the Creative Commons Attribution 4.0 License.
the Creative Commons Attribution 4.0 License.
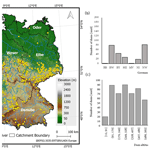
Inventory of dams in Germany
Gustavo Andrei Speckhann
Heidi Kreibich
Bruno Merz
Dams are an important element of water resources management. Data about dams are crucial for practitioners, scientists, and policymakers for various purposes, such as seasonal forecasting of water availability or flood mitigation. However, detailed information on dams on the national level for Germany is so far not freely available. We present the most comprehensive open-access dam inventory for Germany (DIG) to date. We have collected and combined information on dams using books, state agency reports, engineering reports, and internet pages. We have applied a priority rule that ensures the highest level of reliability for the dam information. Our dam inventory comprises 530 dams in Germany with information on name, location, river, start year of construction and operation, crest length, dam height, lake area, lake volume, purpose, dam structure, and building characteristics. We have used a global, satellite-based water surface raster to evaluate the location of the dams. A significant proportion (63 %) of dams were built between 1950–2013. Our inventory shows that dams in Germany are mostly single-purpose (52 %), 53 % can be used for flood control, and 25 % are involved in energy production. The inventory is freely available through GFZ (GeoForschungsZentrum) Data Services (https://doi.org/10.5880/GFZ.4.4.2020.005) (Speckhann et al., 2020).
- Article
(2847 KB) - Full-text XML
- BibTeX
- EndNote
Dams are an important element of water resources management. Their main function is to store water in order to balance variations in streamflow and in demand of water and energy (World Commission on Dams, 2000). Most daily activities rely, to some extent, on the existence of dams: natural hazard mitigation (flood and drought control), energy production (hydropower plants), food production (irrigation), transport of goods (inland navigation), and the daily water used in our houses and industries (water supply) (Bertoni et al., 2019; Gernaat et al., 2017; Moran et al., 2018; Poff and Olden, 2017; Sordo-Ward et al., 2012, 2013; Tullos et al., 2009).
Dams are classified as multi-purpose dams (i.e. the same structure is used for more than one purpose like water supply and flood control) or single-purpose dams. Multi-purpose dams can address multiple conflicting demands at different times; i.e. dams may have one purpose (e.g. water supply) during normal hydrological conditions and have another purpose during high-precipitation periods in order to serve as a buffer for flood attenuation (Quinn et al., 2019). The ability to perform adequately for different tasks presents multi-purpose dams as a potential answer to multiple societal challenges (Zarfl et al., 2019).
Dam operation is a challenge, especially due to the complexities and the nonlinearity of the operations which may be driven by anthropogenic and environmental influences (Gutenson et al., 2020). For instance, a flood event was aggravated due to the opening of floodgates of upstream reservoirs in combination with extreme precipitation downstream in 2018 in the Kerala region in India (Lal et al., 2020). In addition to such complexities, dam operators are compelled to make decisions in the short term (Mediero et al., 2007). Simple operating rules may not be ideal for a proper representation of the flow downstream, and to assume normal hydroclimatic conditions while considering a restricted number of operating objectives has its limitations (Giuliani et al., 2016). On the other hand, nonlinear operating policies are not trivial to understand, so many dam operators tend to apply simpler rules, even though they may not provide the best answer for multiple-purpose conflicts (Quinn et al., 2019).
According to the International Commission on Large Dams (ICOLD, 2019), the majority of dams throughout the world serve irrigation purposes, in terms of both single-purpose and multi-purpose dams. Irrigation represents the largest consumptive use of fresh water and is used on approximately 20 % of the world's agricultural land (World Commission on Dams, 2000). Hydropower dams, the second most common application, produce 72 % of global renewable energy (Gernaat et al., 2017). Many countries aim to expand their hydropower potential in the near future (Bertoni et al., 2019; Timpe and Kaplan, 2017; Zarfl et al., 2014), especially due to its advantage in producing energy only when the electrical system demands it (Zarfl et al., 2019). Pacific Asia, South America, and Africa have a significant potential for the construction of new hydropower plants (Gernaat et al., 2017; Timpe and Kaplan, 2017), while in Europe and North America, the current number of dams being built is smaller than the number of dams being removed (Moran et al., 2018).
In Germany, the first dams, i.e. fish and mill ponds with a small reservoir volume, are documented from the end of the eighth century (Bettzieche, 2010). The construction of dams gained importance when energy was needed for mining in the Harz and Ore mountains in the mid-16th century. Modern dam construction began with the Eschbach Dam (1891) and was driven by the goal of the industry in the low mountain range to remain competitive with the newly emerging industry in the Ruhr area (Bettzieche, 2010). Later, other dam purposes such as flood control, recreation, and water supply became much more important. Most of the dams in Germany were built between 1950 and 2000 (Deutsche TalsperrenKomitee e.V., 2013).
