the Creative Commons Attribution 4.0 License.
the Creative Commons Attribution 4.0 License.
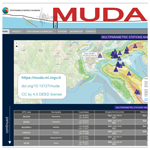
MUDA: dynamic geophysical and geochemical MUltiparametric DAtabase
Marco Massa
Andrea Luca Rizzo
Davide Scafidi
Elisa Ferrari
Sara Lovati
Lucia Luzi
In this paper, the new dynamic geophysical and geochemical MUltiparametric DAtabase (MUDA) is presented. MUDA is a new infrastructure of the National Institute of Geophysics and Volcanology (INGV), published online in December 2023, with the aim of archiving and disseminating multiparametric data collected by multidisciplinary monitoring networks. MUDA is a MySQL relational database with a web interface developed in PHP, aimed at investigating possible correlations between seismic phenomena and variations in endogenous and environmental parameters in quasi real time. At present, MUDA collects data from different types of sensors such as hydrogeochemical probes for physical–chemical parameters in waters, meteorological stations, detectors of air radon concentration, diffusive flux of carbon dioxide (CO2) and seismometers belonging both to the National Seismic Network of INGV and to temporary networks installed in the framework of multidisciplinary research projects. MUDA publishes data daily, updated to the previous day, and offers the chance to view and download multiparametric time series selected for different time periods. The resultant dataset provides broad perspectives in the framework of future high-frequency and continuous multiparametric monitoring as a starting point to identify possible seismic precursors for short-term earthquake forecasting. MUDA can be accessed at https://doi.org/10.13127/muda (Massa et al., 2023).
- Article
(15793 KB) - Full-text XML
- BibTeX
- EndNote
Today, there is an increasing awareness of the role played by the interaction between tectonics and fluid dynamics in triggering seismicity. Yet, the simultaneous monitoring of the relevant key factors is still lacking, even though it could be crucial in recognizing precursory signals. Changes in water chemistry and levels, spring discharges, soil flux regimes (e.g. CO2, CH4, radon), and compositions of dissolved gases in water are well-documented in the literature (e.g. Italiano et al., 2001, 2004; Chiodini et al., 2020; Gori and Barberio, 2022, and references therein), as being pre-, co- and post-seismic modifications as well as markers of the local tectonic stress acting in the crust. These recognized seismic-induced variations in groundwaters and springs have led, in recent years, to scientists giving more attention to the development of multiparametric monitoring, in order to capture the main evidence concerning abrupt changes in chemical and physical parameters recorded before (and also after) energetic seismic events (Rikitake and Hamada, 2003; Cicerone et al., 2009; Martinelli, 2018, and references therein). The ultimate goal is to find systematic signals that can be assumed as possible “precursors” or indicators that a seismogenic process is ongoing (Hubbert and Rubey, 1959; Bräuer et al., 2003; Miller et al., 2004; Chiarabba et al., 2009; Di Luccio et al., 2010; Malagnini et al., 2012; Keranen and Weingarten, 2018; Napolitano et al., 2020; De Matteis et al., 2021; Gabrielli et al., 2022, 2023; Ventura and Di Giovambattista, 2012). At the Italian scale, several studies have described the utility of groundwater and spring parameters and soil gas emissions to catch seismic-related signals as well. However, only a few studies reported continuous, high-frequency monitoring, mainly of groundwater level or hydraulic pressure (De Gregorio et al., 2012; Barberio et al., 2017; De Luca et al., 2018), such as Gori and Barberio (2022) concerning spring monitoring (i.e. temperature, pH, electrical conductivity (EC), dissolved oxygen and carbon dioxide) or D'Alessandro et al. (2020) on soil radon emissions related to seismic activity.
MUDA (geophysical and geochemical MUltiparametric DAtabase), a new dynamic multiparametric database published online in December 2023 on the website at https://muda.mi.ingv.it (last access: 15 October 2024) (Fig. 1), has been developed in such a framework. MUDA is a new infrastructure of the National Institute of Geophysics and Volcanology (INGV, https://www.ingv.it, last access: 15 October 2024) devoted to archiving daily data and distributing quasi-real-time geophysical and geochemical multiparametric data recorded in continuous or near-continuous mode at selected sites installed at the most tectonically active Italian areas (Fig. 2). MUDA was designed in the framework of the INGV Dynamic Planet S2-project (i.e. 3D structure of Italy from multidata analysis. Passive/active seismic, magnetic, magnetotelluric, electrical, and gravimetric surveys (https://progetti.ingv.it/it/pian-din, last access: 15 October 2024) are now ongoing in the framework of the INGV Dynamic Planet GEMME project (Integrated Geological, gEophysical and geocheMical approaches for 3D Modelling of complex seismic site Effects).
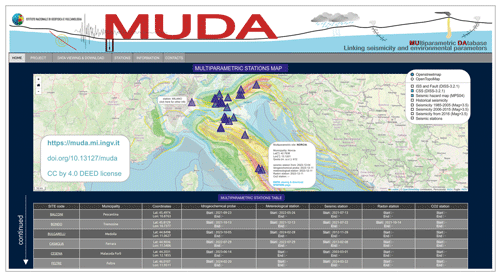
Figure 1MUDA home page: https://muda.mi.ingv.it. Examples of interactive pop-ups are reported in map indicating the available main options concerning station data and metadata, Italian seismic hazard (Stucchi et al., 2011), seismicity rate, and both composite and single seismogenic sources (DISS database, DISS Working Group, 2021). The base map is provided by © OpenStreetMap contributors 2024. Distributed under the Open Data Commons Open Database License (ODbL) v1.0.
The need for an infrastructure capable of acquiring, storing, organizing and publishing multiparameter data in near real time arose following the installation of the Garda multiparameter seismic network, PDnet (https://eida.ingv.it/it/networks/network/ZO, last access: 15 October 2024), installed starting from 2021 as part of the Task-S2 of the INGV Dynamic Planet project (Ferrari et al., 2024). In this framework, MUDA collects information from different types of sensors, such as seismometers, accelerometers, hydrogeochemical sensors for physical–chemical parameters in waters, geochemical sensors for measuring the diffusive flux of carbon dioxide (CO2) from the soil or detecting the air radon concentration, and meteorological stations. The aim is to constraint the influence due to exogenous parameters in order to make potential correlations between seismic phenomena and variations concerning monitored parameters (i.e. groundwater level, temperature, electrical conductivity, CO2 soil flux, air radon concentration; Barberio et al., 2017; Chiodini et al., 2020; Mastrorillo et al., 2020).
The challenge of MUDA is to provide the end user a high-quality dynamic but also simultaneous and continuous monitoring of groundwater physical parameters, meteorological data and seismic signals, together with gas concentration such as radon or soil CO2–CH4 fluxes (Fig. 3). In order to furnish the main information for a detailed interpretation of local phenomena in the framework of multi-hazard assessment, the multiparametric data are provided together with all necessary stations and sites metadata, supplied with a complete geological and morphological description (Fig. 4).
The multiparametric sites now included in MUDA are located in five main target areas (Fig. 2): Lake Garda, the eastern Alps, the Po alluvial basin, and the northern and central Apennine chains (Table 1). Concerning instrumental seismicity (http://terremoti.ingv.it/, last access: 15 October 2024), in the last 40 years, thousands of small- to moderate-energy seismic events (Fig. 2) have occurred in northern Italy. Despite the low-to-medium seismic hazard of the area (Stucchi et al., 2011), the high level of exposure (e.g. metropolitan areas, industrial plants), the local geological conditions and the proximity of active buried seismogenic structure (Burrato et al., 2012) make many portions of northern Italy a zone of medium to high seismic risk (Massa et al., 2022b; Lai et al., 2020).
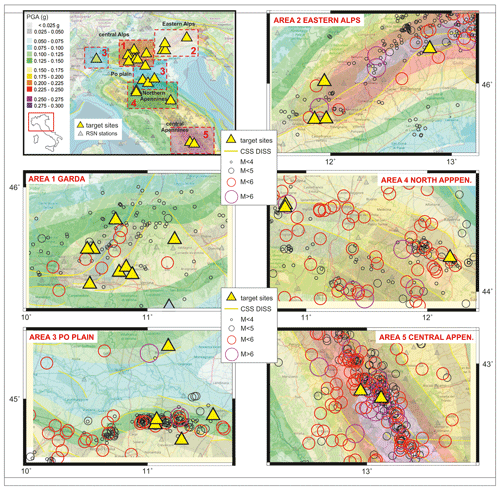
Figure 2Target areas and relative multiparametric sites. Each panel indicates the multiparametric site (yellow triangles), the Italian seismic hazard map in terms of horizontal peak ground acceleration (PGA) with 10 % probability of exceedance in 50 years on hard ground (Stucchi et al., 2011), the instrumental seismicity from 1985 (black circles, https://terremoti.ingv.it, last access: 15 October 2024), the historical seismicity (red circles, CPTI database, Rovida et al., 2020) and the seismogenic sources (CSS-DISS database, DISS Working Group, 2021). The base maps are provided by © OpenStreetMap contributors 2024. Distributed under the Open Data Commons Open Database License (ODbL) v1.0.
In particular, the Garda region (Area 1, Fig. 2) is characterized by low-to-moderate seismicity, with the active tectonic regime located on the margin of the southern Alpine chain controlled by the Africa–Europe convergence. The main active faults affecting the area consist of mainly NNE–SSW-trending thrusts (Galadini and Messina, 2001). For instance, the 24 November 2004, Vobarno Mw 4.8 earthquake (https://terremoti.ingv.it/event/1564989, last access: 15 October 2024), generated maximum macroseismic intensities (Imax) of VII/VIII (https://emidius.mi.ingv.it/CPTI15-DBMI15/, last access: 15 October 2024, Locati et al., 2022). It is worth noting that in the past, the same area was struck by several powerful events, such as the 30 October 1901, Salò Mw=5.4 earthquake (https://emidius.mi.ingv.it/CPTI15-DBMI15/, last access: 15 October 2024, Rovida et al., 2020).
Moving eastwards (Area 2, Fig. 2), the highest rate of energetic events in northern Italy is associated with the south-verging thrust faults typical of the central and east southern Alpine chain (Battaglia et al., 2004; Serpelloni et al., 2005; D'Agostino et al., 2008), due to the north–south convergence between the Adriatic microplate and the Alps. The most recent destructive earthquake occurred in Friuli, during the seismic sequence of 6 May 1976, with Mw=6.5 (Pondrelli et al., 1999, and references therein), whereas the largest historical event was the 1695 Asolo earthquake, with an estimated Mw=6.48 (https://emidius.mi.ingv.it/CPTI15-DBMI15/, last access: 15 October 2024) broadly associated with the thrust system of the Montello area (Danesi et al., 2015).
South of the Alps, the Po alluvial plain (Area 3, Fig. 2) represents a very deep foreland basin of two opposing verging fold-and-thrust belts developing in the framework of the African and European plates' relative convergence (Pieri and Groppi, 1981; Bigi et al., 1990). Despite the flat morphology, the Po Plain is far from being an undeformed domain, since the outermost and most recent thrust fronts of the two belts are buried by the Plio-Quaternary sedimentary sequence (Burrato et al., 2012). The historical and instrumental Italian seismic catalogues show that the southern Po Plain is affected by low to moderate seismicity, with Mw up to 5.8 during the 2012 sequence (Luzi et al., 2013). Considering the historical seismicity (Rovida et al., 2020), the central part of the Po Plain was struck by the more significant north Italy earthquake on 3 January 1117, with an estimated Mw=6.52.
Moving southwards, the northern Apennines (Area 4, Fig. 2) underwent the regional seismicity associated with the Apennine fronts defined by different arcs of blind, north-verging thrusts and folds (Mazzoli et al., 2015; Chiaraluce et al., 2017), capable of generating moderate-energy seismic events with a maximum magnitude of around 6 (i.e. 5 June 1501, Mw 6.05, Rovida et al., 2020). In particular, this area hosts the Nirano site (Table 1), in the Regional Natural Reserve of Salse di Nirano (Giambastiani et al., 2024), an area lying upon an anticline structure of the north-east verging fold-and-thrust Apennine belt characterized by one of the largest mud volcano fields in Europe (Bonini, 2008; Castaldini et al., 2005) coupled to the emission of CH4-dominated gases (e.g. Buttitta et al., 2020).
Finally, two stations included in MUDA are installed in the surroundings of the Norcia alluvial basin (Area 5, Fig. 2), an area characterized by high seismic hazard and seismicity rate due to dense extensional NW–SE active fault systems (e.g. Galadini and Galli, 1999; Brozzetti and Lavecchia, 1994) capable of generating high-magnitude earthquakes (Galli et al., 2018, 2019), such as the 14 January 1703, Mw=6.9 earthquake or other moderate events such as the 1328, Mw=6.3 earthquake; the 1730, Mw=5.9 earthquake; the 1859, Mw=5.5 earthquake; and the 1979, Mw=5.8 earthquake (Rovida et al., 2020). The recent instrumental seismicity highlights the two main events occurring on 24 August 2016 and on the 30 October 2016, with Mw 6.0 and 6.5, respectively, in an area a few kilometres of the Norcia plain (e.g. Improta et al., 2019, and references therein).
