the Creative Commons Attribution 4.0 License.
the Creative Commons Attribution 4.0 License.
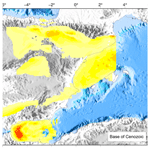
SedDARE-IB: an open-access repository of sediment data for the Iberian Peninsula and its continental margins
Tiago M. Alves
Ivone Jiménez-Munt
Joao Carvalho
Conxi Ayala
Elsa C. Ramalho
Angela María Gómez-García
Hugo Matias
Hanneke Heida
Abraham Balaguera
José Luis García-Lobón
Jaume Vergés
Sediments provide valuable information for geologists and geophysicists whenever they strive to understand, and reproduce, the geological evolution, lithology, rock properties, seismic response, and geohazards of a region. The analysis of sedimentary sequences is thus useful for the interpretation of depositional environments, sea-level change, climate change, and recognition of the sediments' source areas. By integrating sedimentary data into geophysical modeling, such interpretations are improved in terms of their accuracy and reliability. To further help our understanding of the Iberian Peninsula's geological evolution, geological resources, and geohazards, this work presents to the scientific community the SedDARE-IB data repository. This repository includes available data on the depth to the Base of the Cenozoic and Top of the Paleozoic stratigraphic markers for the Iberian Peninsula, the surrounding West Iberian Atlantic Margin and Western Mediterranean Neogene basins, or the acoustic basement as interpreted for the Valencia Trough and Alboran Mediterranean basins. As an example of the broad applicability of the data included in SedDARE-IB, we investigate how sediment thickness affects the depth to the 150 °C isotherm in specific basins, as commonly used in geothermal exploration. The calculated trend suggests that, given constant measured surface heat flow and thermal conductivity, the 150 °C isotherm becomes shallower as the sediment thickness increases, until a critical threshold value is reached for the latter. The SedDARE-IB database has been compiled thanks to a Portuguese–Spanish collaboration promoting open data exchange among institutions and research groups. SedDARE-IB is freely available at https://doi.org/10.20350/digitalCSIC/16277 (Torne et al., 2024), bringing opportunities to the scientific, industrial, and educational communities for diverse applications.
- Article
(7735 KB) - Full-text XML
-
Supplement
(300 KB) - BibTeX
- EndNote
Sediments are crucial to our understanding of the geological evolution, lithology, rock properties, subsurface structure, geological resources, and geohazards affecting particular regions. By analyzing strata and their architecture, geologists can decipher past environmental conditions, changes in sea level, climate variability, and the nature of rocks in source areas. Their thickness is often indicative of the presence of important resources such as oil, gas, or minerals. Also, sedimentary rocks encompass a wide range of lithologies with distinct physical and mechanical properties, detailed knowledge of which is essential in geophysical models. Incorporating sedimentary data into geophysical modeling enhances the accuracy and reliability of subsurface models for subsequent resource exploration. In addition, sediments can contribute to the triggering or amplification of multiple geohazards, e.g., landslides, soil liquefaction, and local seismicity. By studying properties such as cohesion, shear strength, and the degree of compaction in sediments, one can assess potential geohazards in a region and develop proper mitigation strategies.
Sediment data curation contributes to scientific research and holds cultural significance, offering glimpses into human history and the development of morphotectonic landscapes over time. Overall, sediment data curation is integral for advancing knowledge, sustaining resource management, protecting the environment, and making informed decisions in diverse scientific, economic, and social fields. To facilitate our understanding of Iberian geology, resources, and hazards, this work presents SedDARE-IB as a comprehensive repository of (a) the depth to the Base of the Cenozoic strata deposited in both extensional and compressional basins and (b) Top of the Paleozoic units for the majority of the onshore and offshore sedimentary basins of the Iberian Peninsula. SedDARE-IB includes sedimentary data on onshore basins as varied as the foreland basins of the Pyrenean–Cantabrian Orogen (Ebro and Duero basins) and Betic Cordillera (Guadalquivir Basin), the Tagus Basin and the Lusitanian and Lower Tagus basins, and many other small basins whose locations are shown in Figs. 1b and 2. Offshore, SedDARE-IB comprises data from the West Iberian Atlantic Margin (Alentejo, Peniche, Northern Lusitanian Basin, and deep offshore depocenters), the Gulf of Cadiz region (Algarve Basin and its surroundings), and the Base of the Cenozoic for the Western Mediterranean Neogene basins, e.g., the Valencia Trough and the Alboran Basin, also comprising the acoustic basement for the rest of the Western Mediterranean region (see Figs. 1b and 2 for the locations).
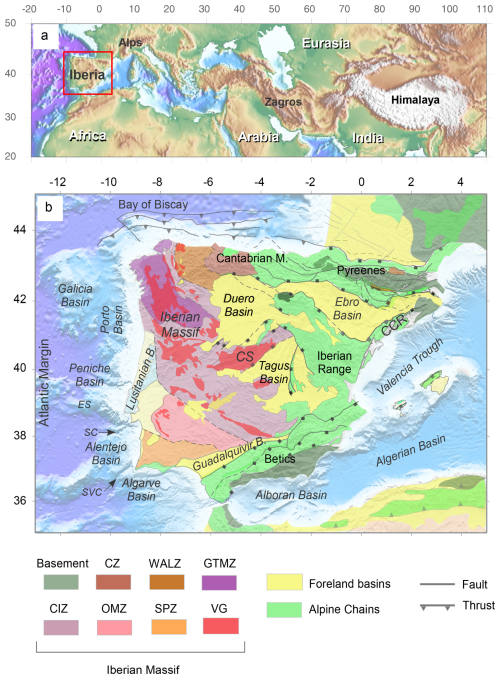
Figure 1(a) Elevation map of the Alpine–Himalayan Orogenic Belt as shown by the shaded area. The red square highlights the locations of the Iberian Peninsula and its surrounding margins. (b) Simplified geological map of the Iberian Peninsula. Iberian Massif abbreviations: CZ – Cantabrian Zone; CIZ – Central Iberian Zone; GTMZ – Galicia-Tras-Os-Montes Zone; OMZ – Ossa Morena Zone; SPZ – South Portuguese Zone; VG – Variscan Granitoids; and WALZ – Western Asturian Leonese Zone. Other abbreviations: Cantabrian M. – Cantabrian Mountains; CCR – Catalan Coastal Range; CS – Central System; ES – Estremadura Spur; Guadalquivir B. – Guadalquivir Basin; LTB – Lower Tagus Basin; SC – Setubal Canyon; and SVC – Sao Vicente Canyon. Adapted from Torne et al. (2015, 2023).
As an example of the potential uses of the SedDARE-IB data, we analyze the depth of the 150 °C isotherm for different surface heat flow values. Sediments play a crucial role in geothermal energy assessments as they serve as a natural insulating layer, regulating heat transfer between the subsurface and Earth's surface. Hence, we examine in this work how sediment thickness influences subsurface temperature. SedDARE-IB can be used in geothermal studies as sediments influence heat conduction, reservoir evaluation, temperature distribution, drilling considerations, thermal conductivity, heat flow modeling, resource assessment, and environmental impact assessments. Understanding sediment thickness is therefore essential for effective planning, development, and use of geothermal energy resources.
The data included in SedDARE-IB are summarized in Table S1 in the Supplement, which details the primary sources of the data; the geological areas covered; key attributes such as the minimum, maximum, and average thickness ranges; and key references associated with the datasets.
2.1 The Iberian Plate during the Alpine Wilson Cycle
The Iberian Plate, presently attached to Europe along the Pyrenean orogenic system, constitutes the westernmost segment of the 12 000 km Alpine–Himalayan Orogenic Belt, which was shaped as a result of the Late Cretaceous–Cenozoic closure of the different branches of the Tethys Ocean. Such a process resulted in the continental collision of Africa, Arabia, and India with Eurasia (e.g., Dercourt et al., 1986). During the Variscan Orogenic Cycle, the relatively small Iberian Plate was located in a hinge region between Pangea and Gondwana, with its northern and southern boundaries marked by large transfer faults – currently the Pyrenees and Betic orogenic systems. In spite of having been relatively bound by the larger African and Eurasian plates, the Iberian Plate evolved independently for hundreds of millions of years, a characteristic that shaped its fairly complex Variscan and Alpine systems of basins and mountain chains. Comprehensive data on the Iberian Peninsula's Variscan evolution are given in Friend and Dabrio (1995), Gibbons and Moreno (2002), Vera et al. (2004), and Quesada and Oliveira (2019).
Permian and Triassic continental rifting in the proto-Atlantic domain culminated with the opening of the Central Atlantic Ocean south of Iberia, with subsequent transtensional motion of Africa and opening of the Ligurian Tethys starting at ca. 156 Ma (Late Jurassic – Vergés and Fernàndez, 2006). This oceanized Jurassic lithosphere shaped the boundaries of Iberia by developing the rift-related Algarve, Prebetic, and Subbetic basins to the south and the Catalan basin to the east. Such basins were later included as part of the Betic and Catalan Coastal ranges' orogenic systems. The northern propagation of the southern North Atlantic starting at about 118 Ma (Early Cretaceous) occurred along the Iberian Atlantic margin and led to the formation of large rift-related basins of Permo–Triassic and Jurassic–Lower Cretaceous ages. Such basins developed along the Iberian Atlantic margin and formed the Alentejo, Lusitanian, Peniche, Porto, and Galicia Bank basins (Fig. 1).
The opening of the central and southern North Atlantic segments initiated the fairly synchronous eastwards drift of both Africa and Iberia with respect to Eurasia along the inherited Variscan northern boundary of the Iberian Peninsula, a phenomenon that started in the Early Cretaceous and ended in the Late Santonian at around 83.5 Ma (Macchiavelli et al., 2017). The salt-rich, transtensional Basque–Cantabrian basin mostly evolved during this time (Cámara, 2017), together with the Organyà (Casini et al., 2023) and North Pyrenees (Ford and Vergés, 2021) basins.
North–south convergence between Africa and Eurasia started at 83.5 Ma (Coniacian) and is still active at present. Africa–Eurasia convergence resulted in significant deformation within Iberia and affected the previous Iberian Mesozoic rift-related basins. Along the northern margin of Iberia, the Pyrenees emerged because of continental collision and limited northward subduction. On the southern margin of Iberia, a more complex region involving the Betics and Rif mountain ranges, the Alboran Sea, and the Gulf of Cadiz formed the subduction-related Betic–Rif orogenic system. The interior of Iberia was also shortened, mostly along relatively weak intra-continental regions associated with Mesozoic rifting. This triggered tectonic inversion in the E–W Basque–Cantabrian Basin, the NW–SE Iberian and Altomira ranges, the NE–SE Central System, and the NE–SW Catalan Coastal Range (Muñoz-Martín and de Vicente, 1998; Casas-Sainz and Faccenna, 2001; Vergés and Fernàndez, 2006; de Vicente et al., 2011; Vergés et al., 2019). All of these inversion-related fold-and-thrust systems are linked at particular connection zones, where distinct fold trends coexist. The smooth variations in structural trends among compressive fold-and-thrust belts, as illustrated on the Iberia Geological Map at a scale of 1:1 000 000 (Rodríguez-Fernández et al., 2015), suggest their simultaneous development, at least to some extent (Anadón and Roca, 1996; Casas-Sainz and Faccenna, 2001; Vergés and Fernàndez, 2006). In such a regional context, the western margin of Iberia along Portugal also shows mild inversion tectonics, which becomes more significant towards the south (e.g., Alves et al., 2003b; Zitellini et al., 2004; Terrinha et al., 2009; Ramos et al., 2017; Cunha, 2019; Alves, 2024).
2.2 Iberian mainland
In mainland Iberia, the SedDARE-IB data were organized into six (6) major areas: (a) the Pyrenees and Ebro Basin (PE), (b) the Duero and Basque–Cantabrian basins (DB), (c) the Iberian Range and the Tagus and Almazán basins (ITA), (d) the Guadalquivir Basin and the External Betics (GB), and the Lower Tagus Cenozoic Basin (LTCB) (Fig. 2). For the Top of the Paleozoic, the Ebro Basin domain and the Tagus and Almazán basin area have been expanded to the southern Pyrenees and the Iberian Range, respectively. (Fig. 2). Figures 3 and 4 show the locations of the points and seismic profiles included in the database, while Figs. 5 and 6 are examples of the grids generated from the database.