The construction of dams can lead to negative socio-economic and environmental consequences (Best, 2019; Zarfl et al., 2019). The relocation of people and conflicts regarding water use at different catchment locations can have a long-term effect on communities. Dams, especially cascades of dams, can be a threat to biodiversity and a driver of species extinction (Winemiller et al., 2016) and are responsible for geochemical cycle modifications at the global level (Maavara et al., 2020). Dams can aggravate stress on rivers' streamflow by altering the hydrological regime (Bond et al., 2008; Zarfl et al., 2019; Zhang et al., 2019) and by modifying sediment fluxes along the river (Maavara et al., 2020; Manh et al., 2015; Winemiller et al., 2016). For instance, in Brazil it is estimated that both the Paraná basin and the Tocantins basin have a bedload trap of approximately 80 % (Latrubesse et al., 2005). In the Amazon River, one of the most explored areas for hydropower plants, a reduction of 20 % of the suspended sediment has been observed (Latrubesse et al., 2017). Global estimations indicate that the majority of large dams have a theoretical sediment trapping efficiency of higher than 50 % (Vörösmarty et al., 2003).
Information and data about dams are crucial for dam operators, scientists, and policymakers (Hecht et al., 2019; Tullos et al., 2009), for instance, for the assessment of hydropower plant construction (Bertoni et al., 2019; Gernaat et al., 2017; Moran et al., 2018; Winemiller et al., 2016), estimation of the hydrological footprint (Bakken et al., 2013, 2014; Popescu et al., 2020; Postel, 2000), seasonal forecasting of water availability and water levels in rivers for navigation (Zhang et al., 2019), flood and drought risk assessment and management (Di Baldassarre et al., 2017; Ehsani et al., 2017; Elmer et al., 2012; Metin et al., 2018; Veldkamp et al., 2017), and assessment of biotic disturbances (Latrubesse et al., 2017; Maavara et al., 2020; Sabo et al., 2017). The consideration of dams in hydrological models improves the model performance downstream (Gutenson et al., 2020), but the lack of information on dams may jeopardize model applications, especially at a national level (Zhao et al., 2020).
Important information about dams comprises the location of the dam, the lake area, and volume. Often it is also important to know when a dam was constructed or went into operation (and out of operation). The purpose of a dam is commonly known, but dam operation rules are hardly available in dam databases. Dam operations are normally complex and influenced by environmental and anthropogenic factors (Gutenson et al., 2020). Many studies have explored the effects of dam operation rules; however, operation rules are not easily available for science, especially those of private hydropower plants (Bertoni et al., 2019; Giuliani et al., 2016; Mediero et al., 2007; Pan et al., 2014; Quinn et al., 2019).
In 1972, the congress of the United States of America authorized the first nationwide survey on dams, which was conducted by the US Army Corps of Engineers (US Army Corps of Engineers, 1975). This was, to our knowledge, the first dam inventory conducted at a national level. Initially, the motivation was to collect data on the dam hazard level (i.e. likely life loss in case of dam failure), height, and storage capacity. This initiative continues until today, and the database currently has information on more than 90 000 dams.
The Global Reservoir and Dam – GRanD – database (Lehner et al., 2011) was the first database to provide global information about dams with a detailed level of information concerning location (municipality, country), dam height, surface area, purpose, dam crest, and elevation. The first version was made publicly available in 2011 with 6862 dams, and an updated version was released in 2019 containing more than 7000 dams. Recently, the GlObal geOreferenced Databased of Dams – GOODD (Mulligan et al., 2020) – was published, containing more than 38 000 dams (and their catchments) across the whole globe. For the German territory, the GRanD database contains 60 dams, while GOODD contains 142 dams. As an outcome of the Sustainable Development Goal 6.6.1 the UN has freely provided GeoTIFF files for all administrative boundaries and basins indicating water surface presence between the period of 2000–2018 at a resolution of 30 m. The products available are the result of a joint cooperation between the European Commission's Joint Research Centre and Google Earth. OpenStreetMap (OSM) is also a freely available online tool that contains information about dams globally. Through http://www.overpass-turbo.eu/ (last access: 25 February 2021), a web-based tool for filtering OSM, one can easily identify the location of dams.
The objective of this work is to provide, as comprehensively as possible, open-source information on dams in Germany. We have compiled data from multiple sources, such as federal-agency reports or scientific publications. Our main source was the German Committee on Large Dams (https://www.talsperrenkomitee.de, last access: 25 February 2021), especially their book published in 2013 which contains information on 340 dams (Deutsche TalsperrenKomitee e.V., 2013). We collected information about 530 dams in Germany and individually identified their location using satellite images from Google Earth. The database was evaluated via a comparison with the available dam databases GRanD (Lehner et al., 2011) and GOODD (Mulligan et al., 2020) and the Sustainable Development Goal 6 product (Pekel et al., 2016). Our database contains significantly more dams than the compilation of the German Committee on Large Dams and combines different sources of information to provide detailed information that is herewith made freely available.