At present, in Italy and Europe, the seismological communities in general are fairly advanced in their running of both network data management and seismic data sharing. In Italy, the main seismic network is represented by the National Seismic Network (RSN, https://eida.ingv.it/it/networks/network/IV, last access: 15 October 2024, Margheriti et al., 2021), managed by INGV and sometimes integrated for real-time data exchange by many local or regional networks (Massa et al., 2022a). The RSN permanent network is codified through the IV code assigned by the International Federation of Digital Seismograph Networks, FDSN (https://www.fdsn.org/, last access: 15 October 2024). The RSN station codes are registered at the International Seismological Centre, ISC (http://www.isc.ac.uk/, last access: 15 October 2024), while data, recorded following the SEED (Standard for the Exchange of Earthquake Data, http://www.fdsn.org/seed_manual/SEEDManual_V2.4.pdf, last access: 15 October 2024) format, are shared (Danecek et al., 2021) through the EIDA-Italia node (European Integrated Data Archive, https://eida.ingv.it/it/, last access: 15 October 2024). In Italy, INGV provides many websites and thematic databases for real-time data quality and distribution, such as EIDA-Italia, ISMDq (INGV Strong Motion Data quality, https://ismd.mi.ingv.it, last access: 15 October 2024, Massa et al., 2022a), ITACA (ITalian Accelerometric Archive, https://itaca.mi.ingv.it, last access: 15 October 2024, Pacor et al., 2011), ESM (Engineering Strong Motion database, https://esm-db.eu/, last access: 15 October 2024, Luzi et al., 2016), BSI (Italian Seismic Bulletin, https://terremoti.ingv.it/bsi, last access: 15 October 2024, Marchetti et al., 2016), TDMT (Time Domain Moment Tensor, https://terremoti.ingv.it/tdmt, last access: 15 October 2024, Scognamiglio et al., 2009) and ShakeMaps (https://shakemap.ingv.it/, last access: 15 October 2024, Michelini et al., 2020).
Differently, the geochemical community has still not developed such a capillary network of automatic stations for data acquisition, management and sharing, as the seismic community has. This mostly depends on the fact that only a few geochemical parameters/tracers can be measured directly in the field and in near real time (e.g. diffusive flux of CO2 from the soil through the accumulation chamber method (Chiodini et al., 1998; Carapezza et al., 2004; Inguaggiato et al., 2011a; Rizzo et al., 2015); radon concentration in the atmosphere or from the soil with specific Geiger counters; concentration of H2O, CO2, SO2, H2S and CH4 halogens in the atmosphere through MULTIGAS sensors (Aiuppa et al., 2005; Shinohara, 2005) or the FTIR technique (e.g. Allard et al., 2005); and the SO2 flux in the atmosphere by differential optical absorption spectroscopy (DOAS) and UV techniques (Burton et al., 2009; Aiuppa et al., 2005)). It must also be highlighted that most of the automatic measurements of geochemical parameters reported above were developed and applied in volcano monitoring, while only recently has the geochemical community been moving to apply some of those tracers to seismic monitoring. In terms of hydrogeochemical monitoring, apart from the physical–chemical parameters in water (e.g. temperature, water level, electric conductivity, and others such as pH and Eh but with less precision and accuracy) for which automatic sensors have existed for a long time, the automatic and high-frequency measurement of the water's composition is limited to a few sensors developed in the last decade or so, which mostly focus on the concentration of a few gas species dissolved in waters (e.g. CO2, CH4, total gas pressure; De Gregorio et al., 2005; Inguaggiato et al., 2011b). As for gas sensors, most of the automatic measurements of water's composition were developed for volcano monitoring applications.
At present, in Italy, hydrogeochemical and geochemical seismic monitoring is limited to selected areas or sites, and it is essentially performed by several departments of INGV in the framework of individual initiative such as the Alto Tiberina Near Fault Observatory (TABOO; https://ingv.it/en/monitoring-and-infrastructure-a/monitoring-networks/the-ingv-and-its-networks/taboo, last access: 15 October 2024, Chiaraluce et al., 2014) or recent and ongoing INGV projects such as the Dynamic Planet (https://progetti.ingv.it/it/pian-din, last access: 15 October 2024), FURTHER (https://progetti.ingv.it/en/further, last access: 15 October 2024), MYBURP (https://progetti.ingv.it/it/pian-din#myburp-modulation-of-hydrology-on-stress-buildup-on-the-irpinia-fault, last access: 15 October 2024), Multiparametric Networks or Rebuilding Central Italy, DL50 and related tasks (e.g. Idro-DEEP CO2, Idro-Calabria, Idro-Nord), concerning the groundwater continuous monitoring (e.g. springs and thermal waters) of different areas of mainly the central and southern Italian Apennines (https://www.pa.ingv.it/index.php/progetti/, last access: 15 October 2024) and radon monitoring (IRON project, https://ingv.it/monitoraggio-e-infrastrutture/reti-di-monitoraggio/l-ingv-e-le-sue-reti/iron, last access: 15 October 2024). In particular, the Alto Tiberina Near Fault Observatory is managed by the EPOS (European Plate Observing System) research infrastructure (https://www.epos-eu.org/, last access: 15 October 2024) of which the mission is to foster the integration of solid earth data and their by-products made by the entire European scientific community: in this case, seismological, geophysical, geodetic and geochemical data recorded by the TABOO Near Fault Observatory are accessible via the FRIDGE European web portal (https://fridge.ingv.it/index.php, last access: 15 October 2024).
Further local monitoring initiatives are provided by other institutions or universities through the installation of geochemical stations and probes in different parts of the national territory such as in Tuscany, by the IGG-CNR (Institute of Geoscience and Earth Resources, https://www.igg.cnr.it/en, last access: 15 October 2024); in southern Italy, by the IMAA CNR (Institute of methodologies for environmental analysis, https://www.cnr.it/en/institute/055/institute-of-methodologies-for-environmental-analysis-imaa, last access: 15 October 2024); or central Italy, by the Department of Earth Sciences (DES, https://www.dst.uniroma1.it/en, last access: 15 October 2024) of Sapienza University in Rome (Martinelli et al., 2021).
Consequently, at a national scale, the hydrogeochemical and geochemical monitoring is not organized using an ad hoc reference institutional database or web portal able to homogeneously archive and distribute high-quality multiparametric data to the scientific community. At present and to the best of our knowledge, the only existent databases focus only on mapping gas emissions (e.g. MaGa, http://www.magadb.net/, last access: 15 October 2024) or thermal springs, without archiving data from a regular monitoring. A first attempt was recently made in the framework of an agreement between the National Institute of Geophysics and Volcanology (INGV) and the National System for Environmental Protection (SNPA, https://www.snpambiente.it/, last access: 15 October 2024, comprising the Regional Environmental Protection Agencies, ARPA, and the Italian Institute for Environmental Protection and Research, ISPRA, https://www.isprambiente.gov.it/, last access: 15 October 2024), with the aim of sharing data from the continuous monitoring of water wells and springs, in particular the piezometric level, temperature, electrical conductivity, salinity and total dissolved solids (Comerci et al., 2019).
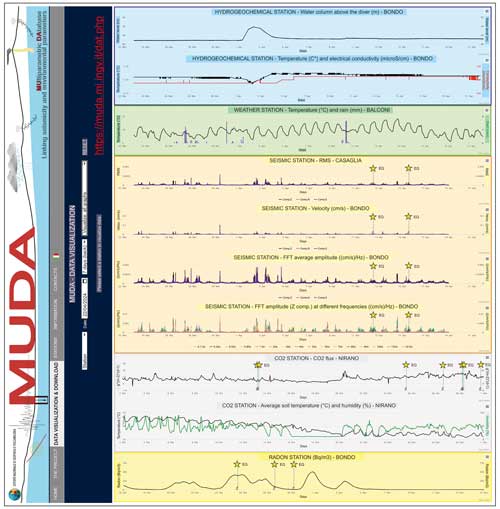
Figure 3Dynamic multiparametric data web page: https://muda.mi.ingv.it/dat.php (last access: 15 October 2024). From top to bottom: hydrogeochemical data (light-blue panels: water level, m; water electrical conductivity, µS cm−1; water temperature, °C), meteorological data (green panel: soil temperature, °C; rain, mm), seismic data (light-brown panels: rms, ground motion velocity, cm s−1; FFT, cm s −1 Hz−1; FFT(f), cm s −1 Hz−1), CO2 data (grey panels: soil flux, g m−2 d−1; humidity, %; soil temperature, °C) and radon data on air (yellow panel: Bq m−3). All time series (CSV format) and each single graph (PDF and PNG formats) are downloadable using the menu available in the top right corner of each panel.
MUDA is a dynamic and relational multiparametric database designed and built using a table structure that can correlate data of a different nature (i.e. seismic, hydrogeochemical, geochemical, meteorological). It is adaptable to further types of data from other projects and capable of integrating perfectly with those already acquired via both real-time and offline transmission vectors (Fig. 5).
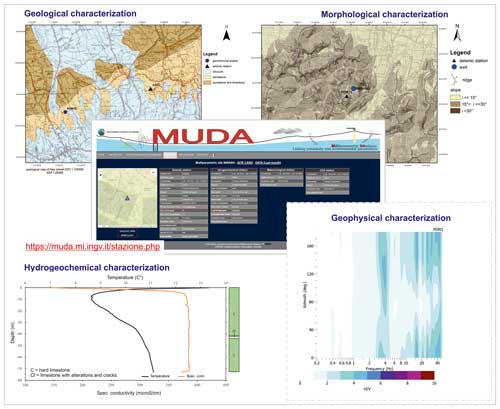
Figure 4Multiparametric site web page: https://muda.mi.ingv.it/stazione.php (last access: 15 October 2024). The single site web page indicates the main features of both instrumentation and installation; downloadable thematic maps, such as the geological map (1:100 000, Società Geologica Italiana http://www.isprambiente.gov.it/it/cartografia/, last access: 15 October 2024) and the topographic map (base at 1:25 000, Istituto Geografico Militare, http://www.igmi.org/prodotti/cartografia/carte_topografiche/, last access: 15 October 2024); and log and stratigraphy, concerning the available wells for water, a preliminary geophysical soil characterization in term of horizontal-to-vertical spectral ratio performed on ambient seismic noise and the complete hydrogeochemical time series. Sources for base maps: Esri, DigitalGlobe, GeoEye, i-cubed, USDA FSA, USGS, AEX, Getmapping, Aerogrid, IGN, IGP, swisstopo, and the GIS user community.
The MUDA database is based on MySQL (https://www.mysql.com/it/, last access: 15 October 2024), a popular and efficient open-source relational database management system for handling large amounts of data. Particular attention has been paid to optimizing and, above all, integrating all the different types of data taken from different sources while trying to maintain a certain structural uniformity and also being open to possible future new implementation.
Data collection takes place separately for each type of monitoring station (Table 1), each according to its preferred channels (email, FTP system, application programming interface (API), structured query language (SQL)) with the effort to improve each procedure, avoid data loss and minimize the time taken to receive data.
Data are acquired and then archived on a centralized server from which all pre-processing procedures are then carried out to insert this data, after appropriate checks and automatic analysis, into the MUDA database (see next chapter for details). All data downloaded from the remote stations, after the check and processing phase, are stored in files before the population of the MySQL database. This is also convenient to have a native and complete data backup, for future requirements.
The MUDA database is structured to consider all the different types of monitoring stations at the same multiparametric site through a univocal internal site code, linked to all different types of data. At the same time, however, the independence of the data of each different station is also maintained, as each individual site may have its own particular condition and metadata. For each type of monitoring station, the MUDA database includes two tables, one for the station metadata and the other for the recorded data, linked by a unique station ID.
4.1 Processing of raw data
The MUDA project currently includes five types of data: hydrogeochemical, meteorological, radon, CO2 and seismic. All data are pre-processed to align each time series to the common UTC (coordinated universal time) time. Hydrogeochemical, seismic, meteorological and radon data are moreover resampled in order to have representative data every few minutes (i.e. from 1 to 5), namely a good enough interval to see possible cross-correlation signals on different parameters. A data resample is necessary a priori in order to, at first, homogenize data for viewing and comparison but also to allow the web page to have a fast response to any query involving long time periods (actually up to a maximum of 30 d) of continuous and high-frequency multiparametric recordings. In particular, while hydrogeochemical, gas and meteorological data are uploaded into the MUDA database as raw data with only a consistency check, the seismic data are pre-processed in order to obtain waveform metadata to be included in the MUDA database and to be easily comparable in terms of time series to the other parameters.
The processing of raw data for each of the five parameters included in MUDA is described in detail in the following.