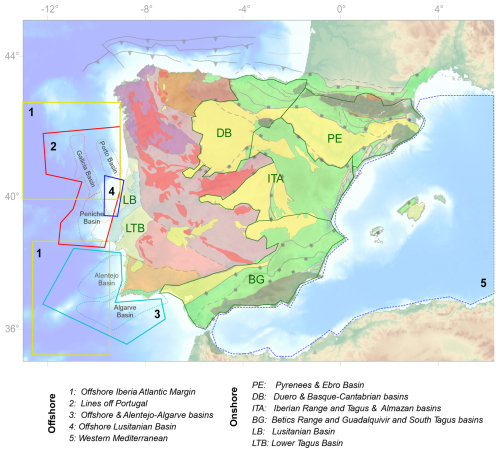
Figure 2Simplified geological map showing the locations of the datasets listed in Table S1. Modified from Torne et al. (2015, 2023).
2.2.1 Ebro Foreland Basin
The Ebro Foreland Basin, spanning approximately 85 500 km2, is limited by the Pyrenees and the Basque–Cantabrian Fold Belt to the north. The basin represents the latest stage of the long-lived evolution of the southern Pyrenean foreland basin, which has lasted from the latest Cretaceous until the present day. The Ebro Foreland Basin comprises a 5–6 km Paleocene–Oligocene sedimentary infill, displaying an irregular geometry in map view, as it is also bounded by the Catalan Coastal Range to the southeast and the Iberian Range to the southwest. The basin records a long-lasting evolution: this first originated as the Pyrenean foreland basin and was later filled with shallow- to deep-marine deposits along a tectonic trough that became subparallel to the southern front of the Pyrenees (Puigdefàbregas et al., 1992). The basin changed to an endorheic system at approximately 36 Ma as the western Pyrenees marine gateway was closed (Costa et al., 2009). Alluvial, fluvial, and lacustrine depositional systems prevailed until the Middle to Upper Miocene (García-Castellanos et al., 2003).
The Ebro Foreland Basin remained endorheic for about 27 million years until the Oligo-Miocene opening of the Western Mediterranean basins. This opening triggered the formation of a Mediterranean river system, leading to the capture of its drainage network (Lewis et al., 2000). It also caused significant denudation: an area of 25 000 to 45 000 km2 was eroded at a rate of 47–80 mm kyr−1, a phenomenon that resulted in the subsequent isostatic rebound of the basin by up to 630 m (García-Castellanos and Larrasoaña, 2015). The depositional record of this erosion is found in the Castellón Group of the Valencia Trough (Martínez del Olmo, 1996; Arche et al., 2010).
2.2.2 Duero and Basque–Cantabrian basins
The intermontane Duero Basin, with an area of approximately 98 500 km2, is bounded by several mountain ranges and is one of the highest alpine intra-montane basins in Europe (Casas-Sainz and De Vicente, 2009) (Fig. 1b). Its average altitude is in excess of 800 m. It contains a thicker sedimentary infill in its northern sector that is associated with the alpine fronts of the inverted Basque–Cantabrian Basin and the alpine reactivation of the Variscan Cantabrian Range. This fill gradually thins out towards the southern and western boundaries of the basin on Variscan basement rocks (Civis and Vera, 2004; Herrero et al., 2004; Quesada and Oliveira, 2019). The sediment infill above Mesozoic deposits varied across different geological epochs, with changing thicknesses and areal extents. The Paleogene to Upper Miocene sedimentary infill generally exceeds 2500 m in the basin's center. Notably, the youngest sedimentary infill in the basin is correlated with the top of the fluvial–lacustrine carbonates of the Páramo Formation, which are dated to about 9.6 Ma (Krijgsman et al., 1996; Cunha, 2019). The opening of the Duero Basin to the Atlantic Ocean occurred in the late Pliocene to Pleistocene, with further incision occurring later. The exact timing of this opening is still debated, with some suggesting that it occurred around 1.1–1.9 Ma and others proposing that it might have happened earlier, in the middle Tortonian. Regardless of its age, the opening of the Duero Basin to the Atlantic Ocean marked a significant reorganization of fluvial networks within itself (Antón et al., 2012, 2019; Silva et al., 2017; Struth et al., 2019 and 2021).
2.2.3 Tagus and Almazán basins
The Tagus Basin, located in the center of the Iberian Peninsula and covering approximately 20 000 km2, is bordered by the Central System to the north, the Iberian Range to the northeast, and the western sector of the Toledo Mountains to the south. It comprises two distinct subbasins: the intra-montane Madrid Basin and the Loranca (also known as the Intermediate Depression), which comprises a piggy-back depocenter in the eastern part of the Tagus Basin (Muñoz-Martín, 1997). These basins were individualized due to tectonic uplift of the Sierra de Altomira during the late Paleogene, and their Cenozoic evolution was influenced by tectonics and climate change (Alonso-Zarza et al., 2004). Sediments in the Tagus Basin consist of Paleogene shales, gypsum, conglomerates, and limestones predominantly formed in alluvial fan systems alongside evaporitic lakes and lacustrine marls. The estimated thickness is up to 3000 m, and its maximum deposition occurred during the Miocene period. Miocene sediments comprise alluvial fan and lacustrine facies, which are capped by lacustrine limestones and Pliocene detrital sediments. The Intermediate Depression is a narrow depocenter located to the east of the Madrid Basin and contains Upper Cretaceous shales and gypsum, younger alluvial and lacustrine fan intervals, fluvial and floodplain sediments, Pliocene lacustrine sands and limestones (Paramo limestones), and other detrital materials (Alonso-Zarza et al., 2004; de Vicente et al., 2004).
2.2.4 Lower Tagus Basin
The Lower Tagus Basin is surrounded by Mesozoic sediments of the Lusitanian Basin to the west and pre-Mesozoic igneous and metamorphic rocks to the east. The basin is exclusively Cenozoic in age and sits on Cretaceous strata in its southern part, Middle Jurassic units in its northern part, and Paleozoic rocks in its eastern part. Paleogene continental strata, 200–400 m thick, accumulated first in the basin during the Late Eocene and Oligocene in response to NNE–SSW convergence of Iberia and Africa (Carvalho, 1995; Cabral et al., 2003). Miocene tectonics overprinted the Paleogene structures, with the Lower Tagus Basin evolving as a compressive foredeep basin related to tectonic inversion of the Lusitanian Basin under NW–SE compression. Miocene strata, exceeding 800 m in local depocenters near Lisbon (Cabral et al., 2003), include sandstones, clays, and limestones deposited in shallow-marine, mixed lagoonal, shallow-water, and continental environments (Pais et al., 2012). Tectonic subsidence in the basin slowed down during the Pliocene, as marked by the deposition of about 300 m of fluvial sediments, mainly sands and clayey or silty sands. Tectonic uplift predominated after the Early Pleistocene, while sands and clays topped by levels of pebbles are typically found in Pleistocene strata. These are overlain by Holocene alluvium deposited by the Tagus River. Tectonic compression continues to the present day, with maximum horizontal compressive stress trending NW–SE to WNW–ESE (e.g., Ribeiro et al., 1996; Borges et al., 2001).
2.2.5 Guadalquivir Foreland Basin
The Guadalquivir Foreland Basin, located in the southern part of the Iberian Peninsula and spanning ca. 57 000 km2, is a foreland basin bounded to the north by the Iberian Massif and to the south by the Betic Cordillera, which continues into the Rif Chain of northern Africa. Like the westernmost part of the Betics, it was influenced by post-Cretaceous tectonic movements between Africa and Eurasia. Studies suggest significant N–S convergence to have affected the Guadalquivir Basin from the mid-Oligocene to the late Miocene, followed by WNW-directed oblique convergence until the present day (Macchiavelli et al., 2017). According to Barnolas et al. (2019) and the references therein, the basin can be divided into two zones. The northern zone is filled with autochthonous sediments, whereas the southern zone also contains chaotic masses of Mesozoic and Cenozoic allochthonous materials that slid from the Subbetic units during the Late Miocene compression in the external Betics. The autochthonous sedimentary infill involves six Miocene seismic–stratigraphic sequences, ranging in age from the Middle Miocene (the late Langhian to the early Serravallian) to the Late Miocene (Messinian). Overlaying these Miocene sequences are Pliocene–Quaternary strata that record westward sediment progradation along the basin axis (Berástegui et al., 1998). The southern border of the basin involves several salt diapirs, with Triassic evaporites in their cores, that were tectonically compressed during the Cenozoic. They form multiple frontal imbricate wedges. The frontal imbricates involve late Serravallian to late Tortonian sediments (sequences 3 to 5, as described by Berástegui et al., 1998). In particular, Sequence 6, which spans the late Tortonian to the late Messinian, clearly postdates all the structural features. This indicates that significant shortening in the External Betics was concluded by approximately 6.3 Ma (Messinian). Tectonic activity, including normal faulting at the basement level, occurred during the early to middle Serravallian. The basin primarily originated through orogenic wedge accretion along its active southern margin, with flexural subsidence propagating towards the foreland basin per se (García-Castellanos et al., 2002). The Guadalquivir Foreland Basin depocenter is 600 m thick in the east but is up to 1800 m thick in its western region. Readers are directed to the work of Civis and Vera (2004) and Barnolas et al. (2019) for further details on the basin's sedimentary infill and evolution.
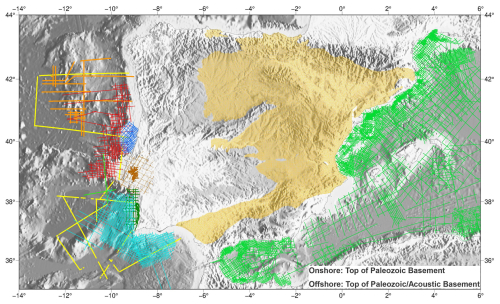
Figure 3Offshore: locations of the seismic profiles used to interpret and gather data for the Top of the Paleozoic or acoustic basement for most of the Western Mediterranean region. Exceptions are the Valencia Trough and the Alboran Basin (see Fig. 1 for the locations), where the acoustic basement corresponds to the Base of the Cenozoic or the so-called Oligocene unconformity. Onshore: locations of data obtained from the available seismic and log data and geological information.
2.3 Iberian offshore basins
2.3.1 West Iberian Atlantic Margin
The West Iberian Atlantic Margin is, essentially, a divergent margin reactivated tectonically since the end of the Cretaceous. It witnessed continental rifting from the Late Triassic to the Early Cretaceous. Diachronous continental breakup along this margin occurred over a period spanning from the latest Jurassic to the Albian or Cenomanian (Alves and Cunha, 2018). Breakup propagated from the southwest to northern Iberia, extending towards the Bay of Biscay (Grevemeyer et al., 2022). Two main episodes of magmatism are recorded during this process: the first around 200 Ma (Hettangian) associated with the Central Atlantic Magmatic Province (CAMP) and the second during the Valanginian period at 135–130 Ma (Martins et al., 2008).
Post-rift magmatism occurred after 80 Ma (Campanian) and was predominantly alkaline. It is recorded onshore by subvolcanic complexes near Sintra, Sines, and Monchique, with volcanic complexes also present near Lisbon and offshore from the Algarve (Terrinha et al., 2009). Also, onshore, Lower Cretaceous magmatism has been dated as spanning the range from 94 to 69 Ma in Portugal and occurring at ca. 79 Ma in northeastern Spain (Ubide et al., 2014).
At present, the continental slope of Iberia dips markedly to the west in its northwestern part but is gentler in its central and southwestern parts due to the accumulation of thick Cretaceous–Cenozoic strata (Alves et al., 2009). Late Cretaceous–Cenozoic exhumation and erosion are evident, particularly in the proximal part of the slope (Terrinha et al., 2002; Pereira and Alves, 2013; Alves, 2024). The Estremadura Spur, separating southwestern Iberia from its northwestern part, has experienced significant post-rift tectonics and magmatism (Miranda et al., 2009) associated with compressional tectonics and tectonic inversion (Ribeiro et al., 1990) (Fig. 1).
