the Creative Commons Attribution 4.0 License.
the Creative Commons Attribution 4.0 License.
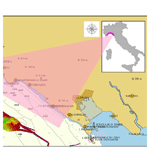
Deep-water hydrodynamic observations around a cold-water coral habitat in a submarine canyon in the eastern Ligurian Sea (Mediterranean Sea)
Tiziana Ciuffardi
Zoi Kokkini
Maristella Berta
Marina Locritani
Andrea Bordone
Ivana Delbono
Mireno Borghini
Maurizio Demarte
Roberta Ivaldi
Federica Pannacciulli
Anna Vetrano
Davide Marini
Giovanni Caprino
A 2-year dataset of a stand-alone mooring, deployed in November 2020 down the Levante Canyon in the eastern Ligurian Sea, is presented. The Levante Canyon Mooring (LCM) is a deep submarine multidisciplinary observatory positioned at 608 m depth in a key ecosystem area. The Levante Canyon hosts a valuable and vulnerable ecosystem of deep-living cold-water corals (CWCs), studied and monitored since 2013 through integrated mapping of the seabed and water column. The 2-year dataset, acquired on the mooring and presented here (data from November 2020 to October 2022), includes measurements conducted with both current meters and conductivity–temperature–depth (CTD) probes and provides information about the hydrodynamics and thermohaline properties across almost the entire water column. The observatory is still ongoing, and the dataset is regularly updated. All the described data are publicly available from https://doi.org/10.17882/92236 (Borghini et al., 2022). They must therefore be preserved and are of considerable scientific interest.
- Article
(9500 KB) - Full-text XML
- BibTeX
- EndNote
The Mediterranean Sea is often seen as an incredible open-air laboratory ideal to study processes and ecosystems at different spatial and temporal scales. It is both a climate-change and biodiversity hotspot characterized by a high level of marine endemism but is also subject to a constant increase in anthropogenic pressures (e.g., fishing, including deep-sea fishing, aquaculture, tourism, exploration and production of oil and gas, coastal development) and their effects (e.g., eutrophication, climate change, proliferation of alien species).
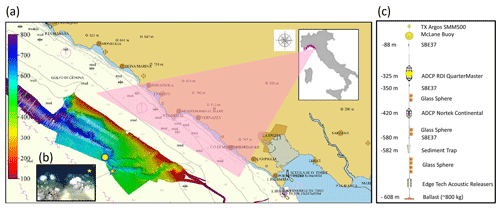
Figure 1Study area. Panel (a) represents the eastern Ligurian Sea with the superimposed multibeam survey (acquired in 2013 and 2014) of the Levante Canyon (from Delbono et al., 2014; Pratellesi et al., 2014). The yellow star indicates the site where dense populations of living, 1 m high colonies of Madrepora oculata (panel b, from Ciuffardi et al., 2020) were found by IIM and ENEA in 2014. The yellow circle represents the LCM mooring position: the mooring layout (not in scale) is described in panel (c).
The huge biological complexity characterizing marine communities makes the development of ecological monitoring increasingly indispensable beyond traditional time-consuming, high-cost sampling cruises, especially for the deep sea, where less information is available. Furthermore, identifying and quantifying the effect of different anthropogenic stressors on marine ecosystems requires an integrative and multidisciplinary approach (encompassing the simultaneous measurement of biogeochemical and oceanographic variables). For this purpose, there are many observational systems and initiatives for the study and monitoring of the Mediterranean that see Italian institutions as the main actors. One of these initiatives, in particular, is characterized by the collaboration, in terms of human resources, infrastructures and instruments, between the Ligurian DLTM (Ligurian District of Marine Technologies acting as the coordinator), CNR-ISMAR (National Research Council-Institute of Marine Sciences), ENEA (Italian National Agency for New Technologies, Energy and Sustainable Economic Development), IIM (Italian Navy – Hydrographic Institute) and INGV (National Institute of Geophysics and Volcanology). These institutions have developed an observatory composed of two stations, the first one deployed at 10 m depth in the Gulf of La Spezia, in Santa Teresa Bay (where a smart observatory is under development, https://smartbaysteresa.com/), and the second one installed in the Levant Canyon in the eastern Ligurian Sea (Fig. 1), the so-called Levante Canyon Mooring (LCM hereafter).
The coastal station monitors temperature, pressure, water conductivity and derived salinity (Bordone et al., 2022), and its main purpose is the study of coastal ecosystems, hydrodynamic processes and, in the long term, the effects of climate change. Moreover, the station hosts an experiment of plastic and bioplastic degradation in marine environments (De Monte et al., 2022).
The LCM provides temperature, salinity, pressure and horizontal currents in order to monitor the water dynamics in a Mediterranean subbasin, which is extremely interesting for different aspects: the eastern Ligurian Sea continental platform has a reduced extension and constitutes an exclusive marine observation point, not only because it is located inside the Pelagos Sanctuary, an area with a high concentration of cetaceans, but also because of the presence of the submarine Levante Canyon, an underwater canyon off Cinque Terre almost parallel to the coast, which, due to the intensified bottom currents and the considerable contribution of sediments and organic substances, creates an environment favorable to the development and growth of valuable ecosystems such as deep corals.
The installation of the LCM was aimed at investigating this particular deep-sea area, where a joint study by ENEA and IIM carried out in 2013–2014 with the support of a ROV (remotely operated vehicle) highlighted the presence of living colonies of Madrepora oculata (cold-water corals, CWCs) at a depth of about 570 m (see Fig. 1 and Delbono et al., 2014; Pratellesi et al., 2014; Fanelli et al., 2017). These organisms give rise to deep ecosystems with high biodiversity but suffer from a strong impact caused by trawling. It is well documented that these kinds of areas should be prioritized in monitoring (e.g., Canals et al., 2006; Thurber et al., 2014), as they play a fundamental role in shelf-slope connectivity and in the ecological status of continental margins.