2.1 Data compilation procedure
There are no agreed standards regarding the collection of dam data. At a global level, there are only two open-access data publications on dams (Lehner et al., 2011; Mulligan et al., 2020). In order to create a national dam inventory for Germany (DIG), we followed six steps (Fig. 1). The first step was to search for information about dams across a variety of sources: books, journals, federal-agency reports, and web pages. We searched for any reference in English or German that may lead to some information about dams in Germany. We have searched for any reference to “dams” and/or “Germany” in libraries and on internet search engines. This yielded a considerable number of possible sources. The most comprehensive source was the book Talsperren in Deutschland (Deutsche TalsperrenKomitee e.V., 2013) from where a significant part of the data in this inventory were obtained.
In the second step, the dams were geolocated using satellite images. Only dams that could be identified visually were included in our database. The identification was carried out using Google Earth, based on the combination of the name of the dam and the city where it was located. For those cases where a dam could not be identified, additional information on the dam location was used to increase the chance of finding it (e.g. name of the river, neighbourhood, image).
All identified dams were included in the inventory with their name and coordinates (step 3). The dam coordinates were a condition sine qua non for the inclusion of the dam in the inventory. We have not established a threshold for the inclusion, e.g. based on dam height or lake volume, but we have added only dams for which we could provide reliable information. As such, we kept the database as useful as possible for different purposes.
In the fourth step different sources of information were combined to add the missing data to each dam entry. In many cases the information was in agreement between different sources. In the case of conflicting information, the following hierarchy of information sources was applied taking into consideration the perceived reliability of the sources: (1) book of dams in Germany (Deutsche TalsperrenKomitee e.V., 2013), (2) state agency reports (e.g. Landesanstalt für Umwelt Baden-Württemberg), (3) other dam databases (Lehner et al., 2011; Mulligan et al., 2020), (4) engineering reports, and (5) web pages. In order to have as much information as possible for each dam, the search was an iterative process, where the priority rule was applied during every round.
The fifth step was the evaluation of the dam's location, and it was conducted as a validation. We used the location and persistence of surface water obtained from the analysis of Landsat satellite images (Pekel et al., 2016) as a proxy for the presence of the dam's location. Using GIS software, we compared the location of the dam with the water surface occurrence by looking at the intersection of the dam buffer and the water surface presence. We used the latest available grid information on surface water, downloaded from the United Nations platform on Sustainable Development Goal 6.6 (https://www.sdg661.app/downloads, last access: 25 February 2021), to indicate the presence of water and therefore confirm that a water surface was spotted at the dam's location.
The last step was to make the inventory publicly available at GeoForschungsZentrum (GFZ) Data Services (http://dataservices.gfz-potsdam.de/portal/, last access: 25 February 2021), where a shapefile and tab-delimited file containing all information can be downloaded.
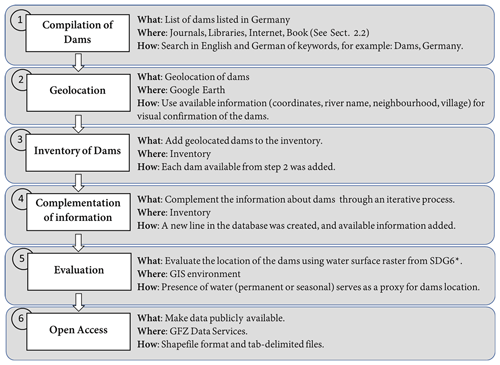
Figure 1Procedure for the compilation of the dam inventory. * Sustainable Development Goal 6 app (https://www.sdg661.app/data-products/data-downloads, last access: 25 February 2021).
2.2 Data sources
The information available was classified into five main sources as described under step 4 and listed in detail in Table 1. The book of dams in Germany (Deutsche TalsperrenKomitee e.V., 2013) is the most detailed information source providing technical information for 350 dams in Germany. State agency reports with dam information are available from all states in Germany, except for Saarland, Rhineland-Palatinate, Schleswig-Holstein, and Mecklenburg-Western Pomerania. A possible explanation for that fact is the smaller number of dams in those states. On the other hand, Baden-Württemberg, North Rhine-Westphalia, and Bavaria provide dam information on the websites of their environmental agencies. The two global databases tend to focus on big dams using automated algorithms for reservoir identification based on topography. The GRanD database by Lehner et al. (2011) is the second most complete set of dam information for Germany, containing information of geolocation, dam height, purpose, and lake area for 60 dams in Germany. GOODD (Mulligan et al., 2020) covers 142 dams in Germany but contains no information apart from the geolocation and watershed size. The global databases are the only information that can be used directly in a GIS environment and provide coordinates for each dam. The information apart from that of the global databases is only available as document pages that cannot be exported to a user-friendly format; the use of this information requires copying the information manually for each dam. Some of the information from state agency files (tables, texts) is protected and does not allow any selection. Engineering reports are normally available for more recent dams or for dams that have been under restoration or retrofitting, e.g. to increase dam capacity. Web pages are also a source of dam information. However, verifiable references or links are often missing, and in some occasions differences in naming the dams occurred. Hence, we decided to avoid web pages as the main information source of a dam. Their information was used as complementary data and was combined with other sources. Table 1 presents the most relevant sources of information used to compile the DIG.