In summary, the West Iberian Atlantic Margin features a relatively narrow, westerly-dipping continental shelf, onto which majors rivers feed significant volumes of sediments north of 41° N. Sediment accumulation is significant in estuaries south of this latitude (Dias and Nittrouer, 1984). During sea level lowstands, sediment bypasses the shallower zones of the margin through large submarine canyons, leading to a reduced terrigenous sediment contribution to the outer shelf region (Dias and Nittrouer, 1984; Dias et al., 2002). Sediment composition on the shelf varies with depth, from fine micaceous sand at shallow depths to biogenic carbonate at greater depths (Dias and Nittrouer, 1984). The shelf edge comprises rocky outcrops and fine-grained sediment, resembling the gentler Alentejo continental margin (Dias et al., 2002). The continental slope is narrow and steep, bounded by north-trending to north-northeast-trending faults (Alves et al., 2003b), except for the wider and structurally deformed Estremadura Spur. The continental rise is relatively wide, featuring multiple seamounts and prominent fault-bounded depressions between seamounts where sediment transported by submarine canyons accumulates (Alves et al., 2003a).
2.3.2 Alentejo Basin
The offshore Alentejo region is characterized by its broad continental shelf, which blends with a gentle continental slope (∼ 0.5°) due to thick Cenozoic sediment deposition west of 10° W (Alves et al., 2003a). Steeper gradients (> 2°) occur near seamounts in areas of localized deformation (Coppier and Mougenot, 1982; Alves et al., 2003a). Alves et al. (2003a) recognized three main types of Cenozoic deposits on the Alentejo Margin: shelf-related, turbidite, and contourite or canyon fill. A wide range of sedimentary deposits, from deep-marine turbidites to fluvial or deltaic sequences (Shanmugam and Moiola, 1988), has been identified in seismic data crossing the continental slope of Alentejo. However, dredge samples near the shelf break contain shallow-marine to shelf-break limestones that are rich in foraminifera (Alves et al., 2003a). Deep-marine deposits, including mass-flow and channel-fill deposits, are common in more distal parts of the margin, while shallow-marine breccias are common on the continental slope (Coppier and Mougenot, 1982).
The Alentejo Basin, structured during the Upper Jurassic, is delimited by the Setúbal and São Vicente canyons. Limited exploratory drilling has revealed Hettangian–Triassic evaporites, suggesting that the Alentejo Basin constitutes the southern continuation of the Lusitanian Basin (Alves et al., 2003a. Extensive Late Cretaceous magmatic activity led to the emplacement of the Sines and underwater Côvo igneous complexes (Neres et al., 2023) when crustal extension shifted to compressional tectonics, reactivating crustal faults (Alves, 2024). Several compressional pulses with different directions affected the region, driven by interactions between Iberia, the Nubia microplate, and the larger European and African tectonic plates (e.g., Juarez et al., 1998; Rosenbaum et al., 2002; Vissers and Meijer, 2012).
2.3.3 Algarve Basin
The Algarve Basin, located in southwestern Iberia, originated during Mesozoic rifting due to the Atlantic and Neo-Tethys opening; it later experienced tectonic inversion during the Alpine orogeny (Terrinha et al., 2019). This basin trends east to west and extends for about 150 km, reaching a maximum width of 25 km in its onshore portion and about 100 km offshore. Bounded by Carboniferous basement rocks to the north and the Guadalquivir Bank to the south, crustal extension was accommodated by N–S and NE–SW structures in the westernmost part of the basin and ENE–WSW to E–W structures in the central and eastern parts. N–S- to NW–SE-striking faults bound the main grabens and half-grabens (Terrinha et al., 2019). Five main extensional episodes occurred from the Late Triassic to Cenomanian and were interspersed with transient compressive events that resulted in depositional hiatuses throughout the basin.
Paleozoic rocks below the Algarve Basin consist of low-grade metamorphic rocks (shales and graywackes) that are part of the Baixo Alentejo Flysch Group. In the basin per se, the oldest deposits are Lower Triassic in age (red mudstones) and are overlain by conglomerates and red clays of the “Silves sandstones” (Palain, 1976). Sedimentation continued through the Mesozoic and was characterized by the accumulation of alternating carbonate and siliciclastic units, indicating shallow-marine to continental environments. This was interrupted in the Cretaceous by tectonic inversion resulting from oblique convergence between northwestern Africa and Iberia, causing important depositional hiatuses and unconformities. The oldest offshore Cenozoic deposits in the Algarve Basin are dated as Paleocene to Oligocene, laying unconformably over folded Lower Cretaceous strata (Lopes et al., 2006; Matias et al., 2005a, b).
In summary, Cenozoic strata overlay folded and thrusted older units, suggesting that tectonic inversion occurred predominantly after the Cenomanian and lasted until the Late Oligocene to Aquitanian. Nevertheless, present-day compression is still recorded in the Algarve Basin, being NW–SE-oriented and driven by oblique collision between northwestern Africa and southwestern Eurasia (Ribeiro et al., 1996). Figures 3 and 4 show the locations of the points and seismic profiles included in the database, while Figs. 5 and 6 are examples of the grids generated from the database.
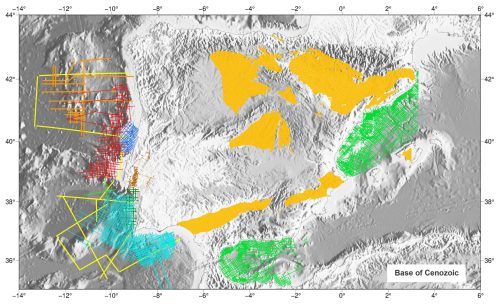
Figure 4Offshore: locations of the seismic profiles used for interpreting and gathering data on the Base of the Cenozoic. Onshore: locations of data obtained from the available seismic well-log data, and geological information. The main references from which data have been gathered are shown in Table S1. Additional references can also be found throughout the text.
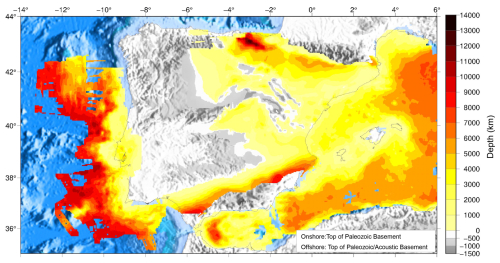
Figure 5Offshore: depth to the Top of the Paleozoic or acoustic basement for the majority of the Western Mediterranean region. Exceptions are the Valencia Trough and the Alboran Basin (see Fig. 1 for the locations), where the top of the basement corresponds to the Base of the Cenozoic or the so-called Oligocene unconformity. Onshore: depth to the Top of the Paleozoic Basement interpreted as the Paleozoic cover sequence boundary or Hercynian discordance (Garcia-Lobón et al., 2010a, and references therein).
3.1 Iberian mainland
The compilation of the Top of the Paleozoic and Base of the Cenozoic stratigraphic markers for the Spanish sedimentary basins was made by the Instituto Geológico y Minero de España (IGME), complemented by a significant amount of subsurface information from the Hydrocarbons Service of the former Ministry of Industry, Tourism, and Commerce (ATH) as well as studies by the Empresa Nacional de Residuos Radioactivos SA (ENRESA), universities, associations, working groups, and research and development projects stemming from the ALGECO2 project (García-Lobón et al., 2010a). The objective of the project was the selection and characterization of areas and structures favorable for CO2 storage in Spain. The locations of the data points and seismic profiles included in the database are shown in Figs. 3 and 4.
For that purpose, the analysis and geoscientific information included a review of about 50 000 km of seismic lines and 400 exploration wells, in addition to the available regional cross sections and subsurface maps largely derived from historical petroleum exploration. For further details on the digital geological reference mapping, data from the oil exploration wells, seismic data, reference surfaces, and other information, the reader is referred to the work of García-Lobón et al. (2010a) and the references therein.
3.1.1 Ebro Basin
Data on the Ebro Basin were extracted mainly from regional and geological studies at various scales (García-Lobón et al., 2010b), including the MAGNA projects, and from geological maps at scales of 1:400 000 and 1:50 000. Additionally, geophysical information and deep-drilling data were considered together with information gathered in the Documents on the Subsurface Geology of Spain (IGME 1992) and the Subsurface Geology of Spain (ITGE) (seven documents). IGME's geophysical information includes all drilling documentation (composite and master logs) from hydrocarbon exploration in the Technical Archive of the Ministry of Industry (which contains information on mining boreholes with depths of up to 2500 m and oil boreholes with depths of up to ca. 5400 m) as well as the deepest boreholes from the Database of the Ebro Hydrographic Confederation with depths of up to 1658 m, which were reanalyzed in this work. In addition, over 40 PhD and MSc-level theses, together with more than 100 research articles, were re-examined, with a particular focus on those completing regional structural analyses and geological cross sections. This approach was especially relevant in regions with limited well data such as the shoulder areas of the Ebro Basin. Other data sources such as the databases of CHE (Ebro Hydrographical Confederation), Gessal, Repsol, Enresa, and Union Texas were also consulted, providing relevant data for updating previously published maps. Subsequently, the interpretation of seismic lines and wells, in accordance with these cross sections, led to the generation of maps of the depth contours (isobaths) presented in this study.
As pointed out by García-Lobón et al. (2010b), the isobath map of the Base of the Cenozoic is not consistently defined due to discrepancies arising from both multiple unconformities in syntectonic depositional sequences and potential blind thrusts along the boundaries of the basin. This makes the correlation between subsurface data and surface outcrops particularly difficult. For a complete list of the references and technical details, the reader is referred to the work of García-Lobón et al. (2010b).
3.1.2 Duero and Basque–Cantabrian basins
Isobath maps for these two basins were compiled based on information from 239 hydrocarbon exploration wells reaching depths between 500 and > 2500 m. The available borehole data allowed the identification of multiple stratigraphic tops in the Mesozoic sequence. Additionally, reference points from regional cross sections and isobath data from previous work were used to create new isobath maps. Using these same datasets, contour lines were generated and later edited to test the presence (and effect) of multiple geological structures based on the existing geological and structural data for the Basque–Cantabrian and Duero basins. This process allowed the creation of isobath maps for the Base of the Cenozoic and the Top of the Paleozoic, adjusting them to the main faults and structures identified in regional geological maps. For the eastern and northeastern parts of the Duero Basin, the calculated isobaths include subsurface information from oil exploration wells. For a complete reference list and additional technical details, the reader is referred to García-Lobón et al. (2010c).
3.1.3 Tagus and Almazán basins
For the creation of isobath maps in the Iberian Range and the Tagus and Almazán basins, two different approaches were developed based on the available data on these two Tertiary basins, their reliability, and their spatial distribution. Thus, the data presented in this study for these areas were mainly constrained by geological cross sections, as there was a scarcity of subsurface data, and from available reflection seismic profiles and borehole information. An effort was made to ensure the consistency of all final products at the regional scale considered in this work.
Geological cross sections were primarily sourced from pre-existing or new cross sections, with the latter predominantly derived from boreholes and pre-existing isobath maps. Where there was a scarcity of data and structural information, e.g., in the Iberian Range, an effort was made to increase the data density from the surface cartography, boreholes, and regional cross sections. For a complete reference list and additional technical details, the reader is referred to García-Lobón et al. (2010d). Additional data can be found in de Vicente and Muñoz Martín (2013).
3.1.4 Guadalquivir Basin
Stratigraphic information from the Guadalquivir Basin was gathered after reassessing the surface and subsurface exploration data. The primary sources of this information consisted of reports and databases from IGME. This dataset was further enriched by substantial subsurface documents and data acquired from the Hydrocarbons Service of the former Ministry of Industry, Tourism, and Commerce, together with contributions from various public entities, universities, associations, and working groups participating in research and development projects, including the ALGECO2 project (García-Lobón et al., 2010a).