The coastal dynamic of the Ligurian Sea is characterized by an east–west cyclonic flux composed of waters arriving from both sides of Corsica: warm waters coming from the Tyrrhenian Sea through the Corsica Channel and cold waters rising from the Western Mediterranean Current. Merging to the north of Corsica, these two circular fluxes generate a current with intermediate properties (Astraldi and Gasparini, 1992). Strong time (mainly seasonal) and spatial variations characterize eastern Ligurian circulation with complex circulation patterns, especially in summer. The area is characterized by several sources of frontogenesis and the development of mesoscale–submesoscale instabilities (Ciuffardi et al., 2016): on the one hand the interaction of coastal and shelf waters with the colder and saltier Northern Current (NC) at the basin scale (Astraldi and Gasparini, 1985) and on the other hand the interaction of coastal waters with buoyant river output, such as from the Arno and Magra rivers (Cattaneo Vietti et al., 2010; Schroeder et al., 2012). Wind forcing affects the upper-layer circulation as well and therefore the interaction between water masses (Astraldi and Gasparini, 1986; Poulain et al., 2020). The interplay of water masses at different scales generates mesoscale–submesoscale fronts and filaments which have been recognized as playing an important role in the surface dispersion and patchiness of various types of tracers (biological, pollutant and marine debris) and in the transfer from the surface to the interior ocean. The challenge in observing directly the submesoscale range, due to the high variability in both time and space, recently encouraged a transnational (Italian–French) multiplatform experiment in this same area under the framework of the JERICO-S3 project and the H2020 EU program (https://www.jerico-ri.eu/projects/jerico-s3/, last access: 18 April 2023). In this context, multiple observation platforms have been used to span across different scales and to provide comparable measurements (e.g., glider SeaExplorer, CTD probe, Ferrybox, CARTHE drifters). The area of interest is also monitored by a high-frequency radar network (CNR-ISMAR group, http://radarhf.ismar.cnr.it, last access: 18 April 2023) providing continuous (hourly) sea surface current maps covering medium ranges (about 40 km) with high resolution (approx. 1.5 km).
Despite a few studies focusing on the variability in the area at short temporal–spatial scales (Locritani et al., 2010; Schroeder et al., 2012; Berta et al., 2020; Poulain et al., 2020), there are no studies, to our knowledge, focusing also on deep hydrodynamics, due to a lack of targeted observations in the area. The extended time series presented in this work aims to fill this gap. Data were continuously collected between November 2020 and October 2022 and provide unique observations about the hydrodynamic processes of the site.
Data come from the LCM, a shared infrastructure located in the eastern Ligurian Sea (Fig. 1a) at around 600 m depth in the Levante Canyon, offshore the Cinque Terre Marine Protected Area at 44∘05.443′ N, 9∘29.900′ E (Ciuffardi et al., 2020). It was first deployed in October 2019, but the instrumented line accidentally detached from the mooring site and drifted toward the French coast, where instruments were collected to be finally redeployed in fall 2020. The LCM is a standalone offshore mooring dedicated to the long-term monitoring of hydrological properties of water masses. The installation and maintenance operations of the LCM are carried out thanks to the CNR R/V Dallaporta and the MM Leonardo operated by the Italian Navy, allowing the deep observatory to be positioned at about 6.5 nautical miles off the coast. The mooring operates in delayed mode and is equipped with sensors that measure physical and biogeochemical parameters along the water column from 83 to 580 m. The 530 m-long mooring scheme is represented in Fig. 1c. Starting from the bottom (Fig. 1c), the offshore monitoring station is equipped with a sediment trap placed at a depth of 582 m, which provides information on the supply of sediments and nutrients from the surface to the seabed. Further above, CTD probes are placed at three different depths at 579, 335 and 85 m, respectively. The LCM also includes two acoustic Doppler current profilers (ADCPs) placed at depths of 406 and 325 m, respectively, that measure currents throughout the water column in order to monitor the link between near-surface waters and deep ones.
Both the ADCP systems measure the intensities and directions of currents along the water column and have a temperature sensor in their transducer head. The CTD probe provides measurements of temperature and salinity (along with pressure). Acoustic releases guarantee the recovery by bringing the entire instrumented line back to the surface during ordinary or extraordinary maintenance. The LCM, still operative, is configured and maintained for continuous long-term monitoring. Ordinary maintenance operations are planned every 6–8 months, when the whole structure is recovered for instrumentation check, data download and maintenance (e.g., changing batteries and sediment trap bottles, cleaning, calibrating sensors or substituting components).
The upward-looking ADCP used is an RDI QuarterMaster (Teledyne RD Instruments USA, Poway, California) with a four-beam convex configuration with a beam angle of 20∘ and a working frequency of 153.6 kHz. The instrument is moored at a mean nominal depth of 325 m with the number of depth cells set to 44, a cell size of 8 m and a blanking distance of 3.5 m. The temperature sensor has the following characteristics: range −5 to 45 ∘C, precision ± 0.4 ∘C and resolution 0.01 ∘C.
The downward-looking ADCP used is a Nortek Continental (Nortek AS, Norway) with a three-beam convex configuration with a beam angle of 25∘ and a working frequency of 190 kHz. The instrument is moored at a mean nominal depth of 420 m with the number of depth cells set to 30, a cell size of 8 m and a blanking distance of 6.1 m. The temperature sensor has the following characteristics: range −4 to 40 ∘C, precision 0.1 ∘C and resolution 0.01 ∘C.
The velocity accuracies are ± 1 % and ± 0.5 cm s−1, and the sampling interval is 1 h for both the RDI and Nortek. The sound speed is computed by the pressure and temperature sensors embedded in the transducer head joint to the assumed salinity.
