Geophysikalische Kommission, Zürich, Switzerland, 2002.
Schintgen, T. V.: The Geothermal Potential of Luxembourg, PhD dissertation, University of Potsdam, Universität Potsdam, available at: http://nbn-resolving.de/urn/resolver.pl?urn=urn:nbn:de:kobv:517-opus4-87110 (last access: 14 August 2020), 2015.
Schmid, R.: Descriptive nomenclature and classification of pyroclastic deposits and fragments: Recommendations of the IUGS Subcommission on the Systematics of Igneous Rocks, Geology, 9, 41–43, 1981.
Schön, J. H.: Petrophysik: Physikalische Eigenschaften von Gesteinen und Mineralen, F. Enke, Stuttgart, 1983.
Schön, J. H.: Handbook of geophysical exploration. Physical Properties of Rocks: Fundamentals and Principles of Petrophysics, Pergamon Pr., Oxford, 421 pp., 1996.
Schön, J. H.: Physical properties of rocks: fundamentals and principles of petrophysics (Bd. 18), Pergamon Pr., Oxford, 1998.
Schön, J. H.: Physical Properties of Rocks: Fundamentals and Principles of Petrophysics, in: Handbook of Geophysical Explorations, Section I, Seismic Exploration, 18, Redwood Books, Trowbridge, 2004.
Schön, J. H.: Physical Properties of Rocks: A Workbook, in: Handbook of Petroleum Exploration and Production, Elsevier, Amsterdam, the Netherlands, 2011.
Schön, J. H. (Ed.): Physical properties of rocks: Fundamentals and principles of petrophysics, Developments in petroleum science, 65, Elsevier, Amsterdam Netherlands, 2015.
Schöpflin, S.: Determination of Rockmechanical Properties of the Palatinate Forest's Buntsandstein, Master's thesis, Albert-Ludwigs-Universität Freiburg, 2013.
Schubert, K. O.: Geothermische Untersuchungen des Buntsandsteins des Odenwaldes, Bachelorthesis, TU Darmstadt, Goethe Universitaet Frankfurt am Main, 2011.
Schumann, A.: GIS basierte Erdwaermepotenzialkarte Spielberg und Wart, Diplomathesis, TU Darmstadt, 2008.
Schütz, F.: Surface heat flow and lithospheric thermal structure of the northwestern Arabian Plate, PhD dissertation, University of Potsdam, University of Potsdam, Germany, available at: https://nbn-resolving.org/urn:nbn:de:kobv:517-opus-69622 (last access: 14 August 2020), 2013.
Schwalb, B.: Thermofazielle und petrophysikalische Untersuchung der Bohrung Solnhofen-Maxberg und weiterer Aufschlussproben der Fränkischen und Schwäbischen Alb, Bachelorthesis, TU Darmstadt, 2012.
Schwalb, B.: Bestimmung geothermischer Kennwerte an Cuttings und Auswertung der bohrtechnischen Daten der Tiefbohrung Geretsried, Masterthesis, TU Darmstadt, 2014.
Shimojuku, A., Yoshino, T., Yamazaki, D., and Okudaira, T.: Electrical conductivity of fluid-bearing quartzite under lower crustal conditions, Phys. Earth Planet. In., 198–199, 1–8, https://doi.org/10.1016/j.pepi.2012.03.007, 2012.
Sighinolfi, G. P., Figueredo, M. C., Fyfe, W. S., Kronberg, B. I., and Oliveira, M. A.: Geochemistry and petrology of the jequie granulitic complex (Brazil): an archean basement complex, Contrib. Mineral. Petr., 78, 263–271, https://doi.org/10.1007/bf00398921, 1981.
Sikora, C.: Strukturgeologische und geothermische Analogstudie verkarsteter Karbonatgesteine im Bereich der Tunnelbaustelle Widderstall, Masterthesis, TU Darmstadt, 2015.
Sims, P. K., Peterman, Z. E., Prinz, W. C., Benedict, F. C., and Klasner, J. S.: Geology, geochemistry, and age of Archean and early Proterozoic rocks in the Marenisco-Watersmeet area, northern Michigan; and Geologic interpretation of gravity data, Marenisco-Watersmeet area, northern Michigan, Tech. rep., Geological Survey Professional Paper, 1292A-B, 41 p. and 13 p., United States Government Printing Office, Washington, https://doi.org/10.3133/pp1292AB, 1984.