In addition to these data, regional geological cross sections were compiled using the available geological maps and subsurface data, particularly those where seismic profiles and boreholes exist. Notably, the Guadalquivir Basin has limited seismic grid coverage and borehole control, particularly when one considers the stratigraphic markers of interest to this work. Consequently, the accuracy of these isobath maps is variable across different locations. For a complete reference list and additional technical details, the reader is referred to García-Lobón et al. (2010e).
3.1.5 Lower Tagus Basin
The depth to the Base of the Cenozoic in this area was obtained using seismic reflection data calibrated by exploration boreholes, by modeling land gravimetric data, and via geological outcrop data. Seismic reflection data comprise legacy surveys acquired with distinct geometries and sources for the oil industry from the mid-1950s until 1982. These surveys were reprocessed and reinterpreted in Carvalho et al. (2005, 2017). Gravimetric data from the national Portuguese grid were also used to increase the geographical coverage of the existing seismic data, which was limited to the western part and central part of the Lower Tagus Basin (Carvalho et al., 2017).
Seismic reflection data were used, where available, to determine the depth of the Base of the Mesozoic, which was only reached by a few exploration wells. In contrast, aeromagnetic data were used across the entire basin (Carvalho et al., 2014). These datasets do not allow a precise estimate of the depths of pre-Mesozoic rocks; in fact, the acoustic basement in seismic data is often coincident with the Top Dagorda salt member (Hettangian) (e.g., Carvalho et al., 2005; Alves et al., 2003b) and, occasionally, the Triassic Silves Formation. In the areas where the basement was estimated via magnetic data models, errors are also expected due to the non-uniqueness of potential field methods. Despite the calibration of seismic and magnetic data with some sparse boreholes, depth errors in the tops of the pre-Mesozoic rocks may reach a few hundred meters in some areas.
3.2 Offshore from Iberia
3.2.1 West Iberian Atlantic margin
The interpreted 2D multi-client seismic reflection data correspond to a reprocessed seismic grid acquired from TGS during the PDT00 and PD000 cruises and from the IAM seismic survey profiles (IAM-4, IAM-5, IAM-8, IAM-9, IAM-10, IAM-11, T1, T2, GB1, GB2, and GB3). Details on the acquisition parameters and processing data for the two cruises can be found in Alves (2024), Banda et al. (1995), and Torne et al. (1995, 2018), respectively. Figures 3 and 4 show the locations of the seismic profiles included in the database.
The interpretation of the Base of the Cenozoic and Top of the Paleozoic is based on regional (2D) seismic data together with unpublished outcrop and well data (Figs. 5 and 6). The new data are of good enough quality for interpreters to identify a Top Basement stratigraphic marker with confidence, though the real morphology of such a basal horizon is somehow masked by the poor resolution of the data at the depth of occurrence. Nevertheless, the Base of the Meso-Cenozoic strata is clear in the new, reprocessed TGS data (see Alves, 2024, for details). In this work, the criteria of Driscoll et al. (1995), Sinclair (1995), Alves et al. (2009), and Soares et al. (2012) were used in the identification of key tectonic events affecting the North Atlantic region. Therefore, the two stratigraphic markers mapped in the available dataset were correlated with borehole and outcrop data acquired from published and unpublished information from the Lusitanian Basin (Atrops and Marques, 1986; Wilson et al., 1989; Hiscott et al., 1990; Alves et al., 2002, 2003a; Dinis et al., 2008), Porto Basin (Alves et al., 2009), Iberia Abyssal Plain (Wilson et al., 1996, 2001; Eddy et al., 2017), and proximal northwestern Iberia (Groupe Galice, 1979; Boillot et al., 1989; Murillas et al., 1990, Tucholke and Sibuet, 2007).
Unpublished information from exploration wells Pe-1, Go-1, 20B-1, 5A-1, and Lu-1 in western Iberia and dredge data published in Alves et al. (2003a) were used locally to corroborate our seismic–stratigraphic interpretations. DSDP Site 398 and ODP sites 637–641, 897–901, and 1065–1070 provide important information used to correlate seismic–stratigraphic units across the study area (Fig. 1b). These data are complemented by information from DSDP Site 120 and IODP sites U1385, U1391, and U1588, which recently drilled the southwestern Iberian margin (Hernández-Molina et al., 2013; Hodell et al., 2023) (Fig. 1). All the data were integrated into a Schlumberger Petrel® project so that structural, magnetic, and seismic–stratigraphic data could be analyzed together.
3.2.2 Alentejo Basin (continental shelf)
The Top of the Paleozoic Basement and the Base of the Cenozoic were gathered from the interpretation of the Geophysical Service Incorporated (GSI) seismic reflection profiles acquired in 1984 (Carvalho, 1995) and were calibrated by the available well data provided by the Direção de Serviços de Estratégia e Fomento dos Recursos Geológicos (http://www.dgeg.pt), geological outcrop information obtained from seafloor dredges, and the multi-channel seismic reflection data of Alves et al. (2003a). The seismic reflection data interpretation was assisted with gravimetric data (free air anomaly data also collected along the GSI profiles) and aeromagnetic data acquired in 1969 by Fairey Surveys Limited. Descriptions of the seismic and potential field datasets can be found in Carvalho (1995). The locations of the seismic lines included in the database are shown in Figs. 3 and 4.
Potential field data allowed the identification and separation of basement highs or lows (pre-Mesozoic units) from salt and igneous structures. The structural interpretation obtained from seismic data was also compared with derivative maps of the gravimetric and magnetic anomaly maps, such as analytical continuations to several altitudes, horizontal derivatives, vertical derivatives, and shaded reliefs. Seismic interpretation was used afterwards as a starting point for joint 2.5D forward modeling of magnetic and gravimetric data using freeware software (Webring, 1985). The base of the Mesozoic horizon was further corroborated by 3D gravimetric modeling using the software of Broome (1992), which uses the method of Plouff (1976).
3.2.3 Algarve Basin
The geophysical data used to define the Top of the Paleozoic Basement and the Base of the Cenozoic consist of a high-resolution marine gravity survey and a 2D regional seismic reflection survey from TGS (Alves, 2024) and represent a total coverage (58 lines; 5820 km) of 17 890 km2, extending from the shelf to the deep part of the Algarve Basin (Fig. 2). The gravity data provided by TGS have been used to further constrain the top-of-basement data in areas where seismic data coverage was poor or lacked resolution. Well data provided by Direção de Serviços de Estratégia e Fomento dos Recursos Geológicos (DGEG) and from Lanaja et al. (1987) were used as constraints.
The seismic–stratigraphic framework was simplified and divided into four seismic–stratigraphic units based on their seismic character and well-to-seismic calibration (Matias et al., 2007), spanning the Paleozoic basement to Late Cenozoic sediments. Mapping of the top of the basement was aided by the results from gravity enhancement maps and inversion (Matias et al., 2007). A time-to-depth conversion of the interpreted horizons was performed using a layer-cake approach as detailed in Matias et al. (2007). The locations of the seismic lines included in the database are shown in Figs. 3 and 4.
3.2.4 Western Mediterranean
Data on the depth to the acoustic basement for the Western Mediterranean region have been downloaded from SEOANE (Sea Scientific Open Data Publication; Bellucci et al., 2021, https://doi.org/10.17882/80128). These data derive from the compilation, reprocessing, and, in some cases, digitalization of the available industry and academia multichannel seismic profiles and well log suites. All these data have been acquired since the 1960s and cover the westernmost Mediterranean Neogene basins, i.e., the Valencia Trough and the Algerian and Alboran basins. As detailed in Bellucci et al. (2021), the interpretation of the top of the acoustic basement extends across all of the geomorphological domains, ranging from the shelf to the deep basin (Fig. 2). This is identified as the boundary separating the chaotic seismic facies of the substratum from the stratified seismic facies of the sedimentary column. In the Alboran and Valencia Trough basins, the top of the acoustic basement coincides either with the Base of the Cenozoic or the so-called Oligocene unconformity, as documented in studies such as Do Couto et al. (2014), Pellen et al. (2016), and Etheve et al. (2016) (Figs. 3 and 4). For more detailed information, the reader is referred to the work of Bellucci et al. (2021) and the references therein.
As the original data file presents different information relative to the age of the acoustic basement, we split the original file into three subfiles corresponding to the Valencia Trough and Alboran Basin regions (Base of the Cenozoic or the Oligocene unconformity) and the Algerian basin (boundary between chaotic and stratified seismic facies). For more details, please refer to Table S1. The locations of the seismic lines included in the database are shown in Figs. 3 and 4.
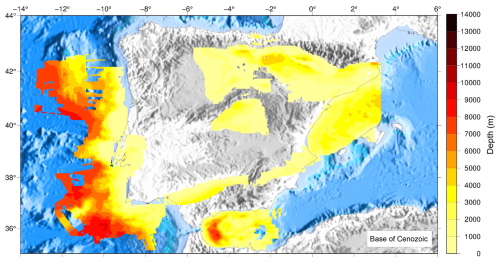
Figure 6Offshore and onshore depths to the Base of the Cenozoic. The main references from which data have been gathered are shown in Table S1. Additional references can also be found throughout the text. Data from the Western Mediterranean have been taken from Bellucci et al. (2021) and the references therein).
Sediments are widely recognized for their capacity to act as thermal insulators, thereby attenuating the vertical transfer of heat from deeper geological strata. However, the thermal regime at a given site is governed by a complex interplay of factors, including surface heat flow, the thermal conductivity of both sedimentary deposits and underlying lithologies, and the radiogenic heat production of these materials. Collectively, these parameters exert a significant influence on the thermal gradient and subsurface temperature distribution. To assess the influence of sediments on the thermal field, we systematically calculated the depth variability of the 150 °C isotherm under different conditions. These conditions included variable surface heat flow values, sediment thickness, thermal conductivity variations, and a fixed radiogenic heat production rate. Temperature profiles have been computed in the one-dimensional field, considering surface heat flow values ranging from 40 to 120 mW m−2 (e.g., Fernàndez et al., 1998) and the International Heat Flow Commission (Fuchs et al., 2023), a range that is representative of the mean and maximum heat flow values recorded in the Iberian Peninsula. We have also considered standard values of radiogenic heat production for sediments and crust at, respectively, 1 µW m−3 and 3 µW m−3, where z is the depth in kilometers. Other parameters considered were a constant crustal thermal conductivity of 3.1 W m−1 K−1 and a constant mean surface temperature of 15 °C.
Figure 7 shows the variations in the depth of the 150 °C isotherm in relation to sediment thickness, surface heat flow, and four different thermal conductivity values for the sediments (Fig. 7a–d). The calculated trend indicates that, for a constant measured surface heat flow and constant thermal conductivity, the isotherm depth decreases as sediment thickness increases until it reaches a critical threshold depth. Beyond this depth value, the isotherm becomes stable. Our results also show that, as expected, for a given thermal conductivity, an increase in surface heat flow leads to a relative shallowing of the isotherm, while a decrease in surface heat flow produces the opposite effect. Notably, an increase in thermal conductivity is associated with greater deepening of the 150 °C isotherm for the same heat flow and sediment thickness values. The crustal thermal conductivity value of 3.1 W m−1 K−1, which is within the upper range of typical values, was selected to provide a broader range for assessing the influence of sediment thermal conductivity on subsurface thermal gradients. Importantly, this threshold depth is independent of the crustal conductivity. However, lower crustal conductivity values would result in a shallower depth of the 150 °C isotherm in regions where the sediment thicknesses are below the threshold depth.