The hydrogeochemical data are acquired by two different types of instrumentation, the first provided by Van Essen Instruments (https://www.vanessen.com/, last access: 15 October 2024) and the second by STS-Italia S.r.l. (https://www.sts-italia.it/, last access: 15 October 2024). Data recorded by Van Essen Instruments and STS-Italia are set to sample records every 1 and 10 min, respectively. In both cases, groundwater level (m), electrical conductivity (µS cm−1) and temperature (°C) are obtained using probes (e.g. CTD-Diver®, https://www.vanessen.com/, last access: 15 October 2024) installed in correspondence of wells or springs using weir flowmeters; the recorded chemical and physical parameters are sent at defined time intervals to the remote company head offices and then to the INGV acquisition centre by proprietary API or email. At present, remote stations send data in ASCII format twice a day (i.e. 09:00 and 21:00, central European time, CET) as an email attachment or by FTP protocol. Depending on the target instrumentation, before populating the MUDA database, pre-processing is necessary: as an example, the Van Essen probes for water wells need a barometric compensation to account for atmospheric pressure variations in order to provide the corrected water level value (Ferrari et al., 2024). Automatic data acquiring and processing tools have been developed in Python (https://www.python.org/, last access: 15 October 2024), while automatic tools for populating the MUDA database have been developed in PHP language (https://www.php.net, last access: 15 October 2024).
The meteorological data included in MUDA are provided by Davis Vantage Vue® instrumentation (https://www.davisinstruments.com/, last access: 15 October 2024). Each single meteorological station, placed near the water well, provides information on atmospheric pressure (mbar), temperature (°C), humidity (%), rainfall amount (mm), and wind speed and direction. Also in this case, data take advantage of GPRS/LTE technologies and are gathered by a dedicated WeatherLink Live cloud platform, making them available in real time on the dedicated website (https://www.weatherlink.com/, last access: 15 October 2024). With samples every 1 min, data are archived into the Davis server cloud and then shared by payment to the end users (e.g. INGV server) through proprietary Application Programming Interface (API) set into an automated ad hoc-developed Python tool, suited for all the different kinds of meteorological instrumentation at each site. Just as for hydrogeochemical data, meteorological parameters are then automatically inserted into the MUDA database twice a day (i.e. 09:00 and 21:00 CET) using a procedure developed in PHP.
The radon data are provided by the IRON network (Italian Radon mOnitoring Network, https://ingv.it/monitoraggio-e-infrastrutture/reti-di-monitoraggio/l-ingv-e-le-sue-reti/iron, last access: 15 October 2024, Cannelli et al., 2018). Stations, placed next to the water well, measure the concentration of gas in the air using a photodiode detector (AER Plus, Algade), with a sensitivity of 15 Bq m−3 by counts h−1. Data are measured and acquired every 4 h together with temperature and humidity, and radon data are transmitted in real time by the Sigfox (https://www.sigfox.com, last access: 15 October 2024) 0G-technology and archived at the Sigfox Cloud, with the exception of particularly remote sites where a periodic local data downloading is also necessary. Radon data are provided in CSV format, where the concentration is measured in Bq m−3, and then uploaded into the MUDA database using an ad hoc-developed PHP tool. In this case, the procedure is manually started after each single data downloading.
The CO2 soil flux measurements are acquired using an accumulation chamber provided by THEAREN S.r.l (https://thearen.com/, last access: 15 October 2024). The permanent stations have a no-stationary flux chamber and are equipped with an infrared analyser measuring CO2 concentrations (g m−2 d−1) in a time frame of 3 min. A single CO2 flux measure is returned each hour already corrected for pressure (mbar) and temperature (°C) recorded inside the chamber. Soil temperature and humidity (%) and meteorological parameters (atmospheric pressure, temperature, humidity, rain, wind speed and direction) are acquired concurrently. Data are sent to the head company server cloud through a dedicated modem with automatic data transmission. Data are acquired daily by the INGV acquisition centre through an ad hoc server to server link using an internal INGV VPN (virtual private network) connection. Data provided in CSV format are then automatically inserted into the MUDA database daily using an ad hoc-developed PHP tool.
The seismic data are acquired by selected stations of the Italian National Seismic Network (RSN, https://eida.ingv.it/it/networks/network/IV, last access: 15 October 2024) and the multiparametric network of northern Italy (PDnet, https://eida.ingv.it/it/networks/network/ZO, last access: 15 October 2024) placed near the water well used for hydrogeochemical data. Recorded data are codified following the international standard commonly used by the seismological community, namely the FDSN (https://www.fdsn.org/, last access: 15 October 2024) network-station code and SEED (Standard for the Exchange of Earthquake Data, http://www.fdsn.org/seed_manual/SEEDManual_V2.4.pdf, last access: 15 October 2024) format supported by European Integrated Data Archive, EIDA (https://eida.ingv.it/it/, last access: 15 October 2024) and maintained by the FDSN. Data are transmitted by different technologies (LTE, satellite, etc.) to the INGV Milan acquisition centre where they are archived through a Seiscomp4 (https://www.seiscomp.de/doc/apps/seedlink.html, last access: 15 October 2024) client to improve the SeedLink real-time data acquisition protocol. Data are archived in the standard binary miniSEED format (http://ds.iris.edu/ds/nodes/dmc/data/formats/miniseed, last access: 15 October 2024) and organized in a structured archive. Seismic data are pre-processed every night considering the 24 h of all miniSEED files recorded by stations on the previous day and then checked for quality before being automatically included in the MUDA database using an ad hoc-developed PHP tool.
In dependence of the adopted sampling rate (i.e. 100 Hz for seismometers and 200 Hz for accelerometers) the amount of continuous data stream per day, relative to a single channel at each single station, ranges between 6 and 10 MB for seismometers and between 15 and 20 MB for accelerometers. Considering many seismic stations, the result is a total daily archive on the order of gigabytes, not easy to organize in the framework of a quasi-real-time data distribution provided by a thematic web portal. In this framework, also considering the sampling rate of the other multiparametric instrumentation (i.e. spanning one record per minute to one record every 4 h), the continuous seismic data streams are processed in order to conform the contents to the web portal requirements before inclusion in the MUDA database. In particular, every night at 02:00 miniSEED files relative to the 24 h recordings of the previous day are selected at each station and processed using an ad hoc procedure developed by merging the Bash and SAC (Seismic Analysis Code, https://ds.iris.edu/files/sac-manual/, last access: 15 October 2024) scripting languages.
The processing scheme starts by downloading 24 h of miniSEED files at each single recording station; data are then separated into 288 sub-windows, each one with a length of 5 min starting from the origin time of each single miniSEED file (usually corresponding to the 00:00:00 UTC if the station works well). Then, for each 5 min windows, raw data recorded in counts are converted to the proper unit of measurements (cm s−1 for seismometers and gal for accelerometers), and the sensor response curves are removed by deconvolution and finally filtered using a fourth-order Butterworth filter in the range 0.1–20 Hz. For each single sub-window relative to a specific channel recorded by a specific station, the rms (root mean square; e.g. Goldstein et al., 2003), the maximum ground shaking in terms of velocity (cm s−1), the mean amplitude value of the whole fast Fourier transform (FFT) (cm s −1 Hz−1) (fast Fourier transform, e.g. Bormann, 2012) and the maximum amplitude of the FFT for frequency interval spanning 0.1 to 20 Hz, are calculated, providing for each parameter 288 values per day, corresponding to the daily upload of hydrogeochemical and meteorological data. In particular, the rms is calculated for the entire time windows using the following equation:
where x is the amplitude of the single sample, and n the number of samples of the trace considered. Daily time series of rms, peak ground velocity (PGV), FFT mean and FFT(f) are finally uploaded into the MUDA database using an ad hoc-developed PHP tool.
4.2 Data availability and dissemination
MUDA publishes and shares the available data recorded at each site through a specific web interface developed in PHP (https://www.php.net/, last access: 15 October 2024) to easily and effectively interact with the MUDA SQL database and using a responsive design in HTML5, capable of adapting automatically to any device on which it is displayed (i.e. PC, tablet, smartphone, etc.). As a final step, the data publication required assigning a regular DOI associated with the DB and provided by INGV data management office through a standard procedure. The final DOI of MUDA is https://doi.org/10.13127/muda (Massa et al., 2023). Data have been licensed using the Creative Commons License CC BY 4.0. The MUDA web portal publishes multiparameter data daily that are updated to the previous day. It offers the chance to view and download dynamic time series for all available data and for different time periods, up to a maximum of 30 d. In reference to longer periods, an e-mail request can be sent to muda@ingv.it.
The web portal has a main page showing an interactive map of northern and central Italy, as at present MUDA acquires data from automatic stations located in this part of the country, where the multiparametric stations are indicated by triangles with pop-ups showing the main features (i.e. coordinates and available instrumentation) of the target site, including the direct access to the dynamic data viewing (Fig. 3) and to the single station page (Fig. 4). The home page in the top-right corner shows a pop-up menu with selectable thematic layers, including the reference seismic hazard map of the national territory in terms of peak ground acceleration (MPS04 working group, http://zonesismiche.mi.ingv.it/, last access: 15 October 2024, Stucchi et al., 2011), the seismogenic areas and the active faults taken from the Database of Individual Seismogenic Sources database (DISS, https://diss.ingv.it/, last access: 15 October 2024, DISS working group 2021), recent (https://terremoti.ingv.it/, last access: 15 October 2024) and historical (https://emidius.mi.ingv.it/CPTI15-DBMI15/, last access: 15 October 2024) seismicity bulletins, and the location of seismic stations of the National Seismic Network (RSN, https://eida.ingv.it/it/networks/network/IV, last access: 15 October 2024) managed by INGV.
The `̀View & Download DATA” web page, accessible from the horizontal tool bar of the home page, opens the dynamic data viewing (Fig. 3). Users can select the time series to be displayed backwards in time for each multiparameter site (i.e. 1, 7, 15, 30 d), starting from a selected date. Once the site and period have been chosen, the available data automatically appear synchronized with respect to UTC (coordinated universal time). From top to bottom, these are hydrogeochemical data from well or spring sensors showing water temperature (°C), electrical conductivity (µS cm−1) and the value of the water column (m) above the sensors; meteorological data showing air temperature (°C) and rainfall (mm); seismometric data showing the rms, the maximum ground velocity values (cm s−1), the average FFT amplitude values and the Fourier spectrum values for frequency bands selected in the interval 0.1–20 Hz; and radon gas emissions (Bq m−3) and soil CO2 flux (g m−2 d−1). All interactive graphs can be zoomed in on with the left mouse button, and they enable selecting individual functions using pop-up layers. In each graph, in the top right-hand corner, it is possible to view the individual image in full screen and download the selected data in CSV (comma separated values) format as well as the images in PDF (portable document format), PNG (portable network graphic), JPEG (joint photographic experts group) and SVG (scalable vector graphics) formats. The last selectable item, on the right of this page, gives the possibility of viewing a single parameter, for a more detailed observation.
A further topic of the MUDA web portal is the single station web page (i.e. link “STATIONS”), also reachable from the horizontal tool bar. This web page is designed to provide a first and general geophysical and geochemical characterization of each multiparametric site. In particular, each single station web page shows, on the left, a thematic map indicating the location of the monitoring site. Below the map, the two links provide a geological and morphological setting of the area. For each recording site, a portion selected from the geological map at a 1:100 000 scale (Società Geologica Italiana, http://www.isprambiente.gov.it/it/cartografia/, last access: 15 October 2024) is provided, with topographic base at 1:25 000 scale (Istituto Geografico Militare, http://www.igmi.org/prodotti/cartografia/carte_topografiche/, last access: 15 October 2024). Concerning morphology, for each recording site, a topographic map (i.e. Slope and Ridge) is proposed by considering the available digital elevation model (ASTER GDEM with a cell size of 10 m, https://www.earthdata.nasa.gov/news/new-aster-gdem, last access: 15 October 2024). Starting from the processed DEM, the slope map was constructed with three topographic classes (0–15, 15–30 and > 30°), considering the break values defined in the current Italian seismic code (Ministero delle Infrastrutture e dei Trasporti Aggiornamento delle Norme Tecniche per le Costruzioni, 2018). The ridgelines were extracted using the Topographic Position Index (TPI) algorithm (Pessina and Fiorini, 2014). On the right, the web page shows thematic tables relative to the installed instrumentation. At each site, besides the general information on coordinates and technical features of the instruments, a geophysical characterization of the site is also provided in terms of polarized horizontal-to-vertical spectral ratio (HVSR) on ambient noise (Nakamura, 1989) and each single log performed in the wells regarding water electrical conductivity and temperature as a function of the available stratigraphy, together with the main features of monitored well or spring. Finally, on each station web page, graphs relative to the whole hydrogeochemical time series are also downloadable.