The three SBE37 CTDs deployed have the following characteristics for conductivity and temperature sensors, respectively: ranges 0 to 70 mS cm−1 and −5 to 35 ∘C, accuracies 0.003 mS cm−1 and 0.002 ∘C and resolutions 0.0001 mS cm−1 and 0.0001 ∘C. The probes are also equipped with a strain-gauge pressure sensor with an accuracy of ± 0.1 % of the full-scale range.
2.1 Dataset and metadata description
The collection is composed of five datasets containing observational data and related metadata from the LCM mooring site for the period November 2020–October 2022. Five files, two for ADCP data (in NetCDF format) and three for CTD data (in CSV format), have been submitted, and each file description specifies the probe and its depth (Borghini et al., 2022). Time series of ADCP echo intensities have also been included in the submitted dataset (https://doi.org/10.17882/92236, Borghini et al., 2022) stored in the NetCDF files.
The metadata-report Dataset Information (DI) contains a brief summary description of the dataset and details about its geospatial position, temporal extension and data interval, the institution responsible for measurements, the principal investigator name and contact, the observational network to which the mooring belongs and the keywords vocabulary used. The Variables in Dataset (VD) contain specific information about the data structure and variables. The ADCP dataset provides details about the station name, the geographical position, the time coverage, the bottom depth, the cell depth/range and the current components. The ADCP and CTD variables in the dataset are reported as quality-controlled results of the quality-control (QC) procedure reported in the next chapter. The headers of filtered ADCP variables are followed by the suffix “_QC” and the appropriate flags by the suffix “_QC_flag”.
Mooring deployment and maintenance operations took place regularly twice a year (i.e., on 2 October 2021, 21 June 2022 and 15 October 2022). Table 1 reports all the most significant meta-information about each sensor.
2.2 Data quality check
All data from the LCM, after maintenance operations, are subjected to a quality-validation system according to international protocols and standards (IOC and Commission of the European Communities of UNESCO, 1993).
A first visual check of CTD data time series was first applied in order to detect spikes and anomalous values. This was made possible after a statistical analysis of our dataset on the property (i.e., temperature and salinity gradient) distribution and frequency to identify the proper thresholds. After these quality checks, the CTD at 88 m was cut off in the plots in mid-March 2022, disregarding conductivity data (and consequently salinity and density) that were affected by fouling artifacts or calibration issues. Temperature data at 88 m were assumed to be reliable up to the beginning of August 2022. To avoid similar issues in future deployments, the probe drift will be regularly verified and calibrated by conducting vertical profiles using a SeaBird SBE19 plus calibrated probe.
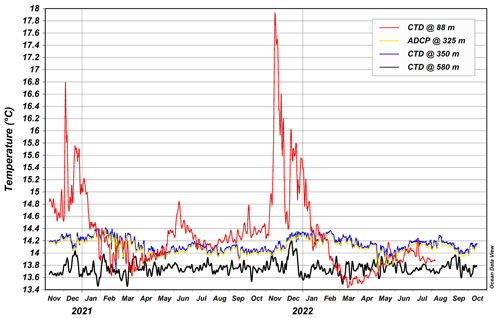
Figure 3CTD and ADCP temperature records on the LCM site. The data are presented with a 3 d temporal smoothing window.
Table 3Statistical parameters of the 3 d temporal averaged temperatures vs. months at the LCM site. SD stands for standard deviation. Both ADCP and CTD data are reported for the depths 325 and 350 m, respectively. The mean difference between temperature measured by ADCP and CTD is in the range 0.033–0.051 ∘C.
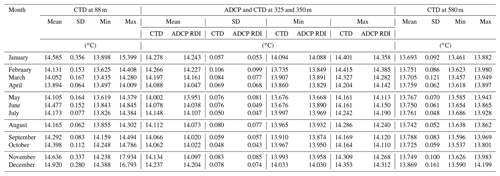
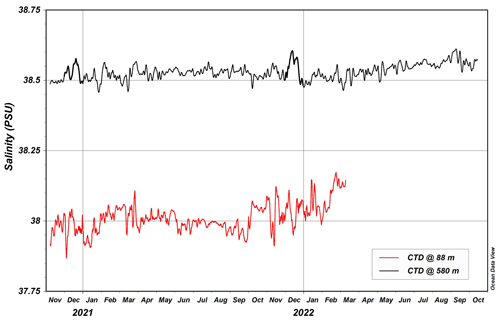
Figure 4CTD salinity records on the LCM site. The data are presented with a 3 d temporal smoothing window.
For the RDI ADCP, the adopted QC procedure was based principally on the Manual for Real-Time Quality Control of In-Situ Current Observations by IOOS (2019) along with the Crout and Conlee (2006) report by the NOAA. Four groups of tests have been applied. (a) The sensors' overall health includes tests for the sensors' tilt and speed-of-sound control. Pressure-sensor extremes for in and out of water checks, along with the sensors' overall health and a temperature-despiking test, are also applied. (b) The signal-quality test controls the quality of the transmitting/receiving signal and includes the correlation-magnitude (CM) test and the percentage-good (PG) ratio. (c) The current velocity tests ensure the validity of the measured current and include the horizontal and vertical velocity along with the error velocity controls. (d) The overall profile tests control the echo-amplitude (intensity) test.
The analysis and processing of the Nortek data are done using the SURGE program provided by Nortek (https://www.nortekgroup.com/softwarelicense/surge, last access: 18 April 2023). For the postprocessing, the configuration is the following. (a) The Sidelobe rejection is set to 90 %, and by that, as it is downward-oriented, it neglects data near the bottom. (b) The low signal-to-noise-ratio (SNR) threshold is set up to 3 dB. (c) Removal of tilt effects is allowed. (d) The velocity-variation test neglects data of over 5 standard deviations (SDs). (e) The echo-spike test limit is set up to 70 dB. Also, a temperature-despiking test is applied as in the RDI dataset.