Siratovich, P.: Thermal Stimulation of Icelandic High-Temperature Geothermal Systems. Mastersthesis, RES – The School of Renewable Energy Science, Iceland, Akureyri, 2010.
Siratovich, P. A., Heap, M. J., Villenueve, M. C., Cole, J. W., and Reuschlé, T.: Physical property relationships of the Rotokawa Andesite, a significant geothermal reservoir rock in the Taupo Volcanic Zone, New Zealand, Geotherm. Energy, 2, 31, https://doi.org/10.1186/s40517-014-0010-4, 2014.
Siratovich, P. A., Heap, M. J., Villeneuve, M. C., Cole, J. W., Kennedy, B. M., Davidson, J., and Reuschlé, T.: Mechanical behaviour of the Rotokawa Andesites (New Zealand): Insight into permeability evolution and stress-induced behaviour in an actively utilised geothermal reservoir, Geothermics, 64, 163–179, https://doi.org/10.1016/j.geothermics.2016.05.005, 2016.
Sizun, J. P.: Modification des structures de porosité de grès lors de transformations petrographiques dans la diagenese et l'hydrothermalisme, PhD dissertation, Institut de Geologie Strasbourg, Université Louis Pasteur, Strasbourg, France, 1995.
Smith, I. E. and Johnson, R. W.: Contrasting rhyolite suites in the Late Cenozoic of Papua New Guinea, J. Geophys. Res.-Sol. Ea., 86, 10257–10272, https://doi.org/10.1029/JB086iB11p10257, 1981.
Smith, R., Sammonds, P. R., Tuffen, H., and Meredith, P. G.: Evolution of the mechanics of the 2004–2008 Mt. St. Helens lava dome with time and temperature, Earth Planet. Sc. Lett., 307, 191–200, https://doi.org/10.1016/j.epsl.2011.04.044, 2011.
Southern Methodist University: SMU Geothermal Lab Heat Flow and Western Geothermal Data Bases References, Tech. rep., Southern Methodist University, Dallas, Texas, USA, 2000.
Stober, I. and Bucher, K.: Hydraulic properties of the crystalline basement, Hydrogeol. J., 15, 213–224, https://doi.org/10.1007/s10040-006-0094-4, 2007.
Stober, I. and Jodocy, M.: Eigenschaften geothermischer Nutzhorizonte im Baden-Württembergischen und französischen Teil des Oberrheingrabens, Grundwasser, 14, 127–137, https://doi.org/10.1007/s00767-009-0103-3, 2009.
Strong, D., Turnbull, R., Haubrock, S., and Mortimer, N.: Petlab: New Zealand's national rock catalogue and geoanalytical database, New Zealand J. Geol. Geophys., 53, 475–481, https://doi.org/10.1080/00288306.2016.1157086, 2016.
Sun, S.-S. and McDonough, W. F.: Chemical and isotopic systematics of oceanic basalts: implications for mantle composition and processes, Geol. Soc. London Spec. Publications, 42, 313–345, https://doi.org/10.1144/GSL.SP.1989.042.01.19, 1989.
Sundberg, J.: Thermal properties of soils and rocks, PhD dissertation, Chalmers University of Technology and University of Goetenburg, 1988.
Surma, F.: Détermination de la porosité des zones endommagées autour des failles et rôle de l'état du matériau sur les propriétés d'echange fluides-roches: minéralogie, structures de porosité, caractéristiques mécaniques, PhD dissertation, Université Louis Pasteur, Strasbourg, 2003.
Surma, F. and Geraud, Y.: Porosity and Thermal Conductivity of the Soultz-sous-Forêts Granite, in: Thermo-Hydro-Mechanical Coupling in Fractured Rock, Birkh_user, Basel, 1125–1136, https://doi.org/10.1007/978-3-0348-8083-1_20, 2003.
Swanberg, C. A.: Vertical distribution of heat generation in the Idaho batholith, J. Geophys. Res., 77, 2508–2513, https://doi.org/10.1029/JB077i014p02508, 1972.
Taylor, S. R., McLennan, S. M., and McCulloch, M. T.: Geochemistry of loess, continental crustal composition and crustal model ages, Geochim. Cosmochim. Ac., 47, 1897–1905, https://doi.org/10.1016/0016-7037(83)90206-5, 1983.
Taylor, W. R., Tompkins, L. A., and Haggerty, S. E.: Comparative geochemistry of West African kimberlites: Evidence for a micaceous kimberlite endmember of sublithospheric origin, Geochim. Cosmochim. Ac., 58, 4017–4037, https://doi.org/10.1016/0016-7037(94)90264-X, 1994.