At present (i.e. 31 March 2024), MUDA includes data from 25 multiparametric sites (Table 1) located in northern and central Italy (Fig. 2), both already monitored by permanent INGV infrastructures or installed in the framework of recent INGV research projects. It is worth mentioning that not all multiparametric sites are characterized by homogeneous multiparametric instrumentation (Fig. 6). In any case, all available data are always sent in real time to the INGV acquisition centres. In particular, concerning seismic data, the multiparametric sites include seven stations belonging to the permanent National Seismic Network (RSN, https://eida.ingv.it/it/networks/network/IV, last access: 15 October 2024) and 12 stations belonging to the temporary multiparametric network of northern Italy (PDnet, FDSN code ZO, https://eida.ingv.it/it/networks/network/ZO, last access: 15 October 2024), installed in the framework of the INGV Dynamic Planet S2-project. These sites are located in northern Italy, around the Lake Garda, at the southern limit of the eastern Italian Alps and in the central portion of the Po Plain (Fig. 2). Two further seismic stations (PDN11, PDN12, Table 1) have recently been installed in the framework of the INGV Dynamic Planet GEMME (Integrated Geological, gEophysical and geocheMical approaches for 3D Modelling of complex seismic site Effects) project, in Norcia basin (Apennine chain in central Italy) and its surroundings (Fig. 2). Finally, one seismic station (PDN10, Table 1) has been installed in cooperation with the Dynamic Planet PROMUD (Definition of a multidisciplinary monitoring PROtocol for MUD volcanoes) project, in the Salse di Nirano Reserve (Italian northern Apennines, Fig. 2).
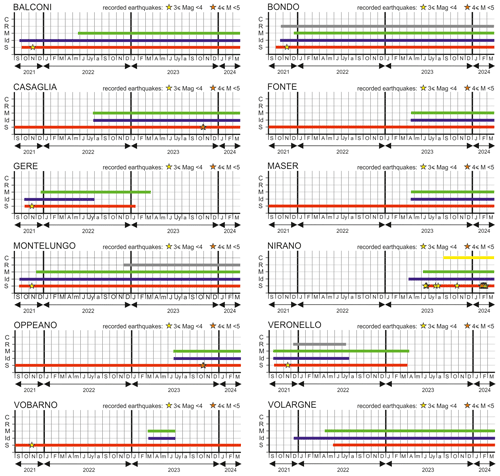
Figure 6Single station dataset for multiparametric sites with at least 6 months of data and three different types of acquisition. S: seismic data (red); ID: hydrogeochemical data (blue); M: meteorological data (green); R: radon data (grey); C: CO2 data (yellow). Yellow and orange stars indicate recorded earthquakes at each site with magnitude (moment or local) lower and higher than 4, respectively.
In general, seismic stations are equipped with high-dynamic 24-bit digital recording systems coupled to enlarged (5 s owner period) or broadband (120 s owner period) seismometers. In particular, at Oppeano multiparametric site (Table 1), borehole instrumentation is installed at 150 m depth. Even if in some cases, accelerometer sensors are coupled to the target seismometer (velocimeter), MUDA only includes seismometric data due to their higher sensitivity and best resolution with respect to possible occurrences of natural phenomena such as microseismicity, local weak motion earthquake occurrences, and/or teleseisms or environmental modifications (i.e. landslides, low atmospheric pressure, tides, etc.).
Hydrogeochemical data are recorded in all 25 multiparametric sites (Table 1), at both wells and springs set up in the framework of INGV Dynamic Planet projects (S2 and GEMME) and INGV Multiparametric Networks or the Rebuilt central Italy, DL50 project. The Toppo site (Table 1), installed in the eastern Alps in the framework of the ECCSEL ERIC consortium (https://www.eccsel.org/, last access: 15 October 2024), is managed by OGS (National Institute of Oceanography and Applied Geophysics, https://www.ogs.it/en, last access: 15 October 2024) in cooperation with the University of Ferrara (Department of Physics and Earth Science, http://fst.unife.it/en, last access: 15 October 2024) and the INGV. At present, data included in MUDA are recorded at 22 wells and 3 springs (Recoaro, Recoaro1, Feltre, see Table 2). In general, wells have depths ranging from a few metres (< 10 m) to a maximum of 300 m for Toppo and Mirandola sites (Table 2). The instrumented wells have a mean depth between 5 and 150 m. Monitored sites are mainly characterized by cold waters, whose average temperatures range from 10.3 to 17.4 °C, except for Triponzo site that contains thermal water (∼ 30 °C; Table 2). A wide variety of water electrical conductivity values (∼ 130–88 000 µS cm−1; Table 2) are illustrative of heterogeneous compositions and salt contents. Information on the presence of a borehole pump (Table 2) and the frequency and intensity of water pumping was carefully collected from well owners. Water level, temperature and electrical conductivity changes are observed because of pumping for water chemical analysis (e.g. Balconi) or irrigation purposes (e.g. Norcia) or functioning of spa (e.g. Triponzo). These anthropogenic variations must be accounted for when analysing groundwater data. A comprehensive example of data-processing able to recognize in groundwater time series both natural phenomena and anthropogenic noise signals is reported in Ferrari et al. (2024) for the site of Balconi.
Table 2Main features of hydrogeochemical probes and seismic monitoring stations. Bold values indicate wells with thermalism (i.e. Triponzo site) and the borehole seismic sensor installed at 150 m depth (i.e. Oppeano site). In detail, water level is the water depth below ground level at the time of installation; water column is the water column above the sensor at the time of installation; water temperature is the average values of water temperature. Water-specific electrical conductivity is also recorded. It is worth noting that for some sites the averages are merely indicative as they refer to short recording intervals (see Table 1 for installation dates). Specific electrical conductivity is recalculated at 25 °C. Water pump is the absence (0) or presence (1) of water pump.
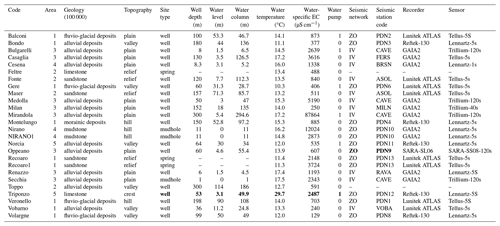
All sites monitored by hydrogeochemical instrumentation are also equipped with a meteorological station able to capture the main atmospheric variations.
Four sites (i.e. Montelungo, Bondo, Norcia, Triponzo; Table 1) are currently equipped with instrumentation able to record the radon concentration in the air. Sensors for radon monitoring are part of the IRON network (Italian Radon mOnitoring Network, https://ingv.it/monitoraggio-e-infrastrutture/reti-di-monitoraggio/l-ingv-e-le-sue-reti/iron, last access: 15 October 2024) of INGV. Finally, just one site (i.e. Nirano; Table 1) is equipped with instrumentation for carbon dioxide (CO2) soil flux measurements.
In general, the multiparametric sites show co-located instrumentation, with a few exceptions due to logistic difficulties during the site installation or other technical problems (e.g. sites with a very high level of background seismic ambient noise or working pump installed in the well). In all cases, the reference seismic station is installed in the same geological, morphological and hydrogeological context as the other instruments, possibly co-located or at least in the proximity. Hydrogeochemical stations installed in a narrow area (e.g. Bulgarelli, Medolla, Mirandola and Secchia sites; Table 2) might refer to the same seismic station.
5.1 Data quality check
In order to verify the completeness and correctness of the recorded data, we carried out several checks.
Concerning seismic data included in MUDA, the results of the processing procedure to produce 5 min interval data are verified to check the capacity of the proposed processing scheme to represent a real marker to detect earthquakes or environmental phenomena. Figure 7 reports an exhaustive example at the Oppeano site for events recorded by the seismic station on 29 December 2020. Figure 7a (top) the occurrences of subsequent events in a narrow time window spanning 29 December 2020 14:02:40 to 15:36:57 UTC. The first evident transient is related to the regional Mw=6.3 Croatia earthquake on 29 December 2020 at 12:19:54 UTC (https://terremoti.ingv.it/event/25870121, last access: 15 October 2024). A few hours later, a small sequence of three local weak earthquakes was recorded 12 km south-west of Oppeano and localized by the INGV bulletin on 19 December 2020 at 14:02:40, 14:44:51 and 15:36:47 UTC with magnitudes of ML=3.4, ML=2.8 and Mw=3.9, respectively (https://terremoti.ingv.it/event/25871441, last access: 15 October 2024). In the bottom of panel (a), the results of our detector procedure in terms of FFT interval are shown for the 288 data points of the 29 December 2020. It is evident how all transients have been clearly recognized by marked peaks: in particular, the regional event, occurring about 400 km east of the Oppeano well highlights a notable contribution at low frequency, showing a clear peak for the FFT around 0.1 Hz. Conversely, the local seismicity is well described by peaks detected at higher-frequency content, in particular ranging from 1 to 10 Hz. In this case, it is worth noting how the high-frequency content of FFTs also highlights variations in ambient noise level between night and day. In Fig. 7b, the results of the detection procedure are presented in terms of relative rms, ground motion velocity and averaged FFTs (from top to bottom).
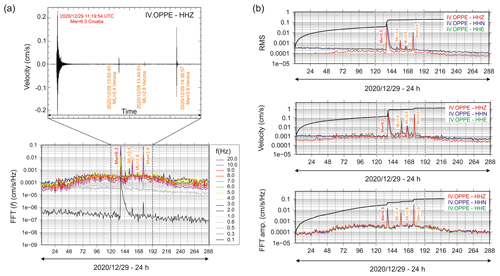
Figure 7Quality check of seismic data at Oppeano site (Table 1). Panel (a) (top) shows the time series recorded on 29 December 2020, by IV.OPPE station (https://terremoti.ingv.it/instruments/station/OPPE, last access: 15 October 2024) showing consecutive earthquakes: the first with Mw=6.3, occurred in Croatia land (https://terremoti.ingv.it/event/25870121, last access: 15 October 2024) 450 km east of IV.OPPE and the others with epicentres 11 km south-west of IV.OPPE with maximum Mw=3.9 (https://terremoti.ingv.it/event/25871441, last access: 15 October 2024). Earthquake origin times (UTC) are reported in the top panel (a). The bottom of panel (a) shows the FFT functions calculated considering 15 frequency intervals from 0.1 to 20 Hz, considering 288 consecutive 5 min-time windows (i.e. 24 h) selected on the vertical component of motion. Panel (b) shows, from top to bottom, the rms; the ground motion velocity; and the mean FFT calculated for 288 consecutive time windows, with a duration of 5 min. Red, blue and green indicate the vertical, the north–south and the east–west horizontal components of motion. The solid black lines indicate the cumulative functions.
In order to publish reliable results, all continuous seismic data streams for all seismic stations are checked daily for quality by the interoperability between MUDA and ISMDq (INGV Strong Motion Data quality; http://ismd.mi.ingv.it, last access: 15 October 2024, Massa et al., 2022a). In this way all seismic stations included in MUDA are checked for gaps (%), availability (%) and ambient noise level variation in terms of PSD (power spectral density) and PDF (probability density function) (in dB), as calculated by McNamara and Buland (2004). Through ISMDq, it is possible to build temporal time series with a maximum time length of up to 2 years for all stations included in MUDA in order to first check the correct functioning of the stations, the accuracy of used metadata, daily and seasonal variations of ambient noise level, and transient or permanent anthropic disturbances. In particular, in the case of failure in data transmission, the continuous monitoring of data gaps and availability allows us to retrieve data directly from station memories, thereby avoiding important gaps in the data.
Hydrogeochemical and geochemical data are checked daily for availability and gaps, usually due to a temporary lack of data transmission platforms (i.e. cellular line or satellite), in particular during rainy and stormy days or more rarely due to malfunctioning of the instrumentation. In the first case, the recorders, thanks to their internal memory and data-logging capacity, are able to archive data up to a maximum of 30 d. If needed, the recovery of data is possible through a manual download. Subsequently data are uploaded into the MUDA database by calling the same ad hoc-developed tools for populating the database for each parameter, with appropriate flags. In some cases, water level time series show unusual abrupt peaks (i.e. spurious spikes that are filtered) due to some problems during the compensation of atmospheric pressure performed by the recording system in order to obtain the correct values of water level: in general, misalignments in pressure compensation lead to wrong water level values, usually with differences of ∼ 10 m, as a consequence of the measured atmospheric pressure usually around or slightly above 1000 mbar, considering that all the stations are within a few hundred metres above sea level.
Other data included in MUDA (i.e. meteorological, radon, CO2 flux) do not need particular processing. In any case, for all meteorological, radon and CO2 measurements, data are always checked for gaps and possible spurious peaks that are deleted. In particular, meteorological data regarding the pluviometry are archived and uploaded into MUDA as a single sample recorded each minute or, even better, in some cases, at each individual movement of the rain gauge's tipping bucket (0.2 mm every time). In these cases, before publishing the rain values on the website, data are cumulated in intervals of 1 h directly in the SQL query made by the web portal, in order to better highlight heavy storms or other particular meteorological phenomena.
In this section, some examples of comparison among multiparametric data are presented and discussed in the framework of their possible applications for research and services devoted to natural hazard risk reduction.