3.1 Data contents
Our dam inventory contains the following: name of the dam, date when the construction and operation started, state, river, length of the crest, height of the wall, area of the lake at full capacity, volume at full capacity, dam type, building characteristics, and geolocation (Table 2). Information on the type, building characteristics and purpose is given using abbreviations; a table containing these abbreviations is provided in the folder for download. We have compiled the most comprehensive dam inventory for Germany; however, we believe that there are more dams in Germany that for many reasons were not identified via our procedure. Hence, there is room for expanding our inventory.
3.2 Data characteristics
Our dam inventory comprises information about 530 dams which are in operation in Germany (Fig. 2). Saxony is the state with the highest number of dams in our inventory with a total of 190 dams. North Rhein-Westphalia is the second one with 83 dams, followed by Baden-Württemberg with 79. For the city states of Berlin, Bremen, and Hamburg no dams were found. Brandenburg (2), Mecklenburg-Western Pomerania (2), Rhineland-Palatinate (4), Saarland (3), and Schleswig-Holstein (1) have a small number of dams (Fig. 2). These states are located at a lower elevation. The majority of dams in the remaining states of Thuringia (43), Bavaria (44), Saxony-Anhalt (32), Hesse (28), and Lower Saxony (19) are located at an altitude between 100 and 500 m. The number of dams is a function of the data availability; i.e. states may have more dams on their territory, but the information was not publicly available or the dam was not detected by satellite image algorithms. We do not claim to have compiled an exhaustive list of all dams in Germany.
Our inventory contains 276 single-purpose dams and 254 multi-purpose dams. Flood control (HWS) is the most common purpose (Fig. 3a) often in combination with other purposes. Only 36 % of 284 dams with a flood control function are used exclusively for flood control. Recreation (NEG) is the second most common purpose with 179 dams, and the majority (86 %) are multi-purpose dams. Energy production (E) is also widespread with 131 dams. A total of 23 dams are used for nature protection (NSG). In order to assure a minimum level of navigability on waterways, especially during summertime, 62 dams are used to regulate the river water level for transport reasons (NWA). Our inventory contains 130 dams for water supply (TWv) which are spread across the country. Industrial and agricultural water supply (BWv) are an important component for food (e.g. irrigation) and energy production (e.g. cooling of nuclear power plants) and other industry-related activities with 91 dams.
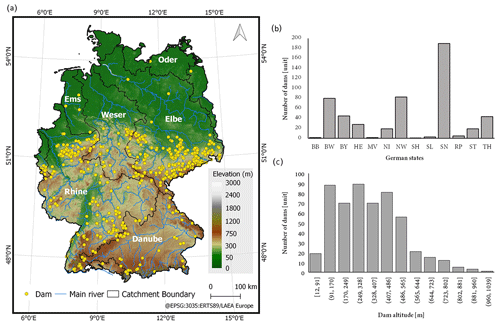
Figure 2Dam locations across Germany (a), number of dams in the German states (b), and dam altitude (c). State abbreviations are BB (Brandenburg), BW (Baden-Württemberg), BY (Bavaria), HE (Hesse), MV (Mecklenburg-Western Pomerania), NI (Lower Saxony), NW (North Rhein-Westphalia), SH (Schleswig-Holstein), SL (Saarland), SN (Saxony), RP (Rhineland-Palatinate), ST (Saxony-Anhalt), and TH (Thuringia).
The building characteristics are dominated by three types: embankment dams, gravity dams, and rockfill dams (Fig. 3b). Embankment dams (EDDs) are by far the most frequent type (289 dams, 54 %), followed by gravity dams (97 dams, 18 %) and rockfill dams (75 dams, 14 %). No relation was observed between the building characteristics and the states.