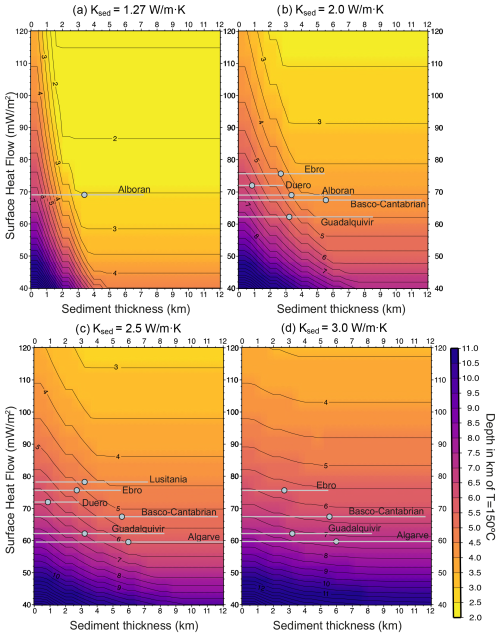
Figure 7Depth to the 150 °C isotherm as a function of sediment thickness and surface heat flow, assuming four different thermal conductivities for sediments: (a) 1.27 W m−1 K−1, (b) 2.0 W m−1 K−1, (c) 2.5 W m−1 K−1, and (d) 3.0 W m−1 K−1. Crustal conductivity is kept constant at 3.1 W m−1 K−1. Radiogenic heat production is 1 µW m−3 and 3 µW m−3 for the sediments and crust, respectively. Each basin is shown by a horizontal grey line indicating the average surface heat flow value and average sediment thickness at the calculated site (grey dot). The minimum-to-maximum sediment thickness in the basin is indicated with the grey line.
Comparing Fig. 7a (showing the minima in conductivity values) and Fig. 7d (showing the maxima in conductivity values), one observes that, for a constant heat flow value, say 70 mW m−2, the 150 °C isotherm is significantly deepened as conductivity increases. Additionally, we observe that changes in sediment thickness wield more influence at lower thermal conductivity values. For instance, if one assumes a thermal conductivity of 1.27 W m−1 K−1 and a surface heat flow of 70 mW m−2, the isotherm depth is reduced until the sediment thickness reaches approximately 3.5 km. Beyond this point, the isotherm stabilizes at a constant depth (Fig. 7a). Conversely, when assuming higher conductivity values, e.g., approaching the conductivity of the crust (e.g., 3.0 W m−1 K−1), the effect of any increase in sediment thickness is notably reduced (Fig. 7d).
Based on the findings presented in Fig. 7, we have computed an initial estimate of the depth of the 150 °C isotherm for the onshore basins presented in this study, where surface heat flow is known, following the published values of Fernàndez et al. (1998) and as compiled by the International Heat Flow Commission (Fuchs et al., 2023). As for thermal conductivities, we have taken the average thermal conductivity values in Torne et al. (2023). For instance, considering the Ebro Basin and the Guadalquivir Basin as end-members for relatively high and low average heat flow values of 75 and 62 mW m−2, we observe that, for a constant conductivity of 2.0 W m−1, K−1, the depth of the isotherm in the Ebro Basin is considerably shallower (ranging from 6 km with no sediments to 3.75 km for a sediment thickness of 3.75 km) compared to the Guadalquivir Basin. In the Guadalquivir Basin, for equivalent sediment thickness values, the isotherm depth fluctuates between 7.75 and 4.50 km, respectively. This difference is attributed to the higher average heat flow values observed in the Ebro Basin compared to the Guadalquivir Basin. Additionally, increases in thermal conductivity led to an increase in the isotherm depth, shifting to 6 and 4.6 km in the Ebro Basin and to 7.75 and 5.70 km in the Guadalquivir Basin. However, it is important to stress that in both basins the impact of increasing conductivity results in the isotherm being situated at a greater depth, with it being less sensitive to variations in sediment thickness. This is clear when one compares Fig. 7a and Fig. 7d. Such an observation generally confirms the impact of sediment thickness and thermal conductivity on the spatial distribution of the 150 °C isotherm, with a heightened significance in areas characterized by lower heat flow values and low thermal conductivities.
SedDARE-IB is available in the Spanish National Research Council's DIGITAL.CSIC repository (https://doi.org/10.20350/digitalCSIC/16277, Torne et al., 2024). A detailed list of the datasets can be found in Table S1.
Analyzing sediments is crucial for understanding a region's geological history, subsurface structure, and potential resources. The thickness and properties of sediment layers play a crucial role in subsurface exploration, providing valuable insights for building more accurate geophysical models. Additionally, studying sediment properties is essential for assessing and mitigating geohazards as well as informing infrastructure planning and engineering. In summary, curating sediment data is essential for advancing knowledge and sustainable management of resources, protecting the environment, and making informed decisions across various scientific, economic, and social domains.
The SedDARE-IB database has been created to facilitate the reuse and preservation of existing data by future generations of geoscientists. It achieves this by hosting sediment data in the online institutional repository DIGITAL.CSIC. The database accomplishes the international mandates of open-access data and findable, accessible, interoperable, and reusable (FAIR) principles of data management. It is a result of close collaboration between Portuguese and Spanish research teams, aiming to make available sediment data on the Iberian Peninsula easily accessible. Presently, SedDARE-IB contains 29 datasets of isobath maps depicting the Top of the Basement and the Base of the Cenozoic of the Iberian Peninsula and its surrounding margins.
As an example of the use of the dataset, we conducted a systematic thermal analysis examining the influence of the sediment thickness on the depth location of the 150 °C isotherm. The obtained results indicate a complex relationship between isotherm depth and sediment thickness. While an increase in sediment thickness initially results in higher temperatures at relatively shallow depths, there is a critical threshold value in sediment thickness beyond which the 150 °C isotherm stabilizes at a constant depth. Moreover, an increase in thermal conductivity correlates with a deepening of the 150 °C isotherm, while an increase in heat flow leads to the opposite effect.
When comparing the Ebro and Guadalquivir basins as end-members of basins with relatively high and low average heat flow values (75 and 62 mW m−2, respectively), one records differences in the depth of the 150 °C isotherm between 1 and 1.5 km. The trend is one of a deeper 150 °C isotherm whenever a relatively higher thermal conductivity is recorded.
The spatial distribution of the 150 °C isotherm is notably affected by variations in thermal conductivity, with a heightened significance in those basins characterized by lower heat flow values. Here, the impact of thermal conductivity is particularly pronounced, influencing the isotherm depth to a greater extent than variations in sediment thickness.
Table S1: a detailed list of the information is included in the database. The supplement related to this article is available online at https://doi.org/10.5194/essd-17-1275-2025-supplement.
MT, IJM, AMGG, TA, JC, and ER conceived the idea. MT, CA, HH, and AB worked on the data curation and collation of the datasets of the Iberian alpine foreland basins (JLGL, CA, MT, and AB) and the Western Mediterranean basins (HH and MT). TS, JC, ER, and HM worked on the data curation and collation of the datasets of the deep offshore Atlantic Margin, Alentejo, and Algarve basins. IJM and MT performed the thermal calculations. JC, JV, TA, HH, and ER prepared the geological section of the paper. MT and AB managed the uploading of the datasets into DIGITAL.CSIC. MT prepared the original manuscript. All the authors contributed to the review and editing. TA handled the English editing of the manuscript.
The contact author has declared that none of the authors has any competing interests.
Data are published without any warranty, whether express or implied. The user assumes all risk arising from his/her use of the data. The data are intended to be of research quality, but it is possible that the data themselves contain errors. It is the sole responsibility of the user to assess whether the data are appropriate for his/her use and to interpret them accordingly. The authors invite users to ask questions and report problems.
Publisher’s note: Copernicus Publications remains neutral with regard to jurisdictional claims made in the text, published maps, institutional affiliations, or any other geographical representation in this paper. While Copernicus Publications makes every effort to include appropriate place names, the final responsibility lies with the authors.
This work has been performed using the facilities of the Laboratory of Geodynamic Modeling at Geo3BCN-CSIC. Figures 1 to 7 were totally or partly drawn using the GMT software (Wessel et al., 2019). The authors thank three anonymous reviewers for their comments and suggestions that improved the previous version of this paper. TGS is acknowledged for the provision of seismic data on the entire Atlantic Margin of Iberia.
This research has been partly supported by projects funded by the Spanish Government: GeoCAM (grant no. PID2022-139943NB-I00) and GEOADRIA (grant no. PID2022-139943NB-I00). The onshore Iberia datasets were compiled under the umbrella of the ALGECO2 project (IGME-CSIC). IAM profiles were acquired through the support of the EU project (grant no. JOU-CT92-0177). The Lower Tagus Basin and Alentejo datasets were prepared by Joao Carvalho in the framework of an academic thesis and updated during projects SISMOD/LISMOT-Finite Seismic Source Modeling by Joint Inversion of Seismic and Geodesic Data and Strong Ground motion in the Lower Tagus Valley (grant no. PTDC/CTE-GIN/82704/2006) and NEFITAG-Strong Ground Motion and Near Field Effects in the Lower Tagus Region (grant no. PTDC/CTE-GIX/102245/2008), financed by the Portuguese Foundation for Science and Technology. Angela María Gómez-García received grant no. FJC2021-047434-I funded by MICIU/AEI/10.13039/501100011033 and by the European Union NextGenerationEU/PRTR.
The article processing charges for this open-access publication were covered by the CSIC Open Access Publication Support Initiative through its Unit of Information Resources for Research (URICI).
This paper was edited by Sabine Schmidt and reviewed by two anonymous referees.
Alonso-Zarza, A. M., Clavo, J. P., Silva, P. G., and Torres, T.: Cuenca del Tajo, in: Geología de España, edited by: Vera, J. A., SGE-IGME, 556–561, ISBN 84-7840-546-1, 2004.
Alves, T. M.: Networks of geometrically coherent faults accommodate Alpine tectonic inversion offshore southwestern Iberia, Solid Earth, 15, 39–62, https://doi.org/10.5194/se-15-39-2024, 2024.
Alves, T. M. and Cunha, T. A.:. A phase of transient subsidence, sediment bypass and deposition of regressive–transgressive cycles during the breakup of Iberia and Newfoundland, Earth Planet. Sc. Lett., 484, 168–183, https://doi.org/10.1016/j.epsl.2017.11.054, 2018.
Alves, T. M., Gawthorpe, R. L., Hunt, D. W., and Monteiro, J. H.: Cenozoic tectono-sedimentary evolution of the western Iberian margin, Mar. Geol., 195, 75–108, 2003a.
Alves, T. M., Manuppella, G., Gawthorpe, R. L., Hunt, D. H., and Monteiro, J. H.: The depositional evolution of diapir- and fault-bounded rift basins: Examples from the Lusitanian Basin of west Iberia, Sediment. Geol., 162, 273–303, https://doi/10.1016/S0037-0738(03)00155-6, 2003b.
Alves, T. M., Moita, C., Cunha, T., Ullnaess, M., Myklebust, R., Monteiro, J. H., and Manuppella, G.: Diachronous evolution of Late Jurassic–Cretaceous continental rifting in the northeast Atlantic (west Iberian margin), Tectonics, 28, TC4003, https://doi.org/10.1029/2008TC002337, 2009.
Anadón, P. and Roca, E.: Geological Setting of the Tertiary basins of Northeast Spain, in: Tertiary Basins of Spain: The Stratigraphic Record of Crustal Kinematics, edited by: Friend, P. F. and Dabrio, C. J., Cambridge Univ. Press, ISBN 0521461715, 1996.
Antón, L., Rodés, A., de Vicente, G., Pallàs, R., García-Castellanos, D., Stuart, F. M., and Bourlès, D.: Quantification of fluvial incision in the Duero Basin (NW Iberia) from longitudinal profile analysis and terrestrial cosmogenic nuclide concentrations, Geomorphology, 165, 50–61, 2012.
Antón, L., Muñoz-Martín, A., and de Vicente, G.: Quantifying the erosional impact of a continental-scale drainage capture in the Duero Basin, northwest Iberia, Quaternary Res., 91, 457–471, 2019.
Arche, A., Evans, G., and Clavell, E.: Some considerations on the initiation of the present SE Ebro River drainage system: Post-or pre-Messinian?, J. Iber. Geol., 36, 73–85, 2010.
Atrops, F. and Marques, B.: Mise en évidence de la zone à platynota (Kimméridgien inférieur) dans le massif du Montejunto (Portugal); consequences stratigraphiques et paléontologiques, Geobios, 19, 537–547, https://doi.org/10.1016/S0016-6995(86)80053-5, 1986.
Banda, E., Torne, M., and The Iberian Atlantic Margins Group: Iberian Atlantic Margins Group investigates deep structure of ocean margins, Eos, 76, 25–29, https://doi.org/10.1029/EO076i003p00025, 1995.