Tchameni, R., Mezger, K., Nsifa, N. E., and Pouclet, A.: Neoarchæan crustal evolution in the Congo Craton: evidence from K rich granitoids of the Ntem Complex, southern Cameroon, J. Afr. Earth Sci., 30, 133–147, https://doi.org/10.1016/S0899-5362(00)00012-9, 2000.
Tejada, M. L., Mahoney, J. J., Duncan, R. A., and Hawkins, M. P.: Age and Geochemistry of Basement and Alkalic Rocks of Malaita and Santa Isabel, Solomon Islands, Southern Margin of Ontong Java Plateau, J. Petrol., 37, 361–394, https://doi.org/10.1093/petrology/37.2.361, 1996.
Tejada, M. L., Mahoney, J. J., Neal, C. R., Duncan, R. A., and Petterson, M. G.: Basement Geochemistry and Geochronology of Central Malaita, Solomon Islands, with Implications for the Origin and Evolution of the Ontong Java Plateau, J. Petrol., 43, 449–484, https://doi.org/10.1093/petrology/43.3.449, 2002.
Thompson, R. N., Gibson, S. A., Dickin, A. P., and Smith, P. M.: Early Cretaceous Basalt and Picrite Dykes of the Southern Etendeka Region, NW Namibia: Windows into the Role of the Tristan Mantle Plume in Paraná–Etendeka Magmatism, J. Petrol., 42, 2049–2081, https://doi.org/10.1093/petrology/42.11.2049, 2001.
Trautwein, U.: Poroelastische Verformung und petrophysikalische Eigenschaften von Rotliegend Sandsteinen. Technische Universität Berlin, Technische Universität Berlin, https://doi.org/10.14279/depositonce-1240, 2005.
Ucok, H.: Temperature dependence of the electrical resistivity of aqueous salt solutions and solution-saturated porous rocks, Master's thesis, University of Southern California, 1979.
Ufondu, L. S.: The geothermal potentials of the Middle and Lower Benue Trough, Nigeria, Masterthesis, TU Darmstadt, 2012.
Van Wees, J.-D., Hopman, J., Dezayes, C., Vernier, R., Manzella A., Bruhn, D., Scheck-Wenderoth, M., Flovenz, O., Páll Hersir, G. Halldórsdóttir, S., and Liotta, D.: IMAGE: the EU Funded Research Project Integrated Methods for Advanced Geothermal Exploration, Proceedings World Geothermal Congress 2015, Melbourne, Australia, 19–25 April 2015.
VDI 4640 Blatt 1: Thermische Nutzung des Untergrundes – Grundlagen, Genehmigungen, Umweltaspekte, VDI, Düsseldorf, 2010.
Verdoya, M., Pasquale, V., Chiozzi, P., and Kukkonen, I. T.: Radiogenic heat production in the Variscan crust: new determinations and distribution models in Corsica (northwestern Mediterranean), Tectonophysics, 291, 63–75, https://doi.org/10.1016/s0040-1951(98)00031-6, 1998.
Vernoux, J. F. and Lambert, M.: Rapport BRGM SGN/IRG ARG 93 T37, Tech. rep., BRGM, Orleans, France, 1993.
Vernoux, J. F., Genter, A., Razin, P., Vinchon, C., Orléans, B. R., and Nord-Pas de Calais, B. R.: Geological and petrophysical parameters of a deep fractured sandstone formation as applied to geothermal exploitation, BRGM Report, 38622, 70, available at: http://infoterre.brgm.fr/rapports/RR-38622-FR.pdf (last access: 14 August 2020), 1995.
Vilà, M., Fernández, M., and Jiménez-Munt, I.: Radiogenic heat production variability of some common lithological groups and its significance to lithospheric thermal modeling, Tectonophysics, 490, 152–164, https://doi.org/10.1016/j.tecto.2010.05.003, 2010.
Villaseca, C., Downes, H., Pin, C., and Barbero, L.: Nature and Composition of the Lower Continental Crust in Central Spain and the Granulite-Granite Linkage: Inferences from Granulitic Xenoliths, J. Petrol., 40, 1465–1496, https://doi.org/10.1093/petroj/40.10.1465, 1999.
Violay, M., Pezard, P. A., Ildefonse, B., Belghoul, A., and Laverne, C.: Petrophysical properties of the root zone of sheeted dikes in the ocean crust: A case study from Hole ODP/IODP 1256D, Eastern Equatorial Pacific, Tectonophysics, 493, 139–152, https://doi.org/10.1016/j.tecto.2010.07.013, 2010.