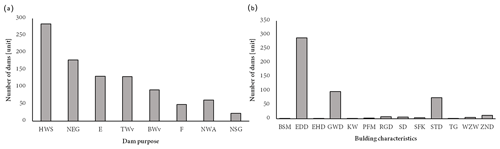
Figure 3Dam purpose (a) and building characteristics (b). Purposes are abbreviated as BWv (industrial agricultural water supply), E (energy production), F (fishing), HWS (flood control), NEG (recreational), NSG (nature protection), NWA (transport), and TWv (water supply). Building characteristics are abbreviated as BSM (arch dam), EDD (embankment dam), EHD (homogenous rockfill dam), GWD (gravity dam), KW (flap weir), PFM (buttress dam), RGD (ring dam), SD (fill dam), SFK (segment with fish belly flap), STD (rockfill dam), TG (residual lake associated with mining), WZW (rolling weir), and ZND (zone dam).
After the Second World War, many countries initiated the construction of dams with a particularly strong increase in Europe and the USA between 1950 and 2000 (Moran et al., 2018). This trend is also seen in Germany (Fig. 4). Between 1950 and 2000 more than 300 dams were constructed, but only 20 dams after 2000 were built. The reasons for this reduction are twofold: (1) the decrease in available locations where dams can be constructed and (2) the chance of structural failure associated with the dam existence and operation.
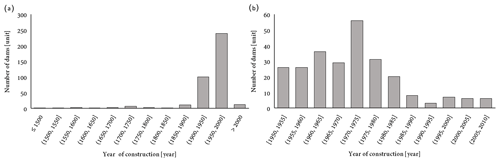
Figure 4Construction year of the dams in Germany (a) and a subplot for the period between 1950–2015 (b).
Dam height is one of the criteria used to classify dams. The International Commission on Large Dams (ICOLD, 2011) defines dams with heights above 15 m as large dams. A total of 266 dams (50 %) in our inventory are considered large dams (Fig. 5b). We could not observe a relation between dam height and the states.
There are 231 dams (43 %) with a lake volume smaller than 1×106 m3. Dams tend to be decentralized; i.e. there tend to be several smaller dams which may act together, instead of one massive dam, within a region. The DIG has 11 dams with a lake volume higher than 100×106 m3, and the majority of them were constructed for energy production and flood control.
3.3 Evaluation of location of dams
Dam location is central in our inventory, so we evaluated it using global data. We used water surface raster information for the year 2018 (Pekel et al., 2016) as a proxy for the location of active dams. This raster is derived from Landsat images; however, they may not be able to correctly identify dams with a lake area smaller than 0.3 km2 and lake width smaller than 30 m (Lehner et al., 2011; Mulligan et al., 2020). For each dam in the inventory, we created a buffer of 200 m around a point and used that polygon as a mask to extract the values from the 30 m resolution water surface raster. The raster contains three possible states: “permanent water”, “seasonal water”, and “no water”. Each dam location was investigated for the presence of permanent water or seasonal water for the year 2018.
From the 530 dams in the inventory, 81 dams did not exhibit the presence of water (permanent or seasonal). Of those dams, 63 are classified as single-purpose dams for flood control. The majority of the remaining dams (14 out of 18 dams) are used for flood control together with other purposes and have a lake area smaller than the threshold for lake identification (0.3 km2). As single-purpose flood control dams are only used in flood situations, it is expected that most of them are not identified by a satellite-derived water surface product for a given year, as they may not have experienced a flood situation in that year or due to cloud interference during floods. Thus, we believe that our evaluation of the dam location is sufficient for compiling the dam inventory.
The data can be freely downloaded via GFZ Data Services at https://doi.org/10.5880/GFZ.4.4.2020.005 (Speckhann et al., 2020).
We have compiled an open-access inventory of dams at the national level in Germany. This information can be used by scientists, practitioners, and policymakers for a range of purposes, such as flood and drought risk assessment and management or assessment of biotic disturbances. We have built the most comprehensive inventory of dams in Germany by providing the name of the dam, the name of the river, and technical specifications (dam height, crest length, building characteristics, purpose, and type) as well as the dam geolocation. With information about 530 dams, our inventory comprises almost 1.5 times the number of dams than the second most comprehensive source, i.e. the book of the German Committee on Large Dams. As states in Germany advance their use of digitalization together with public administrative transparency, we believe that there will be opportunities for the DIG to be updated and extended in the future, in respect of both the number of dams and the amount of information. Further useful extensions of the inventory may also include dams in upstream regions of the Elbe, Rhine, and Danube catchments outside Germany, since these also influence the hydrology in Germany. The DIG consists of a shapefile format which enables the easy use of this information in GIS environments and modelling applications and also a tab-delimited file that can be easily imported for other uses.
GAS, HK, and BM designed the study, and GAS carried it out. GAS prepared the manuscript with valuable contributions from all co-authors.
The authors declare that they have no conflict of interest.
The first author would like to thank the German Academic Exchange Service (DAAD) for financial support during the research.
This research has been supported by the Deutscher Akademischer Austauschdienst (grant no. 57399578).
This paper was edited by David Carlson and reviewed by Benjamin Fersch and one anonymous referee.