Both RDI and the Nortek ADCP data are postprocessed using a first-level QC. Figure 2 shows RDI time series of pitch, roll and tilt for reference.
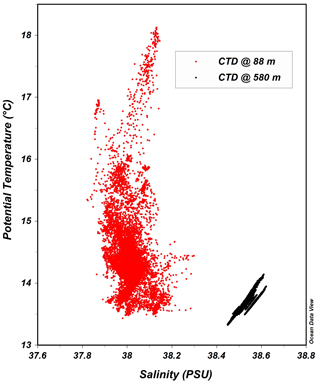
Figure 5Temperature–salinity (T–S) diagram for the LCM site, obtained from CTD measurements covering the period November 2020–October 2022.
A set of flags is used to describe the results of the QC. The OceanSites and Copernicus Marine In Situ flag scales were adapted, simplified and adjusted for a delayed mode of operation for flagging the data (Copernicus In Situ TAC, 2021). Table 2 explains the flags used in all the datasets (https://www.nortekgroup.com/softwarelicense/surge).
The first 2-year dataset of the LCM is presented here.
Hydrological data collected by the mooring and presented here (data from November 2020 to October 2022) include measurements conducted with both current meters and CTD probes and provide information about the hydrodynamics and thermohaline properties across almost the entire water column. These data, presented below, constitute fundamental knowledge for the understanding of the ecological conditions that apply to the CWC habitat and the conditions that favor the coral settlement in the Levante Canyon. Hydrological conditions along the water columns, through seasons and at the sea bottom are for the first time acquired in a deep observatory for long-term monitoring of the health status of the Madrepora oculata ecosystem. Future work will integrate the observed hydrodynamic trends together with the LCM sediment trap data so that the interactions between the oceanography, sediment input, biogeochemistry and spatial distribution of CWC biological communities give an interdisciplinary overview and a deep understanding of the Levante Canyon system functioning.
3.1 Thermohaline records
Temperature records measured by the RDI ADCP (at 325 m depth) and by the three CTD probes (at 88, 350 and 580 m depth) are presented here to compare data at different depths along the water column. Figure 3 shows the temporal sequence resulting from November 2020 to October 2022 across the canyon, considering both the CTD and ADCP measurements as regards temperature records around 325–350 m depth. The data are presented with a 3 d smoothing window. Temperature data recorded at 420 m depth by the Nortek ADCP have been disregarded as being too high due to electronic issues. In fact, the instrument batteries discharged earlier than scheduled due to probable overheating. By contrast, it is interesting to note that the two time series at 325 m (ADCP) and 350 m (CTD) depth have synchronous fluctuations and slight differences. Temperature peaks are evident during winter 2020 and 2021, covering the upper water column around 88 m water depth. By contrast, the intermediate and deeper layers show fewer oscillations, even if some variability and colder periods are present. Interestingly, the measurements cover the period during spring–summer 2022, characterized by the exceptional heat wave that began in the second half of April 2022 and mainly overheated the central and northwestern parts of the Mediterranean. This heat wave is not so evident from our temperature records.
In the upper layer of the Levante Canyon, the temperature recorded by the CTD at 88 m has a mean value of 14.33 ± 0.35 ∘C with a minimum temperature of 13.44 ∘C in March 2022 and a maximum temperature of 17.93 ∘C in November 2021. At 325 m, the ADCP highlighted an average temperature of 14.10 ± 0.11 ∘C with a minimum temperature of 13.67 ∘C in May 2022 and a maximum temperature of 14.39 ∘C in February 2021, in accordance with the nearby CTD positioned at 350 m depth (mean temperature of 14.14 ± 0.11 ∘C, minimum temperature of 13.68 ∘C in May 2022 and maximum temperature of 14.42 ∘C in February 2021).
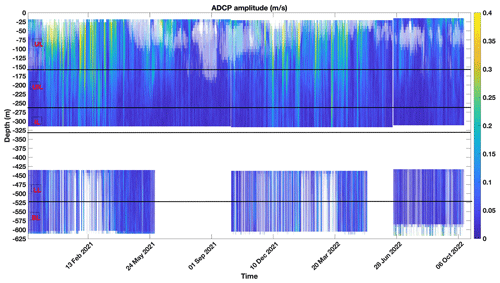
Figure 6Current speed records (m s−1) along the LCM water column (data filtered as described in the data quality-check section); boxes on the left and straight lines indicate the five different layers assumed in the analysis (UL: upper layer; UIL: upper intermediate layer; IL: intermediate layer; LIL: lower intermediate layer; BL: bottom layer).
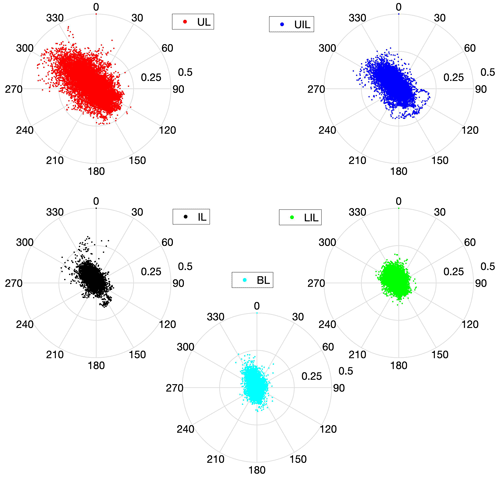
Figure 7Polar scatter plot of observed directional current velocity (m s−1) at the LCM site (UL, UIL, IL, LIL, BL).