An interesting example of multiparametric monitoring, concerning groundwater level variation presumably related to large landslide phenomena has been collected at the Bondo (Table 1) site and aquifer (Lake Garda area, Fig. 2), where on 1 November 2023, the water column above the sensor in the well abruptly increased by ∼ 20 m. This significant modification, also combined with the diminishing temperature and electrical conductivity (Fig. 8a), happened as a consequence of 2 d of intense rainfall with measured values of precipitation up to 400 mm in a narrow area surrounding Bondo. It is important to highlight that the stratigraphy below Bondo is mostly made of fractured karstified limestones belonging to the Dolomia Principale formation (Upper Triassic). To explain such a notable groundwater level variation, additional and contemporary natural phenomena should be hypothesized; on the basis of the information provided by the local media, in the same period the area was affected by diffuse landslides. In particular, between 31 October and 1 November 2023, a clear seismic transient (Fig. 8b) was recorded on all three components of ZO.PDN3 station (Table 2), characterized by very long duration (i.e. some hours), higher amplitudes with respect to the local ambient noise level (i.e. the PGV was equal to 0.01 cm s−1, comparable to a local earthquake with magnitude ranging from 2.5 and 3.0), prevalent high-frequency content (∼ 5–30 Hz) and a strong polarization with preferential amplification of motion along the N–S direction (Fig. 8c). In this case, the recorded transient at ZO.PDN3 could in fact be attributable to local and diffuse landslides that could have modified the volume and/or the extent of the aquifer and eventually the water flow infiltration and circulation through the rock fractures by influencing the water level and leading the aquifer to be more sensitive to meteorological events.
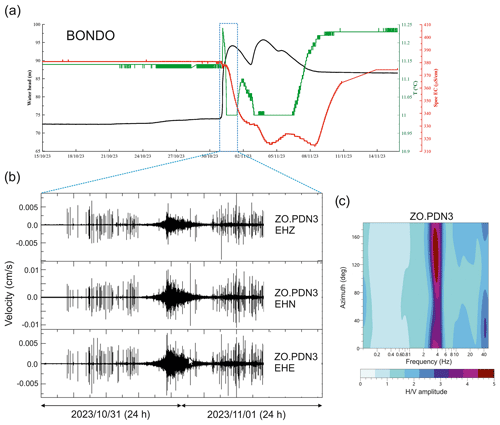
Figure 8Example of multiparametric data recorded at the Bondo site (Table 1). Panel (a) shows the hydrogeochemical data recorded in the time period from 15 October to 15 November 2023: solid black, red and green lines indicate the water level (m), the electrical conductivity (µS cm−1) and the water temperature (°C) variations, respectively. Panel (b) shows the seismic data recorded at ZO.PDN3 station (Table 2) on 31 October and 1 November 2023, while panel (c) shows the polarization analysis in terms of rotated horizontal-to-vertical spectral ratio.
Meteorological events, in particular the intense rainfall period, also seem to have a strong influence on radon emission measurements, usually adopted as a possible marker in case of a tectonic event. An example can be observed considering the complete radon time series at the Bondo site where data highlight a positive correlation with the seasonality, with increasing values in summer and decreasing values in winter, following the trend of both atmospheric pressure and temperature. At least during the monitored period, at the Bondo site no correlation with local seismicity appears to be noticeable, while clear correlations between radon outliers and the rainfall period are evident (Fig. 9a). It is worth mentioning how not all radon sensors show the same behaviour with respect to the season. At the Montelungo site (Table 1), for instance, data show a complete anti-correlation with the seasons (Fig. 9b), with lower values in summer and higher values in winter, probably as a consequence of the different local geological and morphological setting.
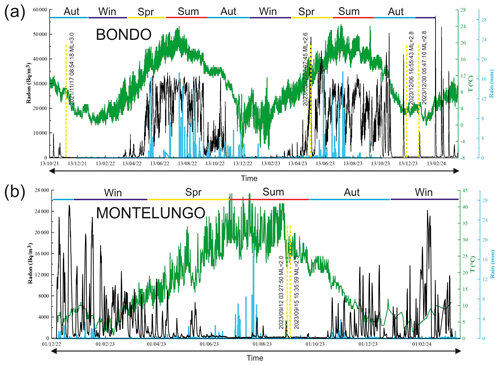
Figure 9Radon time series (Bq m−3, black lines) recorded at Bondo (a) and Montelungo (b) multiparametric sites. Soil temperature (°C, green lines) and rain (mm, light-blue lines) are also indicated. At the top of each panel, seasons are indicated (aut: autumn, win: winter, spr: spring, sum: summer).
A further example regards the CO2 flux variation, measured at the Nirano site (Table 1) in October 2023 (Fig. 10a) during an intense period of weak motion earthquakes localized very close to ZO.PDN10 station, installed in the area of the Salse di Nirano regional park. Starting from June 2023, the area of Nirano showed an increase in the local seismicity. In the period 1 June to 15 November 2023, 32 earthquakes with local magnitude (ML) in the range 2.0–3.5 were recorded with a maximum epicentral distance from Nirano of 30 km. In particular, the strong events with ML=3.5 occurred on 30 October 2023 (04:25:53 UTC). Considering the CO2 time series recorded at Nirano and a time period spanning 15 October to 15 November 2023, it is possible to highlight the presence of many CO2 data points with values exceeding the limit of +1 standard deviation (Fig. 10b), with respect to the average values of the period. Many outliers were recorded just before and also soon after the ML=3.5 target earthquake. It is worth noting that this evidence should be carefully evaluated also considering other parameters (for instance, atmospheric pressure, soil moisture and temperature), even though no relevant rainfall episodes occurred in that period.
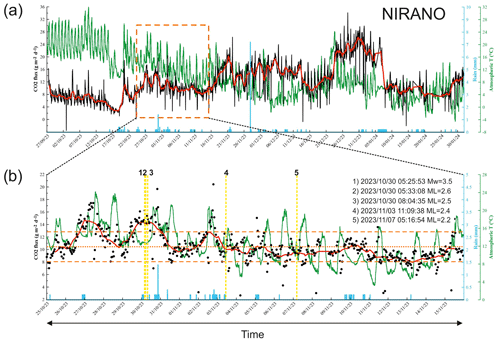
Figure 10Panel (a) shows the CO2 flux (g m−2 d−1, black line) recorded at NIRANO multiparametric site. Solid red and green lines indicate the smoothing function of CO2 flux and the temperature (°C), respectively. The orange box indicates the time window represented in the bottom panel. Panel (b) shows the detailed monitoring for the period 25 October to 15 November 2023, where a seismic sequence, with a maximum local magnitude of 3.5 (vertical dashed yellow lines), occurred at Nirano's mud volcanoes. Dotted and dashed orange lines indicate the mean values ±1 standard deviation of CO2 flux recorded during the analysed time period. Rain values (mm, light blue) and soil temperature (°C) are also indicated. Black dots indicate the CO2 measures (g m−2 d−1).
Further case studies concern the correlation between meteorological (i.e. temperature and rainfall) and groundwater parameters. Understanding water level fluctuation patterns is one of the pillars for designing adaptive management practices that can mitigate the impacts of extreme water levels on infrastructure and associated economic activities (e.g. Gerten et al., 2013; Alley et al., 2002; Taylor and Stefan, 2009; Russo and Lall, 2017). Groundwater recharge is difficult to estimate, especially in fractured aquifers, because of the spatial variability of the soil properties and because of the lack of data at basin scale. A possible solution consists in inferring recharge directly from the observation in boreholes (Guillaumot et al., 2022), even if the direct measures in wells overlook the impact of lateral groundwater redistribution in the aquifer. When evaluating the effect of exogenous parameters on groundwaters, rainfall is the main factor in promptly influencing all monitored groundwater parameters (e.g. Mancini et al., 2022; Guillaumot et al., 2022), with a variable aquifer response with respect comparable amounts of precipitation in the same period. Figure 11 shows an example of groundwater recordings related to the meteorological event of October 2023 at Volargne and Fonte sites (Table 1). It is worth noting how in the first site (Fig. 11a) a gradual and moderate rise in water level is contrasted by a faster and larger (less than 2 h) increase in water level at the Fonte site (Fig. 11b), which shows an intense influence of the rainfall, also proved by the extremely variable temperature and conductivity records not observed in the other sites (Ferrari et al., 2024). The light-grey box in Fig. 11 highlights another instant response of Fonte groundwater to precipitation, which is even more sharper than the one described above and also involves electrical conductivity decrease and temperature increase.
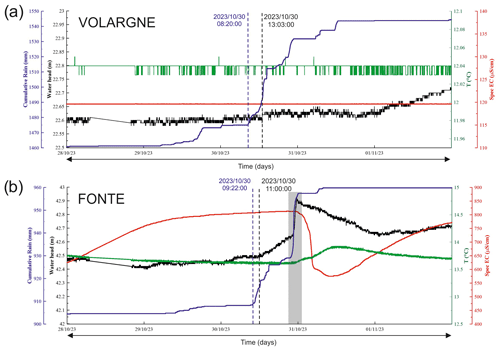
Figure 11Groundwater recharge at Volargne (a) and Fonte (b) sites (Table 1) recorded on 30 October 2023. In both panels, solid black, red and green lines indicate the water level (m), the electrical conductivity (µS cm−1) and the water temperature (°C) variations, respectively, while the solid blue lines indicate the cumulative rain.
The atmospheric temperature is moreover proven to affect groundwater temperature, especially in aquifers down to ∼ 20 m depth (e.g. Lee et al., 2021; Taylor and Stefan, 2009; Menberg et al., 2014). Monitoring sites with at least 1 year of recordings are taken into account to analyse groundwater temperature seasonal oscillations and correlations to air temperature. In our case, the absence of seasonality is detected at the Bondo, Montelungo and Volargne sites (Table 1) where the constant groundwater temperatures could be explained by the aquifer depth (∼ 50 m). In other cases, such as the Casaglia site (Table 1), despite the depth of aquifer of a few metres below ground level, the relevant water (∼ 40 m) column above the hydrogeochemical sensor dampened possible temperature fluctuations (Bucci et al., 2020; Egidio et al., 2022). Conversely, at the Balconi site (Table 1), despite the aquifer depth being greater than 50 m, a nearly seasonal variation, characterized by maximum values reached in summer and minima in winter, is observed, in agreement with the measured air temperature periodicity (Ferrari et al., 2024). However, it should be evaluated whether this temperature variation in specific seasons is directly attributable to meteorological reasons or to anthropogenic causes, due to intense irrigation occurring in the area of Balconi during the most dry and hot months of the year.
Data and metadata presented and described in this paper can be accessed under https://doi.org/10.13127/muda (Massa et al., 2023).
All the procedures to acquire and process data coming from multiparametric remote stations have been specifically developed for the MUDA project in Bash scripting language, Python and PHP language, using, when necessary, proprietary API taken from the manufacturers of the installed remote instrumentation, as detailed in the text. Seismic data are acquired and archived through a Seiscomp4 (https://doi.org/10.5880/GFZ.2.4.2020.003, Helmholtz-Centre Potsdam – GFZ German Research Centre for Geosciences and gempa GmbH, 2008) client, thereby improving the SeedLink real-time data acquisition protocol. Some processing steps on the seismic data are undertaken using the Seismic Analysis Code (SAC, https://ds.iris.edu/ds/nodes/dmc/software/downloads/sac/, Goldstein and Snoke, 2005), a software designed for both real-time and offline seismological analyses of time series data. The MUDA database is developed with MySQL open-source software. The MUDA web portal is developed in PHP and HTML5 languages, and all data are published under the Creative Commons License CC BY 4.0 licence.
The technical validation allowed us to obtain a reliable and homogeneous dataset of continuous multiparametric time series and associated metadata. Hydrogeochemical, meteorological, radon and CO2 data are published in a raw format after a pre-processing step, whose main scope was just to detect gaps and spurious peaks to be deleted from the time series. Seismic data are published after applying a 5 min resampling to the raw miniSEED 24 h continuous data and then by converting the velocity ground motion (cm s−1) in rms and FFT discrete time series. The raw seismic waveforms are however downloadable from the EIDA-Italia web service (https://eida.ingv.it/it/, last access: 15 October 2024).
For the first time, at least in Italy, high-frequency and continuous multiparametric data are dynamically updated daily and published soon after for end users. Data can be used for different purposes, ranging from (i) information regarding environmental and meteorological temporal trends with respect to the global climate change problematic; (ii) details on local aquifer features and seismicity; (iii) recommendations for the civil protection; and (iv) multiparametric geophysical, environmental and geochemical data for research studies. In particular, all seismic stations included in MUDA-db with the code ZO (PDnet, https://eida.ingv.it/it/networks/network/ZO, last access: 15 October 2024) contribute to the national seismic monitoring by sharing a continuous data stream in real time to the INGV National Seismic Network (https://eida.ingv.it/it/networks/network/IV, last access: 15 October 2024). In addition, the dense ZO network installed around Lake Garda contributes significantly to improving the minimum magnitude threshold detection of the area, as reported in Ferrari et al. (2024).