Barnolas, A., Civis, J., Larrasoaña, J. L., Pujalte, V., Scmitz, B., García-Castellanos, D., Sierro, F. J., and van den Berg, B. C. J. C.: Alpine Foreland Basins, in: The Geology of Iberia: A Geodynamic Approach, Regional Geology Reviews, edited by: Quesada, C. and Oliveira, J. T., Springer, Cham., https://doi.org/10.1007/978-3-030-11190-8_2, 2019.
Bellucci, M., Pellen, R., Leroux, E., Bache, F., Garcia, M., Do Couto, D., Raad, F., Blondel, S., Rabineau, M., Gorini, C., Moulin, M., Maillard, A., Lofi, J., Del ben, A., Camerlenghi, A., Poort, J., and Aslanian, D.: A comprehensive and updated compilation of the seismic stratigraphy markers in the Western Mediterranean Sea, SEAONE [data set], https://doi.org/10.17882/80128, 2021.
Berástegui, X., Banks, C. J., Puig, C., Taberner, C., Waltham, D., and Fernàndez, M.: Lateral diapiric emplacement of Triassic evaporites at the southern margin of the Guadalquivir Basin, Spain, Geol. Soc. Lond. Spec. Publ., 134, 49–68, https://doi.org/10.1144/GSL.SP.1998.134.01.04, 1998.
Boillot, G., Girardeau, J., and Winterer, E. L.: Rifting processes of the west Galicia margin, Spain, in: Extensional Tectonics and Stratigraphy of the North Atlantic Margins, edited by: Tankard, A. J. and Balkwill, H. R., AAPG Memoir., 40, 363–377, 1989.
Borges, J. F., Fitas, A. J., Bezzeghoud, M., and Teves-Costa, P.: Seismotectonics of Portugal and its adjacent Atlantic area, Tectonophysics, 337, 373–387, 2001.
Broome, J.: An IBM-compatible program for interactive three-dimensional gravity modeling, Comput. Geosci., 18, 337–348, https://doi.org/10.1016/0098-3004(92)90084-5, 1992.
Cabral, J., Moniz, C., Ribeiro, P., Terrinha, P., and Matias, L.. Analysis of seismic reflection data as a tool for the seismotectonic assessment of a low activity intraplate basin- the Lower Tagus Valley (Portugal), J. Seismol., 7, 431–447, 2003.
Cámara, P.: Salt and strike-slip tectonics as main drivers in the structural evolution of the Basque-Cantabrian Basin, Spain, in: Permo-Triassic Salt Provinces of Europe, North Africa and the Atlantic Margins, edited by: Soto, J. I., Flinch, J. F., and Tari, G., Elsevier, 371–393, https://doi.org/10.1016/B978-0-12-809417-4.00018-5, 2017.
Carvalho, J.: Study of the South Portuguese Zone and Adjacent Atlantic Margin using Geophysical Data, Master thesis, University of Lisbon, 154 pp., https://repositorio.lneg.pt/bitstream/10400.9/4293/1/Tese-Mestrado-JoaoCarvalho.pdf, (last access: 23 March 2025), 1995.
Carvalho, J., Matias, H., Torres, L., Pereira, R., Manupella, G., and Mendes-Victor, L.: The structural and sedimentary evolution of the Arruda and Lower Tagus sub-basins, Portugal, Mar. Petrol. Geol., 22, 427–453, https://doi.org/10.1016/j.marpetgeo.2004.11.004, 2005.
Carvalho, J., Rabeh, T., Dias, R., Dias, R., Pinto, C. C., Oliveira, T., Cunha, T., and Borges, J.: Tectonic and Neotectonic Implications of a New Basement Map of the Lower Tagus Valley, Portugal, Tectonophysics, 617, 88–100, https://doi.org/10.1016/j.tecto.2014.01.017, 2014.
Carvalho, J., Pinto, C., Rabeh, T., Dias, R., Torres, L., Borges, J., Torres, R., and Duarte, H.: Tectonic Evolution of an Intraplate Basin: The Lower Tagus Cenozoic Basin, Portugal, Basin Res., 29, 636–557, https://doi.org/10.1111/bre.12193, 2017.
Casas-Sainz, A. M. and Faccenna, C.: Tertiary compressional deformation of the Iberian plate, Terra Nova, 13, 281–288, https://doi.org/10.1046/j.1365-3121.2001.00355.x, 2001.
Casas-Sainz, A. M. and de Vicente, G.: On the tectonic origin of Iberian topography, Tectonophysics, 474, 214–235, https://doi.org/10.1016/j.tecto.2009.01.030, 2009.
Casini, G., Vergés, J., Drzewiecki, P., Ford, M., Cruset, D., Wright, W., and Hunt, D.: Reconstructing the Iberian Salt-Bearing Rifted Margin of the Southern Pyrenees: Insights from the Organyà Basin, Tectonics, 42, e2022TC007715, https://doi.org/10.1029/2022TC007715, 2023.
Civis, J. and Vera, J. A.: Cuencas cenozoicas, in: Geología de España, edited by: Vera, J. A., SGE-IGME, 531–533, ISBN 84-7840-546-1, 2004.
Coppier, G. and Mougenot, D.: Stratigraphie sismique et evolution geologique des formations neogenes et quaternaires de la plate-forme continentale portugaise au sud de Lisbonne, B. Soc. Geol. Fr., S7-XXIV, 421–431, https://doi.org/10.2113/gssgfbull.S7-XXIV.3.421, 1982.
Costa, E., Garcés, M., López-Blanco, M., Beamud, E., Gómez-Paccard, M., and Larrasoaña, J. C.: Closing and continentalization of the South Pyrenean foreland basin (NE Spain): magnetochronological constraints, Basin Res., 22, 904–917, https://doi.org/10.1111/j.1365-2117.2009.00452.x, 2009.
Cunha, P. P.: Cenozoic Basins of Western Iberia: Mondego, Lower Tejo and Alvalade Basins, in: The Geology of Iberia: A Geodynamic Approach, edited by: Quesada, C. and Oliveira, J. T., 4, 105–130, https://doi.org/10.1007/978-3-030-11190-8_4, 2019.
Dercourt, J., Zonenshain, L. P., Ricou, L-E., Kazmin, V. G., Le Pichon, X., Knipper, A. L., Grandjacquet, C., Sbortshikov, I. M., Geyssant, J., Lepvrier, C., Pechersky, D. H., Boulin, J., Sibuet, J.-C., Savostin, L. A., Sorokhtin, O., Westphal, M., Bazhenov, M. L., Lauer, J. P., and Biju-Duval, B.: Geological evolution of the tethys belt from the atlantic to the pamirs since the LIAS, Tectonophysics, 123, 241–315, https://doi.org/10.1016/0040-1951(86)90199-X, 1986.
de Vicente, G. and Muñoz-Martín, A.: The Madrid Basin and the Central System: A tectonostratigraphic analysis from 2D seismic lines, Tectonophysics, 602, 259–285, https://https://doi.org/10.1016/j.tecto.2012.04.003, 2013.
de Vicente, G., Muñoz-Martín, A., Guimerà, J., Vegas, R., and Cloetingh, S.: Estructura Alpina del Antepaís Ibérico, in: Geología de España, edited by: Vera, J. A., SGE-IGME, 589–534, ISBN 84-7840-546-1, 2004.
de Vicente, G., Cloetingh, S., Van Wees, J. D., and Cunha, P. P.: Tectonic classification of Cenozoic Iberian foreland basins, Tectonophysics, 502, 38–61, 2011.
Dias, J. M. and Nittrouer, C. A.: Continental shelf sediments of northern Portugal, Cont. Shelf Res., 3, 147–165, https://doi.org/10.1016/0278-4343(84)90004-9, 1984.
Dias, J. M., Jouanneau, M., Gonzalez, R., Araújo, M. J., Drago, T., Garcia, C., Oliveira, A., Rodrigues, A., Vitorino, J., and Weber, O.: Present day sedimentary processes on the northern Iberian shelf, Prog. Oceanogr., 52, 249–259, https://doi.org/10.1016/S0079-6611(02)00009-5, 2002.
Dinis, J. L., Rey, J., Cunha, P. P., and Callapez, P.: Stratigraphy and allogenic controls of the western Portugal Cretaceous: An updated synthesis, Cretaceous Res., 29, 772–780, https://doi.org/10.1016/j.cretres.2008.05.027, 2008.
Do Couto, D., Popescu, S. M., Suc, J. P., Melinte-Dobrinescu, M. C., Barhoun, N., Gorini, C., and Auxietre, J. L.: Lago Mare and the Messinian salinity crisis: evidence from the Alboran Sea (S. Spain), Mar. Petrol. Geol., 52, 57–76, https://tel.archives-ouvertes.fr/tel-01148777, 2014.
Driscoll, N. W., Hogg, J. R., Christie-Blick, N., and Karner, G. D.: Extensional tectonics in the Jeanne d'Arc Basin, offshore Newfoundland: Implications for the timing of break-up between Grand Banks and Iberia, in: The Tectonics, Sedimentation and Palaeoceanography of the North Atlantic Region, edited by: Scrutton, R. A., Stoker, M. S., Shimmield, G. B., and Tudhope, A. W., Geol. Soc. Spec. Publ., 90, 1–28, 1995.
Eddy, M. P., Jagoutz, O., and Ibañez-Mejia, M.: Timing of initial seafloor spreading in the Newfoundland-Iberia rift, Geology, 45, 527–530, 2017.
Etheve, N., de Lamotte, D. F., Mohn, G., Martos, R., Roca, E., and Blanpied, C.: Extensional vs contractional Cenozoic deformation in Ibiza (Balearic Promontory, Spain): Integration in the West Mediterranean back-arc setting, Tectonophysics, 682, 35–55, 2016.
Fernàndez, M., Marzan, I., Correia, A., and Ramalho, E.:. Heat flow, heat production, and lithospheric thermal regime in the Iberian Peninsula, Tectonophysics 291, 29–53, https://doi.org/10.1016/S0040-1951(98)00029-8, 1998.
Ford, M. and Vergés, J.: Evolution of a salt-rich transtensional rifted margin, eastern North Pyrenees, France, J. Geol. Soc., 178, jgs2019-157, https://doi.org/10.1144/jgs2019-157, 2001.
Friend, P. F. and Dabrio, C. J. (Eds.): Tertiary Basins of Spain: The Stratigraphic Record of Crustal Kinematics, Cambridge University Press, ISBN 0521461715, 1995.
Fuchs, S., Norden, B., Neumann, F., Kaul, N., Tanaka, A., Kukkonen, I. T., Pascal, C., Christiansen, R., Gola, G., Šafanda, J., Espinoza-Ojeda, O. M., Marzan, I., Rybach, L., Balkan-Pazvantoglu, E., Ramalho, E. C., Dedecek, P., Negrete-Aranda, R., Balling, N., Poort, J., Wang, Y., and Verdoya, M.: Quality-assurance of heat-flow data: The new structure and evaluation scheme of the IHFC Global Heat Flow Database, Tectonophysics, 863, 229976, https://doi.org/10.1016/j.tecto.2023.229976, 2023.
García-Castellanos, D. and Larrasoaña, J. C.: Quantifying the post-tectonic topographic evolution of closed basins: The Ebro basin (northeast Iberia), Geology, 43, 663–666, https://doi.org/10.1130/G36673.1, 2015.
García-Castellanos, D., Fernàndez, M., and Torne, M.: Modeling the evolution of the Guadalquivir foreland basin (southern Spain), Tectonics, 21, 1018, https://doi.org/10.1029/2001TC001339, 2002.
García-Castellanos, D., Vergés, J., Gaspar-Escribano, J., and Cloetingh, S.: Interplay between tectonics, climate, and fluvial transport during the Cenozoic evolution of the Ebro Basin (NE Iberia), J. Geophys. Res., 108, 2347, https://doi.org/10.1029/2002jb002073, 2003.