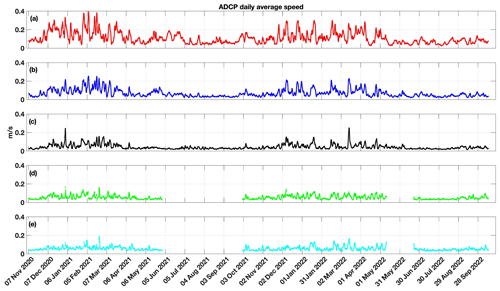
Figure 8Time series of the current speed (m s−1) in the five different layers assumed in the analysis by applying a daily average smoothing (UL, UIL, IL, LIL, BL).
The mean difference between temperatures measured by ADCP and CTD is in the range 0.03–0.05 ∘C (Table 3). In the deepest part of the mooring, near the bottom, at 580 m depth, the mean recorded temperature is 13.76 ± 0.10 ∘C with a minimum temperature of 13.46 ∘C in March 2021 and a maximum temperature of 14.20 ∘C in December 2021.
The first CTD (from the top in the mooring layout) at 88 m depth represents well the seasonal cycle and the interannual variability in the first ∼100 m of the water column, where temperature changes are more evident. In particular, the temperatures experienced high peaks during the fall to early winter months: this is the result of a periodic annual cycle where warmer surface waters, after the summer heating, experienced strong vertical mixing from November until January, producing a period of vertical homogeneity of the surface layers (at least up to 88 m according to the measurements). Wind episodes from late summer through fall contribute to this vertical mixing. By contrast, solar radiation in the spring and summer periods results in the development of water column stratification with greater differences in the annual temperature variation at the sea surface compared with that in the underlying layers (at 88 m). The only exception looks like early summer 2021, when temperatures in the upper layer still oscillate, proving some interannual variability.
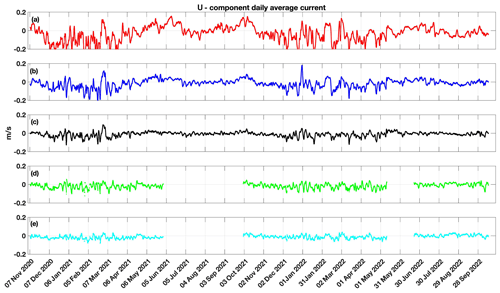
Figure 9Time series of the eastern component of the current speed (m s−1) in the five different layers assumed in the analysis by applying a daily average smoothing (UL, UIL, IL, LIL, BL).
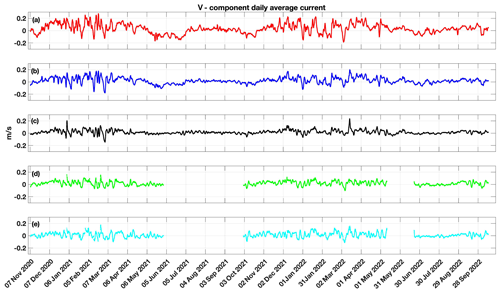
Figure 10Time series of the northern component of the current speed (m s−1) in the five different layers assumed in the analysis by applying a daily average smoothing (UL, UIL, IL, LIL, BL).
With regards to salinity measurements, the latest data from the CTD probe at 88 m and all the data from the CTD probe at 350 m were not plotted as they failed QC due to calibration issues. Hence, the data at 88 m depth end in March 2022 in Fig. 4. In general, seasonal variability shows the necessity of having regular maintenance operations for proper salinity measurements. The salinity is lower in the upper layer of the Levante Canyon, where the salinity recorded by the CTD at 88 m has a mean value of 38.01 ± 0.05 PSU with a minimum of 37.87 PSU and a maximum of 38.17 PSU. Near the bottom, at 580 m depth, the time series of salinity shows intermediate values with respect to the upper and deeper parts: the mean recorded salinity is 38.54 ± 0.03 PSU with a minimum of 38.48 PSU and a maximum of 38.61 PSU. Again, larger oscillations are evident for the upper layer at 88 m depth, while salinity values are less variable in the deepest part of the canyon (Fig. 4).
The potential temperature–salinity (T–S) diagram of the whole dataset is shown in Fig. 5. The large variability at 88 m indicates the complex water mass structure subjected to the strong seasonal influence of heat and water exchanges with the atmosphere, while close to the bottom there is a close correlation indicating relatively stable water masses at these depths in the region, with values in the ranges 13.3–14.1 ∘C and 38.4–38.6 PSU at 580 m depth. These values are consistent with the characteristics of the surface water of Atlantic origin (Atlantic Water, AW; upper 150 m) and of the Levantine Intermediate Water (LIW; from about 200 to 700 m depth) recorded for the western Mediterranean (Iacono et al., 2021; Fedele et al., 2022) and Ligurian Sea (Margirier et al., 2020; Prieur et al., 2020). Statistics about temperature and salinity records grouped by months are reported in Tables 3 and 4.
3.2 Hydrodynamic records
This section reports the hydrodynamic data measured by the two ADCPs along the water column at the LCM site from 2020 to 2022. For better visualization, in order to better solve the dynamic variability of the water column, data have been separated into five vertical layers of approximately 100 m each: UL (upper layer) 25–150 m, UIL (upper intermediate layer) 150–250 m, IL (intermediate layer) 250–350 m, LIL (lower intermediate layer) 400–500 m and BL (bottom layer) 500–600 m. For each layer, polar scatter diagrams have been plotted, with speed sorted every 0.2 m s−1.