Seismic data, together with all geological, morphological and geophysical information collected at each site included in MUDA, can moreover be used to investigate the site response in terms of seismic amplification, in particular for sites installed in the central part of the Po Plain, a deep basin characterized by a significant thickness of incoherent alluvial deposits. Seismic events recorded at each station can also be used for local investigations into the microseismicity of the area and seismic source recognition or to improve the available seismic velocity models at a local scale.
Hydrogeochemical and geochemical data will be used in the framework of a recent agreement between the National Institute of Geophysics and Volcanology (INGV) and the National System for Environmental Protection (SNPA, comprising the Regional Environmental Protection Agencies – ARPA and ISPRA), aimed at gathering information on seismic activity and aquifer/spring status from various acquisition sources, in some cases reaching near-real-time monitoring through the SINAnet facility (https://www.snpambiente.it/attivita/sistema-informativo-nazionale-ambientale/, last access: 15 October 2024).
In general, the multiparametric monitoring is the basis by which to understand and identify possible seismic precursors, an objective not yet achieved in earthquake studies. In particular, the short-term earthquake forecasting remains elusive and largely unattained. An effective solution for such a major issue might be found, in the future, in systematic high-frequency and continuous measurements with multiparametric networks operating over the long term. Owing to the influx from deep crustal fluids in active tectonic areas, groundwater monitoring may especially be considered a fundamental tool for investigating pre-seismic signals of rocks undergoing accelerated strain (e.g. King, 1986; Skelton et al., 2014; Barberio et al., 2017).
Marco Massa (National Institute of Geophysics and Volcanology (INGV), Milan department, Milan, Italy), Andrea Luca Rizzo (National Institute of Geophysics and Volcanology (INGV), Milan department, Milan, Italy; DISAT, University of Milan Bicocca, Milan, Italy), Davide Scafidi (DISTAV, University of Genova, Genoa, Italy), Elisa Ferrari (National Institute of Geophysics and Volcanology (INGV), Milan department, Milan, Italy), Sara Lovati (National Institute of Geophysics and Volcanology (INGV), Milan department, Milan, Italy), Lucia Luzi (National Institute of Geophysics and Volcanology (INGV), Milan department, Milan, Italy), Marino Domenico Barberio (National Institute of Geophysics and Volcanology (INGV), Rome department, Rome, Italy), Gianfranco Tamburello (National Institute of Geophysics and Volcanology (INGV), Bologna department, Bologna, Italy), Gioia Capelli Ghioldi (National Institute of Geophysics and Volcanology (INGV), Bologna department, Bologna, Italy), Santi Mirenna (National Institute of Geophysics and Volcanology (INGV), Milan department, Milan, Italy), Ezio D'Alema (National Institute of Geophysics and Volcanology (INGV), Milan department, Milan, Italy), Anna Figlioli (National Institute of Geophysics and Volcanology (INGV), Milan department, Milan, Italy), Pellegrino Moretti (National Institute of Geophysics and Volcanology (INGV), Milan department, Milan, Italy), Claudio Ventura Bordenca (National Institute of Geophysics and Volcanology (INGV), Milan department, Milan, Italy), Fabio Varchetta (National Institute of Geophysics and Volcanology (INGV), Milan department, Milan, Italy), Antonio Piersanti (National Institute of Geophysics and Volcanology (INGV), Rome department, Rome, Italy), Valentina Cannelli (National Institute of Geophysics and Volcanology (INGV), Rome department, Rome, Italy), Gianfranco Galli G. (National Institute of Geophysics and Volcanology (INGV), Rome department, Rome, Italy), Rodolfo Puglia (National Institute of Geophysics and Volcanology (INGV), Milan department, Milan, Italy).
This study started from an original idea by MM and ALR. MM, ALR, EF and SL contributed to all phases of site installations, data acquisition, processing and archiving. DS developed MySQL-Db and the associated web page, as well as the procedures to upload data into MUDA-db. SL and EF contributed to organizing the technical data sheet related to each multiparametric site, now available at each single station web page. MM developed the procedures for multiparametric data pre-processing and seismic data post processing. All authors participated in the preparation of the manuscript draft and in the phases of revision.
The contact author has declared that none of the authors has any competing interests.
Publisher's note: Copernicus Publications remains neutral with regard to jurisdictional claims made in the text, published maps, institutional affiliations, or any other geographical representation in this paper. While Copernicus Publications makes every effort to include appropriate place names, the final responsibility lies with the authors.
We would like to thank all those who contributed to the site search phase and the installation of the PDnet network, in particular Tullia Bonomi (University of Milan Bicocca), Michela Biasibetti and Bruno Pannuzzo (Acque Bresciane company), Massimo Carmagnani and Ignazio Leone (Acque Veronesi company), Giovanni Lepore and Fabrizio Brunello (Azienda Gardesana Servizi company), Paolo Pizzaia (Alto Trevigiano Servizi company), Luca Bertanza (municipality of Tremosine Garda, BS), Umberto Minuta (municipality of Dolcè, VR), Elena Beraldini (municipality of Negrar, VR), Marzia Conventi (municipality of Fiorano Modenese, MO – Dir. Riserva Salse di Nirano), Salviani (Norcia), Bagni Triponzo Terme S.p.A. (municipality of Cerreto di Spoleto), and Luca Martelli and Paolo Severi (Emilia Romagna Region). Particular thanks go to OGS (National Institute of Oceanography and Applied Geophysics, https://www.ogs.it/en, last access: 15 October 2024) and University of Ferrara (Department of Physics and Earth Science, http://fst.unife.it/en, last access: 15 October 2024) for sharing data recorded at the Toppo site in the framework of the ECCSEL ERIC (European Research Infrastructure for CO2 Capture, Utilisation, Transport and Storage (CCUS), https://www.eccsel.org/, last access: 15 October 2024) consortium.
The production of the seismic data published in the MUDA database involves many INGV colleagues covering different tasks in the data production chain, from the correct operation of the seismic stations to data acquisition, data processing, archiving and subsequent distribution. We would like to thank all the colleagues of the INGV sections and offices who contribute daily to the management of the National Seismic Network (RSN, https://eida.ingv.it/it/networks/network/IV, last access: 15 October 2024), as well as providing access to the data they produce. Finally, we thank the ESSD topic editor, the reviewers, Simona Petrosino, Galina N. Kopylova and Polina Lemenkova, and the ESSD editorial team.
This research has been supported by the Istituto Nazionale di Geofisica e Vulcanologia (Institutional funds).
This paper was edited by Giulio G. R. Iovine and reviewed by Polina Lemenkova, Galina N. Kopylova, and Simona Petrosino.
Aiuppa, A., Federico, C., Giudice, G., and Gurrieri, S.: Chemical mapping of a fumarolic field: La Fossa Crater, Vulcano Island (Aeolian Islands, Italy), Geophys. Res. Lett., 32, 1–4, https://doi.org/10.1029/2005GL023207, 2005.
Allard, P., Burton, M., and Murè, F.: Spectroscopic evidence for a lava fountain driven by previously accumulated magmatic gas, Nature, 433, 407–410, https://doi.org/10.1038/nature03246, 2005.
Alley, W. M., Healy, R. W., LaBaugh, J. W., and Reilly, T. E.: Flow and storage in groundwater systems, Science, 296, 1985–1990, https://doi.org/10.1126/science.1067123, 2002.
Barberio, M. D., Barbieri, M., Billi, A., Doglioni, C., and Petitta, M.: Hydrogeochemical changes before and during the 2016 Amatrice Norcia seismic sequence (central Italy), Sci. Rep.-UK, 7, 11735, https://doi.org/10.1038/s41598-017-11990-8, 2017.
Battaglia, M., Murray, M. H., Serpelloni, E., and Bürgmann, R.: The Adriatic region: an in dependent microplate within the Africa-Eurasia collision zone, Geophys. Res. Lett., 31, L09605, https://doi.org/10.1029/2004GL019723, 2004.
Bigi, G., Costantino, D., Parotto, M., Sartori, R., and Scandone, P.: Structural Model of Italy. Firenze, Società Elaborazioni Cartografiche (S.EL.CA.), Consiglio Nazionale Delle Ricerche Progetto Finalizzato Geodinamica, Scala 1:500.000, 9 Fogli, 1990.
Bonini, M.: Elliptical mud volcano caldera as stress indicator in an active compressional setting (Nirano, Pede-Apennine margin, northern Italy), Geology 36, 131–134, https://doi.org/10.1130/G24158A.1, 2008.
Bormann, P.: New manual of seismological observatory practice (NMSOP-2), IASPEI, GFZ German Research Centre for Geosciences, Potsdam, https://doi.org/10.2312/GFZ.NMSOP-2, 2012.
Bräuer, K., Kämpf, H., Strauch, G., and Weise, S. M.: Isotopic evidence (3He/4He, 13C) of fluid-triggered intraplate seismicity, J. Geophys. Res., 108, 1–11, https://doi.org/10.1029/2002JB002077, 2003.
Brozzetti, F. and Lavecchia, G.: Seismicity and related extensional stress field: the case of the Norcia Seismic Zone (Central Italy), Annales Tectonicae, 8, 36–57, 1994.
Bucci, A., Lasagna, M., De Luca, D. A., Acquaotta, F., Barbero, D., and Fratianni, S.: Time series analysis of underground temperature and evaluation of thermal properties in a test site of the Po plain (NW Italy), Environ. Earth Sci., 79, 185, https://doi.org/10.1007/s12665-020-08920-9, 2020.
Burrato, P., Vannoli, P., Fracassi, U., Basili, R., and Valensise, G.: Is blind faulting truly invisible? Tectonic-controlled drainage evolution in the epicentral area of the May 2012, Emilia-Romagna earthquake sequence (northern Italy), Ann. Geophys., 55, 525–531, https://doi.org/10.4401/ag-6182, 2012.
Burton, M. R., Caltabiano, T., Murè, F., Salerno, G. G., and Randazzo, D.: SO2 flux from Stromboli during the 2007 eruption: results from the FLAME network and traverse measurements, J. Volcanol. Geotherm. Res., 182, 214–220, https://doi.org/10.1016/j.jvolgeores.2008.11.025, 2009.
Buttitta, D., Caracausi, A., Chiaraluce, L., Favara, R., Morticelli, M. G., and Sulli, A.: Continental degassing of helium in an active tectonic setting (northern Italy): the role of seismicity, Sci. Rep., 10, 162, https://doi.org/10.1038/s41598-019-55678-7, 2020.
Cannelli, V., Piersanti, A., Galli, G., and Melini, D.: Italian Radon mOnitoring Network (IRON): A permanent network for near real-time monitoring of soil radon emission in Italy, Ann. Geophys., 61, SE444, https://doi.org/10.4401/ag-7604, 2018.
Carapezza, M. L., Inguaggiato, S., Brusca, L., and Longo, M.: Geochemical precursors of Stromboli 2002–2003 eruptive events, Geophys. Res. Lett., 31, L07620, https://doi.org/10.1029/2004GL019614, 2004.
Castaldini, D., Valdati, J., Ilies, D. C., Chiriac, C., and Bertogna, I.: Geo-tourist map of the natural reserve of Salse di Nirano (Modena Apennines, Northern Italy), Italian J. Quaternary Sci., 18, 245–255, 2005.
Cicerone, R. D., Ebel, J. E., and Britton, J.: A systematic compilation of earthquake precursors, Tectonophysics, 476, 371–396, https://doi.org/10.1016/j.tecto.2009.06.008, 2009.
Chiarabba, C., Piccinini, D., and De Gori, P.: Velocity and attenuation tomography of the Umbria Marche 1997 fault system: Evidence of a fluid-governed seismic sequence, Tectonophysics, 476, 73–84, https://doi.org/10.1016/j.tecto.2009.04.004, 2009.
Chiaraluce, L., Collettini, C., Cattaneo, M., and Monachesi, G.: The shallow boreholes at The AltotiBerina near fault Observatory (TABOO; northern Apennines of Italy), Sci. Dril., 17, 31–35, https://doi.org/10.5194/sd-17-31-2014, 2014.
Chiaraluce, L., Di Stefano, R., Tinti, E., Scognamiglio, L., Michele, M., Casarotti, E., Cattaneo, M., De Gori, P., Chiarabba, C., Monachesi, G., Lombardi, A., Valoroso, L., Latorre, D., and Marzorati, S.: The 2016 Central Italy Seismic Sequence: A First Look at the Mainshocks, Aftershocks, and Source Models, Seismol. Res. Lett., 88, 757–771, https://doi.org/10.1785/0220160221, 2017.
Chiodini, G., Cioni, R., Guidi, M., Raco, B., and Marini, L.: Soil CO2 flux measurements in volcanic and geothermal areas, Appl. Geochem., 13, 543–552, https://doi.org/10.1016/S0883-2927(97)00076-0, 1998.