Bakken, T. H., Killingtveit, Å., Engeland, K., Alfredsen, K., and Harby, A.: Water consumption from hydropower plants – review of published estimates and an assessment of the concept, Hydrol. Earth Syst. Sci., 17, 3983–4000, https://doi.org/10.5194/hess-17-3983-2013, 2013.
Bakken, T. H., Aase, A. G., Hagen, D., Sundt, H., Barton, D. N., and Lujala, P.: Demonstrating a new framework for the comparison of environmental impacts from small- and large-scale hydropower and wind power projects, J. Environ. Manage., 140, 93–101, https://doi.org/10.1016/j.jenvman.2014.01.050, 2014.
Di Baldassarre, G., Martinez, F., Kalantari, Z., and Viglione, A.: Drought and flood in the Anthropocene: feedback mechanisms in reservoir operation, Earth Syst. Dynam., 8, 225–233, https://doi.org/10.5194/esd-8-225-2017, 2017.
Bertoni, F., Castelletti, A., Giuliani, M., and Reed, P. M.: Discovering Dependencies, Trade-Offs, and Robustness in Joint Dam Design and Operation: An Ex-Post Assessment of the Kariba Dam, Earths Future, 7, 1367–1390, https://doi.org/10.1029/2019EF001235, 2019.
Best, J.: Anthropogenic stresses on the world's big rivers, Nat. Geosci., 12, 7–21, https://doi.org/10.1038/s41561-018-0262-x, 2019.
Bettzieche, V.: 100 Jahre technische entwicklung des talsperrenbaus in Deutschland, Wasserwirtschaft, 1–2, 67–72, 2010 (in German).
Bond, N. R., Lake, P. S., and Arthington, A. H.: The impacts of drought on freshwater ecosystems: An Australian perspective, Hydrobiologia, 600, 1–14, https://doi.org/10.1007/s10750-008-9326-z, 2008.
Deutsche TalsperrenKomitee e. V.: Talsperren in Deutschland, 1st ed., edited by DTK, Springer Vieweg, Dresden, 2013 (in German).
Ehsani, N., Vörösmarty, C. J., Fekete, B. M., and Stakhiv, E. Z.: Reservoir operations under climate change: Storage capacity options to mitigate risk, J. Hydrol., 555, 435–446, https://doi.org/10.1016/j.jhydrol.2017.09.008, 2017.
Elmer, F., Hoymann, J., Düthmann, D., Vorogushyn, S., and Kreibich, H.: Drivers of flood risk change in residential areas, Nat. Hazards Earth Syst. Sci., 12, 1641–1657, https://doi.org/10.5194/nhess-12-1641-2012, 2012.
Gernaat, D. E. H. J., Bogaart, P. W., Vuuren, D. P. V., Biemans, H., and Niessink, R.: High-resolution assessment of global technical and economic hydropower potential, Nat. Energy, 2, 821–828, https://doi.org/10.1038/s41560-017-0006-y, 2017.
Giuliani, M., Castelletti, A., Pianosi, F., Mason, E., and Reed, P. M.: Curses, tradeoffs, and scalable management: Advancing evolutionary multiobjective direct policy search to improve water reservoir operations, J. Water Resour. Plan. Manag., 142, 1–17, https://doi.org/10.1061/(ASCE)WR.1943-5452.0000570, 2016.
Gutenson, J. L., Tavakoly, A. A., Wahl, M. D., and Follum, M. L.: Comparison of generalized non-data-driven lake and reservoir routing models for global-scale hydrologic forecasting of reservoir outflow at diurnal time steps, Hydrol. Earth Syst. Sci., 24, 2711–2729, https://doi.org/10.5194/hess-24-2711-2020, 2020.
Hecht, J. S., Lacombe, G., Arias, M. E., Dang, T. D., and Piman, T.: Hydropower dams of the Mekong River basin: A review of their hydrological impacts, J. Hydrol., 568, 285–300, https://doi.org/10.1016/j.jhydrol.2018.10.045, 2019.
ICOLD: Constitution of ICOLD, Int. Comm. Large Dams, 2, 1–21, available at: http://www.icold-cigb.org/userfiles/files/CIGB/INSTITUTIONAL_FILES/Constitution2011.pdf (last access: 24 February 2021), 2011.
ICOLD: International Commision on Large Dams – General Synthesis, available at: https://www.icold-cigb.org/GB/world_register/general_synthesis.asp (last access: 20 May 2020), 2019.
Lal, P., Prakash, A., Kumar, A., Srivastava, P. K., Saikia, P., Pandey, A. C., Srivastava, P., and Khan, M. L.: Evaluating the 2018 extreme flood hazard events in Kerala, India, Remote Sens. Lett., 11, 436–445, https://doi.org/10.1080/2150704X.2020.1730468, 2020.