Violay, M., Gibert, B., Azais, P., Pezard, P. A., and Lods, G.: A New Cell for Electrical Conductivity Measurement on Saturated Samples at Upper Crust Conditions, Transport Porous Med., 91, 303–318, https://doi.org/10.1007/s11242-011-9846-2, 2012a.
Violay, M., Gibert, B., Mainprice, D., Evans, B., Dautria, J.-M., Azais, P., and Pezard, P.: An experimental study of the brittle-ductile transition of basalt at oceanic crust pressure and temperature conditions, J. Geophys. Res.-Sol. Ea., 117, B03213, https://doi.org/10.1029/2011jb008884, 2012b.
Vogel, B. C.: Petrophysikalische und felsmechanische Untersuchung kristalliner Gesteine des Weschnitz-Plutons, Bachelorthesis, TU Darmstadt, 2016.
Volarovich, M. P.: Geophysical Aspects of the Problems of Elastic and Electrical Properties of Rocks at Pressures Up to Tens of Kilobars, in: Geophysical Monograph 12: The Crust and Upper Mantle of the Pacific Area, edited by: Knopoff, L., Drake, C. L., and Heart, P. J., American Geophysical Union, 12, 517–522, https://doi.org/10.1029/gm012p0517, 1968.
Walters, M. A. and Combs, J.: Heat flow in The Geysers-Clear Lake geothermal area of northern California, USA, Monograph on the Geysers Geothermal Field, 43–53, available at: https://www.geothermal-library.org/index.php?mode=pubs&action=view&record=1005473 (last access: 14 August 2020), 1991.
Wang, J.-H., Hung, J.-H., and Dong, J.-J.: Seismic velocities, density, porosity, and permeability measured at a deep hole penetrating the Chelungpu fault in central Taiwan, J. Asian Earth Sci., 36, 135–145, https://doi.org/10.1016/j.jseaes.2009.01.010, 2009.
Weaver, B. L., Wood, D. A., Tarney, J., and Joron, J. L.: Geochemistry of ocean island basalts from the South Atlantic: Ascension, Bouvet, St. Helena, Gough and Tristan da Cunha, Geological Society, London, Special Publications, 30, 253–267, https://doi.org/10.1144/GSL.SP.1987.030.01.11, 1987.
Weber, J. N.: Geothermische Aufschlussanalyse des Steinbruches Mainzer Berg, oestlich von Darmstadt, Bachelorthesis, TU Darmstadt, 2014.
Weber, J. N.: Vorplanung einer geothermischen Nutzung der Tiefbohrung Rotenburg HKZ 1001, Masterthesis, TU Darmstadt, 2016.
Weinert, S.: Geomechanical Characterization of Volcanic Rocks of the Taupo and Coromandel Volcanic Zone, New Zealand, Masterthesis, TU Darmstadt, 2014.
Welsch, B.: Geothermische Untersuchungen an ausgewählten Standorten der Schwäbischen Alb, Bachelorthesis, TU Darmstadt, 2011.
Welsch, B.: Forschungsbohrung Heubach: Untersuchungen zu den geothermischen Reservoireigenschaften des Odenwald Kristallins, Diplomathesis, TU Darmstadt, 2012.
Welsch, B., Baer, K., Schulte, D., Rühaak, W., and Chauhan, S.: Simulation und Evaluierung von Kopplungs- und Speicherkonzepten regenerativer Energieformen zur Heizwärmeversorgung, Final report, TU Darmstadt, 2015.
Wenk, H.-R. and Wenk, E.: Physical constants of Alpine rocks (density, porosity, specific heat, thermal diffusivity and conductivity), Schweizerische mineralogische und petrographische Mitteilungen, 49, 343–357, available at: http://nbn-resolving.de/urn:nbn:de:hebis:30-1096297 (last access: 14 August 2020), 1969.
Weydt, L. M.: Analogue Study of Geothermal Properties of the Rimbey-Meadowbrook Reef Trend in the Alberta Basin, Canada, Masterthesis, TU Darmstadt, 2016.
Whitford, D. J.: Strontium isotopic studies of the volcanic rocks of the Saunda arc, Indonesia, and their petrogenetic implications, Geochim. Cosmochim. Ac., 39, 1287–1302, https://doi.org/10.1016/0016-7037(75)90136-2, 1975.