García Lobón, J. L., Reguera, M. I., Martín León, J., Rey Moral, C., and Berrezueta, E. R.: Plan de selección y caracterización de áreas y estructuras favorables para el almacenamiento geológico de CO2 en España: Resumen ejecutivo, IGME, 76 pp., https://info.igme.es/SidPDF/149000/047/149047_0000001.pdf (last access: 22 March 2025), 2010a.
García-Lobón, J. L., (director del Proyecto), Alcolea, M., Bastante, R., Escalante, S., Hermida, E., Morante, M., Ruíz, G., Pardos, M., Juez, F. J., Muñoz, J. A., Mencos, J., Roca, E., Gratacós, O., Bello, D. A., Bausá, J., Arbués, P., Arche, A., Cardona M. A., Cortés, A. L., Baceta, J. I., Murelaga, X., and Larrasoaña, J. C.: Primera Fase del Plan de Almacenamiento Geológico de CO2 del IGME (Proyecto ALGECO2). Volumen II-1- Cadena Pirenaica y Cuenca del Ebro. Geología. Edición: IGME, MADRID, ESP, 2010, Título de colección: Proyecto: Selección y Caracterización de Áreas y Estructuras Geológicas Susceptibles de Constituir Emplazamientos de Almacenamiento de CO2 (ALGECO2), 47 pp., Código 64046, https://info.igme.es/SidPDF/146000/830/146830_0000001.pdf (last access: 23 March 2025), 2010b.
García-Lobón, J. L. (director del Proyecto), Heredia, N., Gómez, J. A., Molinero, R., León, C., Pineda, A., Delgado, B., Varea, R., Álvarez-Pulgar, J., and Navas, M.: Primera Fase del Plan de Almacenamiento Geológico de CO2 del IGME (Proyecto ALGECO2). Volumen I-1- Cadena Cantábrica y Cuenca del Duero. Geología. Edición: IGME, MADRID, ESP, 2010, Título de colección: Selección y Caracterización de Áreas y Estructuras Geológicas Susceptibles de Constituir Emplazamientos de Almacenamiento de CO2 (ALGECO2), 43 pp., Código 64044, https://info.igme.es/SidPDF/146000/828/146828_0000001.pdf (last access: 22 March 2025), 2010c.
García-Lobón, J. L. (director del Proyecto), López, F. L., Hernaiz, P. P., Mediato, J., Peropadre, C., Monleón, O., García, S., Horno, C., Gómez, M., Iribarren, L., Moreno, F., Huerta, P., Biete, C., Hermida, E., Ruiz, G., and Gómez, J. J.: Primera Fase Del Plan De Almacenamiento Geológico de CO2 del IGME (Proyecto ALGECO2). Volumen III -1- Cadena Ibérica y Cuencas del Tajo y de Almazán, Geología, Título de colección: Selección y Caracterización de Áreas y Estructuras Geológicas Susceptibles de Constituir Emplazamientos de Almacenamiento de CO2 (ALGECO2), 246 pp., Código 64048, https://info.igme.es/SidPDF/146000/832/146832_0000001.pdf (last access: 22 March 2025), 2010d.
García-Lobón, J. L. (director del Proyecto), Marín, C., López, F., Motis, K., Huerta, J., Navarro, J. J., Martínez del Olmo, W., Plaza, J., and Portero J. M.: Primera Fase Del Plan de Almacenamiento Geológico de CO2 del IGME (Proyecto ALGECO2). Volumen IV -1- Cadenas Béticas y Cuenca del Guadalquivir. Geología. Título de colección: Selección y Caracterización de Áreas y Estructuras Geológicas Susceptibles de Constituir Emplazamientos de Almacenamiento de CO2 (ALGECO2), 33 pp., Código 64050, https://info.igme.es/SidPDF/146000/834/146834_0000001.pdf (last access: 22 March 2025), 2010e.
Gibbons, W. and Moreno, T. (Eds.): The Geology of Spain, Geol. Soc. London, 349 pp., ISBN 1-86239-127-0, https://doi.org/10.1144/gospp, 2002.
Grevemeyer, I., Ranero, C. R., Papenberg, C., Sallarés, V., Bartolomé, R., Prada, M., Batista, L., and Neres, M.: The continent-to-ocean transition in the Iberia Abyssal Plain, Geology, 50, 615–619, https://doi.org/10.1130/G49753.1, 2022.
Groupe Galice: The continental margin off Galicia and Portugal: Acoustical stratigraphy, dredge stratigraphy and structural evolution, Initial Rep. Deep Sea, 47, 633–662, 1979.
Hernández-Molina, F. J., Stow, D., Alvarez-Zarikian, C., and Expedition IODP 339 Scientists: IODP Expedition 339 in the Gulf of Cadiz and off West Iberia: decoding the environmental significance of the Mediterranean outflow water and its globa linfluence, Sci. Drilling, 16, 1–11, https://doi.org/10.5194/sd-16-1-2013, 2013.
Herrero, A., Alonso Gavilán, G., and Colmenero, J. R.: Estratigrafía del subsuelo en el sector noroeste de la cuenca del Duero (provincia de León), Rev. Soc. Geol. Esp., 17, 199–216, 2004.
Hiscott, R. N., Wilson, R. C. L., Gradstein, F. M., Pujalte, V., Garcia-Mondejar, J., Boudreau, R. R., and Wishart, H. A.: Comparative stratigraphy and subsidence history of Mesozoic rift basins of North Atlantic, AAPG Bull., 74, 60–76, 1990.
Hodell, D. A., Abrantes, F., Alvarez Zarikian, C. A., and the Expedition 397 Scientists: Expedition 397 Preliminary Report: Iberian Margin Paleoclimate, International Ocean Discovery Program, https://doi.org/10.14379/iodp.pr.397.2023, 2023.
Juarez, M. T., Lowrie, W., Osete, M. L., and Meléndez, G.: Evidence of widespread Cretaceous remagnetization in the Iberian Range and its relation with the rotation of Iberia, Earth Planet. Sc. Lett., 160, 729–743, 1998.
Krijgsman, W., Garcés, M., Langereis, C. G., Daams, R., van Dam, J., van der Meulen, A. J., Agustí, J., and Cabrera, L.: A new chronology for the middle to late Miocene continental record in Spain, Earth Planet. Sc. Lett., 142, 367–380, https://doi.org/10.1016/0012-821X(96)00109-4, 1996.
Lanaja, J. M., Navarro, A., Martínez, J. L., Del Valle, J., Rios, L. M., Plaza, J., Potro, R., and Rodríguez de Pedro, J.: Contribución de la exploración petrolífera al conocimiento de la geología de España, Instituto Geológico y Minero de España, Madrid, 465 pp., ISBN 978-8474743982, 1987.
Lewis, C. J., Vergés, J., and Marzo, M.: High mountains in a zone of extended crust: Insights into the Neogene-Quaternary topographic development of northeastern Iberia, Tectonics, 9, 86–102, 2000.
Lopes, F., Cunha, P. P., and Le Gall, B.: Cenozoic seismic stratigraphy and tectonic evolution of the Algarve margin (offshore Portugal, southwest Iberian Peninsula), Mar. Geol., 231, 1–36, 2006.
Macchiavelli, C., Vergés, J., Schettino, A., Fernàndez, M., Turco, E., Casciello, E., Torne, M., Pierantoni, P. P., and Tunini, L.: A New Southern North Atlantic Isochron Map: Insights into the Drift of the Iberian Plate Since the Late Cretaceous, J. Geophys. Res.-Sol. Ea., 122, 9603–9626, https://doi.org/10.1002/2017JB014769, 2017.
Martínez del Olmo, W.: E3 depositional sequences in the Gulf of Valencia tertiary basin, in: Tertiary basins of Spain: The stratigraphic record of crustal kinematics, edited by: Friend, P. and Dabrio, J., 55–67, Cambridge, Cambridge Univ. Press, https://doi.org/10.1017/CBO9780511524851.012, 1996.
Martins, L. T., Madeira, J., Youbi, N., Munhá, J., Mata, J., and Kerrich, R.: Rift-related magmatism of the Central Atlantic magmatic province in Algarve, Southern Portugal, Lithos, 101, 102–124, 2008.
Matias, H., Barbosa, V., Menezes, P., Sandnes, F., Matias, L., Santos, F., and Reidun, M.: Offshore Algarve Basin, Portugal: Morphology of Paleozoic Basement and Influence on Mesozoic Sedimentation, AAPG Conference & Exibition, Calgary, https://www.searchanddiscovery.com/abstracts/html/2005/annual/abstracts/matias.htm (last access: 23 March 2025), 2005a.
Matias, H., Mohriak, W. U., Menezes, P., Sandnes, F., Barbosa, V. C. F., Matias, L., and Santos, F.: Salt distribution and morphology in the offshore Algarve Basin, in: Petroleum Systems of Divergent Continental Margin Basins, edited by: Post, P. and Rosen, N., Proceedings of the 25th Annual Bob F. Perkins Research Conference, Gulf Coast Section SEPM, Houston, Texas, 4–7 December 2005, 481–509, https://doi.org/10.5724/gcs.05.25.0481, 2005b.
Matias, H., Sandnes, F., Cabrita Da Silva, V., Matias, L., and Santos, F.: Analysis of the Petroleum System in the Western Gulf of Cadiz: Offshore Algarve Basin, AAPG Energy Conference & Exhibition, Athens, Greece, 18–21 November 2007, https://www.searchanddiscovery.com/abstracts/html/2007/athens_conf/abstracts/matias.htm (last access: 23 March 2025), 2007.
Miranda, R., Valadares, V., Terrinha, P., Mata, J., Azevedo, M. R., Gaspar, M., Kullberg, J. C., and Ribeiro, C.: Age constraints on the Late Cretaceous alkaline magmatism on the West Iberian Margin, Cretaceous Res., 30, 575–586, https://doi.org/10.1016/j.cretres.2008.11.002, 2009.
Muñoz-Martín, A.: Evolución geodinámica del borde oriental de la Cuenca del Tajo desde el Oligoceno hasta la actualidad, Tesis doctoral Universidad Complutense de Madrid, 331 pp., https://hdl.handle.net/20.500.14352/6289 (last access: 22 March 2025), 1997.
Muñoz Martín, A. and de Vicente, G.: Origen y relación entre las deformaciones y esfuerzos alpinos de la zona Centro-Oriental de la Península Ibérica, Rev. Soc. Geol. Esp., 11, 1–2, 1998.
Murillas, J., Mougenot, D., Boillot, G., Comas, M. C., Banda, E., and Mauffret, A.: Structure and evolution of the Galicia Interior basin (Atlantic western Iberian continental margin), Tectonophysics, 184, 297–319, https://doi.org/10.1016/0040-1951(90)90445-E, 1990.
Neres, M., Terrinha, P., Noiva, J., Brito, P., Rosa, M., Batista, L., and Ribeiro, C.: New Late Cretaceous and CAMP Magmatic Sources off West Iberia, From High-Resolution Magnetic Surveys on the Continental Shelf, Tectonics, 42, e2022TC007637, https://doi.org/10.1029/2022TC007637, 2023.
Pais, J., Cunha, P. P., Pereira, D., Legoinha, P., Dias, R., Moura, D., Silveira, A. B., Kullberg, J. C., and González-Delgado, J. A.: The Paleogene and Neogene of Western Iberia (Portugal): A Cenozoic Record in the European Atlantic Domain, 1st edn., Springer, 156 pp., https://doi.org/10.1007/978-3-642-22401-0, 2012.
Palain, C.: Une série détritique terrigène, les ”Grès de Silves”: Trias et Lias Inférieur du Portugal, Ser. Geol. Portugal, Memória no. 25, Lisboa, 377 pp., https://docbase.lneg.pt/docs/Memorias/25.pdf (last access: 26 March 2025), 1976.
Pellen, R., Aslanian, D., Rabineau, M., Leroux, E., Gorini, C., Silenziario, C., Blanpied C., and Rubino, J. L.: The Minorca Basin: a buffer zone between the Valencia and Liguro-Provençal Basins (NW Mediterra-nean Sea), Terra Nova, 28, 245–256, 2016.