Latrubesse, E. M., Stevaux, J. C., and Sinha, R.: Tropical rivers, Geomorphology, 70, 187–206, https://doi.org/10.1016/j.geomorph.2005.02.005, 2005.
Latrubesse, E. M., Arima, E. Y., Dunne, T., Park, E., Baker, V. R., D'Horta, F. M., Wight, C., Wittmann, F., Zuanon, J., Baker, P. A., Ribas, C. C., Norgaard, R. B., Filizola, N., Ansar, A., Flyvbjerg, B., and Stevaux, J. C.: Damming the rivers of the Amazon basin, Nature, 546, 363–369, https://doi.org/10.1038/nature22333, 2017.
Lehner, B., Liermann, C. R., Revenga, C., Vörömsmarty, C., Fekete, B., Crouzet, P., Döll, P., Endejan, M., Frenken, K., Magome, J., Nilsson, C., Robertson, J. C., Rödel, R., Sindorf, N., and Wisser, D.: High-resolution mapping of the world's reservoirs and dams for sustainable river-flow management, Front. Ecol. Environ., 9, 494–502, https://doi.org/10.1890/100125, 2011.
Maavara, T., Chen, Q., Van Meter, K., Brown, L. E., Zhang, J., Ni, J., and Zarfl, C.: River dam impacts on biogeochemical cycling, Nat. Rev. Earth Environ., 1, 103–116, https://doi.org/10.1038/s43017-019-0019-0, 2020.
Manh, N. V., Dung, N. V., Hung, N. N., Kummu, M., Merz, B., and Apel, H.: Future sediment dynamics in the Mekong Delta floodplains: Impacts of hydropower development, climate change and sea level rise, Glob. Planet. Change, 127, 22–33, https://doi.org/10.1016/j.gloplacha.2015.01.001, 2015.
Mediero, L., Garrote, L., and Martín-Carrasco, F.: A probabilistic model to support reservoir operation decisions during flash floods, Hydrol. Sci. J., 52, 523–537, https://doi.org/10.1623/hysj.52.3.523, 2007.
Metin, A. D., Dung, N. V., Schröter, K., Guse, B., Apel, H., Kreibich, H., Vorogushyn, S., and Merz, B.: How do changes along the risk chain affect flood risk?, Nat. Hazards Earth Syst. Sci., 18, 3089–3108, https://doi.org/10.5194/nhess-18-3089-2018, 2018.
Moran, E. F., Lopez, M. C., Moore, N., Müller, N., and Hyndman, D. W.: Sustainable hydropower in the 21st century, Proc. Natl. Acad. Sci. USA, 115, 11891–11898, https://doi.org/10.1073/pnas.1809426115, 2018.
Mulligan, M., van Soesbergen, A., and Sáenz, L.: GOODD, a global dataset of more than 38,000 georeferenced dams, Sci. Data, 7, 1–8, https://doi.org/10.1038/s41597-020-0362-5, 2020.
Pan, T. Y., Chang, L. Y., Lai, J. S., Chang, H. K., Lee, C. S., and Tan, Y. C.: Coupling typhoon rainfall forecasting with overland-flow modeling for early warning of inundation, Nat. Hazards, 70, 1763–1793, https://doi.org/10.1007/s11069-011-0061-9, 2014.
Pekel, J. F., Cottam, A., Gorelick, N., and Belward, A. S.: High-resolution mapping of global surface water and its long-term changes, Nature, 540, 418–422, https://doi.org/10.1038/nature20584, 2016.
Poff, N. L. R. and Olden, J. D.: Can dams be designed for sustainability?, Science, 358, 1252–1253, https://doi.org/10.1126/science.aaq1422, 2017.
Popescu, V. D., Munshaw, R. G., Shackelford, N., Montesino Pouzols, F., Dubman, E., Gibeau, P., Horne, M., Moilanen, A., and Palen, W. J.: Quantifying biodiversity trade-offs in the face of widespread renewable and unconventional energy development, Sci. Rep.-UK, 10, 1–12, https://doi.org/10.1038/s41598-020-64501-7, 2020.
Postel, S. L.: Entering an era of water scarcity: The challenges ahead, Ecol. Appl., 10, 941–948, https://doi.org/10.1890/1051-0761(2000)010[0941:EAEOWS]2.0.CO;2, 2000.
Quinn, J. D., Reed, P. M., Giuliani, M., and Castelletti, A.: What Is Controlling Our Control Rules? Opening the Black Box of Multireservoir Operating Policies Using Time-Varying Sensitivity Analysis, Water Resour. Res., 55, 5962–5984, https://doi.org/10.1029/2018WR024177, 2019.