Figure 6 shows the 2-year-long ADCP records as vertical distributions of the speed module along the water column, while polar scatter plots are reported in Fig. 7, which represent the direction and intensity of currents along the water column. Current data show an average weak hydrodynamic field at the BL (0.06 ± 0.02 m s−1) able to reach a speed of 0.76 m s−1 at about 50 m water depth during events of strong currents. From Fig. 6, these currents are generally recorded from winter to early spring (between December and April) every year and affect the whole water column. Stronger currents also occurred during summer 2021 but remained confined to the UL and UIL. The hydrodynamic field of the five selected layers is characterized by currents spreading between 120 and 180∘ N and between 270 and 330∘ N. This behavior indicates a flow oriented toward the canyon axis (see Fig. 1). A northward-prevailing current component is evidenced by the reported scatter plots close to the bottom: in the two deeper layers (i.e., LIL and BL), this directional spreading slightly rotates assuming a north–south orientation. This is in accordance with the role of the topography that in a deep canyon acts to modify the current direction close to the bottom.
Figure 8 shows the time series of the five layers by applying a daily average smoothing. Focusing on the U (eastern) and V (northern) components from the ADCP (Figs. 9 and 10), the time series shows a reversal of the U component in summer/early fall for both 2021 and 2022. In the June–July period especially, the U component shows a significant positive sign (from west to east), while the typical Northern Current (NC) negative sign (from east to west) prevails during winter, also associated with a larger V magnitude compared to the summer period. The NC episodic and local reversal during summer has also been observed at the surface (1 m depth) by current maps from the CNR-ISMAR high-frequency radar network covering the area nearby the mooring since 2016 (http://radarhf.ismar.cnr.it/, last access: 18 April 2023). The future combination of these datasets gives the opportunity to further investigate the origin of the reversal, the coherence of the signal throughout the water column (also considering the typical strongly stratified waters profile in summer) and the extent to which the current reversal affects the canyon dynamics.
All data are made publicly available through https://www.seanoe.org/data/00810/92236/ (last access: 18 April 2023). The registered database DOI is https://doi.org/10.17882/92236 (Borghini et al., 2022).
This paper describes in detail the temporal coverage of the dataset, which is constituted by a quite continuous high-temporal-resolution time series of currents, temperature and salinity from November 2020 to October 2022. The adopted methodology about settings, data records and quality-control procedures ensures the compliance and consistency of the dataset. The dataset presented here ends in October 2022, but monitoring activities are still in progress, and future data collected by this deep observatory will be added to an updated version of the repository at least every 2 years.
This paper presents the results of the first 2 years of data acquired on the Levante Canyon Mooring conducted in the eastern Ligurian Sea throughout the installation in 2020 of a mooring line placed in a canyon area at about 600 m depth characterized by the presence of cold-water living corals. This activity was realized thanks to a multidisciplinary framework, where some major national research centers (CNR-ISMAR, ENEA, IIM and INGV) cooperate under the coordination of the DLTM.
The LCM, equipped with two ADCPs, three CTD probes and a sediment trap, provides valuable and original hydrodynamic and thermohaline measurements along the water column (approx. from 50 m depth to the bottom) at a deep canyon site. The LCM initiative can represent an innovative regional center where members can calibrate, compare and test instrumentations or share tools favoring sustainable technological development in the marine and maritime transport sectors. Furthermore, it can link the various regional monitoring systems already in existence in both the Tyrrhenian Sea and Ligurian Sea regions, contributing to create a regional network of experimental marine stations. This will meet national and international demand for the protection of the marine environment and its potential for innovation and growth (Marine Strategy and Blue Growth). Its data can nourish studies about human impact on coastal and port areas to stimulate the implementation of European directives and/or national/regional laws (e.g., ecosystem approach and environmental status evaluation).
Analyzed data extended from 2020 to 2022 and are characterized by a seasonal cycle in the first ∼100 m of the water column with strong vertical mixing during fall and winter against water column stratification in spring and summer. This was also stressed by Picco et al. (2010) in their work on the Ligurian Sea, where they described a vertical thermal structure in the upper thermocline characterized by high variability during winter related to the presence of the Ligurian front, the occurrence of internal waves and the wind mixing.
The time series covers the period of summer 2022 characterized by the exceptional heat wave impacting the central and northwestern parts of the Mediterranean. In this period, the ESA-funded project CAREHeat (https://eo4society.esa.int/projects/careheat/) reports that the Ligurian Sea was particularly affected as the peak of the heat wave reached its surface waters in late July, with temperatures of 5 ∘C above the average, and, despite a slight drop in temperatures at the end of August, the heat wave was still evident in the measurements of September. From the temperature data at the LCM site, this heat wave is not so evident in the upper layer, where the temperatures are lower than those of summer 2021, whereas a slightly positive increase is recorded for the lower CTD records.
From a hydrological point of view, water mass distribution resulting from the T–S diagrams at the LCM site is coherent with previous evidence in the Ligurian Sea: the canyon area is characterized by the presence of two main water masses: (1) surface water of Atlantic origin (Atlantic Water, AW; upper 150 m), well separated from the underlying intermediate water, and (2) the Levantine Intermediate Water (LIW) that occupies the layer between 200 m and 500–700 m depth. This is something that should be accurately verified during the next data-recovery and probe calibrations, even if already previously documented by Prieur et al. (2020) as regards coastal and frontal zones of the Ligurian Sea.
The first 2-year time series is presented here, but further detailed studies and long-term series of geophysical and hydrological data are necessary to better understand the bottom dynamics, the seabed and water column interaction and the ecological conditions of valuable ecosystems in the Levante Canyon and in the Ligurian Sea more in general, a challenging area for geological, geophysical, oceanographic and ecological research.
Conceptualization was done by TC, ZK and MaBe. Field measurements and data management were carried out by MiB, AB, MD and ZK. Data processing and analysis was performed by TC and ZK. TC prepared the manuscript with contributions from all the coauthors. All the authors have read and agreed to the published version of the manuscript.
The contact author has declared that none of the authors has any competing interests
Publisher's note: Copernicus Publications remains neutral with regard to jurisdictional claims in published maps and institutional affiliations.