Pereira, R. and Alves, T. M.: Crustal deformation and submarine canyon incision in a Meso-Cenozoic first-order transfer zone (SW Iberia, North Atlantic Ocean), Tectonophysics, 601, 148–162, https://doi.org/10.1016/j.tecto.2013.05.007, 2013.
Plouff, D.: Gravity and magnetic fields of polygonal prisms and application to magnetic terrain corrections, Geophysics, 41, 727–741, 1976.
Quesada, C. and Oliveira, J. (Eds.): The Geology of Iberia: A Geodynamic Approach. Regional Geology Reviews, Springer, Cham, https://doi.org/10.1007/978-3-030-11295-0, 2019.
Ramos, A., Fernández, O., Torne, M., Sánchez de la Muela, A., Muñoz, J. A., Terrinha, P., Manatschal, G., and Salas, M. C.: Crustal structure of the SW Iberian passive margin: The westernmost remnant of the Ligurian Tethys?, Tectonophysics, 705, 42–62, https://doi.org/10.1016/j.tecto.2017.03.012, 2017.
Ribeiro, A., Kullberg, M. C., Kullberg, J. C., Manupella, G., and Phipps, S.: A review of Alpine tectonics in Portugal: Foreland detachment in basement and cover rocks, Tectonophysics, 184, 357–366, 1990.
Ribeiro, A., Cabral, J., Baptista, R., and Matias, L.: Stress pattern in Portugal mainland and the adjacent Atlantic region, West Iberia, Tectonics, 15, 641–659, 1996.
Rodríguez-Fernández, L. R., López-Olmedo, F., Oliveira, J. T., Medialdea, T., Terrinha, P., Matas, J., Martín-Serrano, A., Martín Parra, L. M., Rubio, F., Marín, C., Montes, M., and Nozal, F.: Mapa Geológico de España y Portugal a escala 1:1.000.000, IGME-LNEG, Madrid-Lisboa, https://info.igme.es/catalogo/resource.aspx?resource=8833 (last access: 23 March 2025), 2015.
Rosenbaum, G., Lister, G. S., and Duboz, C.: Relative motions of Africa, Iberia and Europe during Alpine orogeny, Tectonophysics, 359, 117–129, 2002.
Shanmugam, G. and Moiola, R. J.: Submarine fans: Characteristics, models, classification, and reservoir potential, Earth Sci. Rev., 24, 383–428, https://doi.org/10.1016/0012-8252(88)90064-5, 1998.
Silva, P. G., Roquero, E., López-Recio, M., Huerta, P., and Martínez-Graña, A. M.: Chronology of fluvial terrace sequences for large Atlantic rivers in the Iberian Peninsula (Upper Tagus and Duero drainage basins, Central Spain), Quaternary Sci. Rev., 166, 188–203, 2017.
Sinclair, I. K.: Sequence stratigraphic response to Aptian-Albian rifting in conjugate margin basins: A comparison of the Jeanne d'Arc Basin, offshore Newfoundland, and the Porcupine Basin, offshore Ireland, in: The Tectonics, Sedimentation and Palaeoceanography of the North Atlantic Ocean, edited by: Scrutton, R. A., Stoker, M. S., Shimmield, G. B., and Tudhope, A. W., Geol. Soc. Spec. Publ., 90, 29–49, 1995.
Soares, D. M., Alves, T. M., and Terrinha, P.: The breakup sequence and associated lithospheric breakup surface: Their significance in the context of rifted continental margins (West Iberia and Newfoundland margins, North Atlantic), Earth Planet. Sc. Lett., 355, 311–326, 2012.
Struth, L., Garcia-Castellanos, D., Viaplana-Muzas, M., and Vergés, J.: Drainage network dynamics and knickpoint evolution in the Ebro and Duero basins: From endorheism to exorheism, Geomorphology, 327, 554–571, 2019.
Struth, L., García-Castellanos, D., Rodríguez-Rodríguez, L., Viaplana-Muzas, M., Vergés, J., and Jiménez-Díaz, A.: Topographic, lithospheric and lithologic controls on the transient landscape evolution after the opening of internally-drained basins: Modelling the North Iberian Neogene drainage, B. Soc. Geol. Fr., 192, 45, https://doi.org/10.1051/bsgf/2021036, 2021.
Terrinha, P., Ribeiro, C., Kullberg, J. C., Rocha, R., and Ribeiro, A.: Compression episodes during rifting and faunal isolation in the Algarve Basins, SW Iberia, J. Geol., 110, 101–113, 2002.
Terrinha, P., Matias, L., Vicente, J., Duarte, J., Luís, J., Pinheiro, L., Lourenço, N., Diez, S., Rosas, F., Magalhães, V., Valadares, V., Zitellini, N., Roque, C., Mendez Vítor, L., and MATESPRO Team: Morphotectonics and strain partitioning at the Iberia–Africa plate boundary from multibeam and seismic reflection data, Mar. Geol., 267, 156–174, https://doi.org/10.1016/j.margeo.2009.09.012, 2009.
Terrinha, P., Ribeiro, C., Lourenço, N., and Duarte, J.: The Alpine Orogeny in the West and Southwest Iberia Margins, in: The Geology of Iberia: A Geodynamic Approach, edited by: Quesada, C. and Oliveira, J., Regional Geology Reviews, Springer, Cham, ISBN 978-3-030-10519-8, https://doi.org/10.1007/978-3-030-10519-8_12, 2019.
Torne, M., Banda, E., Sibuet, J. C., Mendes-Victor, L., Senos, M. L., Long, R., and Watts, A. B.: Iberian Atlantic Margins, IAM Project Final Scientific Report (JOU-CT92-0177), https://doi.org/10.20350/digitalCSIC/8566, 1995.
Torne, M., Fernández, M., Vergés, J., Ayala, C., Salas, M. C., Jiménez-Munt, I., Buffet, G., and Díaz, J.: Crust and mantle lithospheric structure of the Iberian Peninsula deduced from potential field modeling and thermal analysis, Tectonophysics, 663, 443, https://doi.org/10.1016/j.tecto.2015.06.003, 2015.
Torne, M., Banda, E., Sibuet, J. C., Mendes-Victor, L., Senos, M. L., Long, R., and Watts, A. B.: Multichannel seismic reflection and wide-angle and refraction data acquisition along the Iberian Atlantic Margins, DIGITAL.CSIC, https://doi.org/10.20350/digitalCSIC/8549, 2018.
Torne, M., Jiménez-Munt, I., Negredo, A. M., Fullea, J., Vergés, J., Marzán, I., Alcalde, J., Gómez-Rivas, E., and de la Noceda, C. G.: Advances in the modeling of the Iberian thermal lithosphere and perspectives on deep geothermal studies, Geotherm. Energy, 11, 3, https://doi.org/10.1186/s40517-023-00246-6, 2023.
Torne, M., Alves, T. M., Jiménez-Munt, I., Carvalho, J., Ayala, C., Ramalho, E. C., Gómez, A., Matias, H., Heida, H., Balaguera, A., García-Lobón, J. L., and Vergés, J.: SedDARE-IB: An open access repository of sediment data for Iberia and its continental margins, DIGITAL.CSIC [data set], https://doi.org/10.20350/digitalCSIC/16277, 2024.
Tucholke, B. E. and Sibuet, J. C.: Leg 210 synthesis: Tectonic, magmatic, and sedimentary evolution of the Newfoundland-Iberia rift, Proc. Ocean Drill. Program Sci. Results, 210, 1–56, 2007.
Ubide, T., Wijbrans, J. R., Galé, C., Arranz, E., Lago, M., and Larrea, P.: Age of the Cretaceous alkaline magmatism in northeast Iberia: Implications for the Alpine cycle in the Pyrenees, Tectonics, 22, 1444–1460, https://doi.org/10.1002/2013TC003511, 2014.
Vera, J. A., Ancochea, A., Calvo Sorando, J. P., Barnolas Cortinas, A., and Bea Carredo, F.: Geología de España, IGME, Madrid, 884 pp., ISBN 978-84-7840-546-6, 2004.
Vergés, J. and Fernàndez, M.: Ranges and basins in the Iberian Peninsula: their contribution to the present topography, Geol. Soc. Lond., Mem., 32, 223–234, 2006.
Vergés, J., Kullberg, J. C., Casas-Sainz, A., de Vicente, G., Vítor Duarte, L., Fernàndez, M., Gómez, J. J., Gómez-Pugnaire, M. T., Jabaloy Sánchez, A., López-Gómez, J., Macchiavelli, C., Martín-Algarra, A., Martín-Chivelet, J., Muñoz, J. A., Quesada, C., Terrinha, P., Torne, M., and Vegas, R.: An Introduction to the Alpine Cycle in Iberia, in: The Geology of Iberia: A Geodynamic Approach. Regional Geology Reviews, Springer, Cham. https://doi.org/10.1007/978-3-030-11295-0_1, 2019.
Vissers, R. L. M. and Meijer, P. Th.: Mesozoic rotation of Iberia: Subduction in the Pyrenees?, Earth Sci. Rev., 110, 93–110, https://doi.org/10.1016/j.earscirev.2011.11.001, 2012.
Watkinson, M. P.: Triassic to Middle Jurassic sequences from the Lusitanian Basin Portugal, and their equivalents in other North Atlantic margin basins, PhD thesis, Open University, Walton Hall, UK, 390 pp., https://doi.org/10.21954/ou.ro.0000fc40, 1989.
Webring, M.: Saki: Semi-automatic Marquardt inversion of gravity and magnetic profiles using Singular Value Decomposition, U. S. Geological Survey Open File-Report, 85–122, https://pubs.usgs.gov/of/1985/0122/report.pdf (last access: 23 March 2025), 1985.
Wessel, P., Luis, J. F., Uieda, L., Scharroo, R., Wobbe, F., Smith, W. H. F., and Tian, D.: The Generic Mapping Tools Version 6, Geochem. Geophy. Geosy., 20, 5556–5564, https://doi.org/10.1029/2019GC008515, 2019.
Wilson, R. C. L., Hiscott, R. N., Willis, M. G., and Gradstein, F. M.: The Lusitanian basin of west-central Portugal: Mesozoic and Tertiary tectonic, stratigraphy, and subsidence history, in: Extensional Tectonics and Stratigraphy of the North Atlantic Margins, edited by: Tankard, A. J. and Balkwill, H. R., AAPG Memoir., 40, 341–361, 1989.
Wilson, R. C. L., Sawyer, D. S., Whitmarsh, R. B., Zerong, J., and Carbonell, J.: Seismic stratigraphy and tectonic history of the Iberia Abyssal Plain, Proc. Ocean Drill. Program Scientific Results, 149, 617–630, 1996.
Wilson, R. C. L., Manatschal, G., and Wise, S.: Rifting along non-volcanic passive margins: Stratigraphic and seismic evidence from the Mesozoic successions of the Alps and western Iberia, in: Non-volcanic Continental Margins: A Comparison of Evidence from Land and Sea, edited by: Wilson, R. C. L., Whitmarsh, R. B., Taylor, B., and Froitzheim, N., Geol. Soc. Spec. Publ., 187, 429–452, 2001.
Zitellini, N., Rovere, M., Terrinha, P., Chierici, F., Matias, L., Mendes, V.L., Corela, C., Ribeiro, A., Córdoba, D., Dañobeitia, J. J., Gràcia, E., and Bartolomé, R.: Neogene through Quaternary tectonic reactivation of SW Iberia passive margin, Pure Appl. Geophys., 161, 565–587, https://doi.org/10.1007/s00024-003-2463-4, 2004.
- Abstract
- Introduction
- Geological outline of the Iberian Peninsula and its adjacent continental margins
- Technical aspects of the data
- Influence of sediment thickness and nature on geothermal potential
- Data availability
- Conclusions
- Author contributions
- Competing interests
- Disclaimer
- Acknowledgements
- Financial support
- Review statement
- References
- Supplement
- Abstract
- Introduction
- Geological outline of the Iberian Peninsula and its adjacent continental margins
- Technical aspects of the data
- Influence of sediment thickness and nature on geothermal potential
- Data availability
- Conclusions
- Author contributions
- Competing interests
- Disclaimer
- Acknowledgements
- Financial support
- Review statement
- References
- Supplement