Chiodini, G., Cardellini, C., Di Luccio, F., Selva, J., Frondini, F., Caliro, S., Rosiello, A., Beddini, G., and Ventura, G.: Correlation between tectonic CO2 Earth degassing and seismicity is revealed by a 10-year record in the Apennines, Italy, Sci. Adv., 6, eabc2938, https://doi.org/10.1126/sciadv.abc2938, 2020.
Comerci, V., Doglioni, C., Italiano, F., Baiocco, F., Barberio, M. D., Caracausi, A., Cuiuli, E., Guerra, M., Infantino, V., Insolvibile, M., Marcaccio, M., Martinelli, G., Menichetti, S., Onorati, G., Petitta, M., Palumbo, V., Peleggi, M., Richieri, F., Scaramella, A., Scotti, E., and Testa, M.: Towards a national hydrogeochemical monitoring system: a further tool to investigate geological hazards, Misc. INGV, 49, 1–338, 2019.
D'Agostino, N., Avallone, A., Cheloni, D., D'Anastasio, E., Mantenuto, S., and Selvaggi, G.: Active tectonics of the Adriatic region from GPS and earthquake slip vectors, Jour. Geophys. Res., 113, B12413, https://doi.org/10.1029/2008JB005860, 2008.
D'Alessandro, A., Scudero, S., Siino, M., Alessandro, G., and Mineo, R.: Long-term monitoring and characterization of soil radon emission in a seismically active area, Geochem. Geophy. Geosy., 21, e2020GC009061, https://doi.org/10.1029/2020GC009061, 2020.
Danecek, P., Pintore, S., Mazza, S., Mandiello, A., Fares, M., Carluccio, I., Della Bina, E., Franceschi, D., Moretti, M., Lauciani, V., Quintiliani, M., and Michelini, A.: The Italian node of the European integrated data archive, Seismol. Soc. Am., 92, 1726–1737, doi:https://doi.org/10.1785/0220200409, 2021.
Danesi, S., Pondrelli, S., Salimbeni, S., Cavaliere, A., Serpelloni, E., Danecek P., Lovati, S., and Massa, M.: Active deformation and seismicity in the Southern Alps (Italy): The Montello hill as a case study, Tectonophysics, 653, 95–108, https://doi.org/10.1016/j.tecto.2015.03.028, 2015.
De Gregorio, S., Gurrieri, S., and Valenza, M.: A PTFE membrane for the in situ extraction of dissolved gases in natural waters: Theory and applications, Geochem. Geophys. Geosy., 6, Q09005, https://doi.org/10.1029/2005GC000947, 2005.
De Gregorio, S., Federico, C., Cappuzzo, S., Favara, R., Giudice, G., Gurrieri, S., and Boschi, E.: Stress-induced temperature variations in groundwater of the Monferrato area (north-western Italy), Geofluids, 12, 142–149, https://doi.org/10.1111/j.1468-8123.2011.00348.x, 2012.
De Luca, G., Di Carlo, G., and Tallini, M.: A record of changes in the Gran Sasso groundwater before, during and after the 2016 Amatrice earthquake, central Italy, Sci. Rep.-UK 8, 15982, 1–16, https://doi.org/10.1038/s41598-018-34444-1, 2018.
De Matteis, R., Convertito, V., Napolitano, F., Amoroso, O., Terakawa, T., and Capuano, P.: Pore fluid pressure imaging of the Mt. Pollino region (southern Italy) from earthquake focal mechanisms, Geophys. Res. Lett., 48, 2021GL094552, https://doi.org/10.1029/2021GL094552, 2021.
Di Luccio, F., Ventura, G., Di Giovambattista, R., Piscini, A., and Cinti, F. R.: Normal faults and thrusts reactivated by deep fluids: The 6 April 2009 Mw 6.3 L'Aquila earthquake, central Italy, J. Geophys. Res., 115, 1–15, https://doi.org/10.1029/2009JB007190, 2010.
DISS Working Group: Database of Individual Seismogenic Sources (DISS), Version 3.3.0: A compilation of potential sources for earthquakes larger than M 5.5 in Italy and surrounding areas, Istituto Nazionale di Geofisica e Vulcanologia (INGV), https://doi.org/10.13127/diss3.3.0, 2021.
Egidio, E., Mancini, S., De Luca, D. A., and Lasagna, M.: The Impact of Climate Change on Groundwater Temperature of the Piedmont Po Plain (NW Italy), Water, 14, 2797, https://doi.org/10.3390/w14182797, 2022.
Ferrari, E., Massa, M., Rizzo, A. L., Lovati, S., and Di Michele F.: Multiparametric stations for real-time monitoring and long-term assessment of natural hazards, Front. Earth Sci., 12, 1412900, https://doi.org/10.3389/feart.2024.1412900, 2024.
Gabrielli, S., Akinci, A., Ventura, G., Napolitano, F., Del Pezzo, E., and De Siena, L.: Fast changes in seismic attenuation of the upper crust due to fracturing and fluid migration: the 2016–2017 Central Italy seismic sequence, Front. Earth Sci., 10, 909698, https://doi.org/10.3389/feart.2022.909698, 2022.
Gabrielli, S., Akinci, A., De Siena, L., Del Pezzo, E., Buttinelli, M., Maesano, F. E., and Maffucci, R.: Scattering attenuation images of the control of thrusts and fluid overpressure on the 2016–2017 Central Italy seismic sequence, Geophys. Res. Lett., 50, e2023GL103132, https://doi.org/10.1029/2023GL103132, 2023.
Galadini, F. and Galli, P.: The Holocene paleoearthquakes on the 1915 Avezzano earthquake faults (central Italy): implications for active tectonics in the central Apennines, Tectonophysics, 308, 143–170, https://doi.org/10.1016/S0040-1951(99)00091-8, 1999.
Galadini, F. and Messina, P.: Plio-Quaternary changes of the normal fault architecture in the Central Apennines (Italy), Geodinam. Ac., 14, 321–344, https://doi.org/10.1080/09853111.2001.10510727, 2001.
Galli, P., Galderisi, A., Ilardo, I., Piscitelli, S., Scionti, V., Bellanova, J., and Calzoni, F.: Holocene paleoseismology of the Norcia fault system (central Italy), Tectonophysics, 745, 154–169, https://doi.org/10.1016/j.tecto.2018.08.008, 2018.
Galli, P., Galderisi, A., Peronace, E., Giaccio, B., Hajdas, I., Messina, P., Pileggi, D., and Polpetta, F.: The Awakening of the Dormant Mount Vettore Fault (2016 Central Italy Earthquake, Mw 6.6): Paleoseismic Clues on Its Millennial Silences, Tectonics, 38, 687–705, https://doi.org/10.1029/2018TC005326, 2019.
Gerten, D., Hoff, H., Rockström, J., Jägermeyr, J., Kummu, M., and Pastor, A. V.: Towards a revised planetary boundary for consumptive freshwater use: role of environmental flow requirements, Environ. Sustain., 5, 551–558, https://doi.org/10.1016/j.cosust.2013.11.001, 2013.
Giambastiani, B. M. S., Chiapponi, E., Polo, F., Nespoli, M., Piombo, A., and Antonellini, M.: Structural control on carbon emissions at the Nirano mud volcanoes – Italy, Mar. Petrol. Geol., 163, 106771, https://doi.org/10.1016/j.marpetgeo.2024.106771, 2024.
Goldstein, P. and Snoke, A.: SAC Availability for the IRIS Community, Incorporated Research Institutions for Seismology Newsletter [code], https://ds.iris.edu/ds/nodes/dmc/software/downloads/sac/ (last access: 15 October 2024), 2005.
Goldstein, P., Dodge, D., Firpo, M., and Minner, L.: SAC2000: Signal processing and analysis tools for seismologists and engineers, Invited contribution to “The IASPEI International Handbook of Earthquake and Engineering Seismology”, edited by: Lee, W. H. K., Kanamori, H., Jennings, P. C., and Kisslinger, C., Academic Press, London, https://doi.org/10.1016/S0074-6142(03)80284-X, 2003.
Gori, F. and Barberio, M. D.: Hydrogeochemical changes before and during the 2019 Benevento seismic swarm in central-southern Italy, J. Hydrol., 604, 127250, https://doi.org/10.1016/j.jhydrol.2021.127250, 2022.
Guillaumot, L., Longuevergne, L., Marçais, J., Lavenant, N., and Bour, O.: Frequency domain water table fluctuations reveal impacts of intense rainfall and vadose zone thickness on groundwater recharge, Hydrol. Earth Syst. Sci., 26, 5697–5720, https://doi.org/10.5194/hess-26-5697-2022, 2022.
Helmholtz-Centre Potsdam – GFZ German Research Centre for Geosciences and gempa GmbH: The SeisComP seismological software package, GFZ Data Services [code and data set], https://doi.org/10.5880/GFZ.2.4.2020.003, 2008.
Hubbert, M. K. and Rubey, W. W.: Role of fluid pressure in mechanics of overthrust faulting: mechanics of fluid-filled porous solids and its application to overthrust faulting, Geol. Soc. Am. Bull., 70, 115–166, https://doi.org/10.1130/0016-7606(1959)70[115:ROFPIM]2.0.CO;2, 1959.
Improta, L., Latorre, D., Margheriti, L., Nardi, A., Marchetti, A., Lombardi, A. M., Castello, B., Villani, F., Ciaccio, M. G., Mele, F. M., Moretti, M., and The Bollettino Sismico Italiano Working Group: Multi-segment rupture of the 2016 Amatrice-Visso-Norcia seismic sequence (central Italy) constrained by the first high-quality catalog of Early Aftershocks, Sci. Rep., 9, 6921, https://doi.org/10.1038/s41598-019-43393-2, 2019.
Inguaggiato, S., Vita, F., Rouwet, D., Bobrowski, N., Morici, S., and Sollami, A.: Geochemical evidence of the renewal of volcanic activity inferred from CO2 soil and SO2 plume fluxes: the 2007 Stromboli eruption (Italy), B. Volcanol., 73, 443–456, https://doi.org/10.1007/s00445-010-0442-z, 2011a.
Inguaggiato, S., Calderone, L., Inguaggiato, C., Morici, S., and Vita, F.: Dissolved CO2 in natural waters: development of an automated monitoring system and first application to Stromboli volcano (Italy), Ann. Geophys., 54, 209–218, https://doi.org/10.4401/ag-5180, 2011b.
Italiano, F., Martinelli, G., and Nuccio, P. M.: Anomalies of mantle-derived helium during the 1997–1998 seismic swarm of Umbria-Marche, Italy, Geophy. Res. Lett., 28, 839–842, https://doi.org/10.1029/2000GL012059, 2001.
Italiano, F., Martinelli, G., and Rizzo, A.: Geochemical evidence of seismogenic-induced anomalies in the dissolved gases of thermal waters: A case study of Umbria (Central Apennines, Italy) both during and after the 1997–1998 seismic swarm, Geochem. Geophy. Geosy., 5, 1–11, https://doi.org/10.1029/2004GC000720, 2004.
Keranen, K. M. and Weingarten, M.: Induced seismicity, Annu. Rev. Earth Pl. Sc., 46, 149–174, https://doi.org/10.1146/annurev-earth-082517-010054, 2018.
King, C. Y.: Gas geochemistry applied to earthquake prediction: An overview, J. Geophys. Res., 91, 12269–12281, https://doi.org/10.1029/JB091iB12p12269, 1986.
Lai, C. G., Poggi, V., Famà, A., Zuccolo, E., Bozzoni, F., Meisina, C., Bonì, R., Martelli, L., Massa, M., Mascandola, C., Petronio, L., Affatato, A., Baradello, L., Castaldini, D., and Cosentini, R. M.: An inter-disciplinary and multi-scale approach to assess the spatial variability of ground motion for seismic microzonation: the case study of Cavezzo municipality in Northern Italy, Eng. Geol., 274, 105722, https://doi.org/10.1016/j.enggeo.2020.105722, 2020.
Lee, H. A., Hamm, S. Y., and Woo, N. C.: Pilot-scale groundwater monitoring network for earthquake surveillance and forecasting research in Korea, Water, 13, 2448, https://doi.org/10.3390/w13172448, 2021.
Locati, M., Camassi, R., Rovida, A., Ercolani, E., Bernardini, F., Castelli, V., Caracciolo, C. H., Tertulliani, A., Rossi, A., Azzaro, R., D'Amico, S., Conte, S., Rocchetti, E., and Antonucci, A.: Database Macrosismico Italiano (DBMI15), versione 4.0,Istituto Nazionale di Geofisica e Vulcanologia (INGV), https://doi.org/10.13127/dbmi/dbmi15.4, 2022.
Luzi, L., Pacor, F., Ameri, G., Puglia, R., Burrato, P., Massa, M., Augliera, P., Castro, R., Franceschina, G., and Lovati, S.: Overview on the strong motion data recorded during the May-June 2012 Emilia seismic sequence, Seismol. Res. Lett., 84, 629–644, https://doi.org/10.1785/0220120154, 2013.