The authors are deeply indebted to the captains and crews of the CNR R/V Dallaporta and MM Leonardo for continuous support during the whole of the measurement/deployment phases.
This research has been supported by the Distretto Ligure delle Tecnologie Marine (DLTM) through funding obtained from Regione Liguria (PAR-FSC 2007–2013 funds) and by institutional funds from Consiglio Nazionale delle Ricerche – Istituto di Scienze Marine (CNR-ISMAR), Agenzia nazionale per le nuove tecnologie, l’energia e lo sviluppo economico sostenibile (ENEA), Istituto Idrografico della Marina (IIM) and Istituto Nazionale di Geofisica e Vulcanologia (INGV).
This paper was edited by Xingchen (Tony) Wang and reviewed by Paola Picco and one anonymous referee.
Astraldi, M. and Gasparini, G. P.: Elements for a comparison between the Tyrrhenian and Ligurian coastal currents during spring, Nuovo Cimento C, 8, 842–850, https://doi.org/10.1007/BF02558014, 1985.
Astraldi, M. and Gasparini, G. P.: Observation of pressure and wind fields over the Ligurian and Tyrrhenian Seas (West Mediterranean), Meteorol. Atmos. Phys., 35, 139–148, https://doi.org/10.1007/BF01026170, 1986.
Astraldi, M. and Gasparini, G. P.: The seasonal characteristics of the circulation in the north Mediterranean basin and their relationship with the atmospheric-climatic conditions, J. Geophys. Res.-Oceans, 97, 9531–9540, https://doi.org/10.1029/92JC00114, 1992.
Berta, M., Corgnati, L., Magaldi, M. G., Griffa, A., Mantovani, C., Rubio, A., Reyes, E., and Mader, J.: Small scale ocean weather during an extreme wind event in the Ligurian Sea, in: Copernicus Marine Service Ocean State Report, Issue 4, J. Oper. Oceanogr., 13, 149–155, https://doi.org/10.1080/1755876X.2020.1785097, 2020.
Bordone, A., Ciuffardi, T., Delbono, I., Pannacciulli, F., Raiteri, G., Locritani, M., Muccini, F., Cocchi, L., Demarte, M., Ivaldi, R., Berta, M., Borghini, M., Vetrano, A., Caprino, G., Marini, D., and Schierano, E.: LabMare Coastal station: A shallow water cabled observatory in the Eastern Ligurian Sea, SEANOE [data set], https://doi.org/10.17882/87643, 2022.
Borghini, M., Bordone, A., Ciuffardi, T., Kokkini, Z., Demarte, M., Ivaldi, R., Delbono, I., Berta, M., Locritani, M., Vetrano, A., Raiteri, G., Muccini, F., Pannacciulli, F., Marini, D., and Caprino, G.: Moored current and temperature measurements in a submarine canyon of the Eastern Ligurian Sea, November 2020–October 2022, SEANOE [data set], https://doi.org/10.17882/92236, 2022.
Canals, M., Puig, P., De Madron, X. D., Heussner, S., Palanques, A., and Fabres, J.: Flushing submarine canyons, Nature, 444, 354–357, https://doi.org/10.1038/nature05271, 2006.
Cattaneo Vietti, R., Albertelli, G., Aliani, S., Bava, S., Bavestrello, G., Benedetti Cecchi, L., Bianchi, C. N., Bozzo, E., Capello, M., Castellano, M., Cerrano, C., Chiantore, M., Corradi, N., Cocito, S., Cutroneo, L., Diviacco, G., Fabiano, M., Faimali, M., Ferrari, M., Gasparini, G. P., Locritani, M., Mangialajo, L., Marin, V., Moreno, M., Morri, C., Orsi Relini, L., Pane, L., Paoli, C., Petrillo, M., Povero, P., Pronzato, R., Relini, G., Santangelo, G., Tucci, S., Tunesi, L., Vacchi, M., Vassallo, P., Vezzulli, L., and Wurtz, M.: The Ligurian Sea: present status, problems and perspectives, Chem. Ecol., 26, 319–340, https://doi.org/10.1080/02757541003689845, 2010.
Ciuffardi, T., Napolitano, E., Iacono, R., Reseghetti, F., Raiteri, G., and Bordone, A.: Analysis of surface circulation structures along a frequently repeated XBT transect crossing the Ligurian and Tyrrhenian Seas, Ocean Dynam., 66, 767–783, https://doi.org/10.1007/s10236-016-0954-y, 2016.
Ciuffardi, T., Berta, M., Bordone, A., Borghini, M., Celentano, P., Cocchi, L., De Fabritiis, L., Delbono, I., Delfanti, R., Demarte, M., Ivaldi, R., Kokkini, Z., Locritani, M., Marini, D., Marini, S., Muccini, F., Pannacciulli, F., Peirano, A., Raiteri, G., and Vetrano, A.: A new multidisciplinary observatory in the Eastern Ligurian Sea (NW Mediterranean Sea): a combination of deep-sea and coastal measurements, EGU General Assembly 2020, Online, 4–8 May 2020, EGU2020-16533, https://doi.org/10.5194/egusphere-egu2020-16533, 2020.
Copernicus Marine In Situ TAC: Copernicus Marine In Situ NetCDF format manual, Copernicus Marine In Situ Tac Data Management Team, https://doi.org/10.13155/59938, 2021.
Crout, R. L. and Conlee, D. T.: Quality Control of Minerals Management Service – Oil Company ADCP Data at NDBC: A Successful Partnership Implementation, in: Proceedings of the Marine Technology Society and the Inst. of Electrical and Electronics Engineers (MTS/IEEE) OCEANS Conference and Exhibition, Boston, MA USA, 18–21 September 2006, 1–5, https://doi.org/10.1109/OCEANS.2006.307072, 2006.