Sabo, J. L., Ruhi, A., Holtgrieve, G. W., Elliott, V., Arias, M. E., Ngor, P. B., Räsänen, T. A., and Nam, S.: Designing river flows to improve food security futures in the Lower Mekong Basin, Science, 358, eaao1053, https://doi.org/10.1126/science.aao1053, 2017.
Sordo-Ward, A., Garrote, L., Martín-Carrasco, F., and Dolores Bejarano, M.: Extreme flood abatement in large dams with fixed-crest spillways, J. Hydrol., 466–467, 60–72, https://doi.org/10.1016/j.jhydrol.2012.08.009, 2012.
Sordo-Ward, A., Garrote, L., Bejarano, M. D., and Castillo, L. G.: Extreme flood abatement in large dams with gate-controlled spillways, J. Hydrol., 498, 113–123, https://doi.org/10.1016/j.jhydrol.2013.06.010, 2013.
Speckhann, G. A., Kreibich, H., and Merz, B.: Inventory of dams in Germany, V. 1.0, GFZ Data Services, https://doi.org/10.5880/GFZ.4.4.2020.005, 2020.
Timpe, K. and Kaplan, D.: The changing hydrology of a dammed Amazon, Sci. Adv., 3, 1–14, https://doi.org/10.1126/sciadv.1700611, 2017.
Tullos, D., Tilt, B., and Liermann, C. R.: Introduction to the special issue: Understanding and linking the biophysical, socioeconomic and geopolitical effects of dams, J. Environ. Manage., 90, 1–5, https://doi.org/10.1016/j.jenvman.2008.08.018, 2009.
US Army Corps of Engineers: National program of inspection of dams, [Department of Defense], Department of the Army, Office of the Chief of Engineers, Washington, DC, available at: https://books.google.de/books?id=1QtaKF0RiJgC (last access: 24 February 2021), 1975.
Veldkamp, T. I. E., Wada, Y., Aerts, J. C. J. H., Döll, P., Gosling, S. N., Liu, J., Masaki, Y., Oki, T., Ostberg, S., Pokhrel, Y., Satoh, Y., Kim, H., and Ward, P. J.: Water scarcity hotspots travel downstream due to human interventions in the 20th and 21st century, Nat. Commun., 8, 1–12, https://doi.org/10.1038/ncomms15697, 2017.
Vörösmarty, C. J., Meybeck, M., Fekete, B., Sharma, K., Green, P., and Syvitski, J. P. M.: Anthropogenic sediment retention: Major global impact from registered river impoundments, Glob. Planet. Change, 39, 169–190, https://doi.org/10.1016/S0921-8181(03)00023-7, 2003.
Winemiller, K. O., McIntyre, P. B., Castello, L., Fluet-Chouinard, E., Giarrizzo, T., Nam, S., Baird, I. G., Darwall, W., Lujan, N. K., Harrison, I., Stiassny, M. L. J., Silvano, R. A. M., Fitzgerald, D. B., Pelicice, F. M., Agostinho, A. A., Gomes, L. C., Albert, J. S., Baran, E., Petrere, M., Zarfl, C., Mulligan, M., Sullivan, J. P., Arantes, C. C., Sousa, L. M., Koning, A. A., Hoeinghaus, D. J., Sabaj, M., Lundberg, J. G., Armbruster, J., Thieme, M. L., Petry, P., Zuanon, J., Torrente Vilara, G., Snoeks, J., Ou, C., Rainboth, W., Pavanelli, C. S., Akama, A., Van Soesbergen, A., and Sáenz, L.: Balancing hydropower and biodiversity in the Amazon, Congo, and Mekong, Science, 351, 128–129, https://doi.org/10.1126/science.aac7082, 2016.
World Commission on Dams: Dams and development: A new framework for decision-making Overview of the report by the World Commission on Dams, Routledge, London, 446 pp., 2000.
Zarfl, C., Lumsdon, A. E., Berlekamp, J., Tydecks, L., and Tockner, K.: A global boom in hydropower dam construction, Aquat. Sci., 77, 161–170, https://doi.org/10.1007/s00027-014-0377-0, 2014.
Zarfl, C., Berlekamp, J., He, F., Jähnig, S. C., Darwall, W., and Tockner, K.: Future large hydropower dams impact global freshwater megafauna, Sci. Rep.-UK, 9, 1–10, https://doi.org/10.1038/s41598-019-54980-8, 2019.
Zhang, Y., Zheng, H., Herron, N., Liu, X., Wang, Z., Chiew, F. H. S., and Parajka, J.: A framework estimating cumulative impact of damming on downstream water availability, J. Hydrol., 575, 612–627, https://doi.org/10.1016/j.jhydrol.2019.05.061, 2019.
Zhao, G., Bates, P., and Neal, J.: The Impact of Dams on Design Floods in the Conterminous US, Water Resour. Res., 56, 1–15, https://doi.org/10.1029/2019WR025380, 2020.