Wicke, H.: Faziesabhängigkeit geothermischer Kennwerte am Beispiel des Oberen Muschelkalk (Crailsheim, Baden-Württemberg), Diplomathesis, TU Darmstadt, 2009.
Wickham, S. M.: Crustal Anatexis and Granite Petrogenesis During Low-pressure Regional Metamorphism: The Trois Seigneurs Massif, Pyrenees, France, J. Petrol., 28, 127–169, https://doi.org/10.1093/petrology/28.1.127, 1987.
Wiesner, P.: Modellierung einer mitteltiefen Erdwaermesonde zur Heiz-Grundlastabdeckung des Taunusgymnasiums Koenigstein, Masterthesis, TU Darmstadt, 2014.
Wilkinson, M., Dumontier, M., Aalbersberg, I., Appleton, G., Axton, M., Baak, A., Blomber, N., Boiten, J.-W., da silca Santos, L. B., Bourne, P. E., Bouwman, J., Brookes, A. J., Clark, T., Crosas, M., Dillo, I., Dumon, O., Edmunds, S., Evelo, C. T., Finkers, R., Gonzalez-Beltran, A., Gray, A. J. G., Groth, P., Goble, C., Grethe, J. S., Heringa, J., Hoen, P. A. C., Hooft, R., Kuhn, T., Kok, R., Kok, J., Lusher, S. J., Martone, M. E., Mons, A., Packer, A. L., Persson, B., Rocca-Serra, P., Roos, M., van Schaik, R., Sansone, S.-A., Schultes, E., Sengstag, T., Slater, T., Strawn, G., Swertz, M. A., Thompson, M., van der Lei, J., van Mulligen, E., Velterop, J., Waagmeester, A., Wittenburg, P., Wolstencroft, K., Zhao, J., and Mons, B.: The FAIR Guiding Principles for scientific data management and stewardship, Sci Data., 3, 160018, https://doi.org/10.1038/sdata.2016.18, 2016.
Winkler, H. G.: Landolt-Börnstein, Zahlenwerte und Funktionen Bd. III Astronomie und Geophysik, edited by: Bartels, J. and ten Bruggencate, P., Springer-Verlag, Berlin Heidelberg, ISBN 978-3-662-41604-4, 1952.
Wittig, T.: Charakterisierung der Aquifereigenschaften von Dolomiten der Wustkogel-Formation im Raum Tux, Tirol, Masterthesis, TU Darmstadt, 2017.
Zhao, X. G., Wang, J., Chen, F., Li, P. F., Ma, L. K., Xie, J. L., and Liu, Y. M.: Experimental investigations on the thermal conductivity characteristics of Beishan granitic rocks for Chinas HLW disposal, Tectonophysics, 683, 124–137, https://doi.org/10.1016/j.tecto.2016.06.021, 2016.
Zhu, W., Baud, P., Vinciguerra, S, and Wong, T.-F.: Micromechanics of brittle faulting and cataclastic flow in Alban Hills tuff, J. Geophys. Res., 116, B06209, https://doi.org/10.1029/2010JB008046, 2011.
Zhu, W., Baud, P., Vinciguerra, S., and Wong, T.-F.: Micromechanics of brittle faulting and cataclastic flow in Mount Etna basalt, J. Geophys. Res.-Sol. Ea., 121, 4268–4289, https://doi.org/10.1002/2016JB012826, 2016.
Zilch, D.: Geothermische Gesteinskennwerte der palaeozoischen Gesteine des Werra-Grauwacken-Aufbruchs. Bachelorthesis, TU Darmstadt, Goethe Universitaet Frankfurt am Main, 2011.
Zoth, G.: Nordwestdeutschland im Tertiär, edited by: Tobien, H., Gebrüder Bornträger, Stuttgart, Germany, ISBN 9783443110185, 1986.
- Abstract
- Introduction
- Contents and structure of the database
- Status of the database, data availability and quality
- Discussion
- Data availability
- Conclusions and perspectives
- Appendix A: List of references for Figs. 4 and 5
- Appendix B
- Author contributions
- Competing interests
- Acknowledgements
- Financial support
- Review statement
- References
- Abstract
- Introduction
- Contents and structure of the database
- Status of the database, data availability and quality
- Discussion
- Data availability
- Conclusions and perspectives
- Appendix A: List of references for Figs. 4 and 5
- Appendix B
- Author contributions
- Competing interests
- Acknowledgements
- Financial support
- Review statement
- References