Luzi, L., Puglia, R., Russo, E., D’Amico, M., Felicetta, C., Pacor, F., Lanzano, G., Çeken, U., Clinton, J., Costa, G., Duni, L., Farzanegan, E., Gueguen, G., Ionescu, C., Kalogeras, I., Özener, H., Pesaresi, D., Sleeman, R., Strollo, A., and Zare, M.: The European strong-motion database: a platform to access accelerometric data, Seismol. Res. Lett., 87, 987–997, https://doi.org/10.1785/0220150278, 2016.
Malagnini, L., Pio Lucente, F., De Gori, P., Akinci, A., and Munafo', I.: Control of pore fluid pressure diffusion on fault failure mode: Insights from the 2009 L'Aquila seismic sequence, J. Geophys. Res.-Sol. Ea., 117, 1–15, https://doi.org/10.1029/2011JB008911, 2012.
Mancini, S., Egidio, E., De Luca, D. A., and Lasagna, M.: Application and comparison of different statistical methods for the analysis of groundwater levels over time: Response to rainfall and resource evolution in the Piedmont Plain (NW Italy), Sci. Total Environ., 846, 157479, https://doi.org/10.1016/j.scitotenv.2022.157479, 2022.
Marchetti, A., Ciaccio, M. G., Nardi, A., Bono, A., Mele, F. M., Margheriti, L., Rossi, A., Battelli, P., Melorio, C., Castello, B., Lauciani, V., Berardi, M., Castellano, C., Arcoraci, L., Lozzi, G., Battelli, A., Thermes, C., Pagliuca, N., Modica, G., Lisi, A., Pizzino, L., Baccheschi, P., Pintore, S., Quintiliani, M., Mandiello, A., Marcocci, C., Fares, M., Cheloni, D., Frepoli, A., Latorre D., Lombardi, A.M., Moretti, M., Pastori, M., Vallocchia, M., Govoni, A., Scognamiglio, L., Basili, A., Michelini, A., and Mazza, S.: The Italian Seismic Bulletin: strategies, revised pickings and locations of the central Italy seismic sequence, Ann. Geophys., 59, 1–7, https://doi.org/10.4401/ag-7169, 2016.
Margheriti, L., Nostro, C., Cocina, O., Castellano, M., Moretti, M., Lauciani, V., Quintiliani, M., Bono, A., Mele, F.M., Pintore, S., Montalto, P., Peluso, R., Scarpato, G., Rao, S., Alparone, S., Di Prima, S., Orazi, M., Piersanti, A., Cecere, G., Cattaneo, M., Vicari, A., Sepe, V., Bignami, C., Valoroso, L., Aliotta, M., Azzarone, A., Baccheschi, P., Benincasa, A., Bernardi, F., Carluccio, I., Casarotti, E., Cassisi, C., Castello, B., Cirilli, F., D'Agostino, M., D'Ambrosio, C., Danecek, P., De Cesare, W., Della Bina, E., Di Filippo, A., Di Stefano, R., Faenza, L., Falco, L., Fares, M., Ficeli, P., Latorre, D., Lorenzino, M.C., Mandiello, A., Marchetti, A., Mazza, S., Michelini, A., Nardi, A., Pastori, M., Pignone, M., Prestifilippo, M., Ricciolino, P., Sensale, G., Scognamiglio, L., Selvaggi, G., Torrisi, O., Zanolin, F., Amato, A., Bianco, F., Branca, S., Privitera, E., and Stramondo, S.: Seismic Surveillance and Earthquake Monitoring in Italy, Seismol. Res. Lett., 92, 1659–1671, https://doi.org/10.1785/0220200380, 2021.
Martinelli, G.: Contributions to a history of earthquake prediction research, in: Pre-earthquake processes: a multidisciplinary approach to earthquake prediction studies, editod by: Ouzounov, D., Pulinets, S., Hattori, K., Taylor, P. John Wiley & Sons, https://doi.org/10.1002/9781119156949.ch5, 2018.
Martinelli, G., Ciolini, R., Facca, G., Fazio, F., Gherardi, F., Heinicke, J., and Pierotti, L.: Tectonic-related geochemical and hydrological anomalies in Italy during the last fifty years, Minerals, 11, 1–16, https://doi.org/10.3390/min11020107, 2021.
Massa, M., Scafidi, D., Mascandola, C., and Lorenzetti, A.: Introducing ISMDq – A Web Portal for Real-Time Quality Monitoring of Italian Strong-Motion Data, Seismol. Res. Lett., 93, 241–256, https://doi.org/10.1785/0220210178, 2022a.
Massa, M., Lovati, S., Puglia, R., Brunelli, G., Lorenzetti, A., Mascandola, C., Felicetta, C., Pacor, F., and Luzi, L.: Seismo-Stratigraphic Model for the Urban Area of Milan (Italy) by Ambient-Vibration Monitoring and Implications for Seismic Site Effects Assessment, Front. Earth Sci., 10, 915083, https://doi.org/10.3389/feart.2022.915083, 2022b.
Massa, M., Rizzo, L. A., Ferrari, E., Lovati, S., Scafidi, D., Puglia, R., Varchetta, F., D'Alema, E., Mirenna, S., and Luzi, L.: MUDA geophysical and geochemical MUltiparametric DAtabase, MUDA [data set], https://doi.org/10.13127/muda, 2023.
Mastrorillo, L., Saroli, M., Viaroli, F., Banzato, F., Valigi, D., and Petitta, M.: Sustained post-seismic effects on groundwater flow in fractured carbonate aquifers in Central Italy, Hydrol. Process., 34, 1167–1181, https://doi.org/10.1002/hyp.13662, 2020.
Mazzoli, S., Santini, S., Macchiavelli, C., and Ascione, A.: Active tectonics of the outer northern Apennines: Adriatic vs. Po Plain seismicity and stress fields, J. Geodynam., 84, 62–76, https://doi.org/10.1016/j.jog.2014.10.002, 2015.
McNamara, D. E. and Buland, R. P.: Ambient Noise Levels in the Continental United States, B. Seismol. Soc. Am., 94, 1517–1527, https://doi.org/10.1785/012003001, 2004.
Menberg, K., Blum, P., Kurylyk, B. L., and Bayer, P.: Observed groundwater temperature response to recent climate change, Hydrol. Earth Syst. Sci., 18, 4453–4466, https://doi.org/10.5194/hess-18-4453-2014, 2014.
Michelini, A., Faenza, L., Lanzano, G., Lauciani, V., Jozinović D., Puglia, R., and Luzi, L.: The New ShakeMap in Italy: Progress and Advances in the Last 10 Yr, Seismol. Res. Lett., 91, 317–333, https://doi.org/10.1785/0220190130, 2020.
Miller, S. A., Collettini, C., Chiaraluce, L., Cocco, M., Barchi, M., and Kaus, B. J. P.: Aftershocks driven by a high-pressure CO2 source at depth, Nature, 427, 724–727, https://doi.org/10.1038/nature02251, 2004.
Ministero delle Infrastrutture e dei Trasporti Aggiornamento delle Norme Tecniche per le Costruzioni. Part 3.2.2: Categorie di sottosuolo e condizioni topografiche, Gazzetta Ufficiale n. 42, 2018 (in Italian).
Nakamura, Y.: A method for dynamic characteristics estimation of subsurface using microtremor on the ground surface, Q. Rep. Railway Tech. Res. Inst., 30, 25–33, 1989.
Napolitano, F., De Siena, L., Gervasi, A., Guerra, I., Scarpa, R., and La Rocca, M.: Scattering and absorption imaging of a highly fractured fluid-filled seismogenetic volume in a region of slow deformation, Geosci. Front., 11, 989–998, https://doi.org/10.1016/j.gsf.2019.09.014, 2020.
Pacor, F., Paolucci, R., Ameri, G., Massa, M., and Puglia, R.: Italian strong motion records in ITACA: Overview and record processing, Bull. Earthq. Eng., 9, 1741–1759, https://doi.org/10.1007/s10518-011-9295-x, 2011.
Pessina, V. and Fiorini, E.: A GIS procedure for fast topographic characterization of seismic recording stations, Soil Dynam. Earthq. Eng., 63, 248–258, https://doi.org/10.1016/j.soildyn.2014.04.002, 2014.
Pieri, M. and Groppi, G.: Subsurface Geological Structure of the Po Plain, Italy. Progetto Finalizzato Geodinamica/Sottoprogetto “Modello Strutturale”, Rome, Consiglio Nazionale delle Ricerche Publ. No. 414, 1981.
Pondrelli, S., Ekström, G., and Morelli, A.: Seismotectonic re-evaluation of the 1976 Friuli, Italy, seismic sequence, J. Seismol., 5, 73–83, https://doi.org/10.1023/A:1009822018837, 1999.
Rikitake, T. and Hamada, K.: Earthquake prediction, Encyclopaedia of Physical Science and Technology, 3rd edn., Academic Press, San Diego, CA, USA, 4, 743–760, https://doi.org/10.1016/B0-12-227410-5/00195-2, 2003.
Rizzo, A. L., Federico, C., Inguaggiato, S., Sollami, A., Tantillo, M., Vita, F., Bellomo, S., Longo, M., Grassa, F., and Liuzzo, M.: The 2014 Effusive Eruption at Stromboli Volcano (Italy): Inferences From Soil CO2 Flux and 3He/4He Ratio in Thermal Waters, Geophys. Res. Lett., 42, 2235–2243, https://doi.org/10.1002/2014GL062955, 2015.
Rovida, A., Locati, M., Camassi, R., Lolli, B., and Gasperini, P.: The Italian Earthquake Catalogue CPTI15, Bull. Earthq. Eng., 18, 2953–2984, https://doi.org/10.1007/s10518-020-00818-y, 2020.
Russo, T. A. and Lall, U.: Depletion and response of deep groundwater to climate-induced pumping variability, Nat. Geosci., 10, 105–108, https://doi.org/10.1038/ngeo2883, 2017.
Scognamiglio, L., Tinti, E., Michelini, A., Dreger, D. S., Cirella, A., Cocco, M., Salvatore, M., and Piatanesi, A.: Fast Determination of Moment Tensors and Rupture History: What Has Been Learned from the 6 April 2009 L'Aquila Earthquake Sequence, Seismol. Res. Lett, 81, 892–906, https://doi.org/10.1785/gssrl.81.6.892, 2009.
Serpelloni, E., Anzidei, M., Baldi, P., Casula, G., and Galvani, A.: Crustal Velocity and Strain-Rate fields in Italy and Surrounding Regions: New Results From the Analysis of Permanent and Non- Permanent GPS Networks, Geophys. J. Int., 161, 861–880, https://doi.org/10.1111/j.1365-246X.2005.02618.x, 2005.
Shinohara, H.: A new technique to estimate volcanic gas composition: plume measurements with a portable multi-sensor system, J. Volcanol. Geotherm. Res., 143, 319–333, https://doi.org/10.1016/j.jvolgeores.2004.12.004, 2005.
Skelton, A., Andrén, M., Kristmannsdóttir, H., Stockmann, G., Mörth, C.-M., Sveinbjörnsdóttir, Á., Jónsson, S., Sturkell, E., Guðrúnardóttir, H. R., Hjartarson, H., Siegmund, H., and Kockum, I.: Changes in groundwater chemistry before two consecutive earthquakes in Iceland, Nat. Geosci., 7, 752–756, 2014.
Stucchi, M., Meletti, C., Montaldo, V., Crowley, H., Calvi, G. M., and Boschi, E.: Seismic Hazard Assessment (2003-2009) for the Italian building code, B. Seismol. Soc. Am., 101, 1885–1911, https://doi.org/10.1785/0120100130, 2011.
Taylor, C. A. and Stefan, H. G.: Shallow groundwater temperature response to climate change and urbanization, J. Hydrol., 375, 601–612, https://doi.org/10.1016/j.jhydrol.2009.07.009, 2009.
Ventura, G. and Di Giovambattista, R.: Fluid pressure, stress field and propagation style of coalescing thrusts from the analysis of the 20 May 2012 ML 5.9 Emilia earthquake (Northern Apennines, Italy), Terra Nova, 25, 72–78, https://doi.org/10.1111/ter.12007, 2012.
- Abstract
- Introduction
- Seismotectonic framework and seismicity
- State of the art
- MUDA database
- Data records
- Multiparametric monitoring
- Data availability
- Code availability
- Usage notes and conclusions
- Team list
- Author contributions
- Competing interests
- Disclaimer
- Acknowledgements
- Financial support
- Review statement
- References
- Abstract
- Introduction
- Seismotectonic framework and seismicity
- State of the art
- MUDA database
- Data records
- Multiparametric monitoring
- Data availability
- Code availability
- Usage notes and conclusions
- Team list
- Author contributions
- Competing interests
- Disclaimer
- Acknowledgements
- Financial support
- Review statement
- References