De Monte, C., Locritani, M., Merlino, S., Ricci, L., Pistolesi, A., and Bronco, S.: An In Situ Experiment to Evaluate the Aging and Degradation Phenomena Induced by Marine Environment Conditions on Commercial Plastic Granules, Polymers, 14, 1111, https://doi.org/10.3390/polym14061111, 2022.
Delbono, I., Ivaldi, R., Pratellesi, M., Fanelli, E., Peirano, A., Cocito, S., Dialti, L., and Delfanti, R.: Seafloor morphology: nature of the seabed and the cold water corals of the Levante Canyon (eastern Ligurian Sea, NW Mediterranean), EGU General Assembly 2014, Vienna, Austria, 27 April–2 May 2014, Geophysical Research Abstracts Vol. 16, EGU2014-14732, 2014.
Fanelli, E., Delbono, I., Ivaldi, R., Pratellesi, M., Cocito, S., and Peirano, A.: Cold-water coral Madrepora oculata in the eastern Ligurian Sea (NW Mediterranean): historical and recent findings, Aquatic Conserv.-Mar. Freshw. Ecosyst., 27, 965–975, https://doi.org/10.1002/aqc.2751, 2017.
Fedele, G., Mauri, E., Notarstefano, G., and Poulain, P. M.: Characterization of the Atlantic Water and Levantine Intermediate Water in the Mediterranean Sea using 20 years of Argo data, Ocean Sci., 18, 129–142, https://doi.org/10.5194/os-18-129-2022, 2022.
Iacono, R., Napolitano, E., Palma, M., and Sannino, G.: The Tyrrhenian Sea Circulation: A Review of Recent Work, Sustainability, 13, 6371, https://doi.org/10.3390/su13116371, 2021.
Intergovernmental Oceanographic Commission (IOC) and Commission of the European Communities of UNESCO: Manual of Quality Control Procedures for Validation of Oceanographic Data, Manual And Guides 26, UNESCO, https://unesdoc.unesco.org/ark:/48223/pf0000138825.locale=en (last access: 18 April 2023), 1993.
IOOS: Manual for Real-Time Quality Control of In-Situ Current Observations. Version 2.1, U. S. Integrated Ocean Observing System, Silver Spring, MD, U. S. Department of Commerce, National Oceanic and Atmospheric Administration, National Ocean Service, Integrated Ocean Observing System, 54, https://doi.org/10.25923/sqe9-e310, 2019.
Locritani, M., Chiappini, M., Chiarabini, R., Bruni, F., Ciccarelli, V., Natale, L., and De Pauli, R.: Hydrological and physical characterization of Cinque Terre Marine Protected Area (Ligurian Sea) and evaluation of current velocity and direction by AUV navigation tracks, OCEANS 2010 MTS/IEEE SEATTLE, Seattle, WA, 20–23 September 2010, 1–8, https://doi.org/10.1109/OCEANS.2010.5664052, 2010.
Margirier, F., Testor, P., Heslop, E., Mallil, K., Bosse, A., Houpert, L., Mortier, L., Bouin, M.-N., Coppola, L., D'Ortenzio, F., de Madron, X. D., Mourre, B., Prieur, L., Raimbault, P., and Taillandier, V.: Abrupt warming and salinification of intermediate waters interplays with decline of deep convection in the Northwestern Mediterranean Sea, Sci. Rep.-UK, 10, 20923, https://doi.org/10.1038/s41598-020-77859-5, 2020.
Picco, P., Cappelletti, A., Sparnocchia, S., Schiano, M. E., Pensieri, S., and Bozzano, R.: Upper layer current variability in the Central Ligurian Sea, Ocean Sci., 6, 825–836, https://doi.org/10.5194/os-6-825-2010, 2010.
Poulain, P. M., Mauri, E., Gerin, R., Chiggiato, J., Schroeder, K., Griffa, A., Borghini, M., Zambianchi, E., Falco, P., Testor, P., and Mortier, L.: On the dynamics in the southeastern Ligurian Sea in summer 2010, Cont. Shelf Res., 196, 104083, https://doi.org/10.1016/j.csr.2020.104083, 2020.
Pratellesi, M., Ivaldi, R., and Delbono, I.: Dual use Hydrographic Surveys for Seabed Nature and Morphological Research. Integrated Mapping of Seabed Features, Hydro International, 18, 26–29, 2014.
Prieur, L., D'Ortenzio, F., Taillandier, V., and Testor, P.: Physical Oceanography of the Ligurian Sea, chapter 3, in: The Mediterranean Sea in the Era of Global Change 1, edited by: Migon, C., Nival, P., and Sciandra, A., John Wiley & Sons, Ltd., https://doi.org/10.1002/9781119706960.ch3, 2020.
Schlitzer, R.: Ocean Data View [software], https://odv.awi.de (last access: 18 April 2023), 2021.
Schroeder, K., Chiggiato, J., Haza, A. C., Griffa, A., Özgökmen, T. M., Zanasca, P, Molcard, A., Borghini, M., Poulain, P. M., Gerin, R., Zambianchi, E., Falco, P., and Trees, C.: Targeted Lagrangian sampling of submesoscale dispersion at a coastal frontal zone, Geophys. Res. Lett., 39, L11608, https://doi.org/10.1029/2012GL051879, 2012.
The MathWorks, Inc.: MATLAB [software], version: 9.4.0.813654 (R2018a), Natick, Massachusetts, https://www.mathworks.com (last access: 18 April 2023), 2018.
Thurber, A. R., Sweetman, A. K., Narayanaswamy, B. E., Jones, D. O. B., Ingels, J., and Hansman, R. L.: Ecosystem function and services provided by the deep sea, Biogeosciences, 11, 3941–3963, https://doi.org/10.5194/bg-11-3941-2014, 2014.